- 1Instituto de Investigaciones Oceanológicas, Universidad Autónoma de Baja California, Ensenada, Mexico
- 2Escuela Superior de Ingeniería Química e Industrias Extractivas, Instituto Politécnico Nacional, Mexico City, Mexico
- 3Instituto de Ingeniería, Universidad Nacional Autónoma de México, Mexico City, Mexico
Introduction
The needs of modern coastal communities exert high pressure on the ecosystem services that are naturally provided nearby, putting their future socio-economic development at risk. Over the last hundred years, the population and the economy of the coastal states of Mexico have been growing rapidly due to internal migration. It is estimated that by 2030 the total population of Mexico´s coastal states will have reached 60.1 million (Azuz-Adeath et al., 2019). Satisfying the demand for essential services (energy, water, and food security), maintaining ecosystem functionality, and the socioeconomic activities of its communities is a huge challenge. In Mexico, it is estimated that approximately 32% of the population lives in “energy poverty” or has poor quality electricity, making it impossible for them to improve their quality of life (García-Ochoa and Graizbord, 2016). A significant number of these communities are located near the coasts and are vulnerable to climate change, so their adaptation is a priority (Masson-Delmotte et al., 2021).
Renewable energies include many promising options that can mitigate global warming by reducing our dependence on fossil fuels (IRENA, 2021). Diversification and modernization of the energy matrix, improving affordability and efficiency, are of great importance, and marine renewable energies (MREs) can play a crucial role in this. The 1.5% increase in global installed renewable capacity in 2020 was 2.54 TW (representing 35.7% of global installed capacity). Although quite promising, efforts still need to be accelerated to reach the targets to mitigate average carbon emissions by 3.5% per year and reach net zero by 2050 (IRENA, 2021; Europa Publications, 2022) (IRENA, 2021). The reserves of MRE are enormous, with vast potential in the seas and oceans around the world that could enable coastal communities to foster their social and environmental resilience through the development of the blue economy. The creation of multipurpose clusters of MRE systems and coastal industries, such as desalination and aquaculture, could generate many beneficial by-products that improve energy, water, and food security and stimulate the commercialization and competitiveness of the emerging MRE sector (LiVecchi et al., 2019). However, techno-economic challenges, such as the commercial-scale performance, and the high levelized cost of electricity (LCOE) related to higher capital, maintenance, and operating expenses, are obstacles to the deployment of MRE hybrid projects (Babarit et al., 2018). Furthermore, there are other challenges, related to the uncertainties of the potential impacts MRE deployment could have on the coastal environment, society, and the economy (Martínez et al., 2021).
This work evaluates the sustainability criteria of marine energy harnessing through an interdisciplinary approach that identifies the main technical, environmental, social, and economic components to foster the deployment of marine renewable technologies in coastal areas of Mexico.
Off-grid hybrid systems for marine renewable energy harvesting
Mexico has a coastline of around 12,000 km, where a variety of MREs offer significant energy potential for 91–100% of the time, such as Thermal Gradient Energy (TGE), Salinity Gradient Energy (SGE), Ocean Currents Energy (OCE) with 0.5–1 kW/m2, Tidal Energy (TE), Wave Power (WP) with 10–20 kW/m, and Offshore Wind Energy (OWE) (Hernández-Fontes et al., 2019; Posada-Vanegas et al., 2019). These energy resources compare relatively well with other renewable energies (Garduño-Ruiz et al., 2021).
Depending on their physical nature, weather, and climatic conditions, the various types of MREs have different degrees of inter- and intra-annual variability in their spatio-temporal average energy availability. For example, in latitudes where TGE is feasible there is generally low intra-annual variability because the mean sea temperature is practically constant at depth, 800–1,000 m, and can be considered as “base load”. On the other hand, WP is strongly related to the areas where the waves originate, meaning high seasonal dependence and mean annual variability. Figure 1A) shows the monthly variability of three MREs, solar and wind energy in Cozumel and Baja California, Mexico. It can be seen that TGE has low monthly variability compared to ocean currents, wave energy, or solar energy. It is important to note that in regions where the minimum potential for wave energy generation is present, it coincides with the maximum potential of other renewable resources that can be harvested, as solar or ocean currents. Power systems that combine renewable energies could make it possible to reduce monthly variations, allowing a more providing continuous electricity generation throughout the year. For such continuous operation, the harnessing of the MRE can be co-localized with other renewable energy sources, non-renewable energy sources, or energy storage technologies.
