- 1Department of Electrical Engineering, Shanghai Jiao Tong University, Shanghai, China
- 2Marine Design and Research Institute of China, Shanghai, China
Introduction: The structure of green and low-carbon ports is complex, and an integrated port energy system with complex characteristics such as multi-energy flow coupling and information physical fusion has been formed.
Methods: Digital twin technology provides a new solution for the perception, analysis, and optimization of integrated port energy system. The paper explores the digital twin of integrated port energy system. Based on the development trend and function of integrated port energy system, the digital twin of integrated port energy system architecture is proposed to explore its potential application services. Specifically, considering the functions and structural characteristics of the port, the digital space of integrated port energy system is established, which describes the pan electrification, multi energy flow fusion, the “physics-information-value” coupling, and the characteristics of system operation under uncertain scenarios of integrated port energy system from the physical and data dimensions. The hybrid driving method based on data and mechanism is used to explore digital twin of integrated port energy system and find potential better applications.
Results and discussion: Finally, combined with the current development needs of integrated port energy system, this paper analyzes the key problems and technologies that need to be broken through in the specific implementation of digital twin of integrated port energy system, with a view to providing some thoughts and references for the low-carbon development of ports and the future application of digital twin technology under the goal of “double carbon”.
1 Introduction
As a strong support for China’s economic construction, large ports are also the main source of carbon emissions in China. Under this background, construction of a low-carbon green integrated port energy system (IPES) is needed not only to meet the requirements of the development of the times but also for the inevitable trend of port development (Ertem et al., 2017; Wang et al., 2019; Sifakis et al., 2021). In the future, the large-scale operation equipment of the green port will fully adopt the power substitution technology, and the port transportation logistics system and energy system will show strong coupling. At the same time, as the port area also contains many other forms of energy, the coupling relationship between heterogeneous energy sources and between energy and logistics is becoming increasingly complex and the mutual influence is intensifying (Yigit and Acarkan, 2018; Tan et al., 2021).
The coupling mechanism of the IPES is complex, and the external influence factors are random and changeable. In particular, the relevant research considering the coupling of transportation and society is still under exploration (Guo et al., 2021). There is lack of a unified theoretical system of the cross-domain and multi-network coupling at home and abroad, which makes it difficult to achieve systematic and in-depth research. Therefore, it is urgent to need reasonable research theories and analysis methods. Traditional analysis, control, and optimization methods based on local mathematical modeling and computer simulation can no longer accurately, objectively, and comprehensively reflect its operating characteristics. In the past, the traditional port energy system simulation analysis was mostly based on engineering experience, and the equipment was taken as the unit for system analysis. This method relied on the simplification of the physical mechanism and assumptions, and the equipment and environment were typified or extreme. The model and analysis method were relatively solid (Fang et al., 2022; Huang et al., 2022). For the IPES with complex structure, high model order, and strong non-linearity, this method has many disadvantages: on one hand, its errors affect the accurate quantitative analysis and design of the IPES; on the other hand, the analytical expression model of the system is often very complex, which leads to significant amount of calculation and low efficiency. In contrast, a digital twin is virtual, and the evolution is mainly rapidly unfolded in the digital space through the twin model. The twin model can be achieved through pre-training, with strong approximation and high fidelity, and can be continuously optimized through real-time data interaction, closed-loop feedback, and other mechanisms to provide support for its intelligent decision-making and efficient operation. Compared with traditional integrated energy system simulation analysis, the digital twin has more potential. The digital twin has been successfully applied to complex “coupling systems” such as aerospace and electric power, mainly used for equipment production control, operation analysis diagnosis, and full life-cycle management (Glaessgen and Stargel, 2012; Tao et al., 2019; He et al., 2021).
Existing literature works have studied the application of digital twin technology in an integrated land energy system (Wang et al., 2021a). As a special type of an integrated energy system, the integrated port energy system still has its own characteristics, such as multi-energy flow fusion and “physics information value” coupling (Fang and Wang, 2021). Therefore, the related issues of the digital twin in the integrated port energy field, such as overall framework, modeling, deep integration of information and data, and potential applications, need to be specifically studied and discussed. Considering the particularity of the IPES, the digital twin of the integrated port energy system (IPES-DT) is based on advanced communication technology, realizing full data coverage, low delay and high reliable transmission, and mapping the physical system to the digital system in real time. Furthermore, based on signal processing, artificial intelligence, high-dimensional analysis, situation awareness, and other means, the intelligence level of decision-making will be improved, and new solutions will be provided for the technical bottleneck problems faced by the port energy industry in the future, such as state analysis of complex systems, equipment clustering management, multi-time scale energy management, and system reliability evaluation.
2 Development trend and characteristics of the IPES
In terms of port construction, the electrification of marine transportation represented by shore power technology, electric propulsion ships (Sun et al., 2022), and electric-driven port machinery and the zero-carbon fuel technology represented by hydrogen energy/ammonia energy (Wang et al., 2021b) have promoted the deep integration of ports and multi-type energy networks, and a special “marine energy-integrated transportation system” has been initially formed. At the same time, the popularity of multi-modal transport and rapid development of the cold chain transport have brought diversified comprehensive energy demand to the port. At present, the energy side and load side of the world’s major ports have been electrified to varying degrees. A large number of studies show that electrification has a significant effect on improving the energy efficiency of ports. In the future, ports will participate in the power market as independent energy regions, making the energy and power of ports tend to be integrated and pan-electrified. The power grid form is “main power grid + distribution grid + micro grid” (Fang et al., 2019; Mutarraf et al., 2021; Sadiq et al., 2021). The gradually improved port power grid will become the backbone network of the port energy system, undertake the energy supply task of various port machinery and transfer vehicles, become the enrichment point of offshore new energy, and complete the power transaction with the main power grid, as shown in Figure 1.
New energy power generation is one of the important means of low-carbon operation of the port. The port is a renewable energy-intensive area with abundant water resources. New energy enriches the energy types of the port, and the coupling, transformation, and use of multi-type energy flows not only bring energy efficiency improvement potential for the port but also put forward higher requirements for the control and management of the energy side and load (Zis et al., 2014; Yigit et al., 2016).
