- 1Department of Biology, College of Natural Sciences, Kyungpook National University, Daegu, South Korea
- 2School of Life Sciences, BK21 FOUR KNU Creative BioResearch Group, Kyungpook National University, Daegu, South Korea
- 3Advanced Bio-Resource Research Center, Kyungpook National University, Daegu, South Korea
- 4Department of Hydrogen and Renewable Energy, Kyungpook National University, Daegu, South Korea
Pharmaceutical and personal care products (PPCPs) are discharged into receiving water bodies mainly from sewage treatment plants. Due to the inefficient removal in conventional wastewater treatment facilities, PPCPs have become a major concern to aquatic ecosystems, water quality, and public health worldwide since they cause harmful effects on aquatic life and human even at low doses. Among the PPCPs, carbamazepine (CBZ) is one of the most commonly prescribed anticonvulsant drugs and consumed more than 1,000 tons per year. Due to its structural complexity, CBZ is known as recalcitrant compound highly stable during wastewater treatment. Consequently, it has become one of the most frequently detected pharmaceuticals in waste water, surface water, and even drinking water. In this study, Korean indigenous microalgae strains were tested as eco-friendly and cost-effective solutions for CBZ removal. Based on the preliminary biological CBZ degradation tests, Tetradesmus obliquus KNUA061 demonstrating the best CBZ removal rate was selected for further experiments. In order to increase strain KNUA061's CBZ removal efficiency, NaOCl, which is widely accepted in the water purification process, was used as an additional stimulus to induce stress conditions. At around 20 μg L−1 CBZ, addition of 1.0 mg NaOCl resulted in approximately 20% of removal rate increase without suppressing cells growth. Roughly 90% of CBZ remained its original form and the composition of the transformed secondary metabolites was less than 10% during the biodegradation process by the microalga. Based on the results of the antioxidant enzyme activities, degree of lipid oxidation, and amino acid contents, it was concluded that the redox-defence system in microalgal cells may have been activated by the NaOCl treatment. Biomass analysis results showed that higher heating value (HHV) of strain KNUA061 biomass was higher than those of lignocellulosic energy crops suggesting that it could be utilized as a possible renewable energy source. Even though its biodiesel properties were slightly below the international standards due to the high PUFA contents, the biodiesel produced from T. obliquus KNUA061 could be used as a blending resource for transportation fuels. It was also determined that the microalgal biomass has acceptable feasibility as a sustainable dietary supplement feedstock due to its high essential amino acid contents.
1 Introduction
Emerging micropollutants can be divided categories such as personal care products, pesticides, pharmaceuticals, plasticizers, and preservatives, which are produced and consumed thousands of tons per year and released into various ecosystems (Rempel et al., 2021). PPCPs are defined as “any product used by individuals for personal health or cosmetic reasons.” by the United States Environmental Protection Agency. All PPCPs have complex molecules and they comprise a diverse group of chemicals including all human and veterinary drugs and non-medicinal consumer chemicals such as personal hygiene products and household cleaning detergents. PPCPs are released to the aquatic environments by multiple sources including human excretion in urine and feces, discharge from pharmaceutical manufacturers, and residues from agricultural activities (Al-Baldawi et al., 2021). Among all these sources, human contribution by discharge into the sewer systems is one of the major PPCPs sources in the environment. Thereby, the main pollutant source of PPCPs remaining in surface water that is used as drinking and irrigation water is sewage treatment effluent.
Due to the improvement of living standards, a large amount of medicines and personal hygiene substances have been discharged into the water system, resulting in an increase in the frequency of detection and concentration of PPCPs every year (Boxall et al., 2012). Although not every PPCP is necessarily detrimental to human and aquatic organisms, numerous studies have demonstrated that some of these chemicals could act as toxicants to a variety of biological processes in ecosystems (Chee-Sanford et al., 2001; Ash et al., 2002; Costanzo et al., 2005; Chen et al., 2008; Hoppe et al., 2012; Rosi-Marshall and Royer 2012; Rosi-Marshall et al., 2013; Richmond et al., 2017).
Because of the recalcitrant physicochemical properties of PPCPs, a complete removal of PPCPs by conventional wastewater treatment processes is not feasible and thereby their presence in drinking water poses a potential threat to aquatic life and public health (Snyder 2008; Wee et al., 2020). It has been reported that PPCPs and their secondary metabolites are biologically active and they can be accumulated in aquatic organisms especially in fish (Ebele et al., 2017; Muir et al., 2017; Yin et al., 2017). Hence, water quality management to reduce the concentration of PPCP in inflow sewage and discharged water and preventive measures for recalcitrant PPCP pollutants with high detection frequency and concentration are required.
Priority lists of pollutants have been developed by the United States Environmental Protection Agency (USEPA) and European Union (EU) aimed at identifying substances that may pose a risk to receiving waters and require regulation since 1970s. In 2007, PPCPs such as diclofenac, iopamidol, musks, and carbamazepine were identified as future emerging priority candidates (Ellis, 2008; Ebele et al., 2017). Then, the European Commission established regulatory guidelines (Directive 2013/39/EU) to monitor the pharmaceuticals in aquatic environments and ibuprofen, clofibric acid, triclosan, phthalates, and bisphenol A were also added to this watch list (EU Decision 2018/840, Loos et al., 2018; Alaoui et al., 2021).
PPCPs are either non-volatile or semi-volatile (Delorenzo and Fleming 2008) and they are generally hydrophilic compounds with a high water solubility (Ohoro et al., 2022). These physiochemical properties of PPCPs indicate that their distribution in nature mainly occurs through aqueous transport (Caliman and Gavrilescu 2009). PPCPs enter the environment as they pass-through the human body or when unused and/or unwanted PPCPs are improperly disposed of down the drain. Consequently, PPCPs are found virtually everywhere where domestic wastewater is discharged. Therefore, municipal wastewater treatment plants play a crucial role in safeguarding our ecosystems and public health. In the waste water treatment facilities, transformation of PPCPs occur depending on the structures and properties of each compound and the conditions of the operations. During the process, they may be completely or partially broken down or may remain intact in some cases (Xia et al., 2005; Kosma et al., 2014; Yang et al., 2017). In general, PPCP compounds that are frequently detected in wastewater effluents were recalcitrant or only partially degraded by conventional wastewater treatment facilities (Reyes et al., 2021). Previous studies have shown that PPCP removal during primary wastewater treatment which include coagulation, flocculation, and sedimentation is relatively insignificant (Oluwole et al., 2020). More advanced and complex wastewater treatment approaches which include membrane bioreactors, sand filtration, ultra-, micro-, and nanofiltration, reverse osmosis, activated carbon, and chemical oxidation via ozonation may enhance PPCP removal rates from wastewater, but these processes are chemically and operationally intensive and therefore they require large systems, infrastructure, and high operational costs (Oulton et al., 2010).
PPCPs can be degraded via abiotic transformation including hydrolysis, photodegradation, reductive catalysis, volatilization, and ozonation (McDowell et al., 2005; Kim and Tanaka 2009; Calza et al., 2012; König et al., 2016; Maldonado-Torres et al., 2018; Wang et al., 2020b; Bonnot et al., 2022) and biological transformation such as bioaccumulation, adsorption, and intracellular and extracellular biodegradation by a verity of microorganisms such as bacteria, fungi, and microalgae (Kang et al., 2008; Jelic et al., 2012; Hena et al., 2021; Morais et al., 2021; Narayanan et al., 2022). Recently, microalgae-based PPCP bioremediation has gained increasing scientific attention due to its low operational costs and carbon-neutral natures (Hassaan et al., 2017; Daneshvar et al., 2018). Microalgae offer several advantages over bacteria and fungi as they do not require carbon sources for growth and PPCP breakdown and various microalgal species are capable of bioremediation of PPCPs (Abdelfattah et al., 2023).