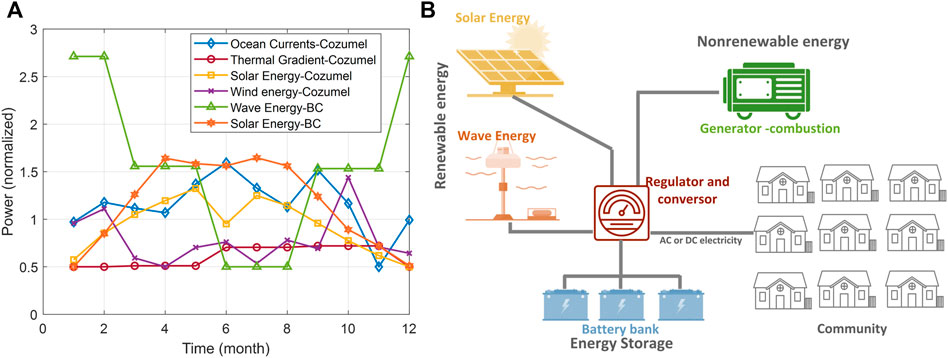
FIGURE 1. (A) Mean monthly variability for different MREs in Cozumel and Baja California. Data taken from CEMIE-Oceano research (Garduño-Ruiz et al., 2021; Gorr-Pozzi et al., 2021; Olmedo-González et al., 2021; Tobal-Cupul et al., 2022). Note: The power units are “normalized” because the graph only represents the power variation considering a minimum power generation of 0.5 units. (B) An off-grid, hybrid energy system for isolated communities.
The combination of different energy sources in an off-grid system is known as hybridization, and these promising options would thus become economically more viable and, therefore, more attractive to investors. The main function of hybridization is to compensate for daily and monthly fluctuations in electricity generation. However, since up to 60% of the total cost of an off-grid system comes from the energy storage system, it is vital to determine the optimal size and the right degree of hybridization (EERE, 2021; Olmedo-González et al., 2022).
Most often, electricity is supplied via centralized generation (large-scale generation), with power plants distributing electricity through electric power gird. However, distributed generation (on-site generation) has been gaining importance as renewable energies are developed (Gorr-Pozzi et al., 2021). Off-grid systems and mini-grids or microgrids can generate electricity efficiently as they are located close to the point of consumption. They are considered an attractive solution for isolated areas lacking electricity services, where the installation of power lines is not profitable.
In many developing countries, it is common to find communities lacking a quality electrical interconnection along the coastline (Alstone et al., 2015). The cost of deploying more traditional electrical infrastructure to provide a service for such a small number of inhabitants and the distance between the communities and the nearest cities mean that off-grid systems, in particular, are a potentially cost-effective option for these areas and could meet part of the electricity demand of isolated communities in Mexico. For example, the fishing community of Puertecitos in Baja California, has only 80 inhabitants. Electricity is supplied by a 60.2 kW hybrid microgrid (55.2 kW solar PV/5 kW Wind energy) and a 522 kWh battery energy storage (Cota-Soto et al., 2017). The Inter-American Development Bank (IDB) co-financed 11 projects for rural electrification in Latin America and the Caribbean for communities with 100–7,000 inhabitants (five now operating and six still being developed) (Graham et al., 2021). Off-grid systems offer the advantage of using emerging technologies for power generation, as their power rating (kW to MW) allows test prototypes in field conditions of an operational environment.
Off-grid systems are quite versatile since their configuration can be adapted to the local community’s needs, even if energy demand grows. Figure 1B shows an off-grid renewable energy system with a hybrid renewable energy source, a backup power source (typically a non-renewable system, such as a diesel generator), an energy storage system (e.g., batteries), and a power conditioning system (a regulator DC/DC, DC/AC), (Sawle et al., 2018). For the design and optimization of such systems, several methodologies have been suggested (Siddaiah and Saini, 2016). These focus on minimizing costs by maximizing generation time and reducing energy storage capacity. Furthermore, others also take into account social and environmental aspects to try to overcome potential negative impacts on ecosystems.