The IPES is composed of an integrated energy system, transportation logistics system, and information value system, as shown in Figure 1. The integrated energy system is established based on the concept of energy hub, including the energy supply side, energy conversion side, and load side. The transportation logistics system not only performs transportation logistics scheduling but also serves as a part of the load of the integrated energy system. The coupling of the energy network and the transportation network enables them to provide each other with operating power. At the same time, through the intelligent perception and sharing of the information network, the coupling of the transportation network and the energy network is optimized synergistically and is affected by social value behaviors such as management, service, and transaction. The port management coordinates the number of ships entering and leaving the port and allocation of shore bridges, as well as the output of various types of energy equipment and the start and stop status of ship equipment, so as to ensure the coordinated and orderly operation of the port transportation energy system. Meanwhile, the IPES also needs to provide charging service and clean energy service for transfer vehicles that complete the three problems of loading and unloading scheduling, cargo transfer, and yard scheduling. Also, the operation constraints of cold chain management cause the port to have the characteristics of “electricity heat” coupling. It can be observed that the energy side and the traffic side in the integrated port energy system are deeply coupled, as shown in Figure 2 (Fang et al., 2022).
Intellectualization is the direction and inevitable trend of IPES development. The establishment and improvement of the IPES need to fully consider the needs of future technological changes. In the current application and research process, there are problems that restrict the development: in terms of perception ability, the change in the IPES source network load and the perception depth and breadth of existing systems are insufficient. In terms of data transmission and processing capacity, the universality and real-time of network connection are not enough, and it is difficult to achieve efficient access and management of massive terminals and data. At the same time, the collected data have not been fully processed and applied. In terms of control ability, the control means on each side of the source network load are single, and the interaction ability with the port manager is insufficient.
3 Core and framework of the IPES-DT
3.1 Demand analysis and challenges of the IPES-DT
As a technology that makes full use of models, data, and intelligence and integrates multiple disciplines, the digital twin faces the whole life cycle process of products, plays the role of a bridge and link between the physical world and the information world, and provides real-time, efficient, and intelligent services, as shown in Figure 3 (Pang et al., 2022).
The integrated port energy system involves diversified energy forms, covering the whole process from production to consumption. It has complex internal physical composition and technical combination characteristics. Its development is facing many characteristic problems. The integrated port energy system has opened up the coupling channels of various energy sources at the physical level, but it still faces barriers at the information and data level. The measurement and management of power, heat, gas, wind, and other energy forms are mostly based on the conventional technical means in their respective fields, and there are many differences in data structure, time scale, error accuracy, etc. The lack of effective correlation, verification, and fusion utilization mechanism among multi-source data leads to difficulties in realizing accurate perception and analysis of the overall system operation situation. Large ports include multiple logistics subsystems and energy subsystems. The operation modes, physical characteristics, and time scales of each subsystem are different, so there are essential differences in the operation characteristics between the logistics system and the energy system. Therefore, in different scenarios such as planning, scheduling, operation, and maintenance of the integrated port energy system, different contents need to be described, which puts forward various requirements for the granularity and integrated computing capacity of the model. As a special comprehensive energy system, in addition to the similar characteristics of the coupling modeling method, the integrated port energy system has its own characteristics compared with the integrated land energy system, including multi-energy flow integration, energy transportation integration, multi-stakeholder coordination, and system operation characteristics under uncertain scenarios. Therefore, under the joint action of these factors, new methods and means are needed to analyze the complex characteristics of the port integrated energy system. A digital twin is a set of technology systems developed on the basis of existing modeling and simulation, big data analysis, information physics fusion, and other technologies with the help of new generation information technology. Simulation builds simplified models of physical objects based on simplification and hypothesis methods. However, due to the lack of interaction with the environment, it is difficult to achieve autonomous learning of simulation behavior and synchronous updating of the physical entity state. The cyber physical system (CPS) focuses on the role and interaction of information systems on physical systems and generally does not consider its specific business applications and related intelligent algorithms. However, the digital twin is a kind of high-fidelity dynamic simulation that evolves with time and space. It fully integrates data and mechanism and has the ability of autonomous learning. Additionally, its model can realize the spatiotemporal evolution analysis and feedback guidance of virtual space to the real world.
Based on practical experience, the author’s team believes that with the continuous development of the digital twin, its application in the IPES field will be deepened, and the research work that needs to be broken through includes 1) building a digital twin framework with high applicability and scalability; 2) establishing a complex network model suitable for analyzing its adaptive evolution mechanism for the energy information transportation society coupling network to realize physical digital fusion modeling in the aspect of entity to virtual mapping; 3) building boundary constraints for digital twin image states based on the limited measurements of the real system; 4) carrying out the iterative interaction and dynamic evolution between the entity and the virtual to achieve collaborative interaction in the aspect of feedback.
3.2 Significance and kernel of the IPES-DT
At present, the intelligence level of port automation is still in its infancy. The performance, control methods, and ability to solve complex problems of the energy system are also lacking, and the utilization and integration of information are not high. The existing energy system analysis and control methods are more limited to physical entities or information space itself. The significance of the construction of the digital twin system of integrated port energy is as follows:
1) Perception and operation control of the IPES. For the integrated port energy system itself, affected by the deep coupling of various energy forms in the whole process of production distribution storage transportation, its operating characteristics will be significantly different from those of the traditional independent energy system, and it will reflect the hybrid characteristics of multi-physical system coupling, multi-time scale dynamic characteristic correlation, and strong non-linearity and uncertainty. In addition, it is also necessary to ensure the safe and efficient operation of the integrated port energy system under uncertain scenarios such as climate change, which leads to complex issues such as the planning and design of the integrated energy system, energy management, and equipment status monitoring and maintenance, and must rely on a deep understanding and control of the system’s operating status and characteristics. At this time, the fusion of multi-source measurements, analysis of system characteristics, prediction of development trends, and optimization of control decisions need to be completed more efficiently in the digital space.