Among many PPCPs, carbamazepine (CBZ) is one of the most widely used antiepileptic drugs in the world to treat neurosis and bipolar disorder (Mojiri et al., 2021). Approximately 1,014 tons of CBZ are consumed annually worldwide (Zhang et al., 2008). Consequently, CBZ that cannot be completely metabolized by the human body usually flow into receiving water bodies and it is one of the most frequently detected PPCPs in wastewater treatment plants around the world (Zhang et al., 2008; Zhang et al., 2018; Seo et al., 2020; Huang et al., 2022; Ma et al., 2022). However, CBZ has been reported to have very low removal efficiency (7%–10%) in conventional wastewater treatment facilities (Zhang et al., 2008) due to its relatively low attenuation rate during both abiotic and biotic processes (Baena-Nogueras et al., 2017; Thanekar et al., 2018; Dao et al., 2022). Many researches have been attempted related to the chemical oxidation of CBZ in aqueous solution in conventional advanced oxidation processes via the generation of strong oxidizing oxygen species such as O3/UV/H2O2 (Im et al., 2012; Esquerdo et al., 2021; Schoenell et al., 2022), UV/H2O2 (Vogna et al., 2004; Keen et al., 2012; Liu et al., 2015; Alharbi et al., 2017), photocatalytic (Doll and Frimmel 2005; Xu et al., 2013; Carabin et al., 2016; Yang et al., 2019), and photo-Fenton (Klamerth et al., 2010; Guo et al., 2021; Villota et al., 2021; Gong et al., 2022; Sun et al., 2022). Some of these methods achieved a 100% removal rate from different concentrations of CBZ in wastewater (Supplementary Table S1). However, these technologies use harsh chemicals and some of the catalysts may act as additional contaminants in the aquatic environments (Mohapatra et al., 2014). Moreover, higher capital and operating costs and energy input and generation of additional toxic by-products attribute for the limited acceptance of the advanced oxidation processes in CBZ treatment (Wang and Zhu 2016; Zhai et al., 2018; Wang et al., 2020a). Hence, it is needed to find improved remediation solutions for CBZ-contaminated wastewater that may threat aquatic ecosystems and human’s health.
In this study, CBZ removal screening was conducted using 12 microalgal strains previously isolated by our research group from primary settled wastewater in the local municipal wastewater treatment plants. The removal rate of CBZ and its secondary metabolites were analyzed using the selected elite microalgal strains. In addition, it was investigated whether bioremediation efficiency could be increased by stimulating the microalgal redox system. NaOCl was used as a stressor as it is generally used for sterilization in water treatment. The physiological and biochemical characteristics of the resulting microalgal biomass were investigated to examine its potential for use as bioenergy and high-value products.
2 Materials and methods
2.1 Chemicals
All the chemicals used in this study were analytical grade. CBZ (purity: ≥ 99.9%) was purchased from Sigma-Aldrich (St. Louis, MO, United States) and NaOCl was obtained from Duksan (Ansan, Korea), respectively. Methanol from Merck (Darmstadt, Germany) and carbamazepine-10,11-epoxide (EP-CBZ), 10,11-dihydrocarbamazepine (DI-CBZ), 9-acridine carboxaldehyde (= acridine-9-carbaldehyde), 3-hydroxy carbamazepine (3-OH-CBZ), and 10,11-dihydro-10-hydroxy carbamazepine (DiOHCBZ) manufactured by Sigma-Aldrich were used in this research.
2.2 Selection of microalgal strains and growth conditions
In the preliminary experiments, a total of 13 microalgal strains were tested for their CBZ-degrading capabilities at a concentration of approximately 1.0 mg L−1 CBZ (Supplementary Table S6). Four best microalgae with the highest removal rates were selected and test for the second CBZ degradation tests at a concentration of about 250 μg L−1 (Supplementary Table S7). Tetradesmus obliquus strain KNUA061 isolated from a wastewater treatment plant was finally chosen as the best candidate for further CBZ removal experiments. Each culture was inoculated in 1 L Erlenmeyer flasks containing 500 ml BG-11 medium (Sigma-Aldrich) with an initial CBZ concentrations of around 50 μg L−1 and then 20 μg L−1. All samples were cultivated at 25°C with shaking at 120 rpm and supplying with 1% CO2 under approximately 100 μmol m−2 s−1 of light intensity in 16-h/8-h light/dark cycle. NaOCl was added as a stimulant to enhance CBZ removal efficiency at three different concentrations (1 mg L−1, 5 mg L−1, and 10 mg L−1) that did not significantly inhibit microalgal growth as confirmed in the preliminary tests.
2.3 Microalgal growth characteristics
Microalgal biomass productivity was measured as dry weight (DW) using a gravimetric method proposed by the American Public Health Association. Microalgal growth rates were monitored by measuring the optical density (OD at 680 nm) at 5 days intervals using an Optimizer 2120 UV spectrophotometer (Mecasys, Daejeon, Korea). In addition, pH was measured using a pH meter (Seven Easy, Mettler Toledo, Columbus, OH, United States) to determine the effects of CBZ and NaOCl on microalgal cultivation.
Microalgal growth rates were monitored by measuring the optical density (OD at 680 nm) and specific growth rate (μ) calculated as: μ = ln (N2/N1)/(t2−t1), where N2 and N1 refer to optical density at times t2 and t1 during the exponential growth phase (Levasseur et al., 1993; Humphrey et al., 2021).
After 20 days of cultivation, chlorophyll a, chlorophyll b, and total carotenoid contents were measured. Two milliliters of the culture was centrifuged (Centrifuge 5424, Eppendorf, Hamburg, Germany) and washed twice with distilled water. The harvested cell pellets were suspended in 2 ml of methanol and disrupted in an ice bath using ultrasonication (550 Sonic Dismembrator, Fisher Scientific, Hampton, NH, United States) for 5 min. The extracted solution was incubated at 4°C for 24 h in the dark and centrifuged at 16,022 g for 10 min. The cleared supernatant was measured using a spectrophotometer at 470, 653, and 666 nm. Pigment contents were calculated using the following equations (Wellburn, 1994):
2.4 Measurement of CBZ removal efficiency and metabolites
Abiotic controls were included in the experiments to investigate photolysis-driven and NaOCl-driven degradations of CBZ along with the microalgae cultures supplemented with different levels of NaOCl as a stimulator (Figure 2). The samples were collected every 5 days, filtered through a 0.20 μm membrane filter (Sartorius Minisart, Göttingen, Germany), and subjected to high-performance liquid chromatography (HPLC; Agilent 1,200 series, Agilent, Santa Clara, CA, United States) with a mobile phase of 40:60 (v/v) water and methanol. The solution was separated using a SunFire column C18 (2.4 × 250 mm, 5 μm, (Waters, Milford, MA, United States) at a flow rate of 1 ml min−1, column temperature of 40 ± 1.0°C, and sample injection volume of 5 μL. CBZ was detected using a diode array detector set to 285 nm. All experiments were conducted in triplicate.
For secondary metabolite analysis, strain KNUA061 was cultivated in BG-11 medium supplied at 20 μg L−1 CBZ without NaOCl (control) and with 1 mg L−1 NaOCl (treatment group), respectively. Acquity ultra high sensitivity (UPLC) H-Class Plus system with Xevo TQ-S micro triple quadrupole mass spectrometry (Waters) was used to analyze CBZ and its metabolites with a mobile phase of 50:50 (v/v) water and acetonitrile (Sigma-Aldrich) with 0.1% formic acid (Sigma-Aldrich). Chromatographic separation of the target compounds was achieved on an Acquity BEH C18 column (2.1 × 100 mm, 1.7 µm, Waters) at a flow rate of 0.2 ml min−1 and column temperature at 40°C ± 5.0°C.
2.5 ROS-related redox system
2.5.1 Measurement of ROS production
Oxidation-sensitive fluorescent probe, H2DCFDA, was used to analyze intracellular ROS levels according to previously reported publication by Astuya et al. (2018), except for washing the cells with phosphate-buffered saline (PBS). PBS is a widely used solution in many biological research applications before fluorescence dye staining since it is isotonic and non-toxic to cells (Tripathi et al., 2015; Jeyakumar et al., 2020; Yan et al., 2021; Nigam et al., 2022). Cells treated with NaOCl and CBZ were harvested, washed twice with PBS (Sigma-Aldrich), and incubated at a final concentration of 5 μMH2DCFDA at 30°C for 30 min in the dark. The cells were then centrifuged, washed twice with PBS, and diluted with 1 ml PBS. Fluorescence images were obtained using a super-resolution confocal laser scanning microscope (LSM 800 with Airy scan, Carl Zeiss) for H2DCFDA (green; excitation at 495 nm, emission at 520 nm). The fluorescence was also analyzed using flow cytometric analysis with a BD FACSAria III (Becton Dickinson and Company, New Jersey, NJ, United States) using 10,000 events.
2.5.2 Measurement of redox status
Microalgal samples were harvested after 24 h with 1 mg L−1 NaOCl and approximately 20 μg L−1 CBZ and centrifuged at 1,516 g for 10 min at 4°C. The pellet was resuspended in PBS, extracted in an ice bath using a probe-type sonicator (Sonics Vibra Cell VC 505, Sonics & Materials, Newtown, CT, United States), and centrifuged at 16,022 g for 10 min at 4°C. The supernatant was transferred to a new tube and used for the antioxidant enzyme assays. A superoxide dismutase (SOD), catalase (CAT), and peroxidase (PER) activities in the samples were measured using BO-SOD-250, BO-CAT-400, and BO-PER-500 assay kits (Biomax, Seoul, Korea), respectively, according to the manufacturer’s instructions.