A policy framework is needed for national governments to unite countries internationally to commit to a just and inclusive energy transition that strengthens the flow of finance and attracts investors, capacity, and technologies. Five renewable energy innovation centers were created following the Mexican Energy Transition Law of 2013, to contribute to the growth and diversification of the national energy sector. The Mexican Center for Ocean Energy Innovation (CEMIE-Oceano) has been evaluating the possibilities of MRE, conducting studies on potential sites for implementation, taking into account environmental and social impacts. In 2017, the Mexican government developed a “Road Maps” series for ocean energy implementation (IEA-OES, 2022).
Criteria for the deployment of sustainable marine renewable energies
Availability of MRE resources, environmental constraints, regulatory and legal aspects, protection instruments, and maritime spatial planning are factors to be considered when evaluating potential sites for hybrid MRE systems. Most of these factors are site-specific. When considering the different criteria for sustainable MRE deployment, the potential benefits, and adverse effects can be evaluated to promote solutions that mitigate negative impacts. Table 1 shows an overview of the advantages and disadvantages of the technical, environmental, economic, and social components of deploying different MRE systems in Mexico. In turn, these are classified by criteria rated according to a color-coded for each MRE. Green indicates low risk, relative ease of deployment or few additional requirements, while red indicates high risk and less ease of implementation or the need for more conditions.
Discussion
Improving the sustainability of a hybrid MRE generation plant should consider all the components in Table 1 and analyse them using an interdisciplinary and holistic vision. This must reduce the likelihood of failure or potential impacts of MRE projects (Wang and Zhan, 2019).
From the ratings of all the criteria considered, the capacity factor, the climate change potential, and the exclusion zone are the features that most highly endorse MRE implementation in Mexico. However, the MRE variability, the associated potential impacts on threatened ecosystems, and the relatively low generation of by-products highlight the need for continued efforts in developing the feasibility of deploying sustainable energy harvesting devices. Among all current MRE technologies, the most suitable for its sustainable deployment at potential coastal sites in Mexico are TGE and OWE.
TGE offers a low annual variability, and its capacity factor gives a base load of annual energy production and a low LCOE. Furthermore, TGE presents positive environmental aspects, such as negative-CO2 emissions, and the TGE by-products make it more viable (e.g., freshwater production, aquaculture, and seawater conditioning. OWE is the most advanced MRE technology due to its learning rate, which reflect an accelerated increase in the installed capacity of commercial systems tested in the operating environment. It also has a competitive LCOE and more mature commercial deployment than other MREs. In contrast, the deployment of SGE systems presents several challenges as it still lacks technological development, has a high environmental risk related to its low carbon mitigation capacity, and carries a risk to nearby ecosystems associated with brine spills from the discharge pipelines. In addition, SGE is less economically attractive, primarily due to its high capital, operating, and maintenance costs. It is suggested that TGE and OWE prototypes-technologies be tested under field operating conditions to validate their performance, to have site-specific information on environmental and social impacts, and improve their profitability.
Mexico has substantial detailed information on the MREs available around its coasts. Improving capacity factors and energy production by more efficient MRE prototypes adapted to Mexico’s climatic conditions will directly impact its technological capabilities. The combination of electricity generation from various MRE sources, or hybridization, and their integration into off-grid systems will compensate for the variability of the electricity generated and allow isolated communities on the coast of Mexico to have access to this vital resource in a sustainable way. The use and commercialization of by-products in such a hybrid plant will boost the blue economy, creating numerous benefits and accelerating the pre-commercial stage. These conditions could increase the installed capacity of MRE systems and strengthen local human capabilities. In turn, this could improve credibility in MRE and increase confidence in investing in it in the medium term, as well as the perception and social acceptance of using these technologies. Joint work between the different levels of government, the private sector, academia, and the local population must be strengthened to develop public policies and regulations that encourage the sustainable installation of hybrid MRE systems in Mexico.