2) Fusion modeling of mechanism and data. In the research of the traditional integrated energy system, the research on the power network, thermal network, and natural gas network has been relatively sufficient. However, the integrated port energy system also includes the liquid network, such as the energy network of crude oil, liquefied natural gas, and hydrogen. It is necessary to establish a mathematical model that reflects the operating characteristics of the liquid network, further establish a refined model that can characterize the energy conversion process and energy lag characteristics of heterogeneous energy coupling within the equipment, and finally establish the dynamic optimization mathematical model of multi-energy and multi-network characteristics suitable for the integrated port energy system. In addition, on the basis of analyzing and revealing the coupling mechanism of the port logistics system and energy system, it is necessary to reveal the space–time correlation characteristics of each logistics operation link and its impact on the operation of the energy system and analyze the dynamic characteristics and dynamic behavior mechanism between each energy supply system and logistics load. Considering the complexity of the integrated port energy system, it is difficult to construct an analytical mathematical model for a large number of links with an unclear mechanism and opaque state. It will rely more on the data-driven method to reveal its operation characteristics. As a direct reflection of the physical system state and characteristics, the system operation data can be used to drive the continuous improvement of the digital twin model and parameters and the synchronous evolution of the virtual reality system. The digital twin has good non-linear fitting ability and strong online decision-making ability for multi-source heterogeneous data (He et al., 2017). At the same time, a priori knowledge is introduced to improve the interpretability, robustness, and generalization of artificial intelligence algorithms. Traditional physical simulation adopts the offline mode, which is often used as a computing tool for analysis and decision-making, and can only be manually invoked to serve a certain business, while the digital twin fully integrates data and mechanism through real-time interaction with physical objects to complete automatic updating of model structure/parameters and full digitization of the business chain. The IPES-DT can realize efficient solutions under the constraint of limited computing resources by establishing a multi-granularity model and supporting adaptive dynamic matching and seamless switching according to the changes of different objects and scenarios in the coupling process of the energy supply side, energy conversion side, load side, and logistics transportation system.
3) Design and construction. The digital twin realizes the holographic mirror image including the spatial structure characteristics of the energy system and can support the integration of the planning and design scheme and the construction implementation scheme, that is, in the planning and design stage, the matching relationship between the planning scheme and the port power grid, the shore bridge/yard bridge, the cold chain transport container and the energy transmission pipeline, etc. is considered to make the planning scheme realizable in the real environment, and the coordination with the overall construction plan of the port can be an important constraint for planning and design. The digital twin can provide a more real test environment for the evaluation of planning schemes. The source, load, and uncertainty of the environment in the operation of the integrated port energy system will significantly affect the actual performance of the planning scheme. Furthermore, on one hand, the digital twin can provide more complete operation simulation capability and support the consideration of interaction with various real factors such as external wind, light resources, and environmental temperature. On the other hand, it can gather the operating experience of similar systems in the whole life cycle, make the performance evaluation of the planning scheme more objective and accurate, and lay a foundation for the optimal planning decision close to reality.
4) Building a port ecosphere that intelligently integrates green and versatile flow. The IPES-DT will combine the current operation and historical data to form an operation decision support library to provide suggestions for multi-stakeholders of the government, port operators, ship owners, and logistics service providers and to help them judge and manage the operation status of IPES devices so as to improve the utilization rate of devices and system stability. At the same time, cluster management is used to provide value-added services such as life-span health prediction, failure prediction, and life-cycle management for energy equipment and provide more abundant and accurate decision-making basis for port planning and construction. Using the user-side models and data provided by digital twins, the energy consumption habits of ships can be depicted and new port management and business operation modes can be formed.
3.3 General framework of the IPES-DT
The IPES-DT builds a digital twin of complete mapping of the IPES through accurate perception and real-time connection of real-time operation information at the system level and the equipment level. At the digital level, it adopts modeling methods of mechanism and data fusion, intelligent control strategies, etc., and combines cloud collaboration and physical system analysis supported by physical digital twin Technology, etc., to achieve intelligent decision-making of system operation. The interconnection and interaction between various subsystems of the IPES-DT enables the elements at different levels to operate efficiently and allocate resources reasonably and feeds back to the physical system through “human–machine cooperation,” which makes the operation control strategy and model more perfect. The overall framework can be composed of three levels: intelligent infrastructure, intelligent operation hub, and intelligent application system, as shown in Figure 4.
The information infrastructure is the foundation of system operation, mainly including intelligent sensing collection equipment terminal, to fully realize the state perception, quantity value transmission, environmental monitoring, and behavior tracking of different objects by the IPES; the IPES is the channel of global information exchange and sharing network. The network layer integrates the data collected by the intelligent sensing collection device or the knowledge obtained from the physical object into the digital twin system and realizes the connection and communication between the physical entity and the virtual entity of the IPES; intelligent computing operating environment—cloud. By endowing each resource element of the system to realize digitization and intellectualization, the intelligent hub is carried by three core platforms: the first is the IPES-DT data platform, which breaks through the real-time high-performance analysis and management technology of massive multi-source heterogeneous information of different time scales of the energy and transportation coupling system. Based on the cloud computing architecture, it forms a spatiotemporal big data platform integrating data management and platform management. Through the blockchain technology, it improves the reliability of data and integrates with the model platform to show the overall picture and operation status of the system and become a strong foundation for the mechanism and data-driven mode. The second is the IPES-DT model platform, which integrates with the data platform into a digital base and is the core of IPES-DT ‘s accurate mapping of virtual and real interaction. The third is the generic technology empowerment and application support platform, which gathers the basic service capabilities of artificial intelligence, big data, and other new technologies, as well as the unique scene services, data services, and simulation services of the intelligent platform, to provide technology empowerment and unified development services for upper layer applications.
The application layer is the embodiment of the specific functions of the system. Based on the support of the information infrastructure and the intelligent hub, around the two major requirements of IPES operation control and energy management optimization, the development of intelligent application function modules can meet the needs of the IPES under various working conditions and achieve application integration. The decision-making management link is that the operator directly transmits the control command to the physical operation object through the functional module of the application layer based on the real-time operation of the IPES-DT, that is, the feedback control closed loop of the IPES-DT is realized in the mode of “people in the loop.”