To confirm the non-enzymatic antioxidant system, the TBARS assay was conducted using a TBARS assay kit (BO-TBR-200, Biomax) in order to observe the change in MDA content as an indicator of lipid peroxidation. BODIPY 581/591 was used to analyze intracellular lipid peroxidation levels, as described previously (Domínguez-Rebolledo et al., 2010). Cells were treated with 1 mg L−1 NaOCl and 20 μg L−1 CBZ, harvested, washed twice with PBS, and incubated at a final concentration of 5 µM BODIPY 581/591 at 25°C for 30 min in the dark. The cells were then centrifuged, washed twice with PBS, and diluted with 1 ml PBS. Flow cytometric analysis was conducted using BD FACSAria III with 10,000 events (excitation at 488 nm, emission at 530 nm).
2.6 Characteristics of the resulting microalgal biomass
2.6.1 Proximate and ultimate analyses
Microalgal cultures were harvested after 20 days of cultivation. Biomass samples of the non-treated control, CBZ treatment, and CBZ + NaOCl treatment groups were prepared to compare their characteristics. The harvested microalgal biomass was lyophilized, pulverized, and sieved through an ASTM 230 mesh (63 μm, Chunggye, Seoul, Korea). Pulverized biomass was used for further analysis. During proximate analysis, 10 mg of biomass was used to measure moisture, volatile matter, and ash content using a thermal analyzer (Shimadzu, Kyoto, Japan). Nitrogen gas was supplied at a rate of 25 ml min−1, and the biomass was heated from 50°C to 900°C at a rate of 10°C min−1.
Ultimate analysis was conducted with a Flash 2000 elemental analyzer (Thermo Fisher Scientific, Milan, Italy) to determine C, H, N, and S contents. The oxygen (O) content was calculated by subtracting the contents of ash and CHNS from the total contents.
The HHV was determined using an equation developed by Given et al. (1986), where C, H, and S contents are provided by the elemental analysis and O content from the proximate analysis.
HHV (MJ kg−1) = 0.3278C + 1.419H + 0.09257S − 0.1379O+ 131N + 0.637.
2.6.2 Biochemical composition of the microalgal biomass
To determine carbohydrate content, 50 mg of freeze-dried biomass was hydrolyzed with 2.5 ml 2 N sulfuric acid (Sigma-Aldrich) at 94°C for 3 h (Jo et al., 2020b). Then the reaction tubes were cooled to ambient temperature, 40% CaCO3 (Sigma-Aldrich) was added to the hydrolysates to neutralize the reaction. Samples were then filtered through a 0.2-μm polytetrafluoroethylene (PTFE) filter (Whatman, Florham Park, NJ, United States) and soluble carbohydrate contents (sucrose, maltose, lactose, glucose, xylose, galactose, fructose, fucose, and arabinose) were determined by HPLC with refractive index detector (HPLC-RI, Shimadzu, Kyoto, Japan). The total carbohydrate content was determined using the phenol-sulfuric acid method described by Mecozzi (2005).
The nitrogen (N) content was determined using ultimate analysis, and the total protein content was calculated from the N content using the conversion factor (× 6.25) (Mariotti et al., 2008). Biomass powder (500 mg) was used to quantitatively determine free amino acid content using an amino acid analyzer (L-8900, Hitachi, Tokyo, Japan).
Total lipid content was measured using the sulfo-phospho-vanillin method (Mishra et al., 2014) and fatty acid methyl ester (FAME) was extracted according to the method described by Breuer et al. (2013) with slight modifications. The harvested biomass samples were freeze-dried and pulverized to enhance the extraction efficiency. Ten mg of biomass was placed in a 1.5 ml tube and the same volume of glass beads (1 mm, Glass Beads 1; Glastechnique, Wertheim, Germany) was added to the tube. Then, 1 ml of CHCl3:MeOH mixture (4:5, v/v, Sigma-Aldrich) was added to the tube and it was bead-beated 8 times in a microtube mixer (MicroMixer E-36, Taitec, Saitama, Japan) for 1 min. The solution was transferred to a 11 ml glass tube and it was vortexed for 5 s and sonicated at 40 kHz and 60°C in an ultrasonic bath (Branson 5800, Branson Ultrasonics, Sterling Heights, MI, United States) for 10 min. Then, 2.5 ml of distilled water containing 50 mM Tris (Sigma-Aldrich) and 1 M NaCl (Duksan) was added to the glass tube and it was vortexed and sonicated again. After the second sonication, the glass tubes were centrifuged for 5 min at 1,200 g and the chloroform phase at the bottom was transferred to a new glass tube using a glass Pasteur pipette (Hilgenberg, Malsfeld, Germany). To further extract the remaining lipid, 1 ml of chloroform was added to the previous tube. Vortexing, sonication, and centrifugation steps were repeated. Collected chloroform phases were evaporated in a rotary evaporator (RV 10, IKA, Staufen, Germany). For transesterification to FAME, 3 ml of methanol containing 5% (v/v) sulfuric acid (Sigma-Aldrich) was added to the glass tube containing the dried extracted lipids and vortexed for 5 s. The tube was then incubated for 3 h at 70°C in a water bath (Daihan Labtech, Namyangju, Korea) with vortexing the tube every 30 min. The tube was cooled down to room temperature and 3 ml of distilled water and 3 ml of hexane were added to glass tubes. Then, it was vortex for 5 s and mixed on an orbital shaker (TW3, FinePCR, Gunpo, Korea) for 15 min. After shaking, the glass tubes were centrifuged for 5 min at 1,200 g and 2 ml of the hexane phase on the top layer was transferred into a new glass tube. In order to wash the collected hexane phase, 2 ml of distilled water was added, vortexed for 5 s, and centrifuge for 5 min at 1,200 g. The hexane phase was then filtered through a 0.2 μm polyvinylidene fluoride (PVDF) syringe filter (Chromdisc, Hwaseong, Korea). FAME composition was analyzed using gas chromatography (GC) mass spectrometry (Agilent 7890A GC equipped with 5975C MSD; Agilent) with a DB-FFAP column (30 m × 250 μm × 0.25 µm, Agilent).
Biodiesel quality was determined by assessing the degree of unsaturation (DU), long-chain saturated factor (LCSF), saponification value (SV), iodine value (IV), cetane number (CN), cold filter plug-going point (CFPP), kinematic viscosity at 40°C (υ), density at 20°C (ρ), and oxidative stability, which were all calculated based on the fatty acid profiles, using the equations below; EN 14214 were the standards used to evaluate biofuel quality (Sakthivel et al., 2018):
Where MUFA is monounsaturated fatty acid, PUFA is polyunsaturated fatty acid, F is the percentage, MW is the molecular weight, N is the number of double bonds, and X is the content of linoleic and linolenic acids in each FAME value. These equations have been previously validated for use in the estimation of microalgae-based biofuel quality (Francisco et al., 2010).
2.7 Statistical analyses
All experiments were conducted in triplicate (at least). All data are indicated as the average of triplicate measurements, and error bars represent the standard deviation (SD). GraphPad Prism 9.0 (GraphPad Software, San Diego, CA, United States) was used to analyze the experimental data. One-way analyses of variance, followed by Dunnett’s multiple comparison tests, was used to perform statistical analyses of the data. The significance level was set at 95% (p-value < 0.05).
3 Results
3.1 Microalgal strain and growth characteristics
The first preliminary test was carried out to screen elite microalgae with high CBZ removal rates among the 13 strains (Supplementary Figure S1; Supplementary Table S6). The second removal rate test was conducted using four best candidates (strains KNUA061, KNUA064, KNUA068, and KNUA069) that showed the highest removal rates in the first screening test (Supplementary Tables S6, S7). Among these microalgae, strain KNUA061 was found to be the most promising one (Supplementary Figure S1) and it was subjected to further CBZ-degradation tests. Next, NaOCl was used as a stressor to promote metabolic process in the microalga of interest and growth rate was measured under different concentrations of NaOCl. In this study, 1 mg L−1 NaOCl resulted in the lowest growth inhibition and highest growth rate (Supplementary Figure S2).