Author contributions
EG-P, JO-G, and RS contributed to conception and design of the study. EG-P and JO-G organized the database and performed the analysis of the criteria to foster the sustainable deployment of MREs in Mexico’s coastal zones. EG-P and JO-G wrote the first draft of the manuscript and RS supervised the manuscript. EG-P, JO-G and RS wrote in all sections of the manuscript. All authors contributed to manuscript revision, read, and approved the submitted version.
Funding
This research was funded by CONACYT-SENER Sustentabilidad Energética project: FSE-2014-06-249795. Centro Mexicano de Innovación en Energía del Océano (CEMIE-Océano).
Acknowledgments
We would like to thank the Centro Mexicano de Innovación en Energía-Océano (CEMIE-Océano) for supporting this research.
Conflict of interest
The authors declare that the research was conducted in the absence of any commercial or financial relationships that could be construed as a potential conflict of interest.
Publisher’s note
All claims expressed in this article are solely those of the authors and do not necessarily represent those of their affiliated organizations, or those of the publisher, the editors and the reviewers. Any product that may be evaluated in this article, or claim that may be made by its manufacturer, is not guaranteed or endorsed by the publisher.
References
Alcérreca-Huerta, J. C., Encarnacion, J. I., Ordoñez-Sánchez, S., Callejas-Jiménez, M., Barroso, G. G. D., Allmark, M., et al. (2019). Energy yield assessment from ocean currents in the insular shelf of Cozumel Island. J. Mar. Sci. Eng. 7, 147–218. doi:10.3390/jmse7050147
Alstone, P., Gershenson, D., and Kammen, D. M. (2015). Decentralized energy systems for clean electricity access. Nat. Clim. Chang. 5, 305–314. doi:10.1038/nclimate2512
Azuz-Adeath, I., Rivera-Arriaga, E., and Alonso-Peinado, H. (2019). Current demographic conditions and future Scenarios in Mexico´s coastal zone. Rev. Gestão Costeira Integr. 19, 85–122. doi:10.5894/rgci-n216
Babarit, A., Gilloteaux, J.-C., Clodic, G., Duchet, M., Simoneau, A., and Platzer, M. F. (2018). Techno-economic feasibility of fleets of far offshore hydrogen-producing wind energy converters. Int. J. Hydrogen Energy 43, 7266–7289. doi:10.1016/j.ijhydene.2018.02.144
Bernal-Camacho, D. F., Fontes, J. V. H., and Mendoza, E. (2022). A technical assessment of offshore wind energy in Mexico: A Case study in Tehuantepec Gulf. Energies 15, 4367. doi:10.3390/en15124367
Cota-Soto, R., Velázquez-Limón, N., and Jímenez-Estévez, G. (2017). Estudio y evaluación de microrredes para comunidades aisladas. Available at: http://catalogocimarron.uabc.mx/cgi-bin/koha/opac-detail.pl?biblionumber=222722.
EERE (2021). Should I Get battery storage for My solar energy system? Department of Energy. Available at: https://www.energy.gov/eere/solar/articles/should-i-get-battery-storage-my-solar-energy-system (Accessed August 20, 2022).
Europa Publications (2022). The Europa directory of international organizations 2022. 24th ed. London: Routledge. doi:10.4324/9781003292548
García-Ochoa, R., and Graizbord, B. (2016). Caracterización espacial de la pobreza energética en México. Un análisis a escala subnacional. Econ. Soc. Territ. 16, 289–337. doi:10.22136/est002016465
Garduño-Ruiz, E. P., Silva, R., Rodríguez-Cueto, Y., García-Huante, A., Olmedo-González, J., Martínez, M. L., et al. (2021). Criteria for optimal site selection for ocean thermal energy conversion (Otec) plants in Mexico. Energies 14, 2121–2123. doi:10.3390/en14082121
Gorr-Pozzi, E., García-Nava, H., Larrañaga, M., Jaramillo-Torres, M. G., and Verduzco-Zapata, M. G. (2021). Wave energy resource harnessing assessment in a subtropical coastal region of the Pacific. J. Mar. Sci. Eng. 9, 1264. doi:10.3390/jmse9111264
Gourvenec, S., Sturt, F., Reid, E., and Trigos, F. (2022). Global assessment of historical, current and forecast ocean energy infrastructure: Implications for marine space planning, sustainable design and end-of-engineered-life management. Renew. Sustain. Energy Rev. 154, 111794. doi:10.1016/j.rser.2021.111794
Graham, N., Malagón, E., Viscidi, L., and Yepez, A. (2021). State of charge: Energy storage in Latin America and the Caribbean. Washington, DC: Inter-American Development Bank. Available at: https://publications.iadb.org/en/state-charge-energy-storage-latin-america-and-caribbean.