Standard specification guarantee is the constraint of system development. On the basis of previous standard systems related to port equipment development, and in combination with the actual demand characteristics of the IPES, the overall standard specification and evaluation framework of the IPES-DT are constructed. Information security guarantee is the guarantee of network and information security of the IPES-DT.
4 Key technology of the IPES-DT
4.1 Panoramic information perception technology
The premise of the IPES-DT construction is to establish a global, all-weather, and omni-directional port environment sensing system to achieve multi-dimensional and multi-level accurate monitoring of the operation of the integrated power system. Highly reliable advanced sensors and intelligent sensing terminals are the basis for information acquisition of the sensing system. The existing sensors have limitations in physical size, acquisition accuracy, electromagnetic protection, etc., and the data acquisition terminal has problems such as weak analysis ability, single function, and poor expansibility. Panoramic information perception technology includes the following aspects:
1) Highly reliable advanced sensors for complex working conditions and environments. It can be applied to the complex climate environment of the port and the harsh electromagnetic environment and has the characteristics of miniaturization, low power consumption, low delay communication transmission, high-precision time synchronization, etc., (Zhang et al., 2021). At the same time, in order to meet the requirements of high sensing capacity of the integrated energy system, it is necessary to study the sensing mechanism and efficient power supply method based on new materials to further improve the sensor sensitivity.
2) According to the structural characteristics and equipment conditions of the IPES at the target port, the monitoring points shall be reasonably deployed, and the environmental interference and damage to the sensors and the effective shielding of the transmission line shall be considered.
3) The intelligent sensing terminal has implementation of edge intelligence, including the chip integration of functional modules such as data acquisition, identification, processing, and communication. Through the built-in function analysis algorithm and edge computing technology, the data collected by different types of sensors are analyzed at the bottom level. The application of physical resources at the edge side is realized through the lightweight container technology defined by the software. Through the multi-parameter Internet of Things agent, a variety of sensor access, service distribution, edge computing, and regional autonomy are realized.
4) Abnormal sensing device detection. The complex climate environment and operating conditions will make the sensing device more prone to failure, and the existence of abnormal sensing devices will cause the data collected to be abnormal, resulting in misjudgment or missing judgment of the equipment operating state, thus affecting the construction of the digital twin model of port equipment. Therefore, it is necessary to judge the effectiveness of the sensor device based on the abnormal characteristics of the real-time monitoring data, evaluate the reliability of the sensor device, find the abnormal sensor device in time, and eliminate the corresponding data to ensure the accuracy of the data model construction of various types of equipment in the port.
4.2 Multi-source data fusion technology
The IPES includes the power network, thermal network, fuel network, and transportation network. Different networks have different structures. IPES data have a wide range of sources, types, and structures.
4.2.1 Significance of multi-source data fusion
On one hand, multi-source data fusion will help the accurate analysis of each energy use scenario in the port, thus effectively improving the accuracy of target perception. It can reduce the impact of sudden changes in the surrounding environment on the monitoring performance of the system, improve the adaptability to environmental changes, make the monitoring and evaluation system have good robustness, and reduce the information ambiguity of the system and the uncertainty of the system.
On the other hand, multi-source data fusion will help analyze the coupling relationship of complex networks. The IPES is a multi-network coupled system, which is more complex in structure, operation mode, and interaction relationship. The traditional isolated network analysis method is no longer applicable. Therefore, making full use of multi-source data from different networks to map complex dependency relationships to data fusion and analysis from a global and unified perspective, so as to fully mine the dependency and coupling relationships between networks, is conducive to the cooperation between multiple networks.
4.2.2 Technical framework of multi-source data fusion
According to IPES-DT data form and fusion purpose, it can be divided into intra- and inter-network fusion. Intra-network fusion is to take multi-modal data of various types, structures, or sources as input and then realize accurate and unified description of the same type of the network through comprehensive analysis. Inter-network integration takes the network structure, network attributes, and network operation data as inputs and then realizes the analysis of association and collaboration among networks, mainly including the integration analysis of two or more types of networks in heterogeneous energy networks, transportation networks, and information networks through comprehensive analysis.
The multi-source data fusion in the network can be divided into three categories: data level, feature level, and decision level. In the application process, different fusion modes will be combined according to needs. At present, the IPES network fusion has achieved preliminary application results, but there are still two key problems to be solved: multi-time scale fusion and multi-structure fusion. For the alignment of structured data, the high-frequency reconstruction can still be carried out based on the data with a high sampling rate, using point estimation or interpolation methods. For unstructured or heterogeneous multi-modal data, the time alignment method can be adopted, that is, the high sampling data at the same or similar sampling time can be selected to align with the low sampling frequency so as to align the multi-modal data on the time scale. The different feature description spaces obtained by different feature extraction are uniformly mapped to the same space or coordinate system, that is, the heterogeneous feature vectors are assimilated, and then feature fusion is performed in the common subspace/sub coordinate system.
Inter-network fusion is applied to the analysis of association and collaboration of any two or more coupled networks. It can be characterized by a set. Each element in the set can be a sub-attribute set, equation description, and language description to describe the multi-attribute of the node. Then, considering the information and structure association, the coupling network and different energy conversion can be described by using the topology-based mode. Finally, based on the multi-attribute node modeling and association relationship modeling process, the mechanism and data-driven hybrid modeling methods are used for fusion analysis.
4.3 Multi-energy flow fusion system mechanism and data mixing modeling
4.3.1 Modeling driven by data and mechanism
In the IPES, the dynamic characteristics of electric, thermal, gas, and liquid heterogeneous energy systems are significantly different, and the dynamic modeling methods are different. Due to different modeling mechanisms, different modeling methods and integration timing will lead to inconsistent data interface forms, and data interaction errors are easy to occur at the energy system interface. Therefore, the dynamic characteristics of the system are described by general expression, and a unified system model is built under the same simulation framework to meet the modeling requirements in different scenarios. At present, there are three problems in the mechanism analysis of the IPES: 1) affected by factors such as network topology and complex working conditions, the operating characteristics of equipment and system change with working conditions, which brings great difficulties to the mechanism analysis; 2) how balance the contradiction between model fidelity and computational complexity; 3) how to improve the similarity between simulation data and actual data and continuously improve the model.