To improve CBZ removal rate of T. obliquus KNUA061, three different NaOCl concentrations (1 mg L−1, 5 mg L−1, and 10 mg L−1) were used and growth characteristics of the microalga were monitored. OD results demonstrated that 1 mg L−1 NaOCl treatment achieved relatively high growth rate, almost similar to that of the control (without NaOCl addition), while 5 mg L−1 NaOCl-treated group showed slightly diminished growth rate. Growth rate increased fractionally in the 10 mg L−1 NaOCl treatment group until the 10th day of culturing and after that point exponential growth was recorded (Figure 1A). pH values also showed similar patterns to those of the OD results. In particular, relatively low pH values were observed during the lag and exponential growth phases in the 10 mg L−1 NaOCl treatment (Figure 1B). DW was measured after 20 days of cultivation and the control and 1 mg L−1 NaOCl treatment groups demonstrated similar biomass productivities of 2.95 and 2.96 g L−1, respectively. Productivities for 5 mg L−1 and 10 mg L−1 treatment groups were 2.81 and 2.21 g L−1, respectively (Figure 1C). Chlorophyll and total carotenoid contents were the highest in 1 mg L−1 treatment group (Figure 1D).
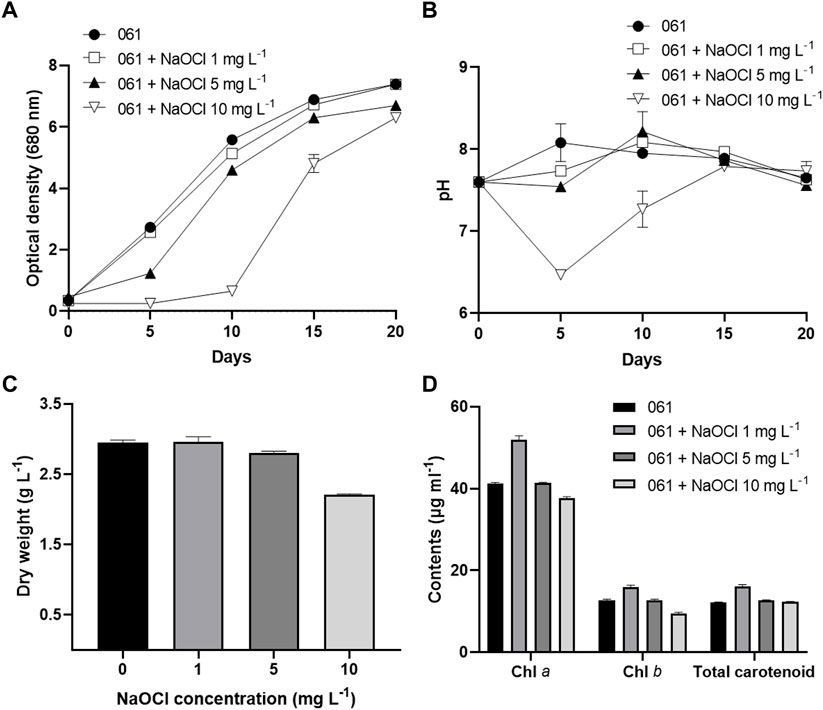
FIGURE 1. Growth characteristics of T. obliquus KNUA061 under different NaOCl concentrations. OD (A) and pH (B) of T. obliquus KNUA061 during the 20 days of cultivation. DW (C) and chlorophyll and total carotenoid contents (D) of microalgal biomass after 20 days of cultivation.
3.2 CBZ removal efficiency and metabolites
CBZ removal rates were analyzed in T. obliquus KNUA061 cultures with different NaOCl concentrations. The highest removal rates were achieved by the microalgal culture with 1 mg L−1 NaOCl which also resulted in high growth rates when initial CBZ concentration of around 50 μg L−1 was used (Supplementary Table S4). The differences between the growth rates were narrowed in the later part of all the microalgal culture and around 30% of final CBZ removal rates were obtained in all the NaOCl treatment groups (Figure 2A and Supplementary Table S4). Since there is only a minute amount of CBZ exist in wastewater plants and surface waters in general, our second CBZ degradation experiment was conducted as low as at around 20 μg L−1. More clear differences between the treatment groups were observed. Abiotic removal rates of the photolysis control and NaOCl groups were 14.6% and 19.5%, respectively, whereas the biotic control of strain KNUA061 only culture (without NaOCl) showed 62.5% of removal rate (Supplementary Table S5). Removal rates of the NaOCl-added microalgal culture groups were 82.0% for 1 mg L−1 NaOCl, 70.5% for 5 mg L−1 NaOCl, and 68.5% for 10 mg L−1 NaOCl, respectively (Figure 2B and Supplementary Table S5).
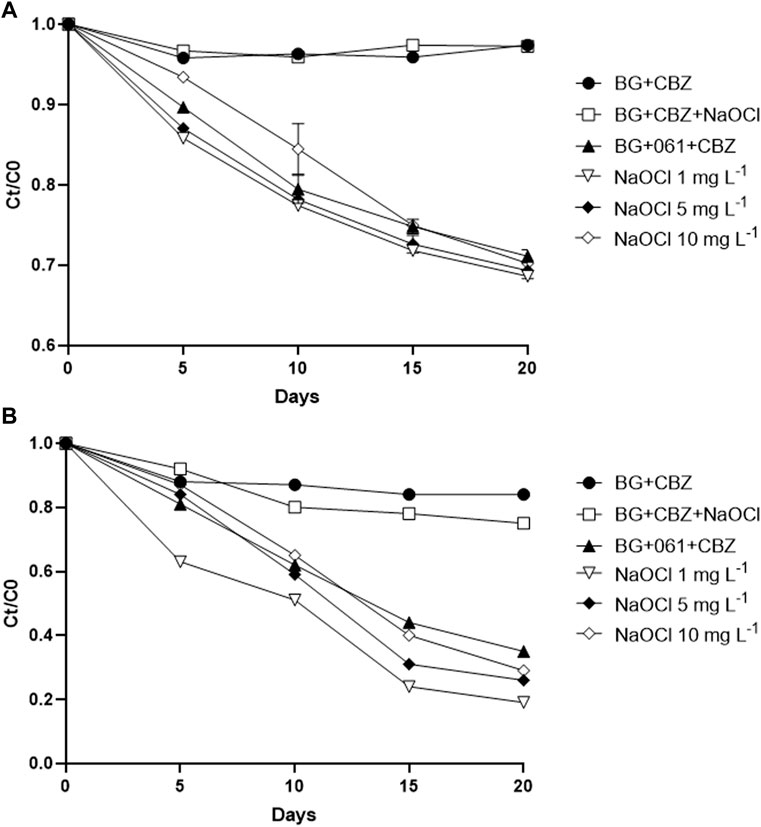
FIGURE 2. Removal of CBZ by T. obliquus KNUA061 with different concentrations of initial CBZ and NaOCl. (A) 50 μg L−1 CBZ. (B) 20 μg L−1 CBZ.
Secondary metabolites produced via the breakdown of CBZ in the microalgal cultures were also analyzed. The microalgal culture only control (without NaOCl) and 1 mg L−1 NaOCl treatment group were compared and CBZ, EP-CBZ, DI-CBZ, 9-acridine carboxaldehyde, 3-OH-CBZ, and DiOHCBZ levels were measured. While DiOHCBZ and 9-acridine carboxaldehyde were not found in either culture, approximately 4.9% of 3-OH-CBZ, 2.3%–2.6% of EP-CBZ, and 0.5% of DI-CBZ were detected in both groups (Figure 3).
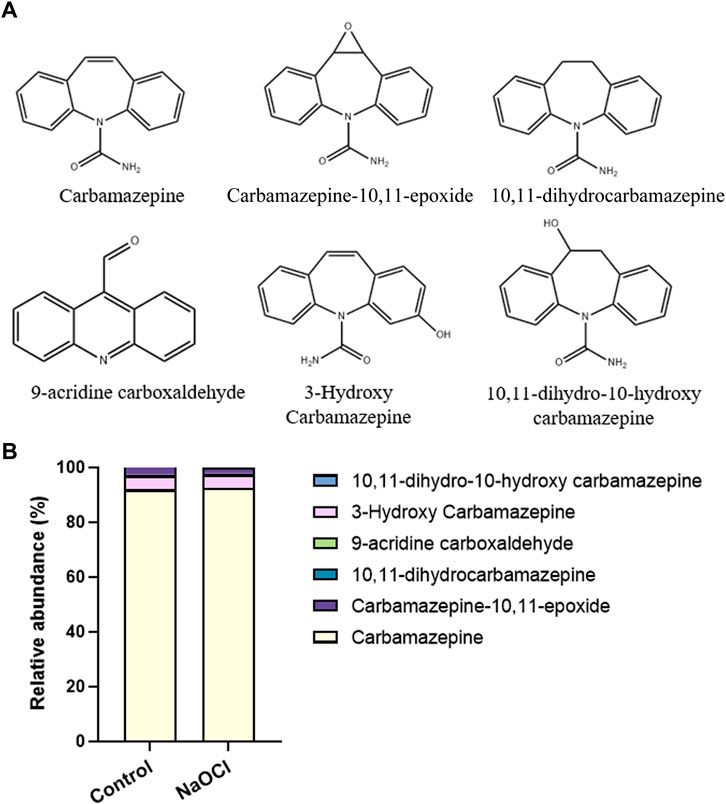
FIGURE 3. Relative abundance of CBZ and metabolites. (A) Chemical structures of CBZ and its 5 metabolites. (B) Relative abundance of CBZ and its metabolites with and without NaOCl treatment.