Hernández Galvez, G., Chuck Liévano, D., Sarracino Martínez, O., Lastres Danguillecourt, O., Dorrego Portela, J. R., Narcía, A. T., et al. (2022). Harnessing offshore wind energy along the Mexican coastline in the Gulf of Mexico—an Exploratory study including sustainability criteria. Sustainability 14, 5877. doi:10.3390/su14105877
Hernández-Fontes, J. V., Felix, A., Mendoza, E., Cueto, Y. R., and Silva, R. (2019). On the marine energy resources of Mexico. J. Mar. Sci. Eng. 7, 191. doi:10.3390/jmse7060191
Huante, A. G., Cueto, Y. R., Silva, R., Mendoza, E., and Vega, L. A. (2018). Determination of the potential thermal gradient for the Mexican Pacific Ocean. J. Mar. Sci. Eng. 6, 20–14. doi:10.3390/jmse6010020
IEA-OES (2022). Annual Report: An Overview of Ocean Energy Activities in 2021. Paris, France: The Executive Committee of IEA Ocean Energy Systems. Available at: https://www.ocean-energy-systems.org/documents/25238-oes-2021-annual-report-vf.pdf/.
IRENA (2019). Demand-side flexibility for power sector transformation. Abu Dhabi. Available at: www.irena.org.
IRENA (2020). Innovation landscape brief: Market integration of distributed energy resources. Abu Dhabi. doi:10.1049/pbpo167e_ch14
IRENA (2021). World energy transitions outlook: 1.5 degrees pathway. Abu Dhabi. Available at: https://irena.org/publications/2021/March/World-Energy-Transitions-Outlook.
Kim, C.-K., Toft, J. E., Papenfus, M., Verutes, G., Guerry, A. D., Ruckelshaus, M. H., et al. (2012). Catching the right wave: Evaluating wave energy resources and potential Compatibility with Existing marine and coastal Uses. PLoS One 7, e47598. doi:10.1371/journal.pone.0047598
LiVecchi, A., Copping, A., Jenne, D., Gorton, A., Preus, R., Gill, G., et al. (2019). Powering the blue economy; Exploring Opportunities for marine renewable energy in maritime Markets. Washington, D.C: Energy Efficiency & Renewable Energy.
Ma, C., Wang, X., and Jiang, B. (2022). ocean energy development under the background of carbon emissions Peak and carbon Neutrality in China. IOP Conf. Ser. Earth Environ. Sci. 966, 012003. doi:10.1088/1755-1315/966/1/012003
Martínez, M. L., Vázquez, G., Pérez-Maqueo, O., Silva, R., Moreno-Casasola, P., Mendoza-González, G., et al. (2021). A systemic view of potential environmental impacts of ocean energy production. Renew. Sustain. Energy Rev. 149, 111332. doi:10.1016/j.rser.2021.111332
Masson-Delmotte, V., Zhai, P., Pirani, A., Connors, S. L., Péan, C., Berger, S., et al. (2021). “IPCC, 2021: Summary for Policymakers,” in Climate change 2021: The physical Science Basis. Contribution of working Group I to the Sixth Assessment Report of the Intergovernmental Panel on climate change. Editors R. Yu, and B. Zhou (Cambridge University Press) In Press.
Olmedo-González, J., González-Huerta, R. de G., and Ramos-Sanchez, G. (2021). “Influencia del almacenamiento de energía en sistemas renovables marinos,” in 1er Congreso Internacional CEMIE-Océano. Mexico City: CEMIE-Océano A.C.