Both the research on the knowledge-driven differential algebra model based on the system mechanism and the research on statistical correlation model based on data-driven modeling have achieved certain results. However, a single model has certain disadvantages. It is necessary to fuse the two types of models at the modeling level and the decision support level. The data-driven statistical correlation model is used to make up for the defects of incomplete mechanism modeling, and the uncertainty information is not considered. The knowledge-driven differential algebra model is used to make up for the defects of limited and biased samples of the data-driven model so as to realize the real description and optimal control of the entity IPES. The advantages of the data and mechanism joint driving method are as follows: 1) through the data-driven dynamic equivalent modeling method, the area where the mechanism modeling is difficult in the IPES is set as the equivalent area, and the network topology of the non-equivalent area is kept unchanged. A variety of disturbances and different operating conditions are set in the non-equivalent area to form a dynamic data sample set, and the dependence between the input and output of the equivalent area is analyzed and obtained. An equivalent model which can approximately represent the dynamic characteristics of the original system is established. 2) By embedding physical knowledge into the data model, a partitioned multi-rate collaborative simulation algorithm suitable for different energy characteristics can be designed to improve the calculation efficiency of the data model and achieve efficient solution under the constraint of limited computing resources. 3) The data-driven method can use the actual system operation information to correct the errors caused by the simplification of the physical mode, so as to improve the physical model.
The mechanism and data-driven hybrid modeling of the IPES-DT mainly includes the parallel mode and feedback mode: 1) the parallel mode integrates the results of data-driven and knowledge mechanism-driven methods as the final output result. 2) The feedback mode relies on various information collection devices to measure the actual state of the equipment, process the data of the actual scene through the data-driven method, and assist in identifying and modifying the parameters of the mechanism model. The mechanism model helps data modeling in sample pretreatment and data set enhancement to improve the accuracy and interpretability of the mixed model. This model is suitable for scenarios with unknown mechanism, mismatched model parameters, or uncertainty (Li et al., 2021).
4.3.2 Operation environment of the platform
The digital twin model platform adopts the adaptive interface integration technology and is integrated into the platform in a plug and play manner. The adaptation interface forms a data access and interoperability interface by abstracting the technical details of various software integration, including data interface and cooperative instruction interface. Receiving/sending data and instructions using the data interface to realize data interaction and collaborative operation between heterogeneous models is established by different software.
In the aspect of the collaborative operation of digital twin models, the multi-disciplinary model collaborative operation method based on a simulation engine can be used to realize the interconnection and interoperability between multi-disciplinary, multi-domain, and mixed heterogeneous models. On one hand, it solves the behavior scheduling and information exchange between local internal models, and on the other hand, it solves the coordination of logic, time management, information, and other aspects with other nodes in other distributed systems to jointly complete the task of the collaborative operation of the digital twin model. The fusion model is used to realize the IPES digital image. The digital twin model platform is composed of the resource layer, interface layer, and core function layer, as shown in Figure 5.
5 Typical application of the IPES-DT
5.1 Equipment cluster management and decision-making
By establishing an integrated perceptual monitoring system based on the digital twin of the integrated port energy system, the real-time monitoring of the operation status and environmental data of the port physical equipment can be realized in the operation and maintenance stage of the integrated port energy equipment, and the perceptual monitoring data of all elements of the equipment can be collected in the data center. Based on the three-dimensional model of port equipment and the perceptual monitoring data, a refined digital twin model of port equipment is built, which combines model driving and data driving, so that the digital twin model of equipment can operate synchronously with the physical equipment under the driving of digital twin data and can form equipment evaluation and fault prediction data. On this basis, artificial intelligence algorithms such as machine learning are applied to carry out simulation calculation and data analysis on the digital twin model. With the continuous iterative optimization of model data, the digital twin model feeds back the data information and instructions obtained from real-time calculation and analysis to the physical entity equipment of the port and drives the physical entity equipment to carry out defect diagnosis and fault warning so as to formulate a reasonable operation and maintenance strategy.
Based on the established refined digital twin model of port equipment, the equipment historical data and real-time monitoring data collected by the data center are input into the digital twin model, and the input massive data are sorted to realize multi-dimensional real-time recording of equipment data. Then, the big data analysis technology and machine learning algorithms are applied to analyze the historical data and real-time data of the port equipment so that the future state of the equipment can be predicted in real-time. At the same time, the digital twin model of the equipment feeds back the real-time prediction information to the physical entity equipment so as to drive the physical entity equipment to conduct independent evaluation and decision-making in the whole life cycle. In addition, based on the established refined digital twin model of typical port equipment, through the horizontal comparison of refined digital twin models of individual equipment in the same type, region, and scene, combined with the operation data of equipment in the region (subsystem), the equipment is classified by cluster analysis and simulation calculation, the information of atypical equipment is derived, and the integrated digital twin model of equipment in the same type, region, and scene is constructed, as shown in Figure 6.
5.2 Optimized operation and energy management of the system
Inshore cities are the core service objects of the IPES. Their main functions include absorbing new energy from the land to supply energy for industrial facilities, providing energy for the port microgrid, and providing ferries to and from various islands. After the port is highly electrified, the microgrid of the port undertakes the power network for the internal energy distribution of the port. It needs to accept the power from the coastal cities and also needs to absorb the new energy of the port, provide berths and shore power services for the docked cargo ships, and assign port machinery to undertake logistics tasks. The production and industrial raw materials provided by offshore drilling platforms, engineering construction ships, etc., are connected to the land/island through pipelines or transported by cargo ships. From the aforementioned operation of the IPES, it can be observed that the IPES realizes the two-way connection between land and sea from the transportation and energy sides.