3.3 ROS-related redox system during CBZ removal
Changes in total ROS content related to NaOCl addition was analyzed. ROS production levels were measured in the CBZ-, NaOCl-, and CBZ + NaOCl-treated groups by using H2DCFDA fluorescence intensity which indicated the total ROS content (Figure 4A). Fluorescence-activated cell sorting (FACS) analysis results showed a decrease in total ROS content after H2DCFDA staining, even though no significant changes were detected by TMRM and DHR123 staining (Figures 4B,C).
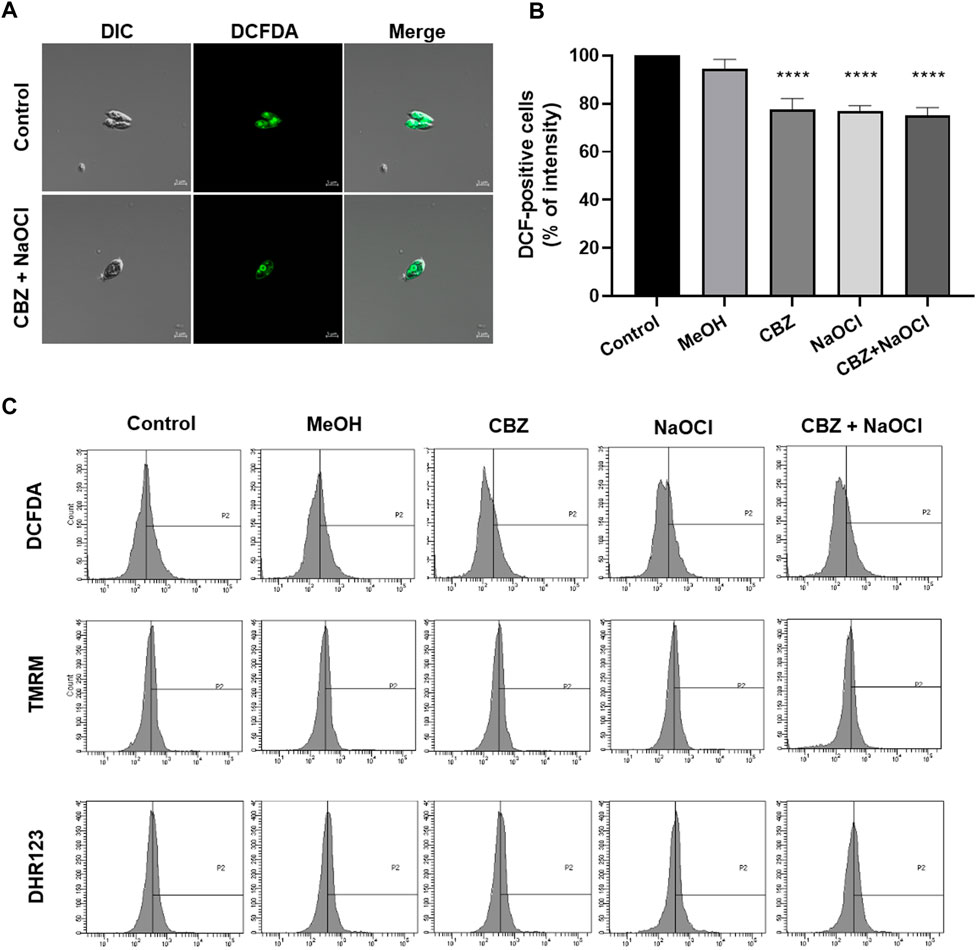
FIGURE 4. Determination of ROS content in T. obliquus KNUA061 after exposure to CBZ and NaOCl. (A) Confocal microscopy images of H2DCFDA-stained T. obliquus KNUA061; scale bars are shown in each image. (B,C) Relative intensity was measured using FACS after H2DCFDA, TMRM, and DHR123 staining. (**** p-value < 0.0001).
Factors related to enzymatic and non-enzymatic redox systems were also investigated under CBZ and CBZ + NaOCl stress conditions. Compared to the non-treated control group, SOD, CAT, and PER activities, which are known as antioxidant enzymes primarily involved in ROS removal, increased by 10%, 19%, and 1% in the CBZ treatment and by 22%, 16%, and 7% in the CBZ + NaOCl treatment, respectively (Figure 5). The amount of MDA, which indicates the degree of lipid oxidation, decreased by 14% in the CBZ treatment group and by 16% in the CBZ + NaOCl group, respectively (Figure 6A). The fluorescence intensity, verified by BODIPY 581/591 staining, also decreased by 4.0% in the CBZ group and 10.8% in the CBZ + NaOCl treatment group, respectively (Figure 6B).
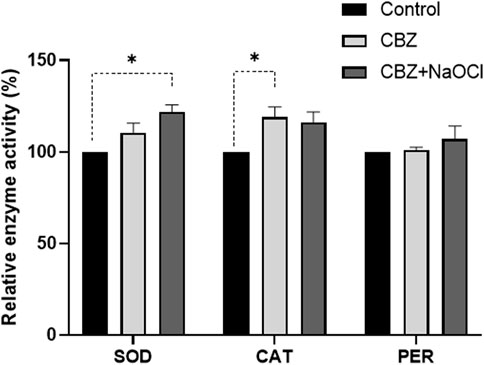
FIGURE 5. Effect of CBZ and NaOCl on SOD, CAT, and PER activities of T. obliquus KNUA061. Error bars indicate standard deviation (*p-value < 0.05, **p-value < 0.01).
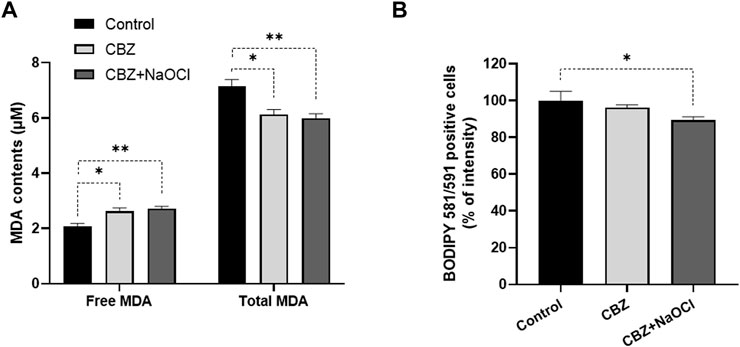
FIGURE 6. Determination of lipid peroxidation in T. obliquus KNUA061 after exposure to CBZ, and NaOCl. Free MDA and total MDA contents (A), and relative green fluorescence intensity of BODIPY 581/591 (B) in T. obliquus KNUA061 (*p-value < 0.05, **p-value < 0.01).
3.4 Characteristics of resulting microalgal biomass
All samples had more than 92.1% VM and less than 6.2% moisture content, respectively. The HHVs of all the samples calculated from the ultimate analysis results were similar to each other (approximately 23.3–23.1 MJ kg−1) as listed in Table 1.
Biochemical analysis showed that total carbohydrate and lipid mass were the highest in the CBZ + NaOCl treatment group and the total protein content was the highest in the CBZ treatment group, respectively (Table 2). Monosaccharides compositions of all the treatment groups were also almost similar to each other (Supplementary Figure S3).
It was found that the CBZ treatment induced the highest total free amino acid content. Regarding essential amino acids, arginine showed the highest content of 0.666 mg g−1 in the control, while lysine was highest at 0.288 mg g−1 in the CBZ + NaOCl treatment. For non-essential amino acids, glutamic acid was in the highest (1.217 mg g−1) in the CBZ + NaOCl treatment, whereas alanine was relatively low (0.477 mg g−1) in the control. No cysteine was detected in any sample and proline was the lowest in the CBZ + NaOCl treatment (0.019 mg g−1) as summarized in Table 3.