Olmedo-González, J., Ramos-Sánchez, G., Garduño-Ruiz, E. P., and González-Huerta, R. de G. (2022). Analysis of Stand-alone Photovoltaic—marine current hybrid system and the Influence on daily and seasonal energy storage. Energies 15, 468–521. doi:10.3390/en15020468
Paredes, M. G., Padilla-Rivera, A., and Güereca, L. P. (2019). Life cycle assessment of Ocean Energy technologies: A systematic review. J. Mar. Sci. Eng. 7, 322. doi:10.3390/jmse7090322
Posada-Vanegas, G., Chávez-Cerón, V., Hernández-Fontes, J. V., Rodríguez-Cueto, Y., Cadena-Sánchez, G., Félix-Delgado, A., et al. (2019). “Energía y cambios globales: El Futuro de las Energías Marinas en México,” in Tópicos de Agenda para la Sostenibilidad de Costas y Mares Mexicanos. Editors E. Rivera-Arriaga, P. Sánchez-Gil, and G. Jorge Campeche, México, 255–274. doi:10.26359/epomex.0519
Rau, G. H., and Baird, J. R. (2018). Negative-CO2-emissions ocean thermal energy conversion. Renew. Sustain. Energy Rev. 95, 265–272. doi:10.1016/j.rser.2018.07.027
Sawle, Y., Gupta, S. C., and Bohre, A. K. (2018). Review of hybrid renewable energy systems with comparative analysis of off-grid hybrid system. Renew. Sustain. Energy Rev. 81, 2217–2235. doi:10.1016/j.rser.2017.06.033
Sheng, L., Zhou, Z., Charpentier, J. F., and Benbouzid, M. E. H. (2017). Stand-alone island daily power management using a tidal turbine farm and an ocean compressed air energy storage system. Renew. Energy 103, 286–294. doi:10.1016/j.renene.2016.11.042
Siddaiah, R., and Saini, R. P. (2016). A review on planning, configurations, modeling and optimization techniques of hybrid renewable energy systems for off grid applications. Renew. Sustain. Energy Rev. 58, 376–396. doi:10.1016/j.rser.2015.12.281
Smoot, G. (2022). What is the carbon Footprint of tidal energy and wave energy? A life-cycle assessment | impactful Ninja. Available at: https://impactful.ninja/the-carbon-footprint-of-tidal-energy-and-wave-energy/(Accessed September 4, 2022).
Tobal-Cupul, J. G., Garduño-Ruiz, E. P., Gorr-Pozzi, E., Olmedo-González, J., Martínez, E. D., Rosales, A., et al. (2022). An assessment of the financial feasibility of an OTEC Ecopark: A Case study at Cozumel island. Sustainability 14, 4654. doi:10.3390/su14084654
Wang, Q., and Zhan, L. (2019). Assessing the sustainability of renewable energy: An empirical analysis of selected 18 European countries. Sci. Total Environ. 692, 529–545. doi:10.1016/j.scitotenv.2019.07.170
Keywords: energy transition, marine renewable energy (MRE), off grid electrification, hybrid reneable energy system, development of sustainable MRE
Citation: Gorr-Pozzi E, Olmedo-González J and Silva R (2022) Deployment of sustainable off-grid marine renewable energy systems in Mexico. Front. Energy Res. 10:1047167. doi: 10.3389/fenrg.2022.1047167
Received: 17 September 2022; Accepted: 17 October 2022;
Published: 31 October 2022.
Edited by:
Zbigniew M. Leonowicz, Wrocław University of Technology, PolandReviewed by:
Jorge Cunha, University of Minho, PortugalCopyright © 2022 Gorr-Pozzi, Olmedo-González and Silva. This is an open-access article distributed under the terms of the Creative Commons Attribution License (CC BY). The use, distribution or reproduction in other forums is permitted, provided the original author(s) and the copyright owner(s) are credited and that the original publication in this journal is cited, in accordance with accepted academic practice. No use, distribution or reproduction is permitted which does not comply with these terms.
*Correspondence: Jorge Olmedo-González, am9yZ2VvbG1lZG9nQG91dGxvb2suY29t