With the connection of the sea–land energy system, the coupling management of transportation energy will become the key factor to realize the sea–land energy synergy. In order to reduce the energy consumption and operation cost of the system, on the basis of fully considering the transportation dispatching rules, by reasonably scheduling the arrival and departure of shore bridges and ships and coordinating the output of various equipment in the energy system, the ships and shore bridges can flexibly adjust their own loads on the basis of completing the logistics dispatching and give full play to the substitution role of different types of energy to improve the flexibility and economy of the system operation and promote the consumption of renewable energy and reduce carbon dioxide emissions. At present, the operation optimization of the IPES is mostly based on specific steady-state models. However, the production, transmission, conversion, and consumption characteristics of multiple types of energy in the actual system are difficult to describe by simple models but are closely related to the system operating environment, working conditions, and other factors. It is of great significance for the operation of the IPES to consider the refined operation scheduling of different devices.
Through the data acquisition and monitoring system, the operation status, weather, season, social factor data, scenery, energy storage, and controllable load status data of the system are obtained. Deep learning and extreme learning are used for state identification and multi-energy flow distribution autonomy, and the mirror system of the physical IPES in virtual space is built through the the mechanism and data-driven hybrid modeling methods. Complex factors such as dispatching response speed, conversion efficiency, equipment state, pipeline blockage, and transmission dissipation of various energy sources are presented in the digital space, and more comprehensive information is incorporated into the optimization scheduling problem. It realizes the refined scheduling and control of the whole process of production, transmission, and consumption of various energy forms and obtains the optimal strategy of energy management and control, thus enhancing the adaptability, robustness, and security of the energy management system and realizing the coordinated operation of multiple stakeholders. Taking advantage of both DT’s efficiency and accuracy in big data processing and information mining to integrate the internal and external data of the system, such as the comprehensive energy status of electricity, heat and gas, and transportation logistics data, it adopts the combination of offline learning and online decision-making to improve the calculation efficiency of online decision-making of energy collaborative management. The optimized operation decision in the application service is fed back to the physical system, as shown in Figure 7.
As shown in Figure 7, the states of ships, shore bridges, yard bridges, and other logistics equipment are modeled by 0–1 state variables, which are associated with the constraints related to logistics operations, which can not only reflect the characteristics of logistics operations but also represent the power load demand of ships and shore bridges so as to obtain the energy model of the port transportation system. The feasible solution set under different rules is obtained through the business rules of the port and brought into the " transportation–energy” coupling model to obtain the feasible solution set. The simplified model is obtained by simplifying the constraints, and the optimal scheduling strategy is obtained using the classical solution method. Finally, the optimal solution is obtained by learning according to the obtained template with the reinforcement learning method.
The following is the case that the project team takes a large port as the research object and uses the mechanism and data-driven hybrid modeling methods to carry out collaborative optimal scheduling of the port’s “traffic energy.” The first scheme is to operate the system without optimization, so the ships tend to dock and depart in the shortest time to reduce the berthing and waiting costs. However, the energy cost in this mode increases, resulting in the total cost being higher than that in the second scheme. The second scheme is that the system runs under the collaborative optimization scheduling method, which has a high scheduling cost. However, the energy system operation parameters are considered at this time so that the total system cost is reduced. The number of quayside bridges allocated in the two schemes is consistent, and the length of the ship in port is shown in Figure 8A.
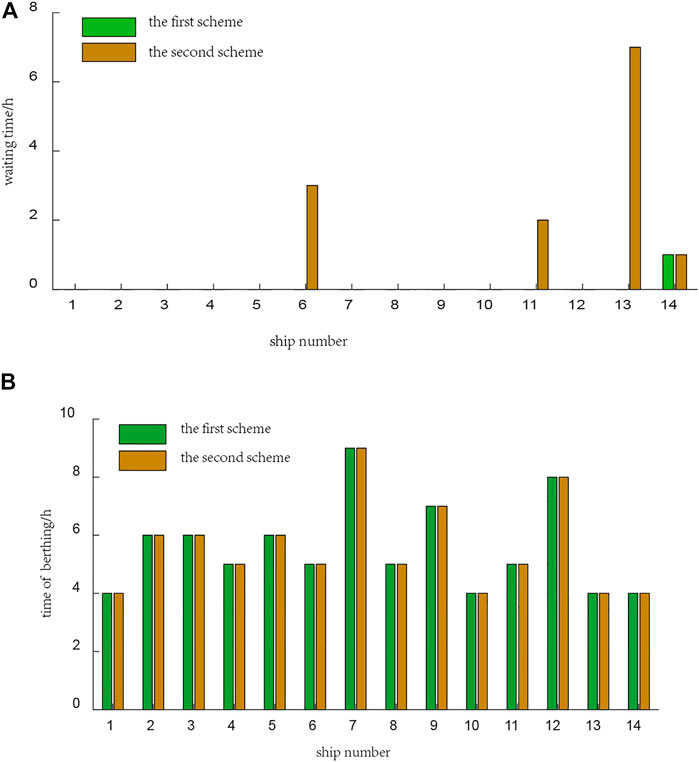
FIGURE 8. (A) Wating time of ships in port. (B) Time of berthing. Wating time and Berthing time of ships in port.
From Figure 8A, it can be observed that the length of ship berthing in Scheme 2 has increased, which indicates that the ship can reduce the cost by balancing the logistics cost and the energy cost and selecting the appropriate berthing time. Due to the limitation of the total number of quayside bridges and the ship’s own parameters, the ship berthing duration and quayside bridge allocation quantity under the two schemes are equivalent, as shown in Figure 8B, which shows that the proposed optimization method fully considers the ship scheduling and reduces the system operation cost in meeting the requirements of production operations.
The comparison of ship loads under the two schemes is shown in Figure 9. In Scheme 1, there is no obvious correlation between ship load distribution and electricity price, and there is no effect of reducing energy consumption costs. In Scheme 2, the ship can flexibly adjust the berthing time and its own load status according to the electricity price and coordinate with the energy system.
As shown in Figure 10, the load change trend of Scheme 2 is closer to the wind power output than that of Scheme 1, which shows that the coordinated optimization of transportation and energy enables ships to better match the wind power output. On the basis of meeting the production requirements, the distribution of load in time is adjusted so that the logistics system can flexibly adjust according to the energy system, realizing the interaction between the two systems.