FAME analysis results demonstrated that palmitic acid (C16:0), oleic acid (C18:1), linoleic acid (C18:2), and α-linolenic acid (C18:3 ω 3) were the major components of in all the 3 treatment groups. The PUFA contents were almost double to the saturated fatty acid (SFA) contents in the CBZ and CBZ + NaOCl treatment groups, while the highest total FAME content (93.52%) was observed in the CBZ + NaOCl-treated microalgal lipid. However, all three samples did not demonstrate statistically significant differences (Table 4). The biodiesel quality results were calculated based on the FAME results and there were no significant differences found between the samples. It was also confirmed that the IV and CN were not within the ranges of the EN14214 standard in all samples (Table 5).
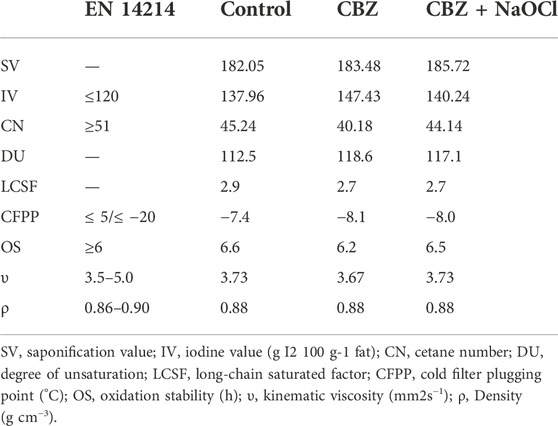
TABLE 5. Biodiesel properties of microalgal biomass calculated by fatty acid methyl ester composition.
4 Discussion
Our preliminary test of CBZ removal efficiency was conducted using 13 indigenous microalgal strains (Supplementary Table S2). Desmodesmus intermedius var. balatonicus KNUA024, which was isolated from the outdoor raceway pond at our mass cultivation facility located in Chilgok, Korea (Jo et al., 2020a), was chosen since this microalga exhibited high environmental stress resistance and excellent biomass productivity and wastewater treatment efficiency in our previous publications (Do et al., 2019; Do et al., 2021). The remaining 12 strains were isolated from the primary settled wastewater obtained from the local municipal wastewater treatment facilities in Daegu, Korea. Since various PPCPs may exist in wastewater treatment plants, they were tested as possible candidate strains as eco-friendly alternatives to wastewater treatment.
Before executing the preliminary experiments, the taxonomic positions for all the microalgal candidates were determined by both morphological and molecular characterizations. In particular, molecular identification results for strain KNUA061 by using three different marker genes such as internal transcribed spacer (ITS), 18S rRNA, and rbcL were conducted (Supplementary Table S3). In addition, the isolate’s taxonomy as was cross-validated by morphological identification and classification. Since both morphological and molecular identification results indicated that strain KNUA061 belonged to the species T. obliquus, it was named as T. obliquus strain KNUA061. It should be noted that accurate species identification is essential for ecological research as well as safe and sustainable use of microalgae (Gantar and Svircev 2008; Champenois et al., 2015; Borowitzka 2016).
The first preliminary experiments were performed at a concentration of 1 mg L−1 CBZ with 13 strains and four most promising candidates were screened. Then, the second preliminary test was carried out with these top four strains at a concentration of 250 μg L−1 CBZ. Strain KNUA061 that demonstrated the best CBZ-degrading capability was finally selected for further study (Supplementary Figure S1).
Strain KNUA061 achieved a removal efficiency of approximately 11.4% in the second screening test at a concentration of around 250 μg L−1 CBZ (Supplementary Table S7). Then, NaOCl was used as a stressor to increase the removal efficiency. NaOCl is widely accepted for disinfection in the water treatment process because chlorination is a very simple, effective, and inexpensive way of wastewater purification (Kesar and Bhatti 2022). It also acts as a strong biocide that can eliminate pathogenic microorganisms and microalgae during water treatment (Emmanuel et al., 2004). Therefore, it was determined that up to which NaOCl concentrations T. obliquus KNUA061 cells still grow normally and its CBZ degrading metabolism can be activated under stress-inducing conditions. Growth rate of the microalga and its CBZ removal efficiency were investigated at 1 mg L−1, 5 mg L−1, and 10 mg L−1 NaOCl levels.
The microalga was cultured for 20 days and it was confirmed that the 1 mg L−1 NaOCl treatment group attained a high growth rate similar to that of the control (without NaOCl) and even produced the highest chlorophylls and carotenoids than others (Figure 1D). NaOCl treatment may have aided production of chlorophylls and carotenoids in strain KNUA061. In OD680-and DW-based measurements, microalgal cells started to be affected at 1 mg L−1 NaOCl and cell growth was inhibited at 5 and 10 mg L−1 NaOCl indicating significant effect of chlorination on microalgal growth. At higher NaOCl concentrations, the cultures almost lost their chlorophylls at the early stage of cultivation, but a few cells that survived started to adapt the conditions and grew well at the later phases of cultivation as the effect of NaOCl in the culture media wore out (Figure 1A). This indicated that the concentrations of NaOCl used in this study were not sufficient for completely eliminating the microalgal cells in the liquid culture media.
CBZ was gradually degraded during the microalgal cultivation and the removal rates of either photolysis or NaOCl alone in the abiotic controls were negligible (Figure 2; Supplementary Tables S4, S5). The removal rates were higher at 1 mg L−1 NaOCl than those of the microalga alone biotic controls (Supplementary Tables S4, S5). However, the difference of the removal rates gradually decreased in the later parts of the cultivation periods. In the groups treated with 10 mg L−1 NaOCl, the initial removal rates were insignificant, but improved rates were observed after 10 days of incubation. Nevertheless, the final removal rate was less than 30.0% in the first CBZ degradation test at 50.0 μg L−1 (Supplementary Table S4). As stated above, only minute concentrations of CBZ are present in surface water, the second CBZ removal experiment was conducted at approximately 20 μg L−1. The second test results demonstrated that the removal rate in the 1 mg L−1 treatment was increased up to 82.0% (Supplementary Table S5). Considering the actual concentrations of CBZ detected in the rivers and wastewater treatment plants worldwide, higher removal efficiencies by using microalgae-based bioremediation can be expected. For instance, 1.1 μg L−1 CBZ and 13.8–145.0 ng L−1 CBZ were detected in the Han River and Nakdong River, respectively (Im et al., 2020; Seo et al., 2020).
To understand the CBZ removal process of microalgae, various methods have been used to determine the relative ratio of bioaccumulation, biosorption, and biodegradation (Hena et al., 2021). Five known secondary metabolites of CBZ including EPCBZ, DI-CBZ, 9-acridine carboxaldehyde, 3-OH-CBZ, and DiOHCBZ were prepared as standard intermediates and their compositions as breakdown products were compared in the control and 1 mg L−1 NaOCl-treated groups. Most of the remaining products after cultivation were in the original CBZ form and DiOHCBZ and 9-acridine carboxaldehyde were not detected at all. However, the compositions of 3-OH-CBZ (4.9%), DI-CBZ (0.5%), and EP-CBZ (2.3%–2.6%) were very similar to each other (Figure 3). Thus, addition of NaOCl to microalgal culture only increased the CBZ removal efficiency and there may be no significant changes occurred in the CBZ biodegradation pathways. However, it should be noted that EP-CBZ, which is one of the main metabolites of CBZ, was reported to be more toxic to Chironomus riparius (commonly known as the harlequin fly) than CBZ with an LC50 of 0.20 mg kg−1 (Heye et al., 2016), but its effects on the microalgal cells seemed neglectable due to very low concentrations. On the other hand, DI-CBZ was tested for its toxicity on zebrafish (Danio rerio) embryos, but no effect was observed (Pohl et al., 2020). To our knowledge, there is no information available on 9-acridine carboxaldehyde toxicity. It was demonstrated a higher acute toxicity of 3-OH-CBZ for Vibrio fischeri (Gram-negative marine bacterium) compared to the toxicity of CBZ (Kaiser et al., 2013; Bahlmann et al., 2014). In V. fischeri toxicity assay, DiOHCBZ exhibited almost unaltered toxicity compared to the its parental substrate (Kaiser et al., 2014). Overall, only minute amounts of harmful intermediates from CBZ were formed during the biodegradation process, but their toxic impact on the microalga is inconsequential.