5.3 System reliability analysis and evaluation
In the future, the operation reliability modeling of equipment in the IPES requires that the model can reflect the real-time operation status of equipment under the premise of continuous changes under operating conditions. The existing time-varying modeling based on the Markov multi-state method has some limitations because it does not consider the interaction between various fault factors. In terms of real-time information perception and online processing, the IPES-DT has obvious advantages. By means of the mechanism and data-driven hybrid modeling, it fully considers climate conditions, renewable energy output, load diversity, and coupling equipment and coupling information within the system. The IPES-DT is used to accurately model the time-varying characteristics of equipment operation, and its feedback mechanism is used to continuously learn and optimize the model. In addition, the link of operation reliability modeling needs to perceive and analyze a large number of operation data of IPES equipment. By using machine learning algorithms such as deep learning, the mapping of data relations can be established through self-learning, and the internal relations among various factors affecting the equipment operation reliability can be deeply explored. In addition, it is good at modeling and analyzing complex non-linear problems and has the potential to break the bottleneck of model-driven operation reliability modeling and improve the accuracy of modeling and subsequent operations (Li et al., 2019).
In terms of the evaluation index system of operation reliability, the IPES-DT adopts the mode of “offline training–online evaluation,” extracts the node operation risk evaluation index by using the data-driven method, mines the deeper internal relationship of the data set based on the learning of historical operation data on the basis of the mechanism model, and applies the model to the online evaluation link to improve the accuracy of the evaluation results. The online evaluation and analysis results of the real-time running state of the system are used to update the offline training model, which makes the real-time response ability of the evaluation stronger and efficiency higher. In this way, the multi-energy coupling degree and energy output priority can be fully considered to realize the comprehensive evaluation of IPES operation reliability in multiple dimensions and time scales, and the redundancy of information in the operation reliability evaluation index system can be considered to extract the important indicators in the index system. By mining the internal relations of different indicators, the coordination and cooperation between different indicators and the allocation of indicator weights can be realized (ZHU et al., 2022). The idea of IPES-DT operation reliability analysis and evaluation is shown in Figure 11.
6 Conclusion
The integrated port energy system is not a simple multi-network integration system, but it uses the effective connection of the basic physical equipment layer, the data acquisition and analysis layer, the intelligent decision-making layer, and the application service management layer to realize the efficient collaborative utilization of energy flow, information flow, and value flow. The integrated port energy system is affected by social behaviors such as management, service, and transaction in different spatial and temporal distribution ranges. The coupling mechanism is complex and the external influence factors are random and changeable. The traditional analysis, control, and optimization methods based on local mathematical modeling and computer simulation can no longer accurately, objectively, and comprehensively reflect the evolution characteristics of self-adaptation, self-optimization, and self-management.
As an important fusion and utilization means of massive data and extensive connections in the integrated port energy system, the digital twin organically combines the global and local features, rules, and experience of the system. Through the joint method with better performance, the integration of the decision support level is realized, and the advantages of the mechanism model and data model are given full play to overcome the outstanding problems such as imbalance and missing of original data categories, difficulty in the modeling mechanism model, and the generalization ability of the intelligent algorithm. It will make it possible for the energy system and all important parts of the port to be interconnected and operated cooperatively in the digital space and finally realize the high proportion of renewable energy consumption and the improvement of energy utilization efficiency.
Data availability statement
The original contributions presented in the study are included in the article/Supplementary Material; further inquiries can be directed to the corresponding author.
Author contributions
PY, WZ, and GY are responsible for writing and translating; TN and WJ are responsible for provision of the materials and review.
Funding
The project is supported by the National Natural Science Foundation of China (51807117); National Key R&D Program of China (2019YFE0102900); Core Innovation Project of Shanghai Municipal Education Commission (2019-01-07-00-02-E00044).
Conflict of interest
The authors declare that the research was conducted in the absence of any commercial or financial relationships that could be construed as a potential conflict of interest.
Publisher’s note
All claims expressed in this article are solely those of the authors and do not necessarily represent those of their affiliated organizations, or those of the publisher, the editors, and the reviewers. Any product that may be evaluated in this article, or claim that may be made by its manufacturer, is not guaranteed or endorsed by the publisher.
References
Ertem, A., İşbilir, M., and Arslan, A. (2017). Review of intermodal freight transportation in humanitarian logistics. Eur. Transp. Res. Rev. 9 (1), 10. doi:10.1007/s12544-017-0226-z
Fang, S., and Wang, H. (2021). Optimization-based energy management for multi-energy maritime grids. Singapore: Springer. doi:10.1007/978-981-33-6734-0
Fang, S., Wang, Y., Gou, B., and Xu, Y. (2019). Toward future green maritime transportation: An overview of seaport microgrids and all-electric ships. IEEE Trans. Veh. Technol. 69 (1), 207–219. doi:10.1109/tvt.2019.2950538
Fang, S., Zhao, C., Ding, Z., Zhang, S., and Liao, R. (2022). Port integrated energy systems toward carbon neutrality (II): Flexible resources and key technologies in energy-transportation integration. Proc. CSEE, 1–20. doi:10.13334/j.0258-8013.pcsee.212121
Glaessgen, E. H., and Stargel, D. S. (2012). The digital twin paradigm for future NASA and U.S. air force vehicles, Proceedings of the 53rd Structures, Structural Dynamics, and Materials Conference, Honolulu, HI, USA, 2012 (Honolulu, HI: AIAA). doi:10.2514/6.2012-1818
Guo, M., Xia, M., and Chen, Q. (2021). A review of the energy-cyber-transportation-social coupling network based on energy self-organization. Proc. CSEE 41 (16), 5521–5539. doi:10.13334/j.0258-8013.pcsee.202231
He, X., Ai, Q., Qiu, R. C., Huang, W., Piao, L., and Liu, H. (2017). A big data architecture design for smart grids based on random matrix theory. IEEE Trans. Smart Grid 8 (2), 674–686. doi:10.1109/TSG.2015.2445828
He, X., Ai, Q., Zhu, T., Qiu, C., and Zhang, D. (2021). Opportunities and challenges of the digital twin in power system applications. Power Syst. Technol. 44 (6), 2009–2019. doi:10.13335/j.1000-3673.pst.2019.1983
Huang, Y., Huang, W., Wei, W., Tai, N., and Li, R. (2022). Logistics-energy collaborative optimization scheduling method for large seaport integrated energy system. Proc. CSEE 42 (17), 1–12. doi:10.13334/j.0258-8013.pcsee.211093
Li, F., Wang, Q., Hu, J., and Tang, Y. (2021). Combined data-driven and knowledge-driven methodology research advances and its applied prospect in power systems. Proc. CSEE 41 (3), 4377–4389. doi:10.13334/j.0258-8013.pcsee.202468
Li, G., Huang, Y., Bie, Z., An, J., and Liu, Y. (2019). Review and prospect of operational reliability evaluation of integrated energy system. Electr. Power Autom. Equip. 39 (8), 12–21.
Mutarraf, M., Terriche, Y., Nasir, M., Guan, Y., Su, C. L., Vasquez, J. C., et al. (2021). A communication-less multimode control approach for adaptive power sharing in ship-based seaport microgrid. IEEE Trans. Transp. Electrific. 7, 3070–3082. doi:10.1109/tte.2021.3087722
Pang, Y., Huang, W., Wu, J., He, X., Tai, N., and Hu, S. (2022). Prospect and key technology technologies for the applications of digital twin for shipboard integrated power system. Power Syst. Technol. 46 (7), 2456–2471. doi:10.13335/j.1000-3673.pst.2021.2424
Sadiq, M., Ali, S., Terriche, Y., Mutarraf, M. U., Hassan, M. A., Hamid, K., et al. (2021). Future greener seaports: A review of new infrastructure, challenges, and energy efficiency measures. IEEE Access 9, 75568–75587. doi:10.1109/access.2021.3081430
Sifakis, N., Konidakis, S., and Tsoutsos, T. (2021). Hybrid renewable energy system optimum design and smart dispatch for nearly Zero Energy Ports. J. Clean. Prod. 310, 127397. doi:10.1016/j.jclepro.2021.127397
Sun, L., Huang, W., Li, R., Gao, F., Tai, N., and Yu, M. (2022). Capacity and volume balance of buffering converters for the marine pulsed power system. IEEE Trans. Ind. Electron. 70, 322–333. doi:10.1109/TIE.2022.3153828
Tan, C., Yan, W., and Yue, J. (2021). Quay crane scheduling in automated container terminal for the trade-off between operation efficiency and energy consumption. Adv. Eng. Inf. 48, 101285. doi:10.1016/j.aei.2021.101285
Tao, F., Zhuang, H., Liu, A., and Nee, A. Y. C. (2019). Digital twin in industry: State-of-the-art. IEEE Trans. Ind. Inf. 15 (4), 2405–2415. doi:10.1109/tii.2018.2873186
Wang, W., Peng, Y., Li, X., Qi, Q., Feng, P., and Zhang, Y. (2019). A two-stage framework for the optimal design of a hybrid renewable energy system for port application. Ocean. Eng. 191, 106555. doi:10.1016/j.oceaneng.2019.106555
Wang, C., Dong, B., Yu, H., Wu, J., Yan, J., and Li, P. (2021a). Digital twin technology and its application in the integrated energy system of smart city. Proc. CSEE 41 (5), 1597–1607. doi:10.13334/j.0258-8013.pcsee.201804
Wang, X., Huang, W., Wei, W., Tai, N., Li, R., and Huang, Y. (2021b). Day-ahead optimal economic dispatching of integrated port energy systems considering hydrogen. IEEE Trans. Ind. Appl. 58, 2619–2629. doi:10.1109/TIA.2021.3095830
Yigit, K., and Acarkan, B. (2018). A new electrical energy management approach for ships using mixed energy sources to ensure sustainable port cities. Sustain. Cities Soc. 40, 126–135. doi:10.1016/j.scs.2018.04.004
Yigit, K., Kokkulunk, G., Parlark, A., and Karakas, A. (2016). Energy cost assessment of shoreside power supply considering the smart grid concept: A case study for a bulk carrier ship. Marit. Policy & Manag. 43 (3/4), 469–482. doi:10.1080/03088839.2015.1129674
Zhang, N., Ma, G., Guan, Y., Hu, J., Lu, C., Wen, J., et al. (2021). Panoramic information perception and intelligent grid. Proc. CSEE 41 (4), 1274–1283. doi:10.13334/j.0258-8013.pcsee.202508
Zhu, J., Luo, T., Wu, W., Li, S., and Dong, H. (2022). A review of operational reliability assessment of integrated energy systems Ⅱ: Data-driven method and model-data hybrid driven method. Trans. China Electrotech. Soc. 37 (13), 3227–3240. doi:10.19595/j.cnki.1000-6753.tces.210928
Keywords: digital twin, integrated port energy system, green low-carbon port, energy transportation value, framework design
Citation: Yu P, Zhaoyu W, Yifen G, Nengling T and Jun W (2023) Application prospect and key technologies of digital twin technology in the integrated port energy system. Front. Energy Res. 10:1044978. doi: 10.3389/fenrg.2022.1044978
Received: 15 September 2022; Accepted: 05 December 2022;
Published: 12 January 2023.
Edited by:
Wentao Huang, Shanghai Jiao Tong University, ChinaCopyright © 2023 Yu, Zhaoyu, Yifen, Nengling and Jun. This is an open-access article distributed under the terms of the Creative Commons Attribution License (CC BY). The use, distribution or reproduction in other forums is permitted, provided the original author(s) and the copyright owner(s) are credited and that the original publication in this journal is cited, in accordance with accepted academic practice. No use, distribution or reproduction is permitted which does not comply with these terms.
*Correspondence: Pang Yu, OTg2NTUwMTQ5QHFxLmNvbQ==