NaOCl is a disinfectant which is widely used in water treatment and it was intended to activate the redox system by applying an appropriate level of NaOCl to the microalgal culture. Therefore, factors related to intracellular ROS regulation were investigated by treatment with 1 mg L−1 NaOCl, at which demonstrated a relatively high growth and the highest CBZ removal rate. Total ROS levels were reduced after CBZ, NaOCl and CBZ + NaOCl treatments (Figure 4). It was expected that the amount of total ROS would be reduced by the activation of the redox system in the microalga. DCFDA staining showed the decrease in ROS (Figures 4B,C) while, no significant difference was observed between the results from TMRM and DHR123 staining (Figure 4C). Antioxidant enzyme activities were measured to determine the factors that reduce ROS activities of SOD, CAT, and PER. These representative antioxidant enzymes were increased after the treatment with CBZ and CBZ + NaOCl (Figure 5). In particular, SOD activity was the highest in the CBZ + NaOCl treatment. These results indicated that CBZ and CBZ + NaOCl treatments somehow triggered the redox-defence system. Decreased MDA content and BODIPY 581/591 fluorescence intensity also showed that the CBZ and CBZ + NaOCl treatments decreased lipid peroxidation and also had some effect on ROS reduction (Figure 6). In conclusion, CBZ and 1 mg L−1 NaOCl NaOCl addition may have induced stress conditions that activate the ROS reducing mechanisms in the microalga without suppressing cell growth.
Amino acids are involved in a significant position of major metabolic processes. Non-essential amino acids are the naturally occurring ones and they do not need to be provided by an outside source since the human body can create them on its own. However, essential amino acids cannot be produced by the body, so they must be supplied from dietary proteins. Cysteine, glutamine, proline, and hydroxyproline are currently considered as conditionally essential amino acids that are non-essential amino acids that become essential under certain circumstances such as illness or pregnancy. Broadly speaking, microalgae have relatively high arginine and leucine contents and low methionine and histidine contents (Kolmakova and Kolmakov, 2019). Therefore, it can be inferred that an increase in lysine will result in advantageous fatty acid metabolism via the production of carnitine under stress conditions. Glutamic acid was the highest among all the free amino acids as shown in Table 3. Proline, which is known to function as a metal chelator, substance for antioxidant defense, and signal transmitter under stress conditions, was not increased along with stress treatment. Previous literatures (Hayat et al., 2012; Liang et al., 2013; Ben Rejeb et al., 2014; Meena et al., 2019) indicated that a stressful environment usually resulted in an overproduction of proline in cells in order to maintain osmotic balance, stabilize membranes, and keep ROS concentration within normal ranges. However, decrease in proline level in the CBZ + NaOCl group was observed suggesting that proline metabolism may not be involved in the antioxidant systems of T. obliquus KNUA061. From our results, it is unclear whether the free amino acid regulation was involved in the redox mechanisms of the microalga to cope with CBZ and/or NaOCl stress conditions. Nevertheless, the high essential amino acid contents would enhance the value of microalgal biomass towards an alternative feedstock for nutrient dietary supplements.
IV is defined as the amount of iodine in a specific biodiesel and it is related to the number of double bonds that affect the DU, OS, and cold flow of biodiesel (Islam et al., 2013.; Cho et al., 2019). CN is also an important parameter that reflects engine performance, the generation of nitrous oxide, and the combustion of biodiesel (Islam et al., 2013.; Cho et al., 2019). Although the biodiesel properties of strain KNUA061 showed slightly higher values of IV (138–147) and lower values of CN (40–45) than the standard values (IV: ≤120; CN: ≥47), it is still expected that the biodiesel produced from T. obliquus KNUA061 could be used as a blending resource for transportation fuels since biodiesel blends with petrodiesel have been widely used as automotive fuels worldwide (Kousoulidou et al., 2010; Salvi and Panwar 2012; Du et al., 2018). However, further studies are needed to promote biodiesel properties by changing the cultivation conditions and other biotechnological techniques.
The ultimate analysis results showed that the HHVs (22.3–23.1) of strain KNUA061 biomass were higher than those of the terrestrial energy crops (17.0–20.0 MJ kg−1) (Ross et al., 2008). Since fine particulate matters have become a national concern in Korea, a couple of coal-burning power stations have already converted to biomass-burning stations and many old coal-powdered plants are considering this move in the near future (Yang 2016; Um and Kang 2019). Hence, microalgal pellet made of mass-cultivated microalgae biomass could be an excellent mixed combustion biofuel for these coal power stations.
Many researches on microalgae-mediated bioremediation of pharmaceuticals have recently been reported (Peng et al., 2014; Matamoros et al., 2016b; Escapa et al., 2017; Xiong et al., 2017; Xiong et al., 2018). Also, a number of studies (Supplementary Table S8) have demonstrated biotransformation of CBZ by using a variety of microalgal species, but their removal rates were relatively lower than those by the advanced oxidation treatment methods (Supplementary Table S1). However, microalgal bioremediation of CBZ has gained growing scientific interest due to its many advantages such as carbon fixation via photosynthesis and low operational costs, and acclimatization of nitrogen and phosphorus into algal biomass over other means (Cai et al., 2013; Gonçalves et al., 2017; Xiong et al., 2018; Koul et al., 2022). Furthermore, this process is considered economically and environmentally sustainable because the resulting microalgal biomass after remediation can be reutilized as nutraceuticals, cosmetics, fertilizers, aquaculture and animal feeds, and biofuels.
Recent works by Özengin and Elmaci (2016), Chen et al. (2018), Casierra-Martinez et al. (2020), and Xie et al. (2022) demonstrated higher CBZ removal efficiencies in constructed wetlands (CWs) than those in conventional wastewater plants. Therefore, it is hoped that integration of CWs with microalgal culturing ponds would facilitate more efficient and environmentally friendly remediation of CBZ in the future.
5 Concluding remarks
This study demonstrated the possibility of biological removal of CBZ by using an indigenous microalga, T. obliquus KNUA061. It was determined that efficiency of the microalgal biodegradation process could be accelerated by applying an appropriate concentration of NaOCl without inhibiting cell growth. This increase may account for the redox-defence system in microalgal cells that is activated to remove ROS generated by NaOCl. Increased levels of antioxidant enzymes and lipid oxidation were also observed when compared to those of the control. Thus, T. obliquus KNUA061 could be a promising candidate for microalgae-mediated bioremediation of CBZ. In addition, it was also confirmed that the resulting biomass was rich in essential amino acids and PUFAs and its HHV is higher than terrestrial energy crops. Therefore, the biomass has a great potential as biodiesel blend and mixed combustion biofuel as well as dietary supplement. Ellis, 2008, Loos et al., 2018.
Data availability statement
The original contributions presented in the study are included in the article/Supplementary Material, further inquiries can be directed to the corresponding authors.
Author contributions
J-MD was involved in the conceptualization, laboratory work, data analysis, drafting, and editing of the manuscript; H-TY was involved in experimental design and data curation; JWH was involved in editing of the manuscript; G-SD was involved in laboratory work; H-SY was involved in the conceptualization and editing of the manuscript.
Funding
This research was supported by Basic Science Research Programs through the National Research Foundation of Korea (NRF) funded by the Ministry of Education (Grant Numbers 2021R1I1A205551712-1-2 and 2022R1F1A1063328). This work was also supported by the National Research Facilities and Equipment Center (NFEC) grant financed by the Ministry of Education, Korea (2019R1A6C1010001). This research was part of a project titled “Efficacy/standardization technology development of marine healing resources and its life cycle safety” funded by the Ministry of Oceans and Fisheries, Korea (Grant Number 20220027).
Conflict of interest
The authors declare that the research was conducted in the absence of any commercial or financial relationships that could be construed as a potential conflict of interest.
Publisher’s note
All claims expressed in this article are solely those of the authors and do not necessarily represent those of their affiliated organizations, or those of the publisher, the editors and the reviewers. Any product that may be evaluated in this article, or claim that may be made by its manufacturer, is not guaranteed or endorsed by the publisher.
Supplementary material
The Supplementary Material for this article can be found online at: https://www.frontiersin.org/articles/10.3389/fenrg.2022.1004613/full#supplementary-material
References
Abdelfattah, A., Ali, S. S., Ramadan, H., El-Aswar, E. I., Eltawab, R., Ho, S. H., et al. (2023). Microalgae-based wastewater treatment: Mechanisms, challenges, recent advances, and future prospects. Environ. Sci. Ecotechnol. 13, 100205. doi:10.1016/j.ese.2022.100205
Al-Baldawi, I. A., Mohammed, A. A., Mutar, Z. H., Abdullah, S. R. S., Jasim, S. S., Almansoory, A. F., et al. (2021). Application of phytotechnology in alleviating pharmaceuticals and personal care products (PPCPs) in wastewater: Source, impacts, treatment, mechanisms, fate, and SWOT analysis. J. Clean. Prod. 319, 128584. doi:10.1016/j.jclepro.2021.128584
Alaoui, K. S., Tychon, B., Joachim, S., Geffard, A., Nott, K., Ronkart, S., et al. (2021). Toxic effects of a mixture of five pharmaceutical drugs assessed using Fontinalis antipyretica Hedw. Ecotoxicol. Environ. Saf. 225, 112727. doi:10.1016/j.ecoenv.2021.112727
Alharbi, S. K., Kang, J., Nghiem, L. D., van de Merwe, J. P., Leusch, F. D. L., and Price, W. E. (2017). Photolysis and UV/H2O2 of diclofenac, sulfamethoxazole, carbamazepine, and trimethoprim: Identification of their major degradation products by ESI–LC–MS and assessment of the toxicity of reaction mixtures. Process Saf. Environ. Prot. 112, 222–234. Part B. doi:10.1016/j.psep.2017.07.015
Andreozzi, R., Campanella, L., Fraysse, B., Garric, J., Gonnella, A., Giudice, R. L., et al. (2004). Effects of advanced oxidation processes (AOPs) on the toxicity of a mixture of pharmaceuticals. Water Sci. Technol. 50, 23–28. doi:10.2166/wst.2004.0304
Ash, R. J., Mauck, B., and Morgan, M. (2002). Antibiotic resistance of gram-negative bacteria in rivers, United States. Emerg. Infect. Dis. 8, 713–716. doi:10.3201/eid0807.010264
Astuya, A., Rivera, A., Vega-Drake, K., Aburto, C., Cruzat, F., Ulloa, V., et al. (2018). Study of the ichthyotoxic microalga Heterosigma akashiwo by transcriptional activation of sublethal marker Hsp70b in Transwell co-culture assays. PLoS One 13, e0201438. doi:10.1371/journal.pone.0201438
Baena-Nogueras, R. M., González-Mazo, E., and Lara-Martín, P. A. (2017). Degradation kinetics of pharmaceuticals and personal care products in surface waters: Photolysis vs biodegradation. Sci. Total Environ. 590-591, 643–654. doi:10.1016/j.scitotenv.2017.03.015
Bahlmann, A., Brack, W., Schneider, R. J., and Krauss, M. (2014). Carbamazepine and its metabolites in wastewater: Analytical pitfalls and occurrence in Germany and Portugal. Water Res. 57, 104–114. doi:10.1016/j.watres.2014.03.022
Bai, X., and Acharya, K. (2017). Algae-mediated removal of selected pharmaceutical and personal care products (PPCPs) from Lake Mead water. Sci. Total Environ. 581–582, 734–740. doi:10.1016/j.scitotenv.2016.12.192
Ben Rejeb, K., Abdelly, C., and Savouré, A. (2014). How reactive oxygen species and proline face stress together. Plant Physiol. biochem. 80, 278–284. doi:10.1016/j.plaphy.2014.04.007
Bonnot, K., Benoit, P., Mamy, L., and Patureau, D. (2022). Transformation of PPCPs in the environment: Review of knowledge and classification of pathways according to parent molecule structures. Crit. Rev. Environ. Sci. Technol. 53, 47–69. doi:10.1080/10643389.2022.2045159
Borowitzka, M. (2016). “Systematics, taxonomy and species names: Do they matter?” in The physiology of microalgae; developments in applied phycology. Editors M. A. Borowitzka, J. A. Beardall, and Raven Dordrecht, Netherlands: Springer International Publishing, 655–681.
Boxall, A. B., Rudd, M. A., Brooks, B. W., Caldwell, D. J., Choi, K., Hickmann, S., et al. (2012). Pharmaceuticals and personal care products in the environment: What are the big questions? Environ. Health Perspect. 120, 1221–1229. doi:10.1289/ehp.1104477
Breuer, G., Evers, W. A. C., de Vree, J. H., Kleinegris, D. M. M., Martens, D. E., Wijffels, R. H., et al. (2013). Analysis of fatty acid content and composition in microalgae. J. Vis. Exp. 80, e50628. doi:10.3791/50628
Cai, T., Park, S. Y., and Li, Y. (2013). Nutrient recovery from wastewater streams by microalgae: Status and prospects. Renew. Sustain. Energy Rev. 19, 360–369. doi:10.1016/j.rser.2012.11.030
Caliman, F. A., and Gavrilescu, M. (2009). Pharmaceuticals, personal care products and endocrine disrupting agents in the environment – A review. Clean. Soil Air Water 37, 277–303. doi:10.1002/clen.200900038
Calza, P., Medana, C., Padovano, E., Giancotti, V., and Baiocchi, C. (2012). Identification of the unknown transformation products derived from clarithromycin and carbamazepine using liquid chromatography/high-resolution mass spectrometry. Rapid Commun. Mass Spectrom. 26, 1687–1704. doi:10.1002/rcm.6279
Carabin, A., Drogui, P., and Robert, D. (2016). Photocatalytic oxidation of carbamazepine: Application of an experimental design methodology. Water Air Soil Pollut. 227, 122. doi:10.1007/s11270-016-2819-x
Casierra-Martinez, H. A., Madera-Parra, C. A., Vargas-Ramírez, X. M., Caselles-Osorio, A., and Torres-López, W. A. (2020). Diclofenac and carbamazepine removal from domestic wastewater using a constructed wetland-solar photo-Fenton coupled system. Ecol. Eng. 153, 105699. doi:10.1016/j.ecoleng.2019.105699
Champenois, J., Marfaing, H., and Pierre, R. (2015). Review of the taxonomic revision of Chlorella and consequences for its food uses in Europe. J. Appl. Phycol. 27, 1845–1851. doi:10.1007/s10811-014-0431-2
Chee-Sanford, J. C., Aminov, R. I., Krapac, I. J., Garrigues-Jeanjean, N., and Mackie, R. I. (2001). Occurrence and diversity of tetracycline resistance genes in lagoons and groundwater underlying two swine production facilities. Appl. Environ. Microbiol. 67, 1494–1502. doi:10.1128/AEM.67.4.1494-1502.2001
Chen, X., Hu, Z., Zhang, Y., Zhuang, L., Zhang, J., Li, J., et al. (2018). Removal processes of carbamazepine in constructed wetlands treating secondary effluent: A review. Water 10, 1351. doi:10.3390/w10101351
Chen, Y. H., Huang, Y. H., Wen, C. C., Wang, Y. H., Chen, W. L., Chen, L. C., et al. (2008). Movement disorder and neuromuscular change in zebrafish embryos after exposure to caffeine. Neurotoxicol. Teratol. 30, 440–447. doi:10.1016/j.ntt.2008.04.003
Cho, K., Heo, J., Cho, D. H., Tran, Q. G., Yun, J. H., Lee, S. M., et al. (2019). Enhancing algal biomass and lipid production by phycospheric bacterial volatiles and possible growth enhancing factor. Algal Res. 37, 186–194. doi:10.1016/j.algal.2018.11.011
Chong, M. N., Jin, B., Laera, G., and Saint, C. P. (2011). Evaluating the photodegradation of carbamazepine in a sequential batch photoreactor system: Impacts of effluent organic matter and inorganic ions. Chem. Eng. J. 174, 595–602. doi:10.1016/j.cej.2011.09.065
Chong, M. N., and Jin, B. (2012). Photocatalytic treatment of high concentration carbamazepine in synthetic hospital wastewater. J. Hazard. Mat. 199-200, 135–142. doi:10.1016/j.jhazmat.2011.10.067
Costanzo, S. D., Murby, J., and Bates, J. (2005). Ecosystem response to antibiotics entering the aquatic environment. Mar. Pollut. Bull. 51, 218–223. doi:10.1016/j.marpolbul.2004.10.038
Daneshvar, E., Antikainen, L., Koutra, E., Kornaros, M., and Bhatnagar, A. (2018). Investigation on the feasibility of Chlorella vulgaris cultivation in a mixture of pulp and aquaculture effluents: Treatment of wastewater and lipid extraction. Bioresour. Technol. 255, 104–110. doi:10.1016/j.biortech.2018.01.101
Dao, K. C., Yang, C. C., Chen, K. F., and Tsai, Y. P. (2022). Effect of operational parameters on the removal of carbamazepine and nutrients in a submerged ceramic membrane bioreactor. Membranes 12, 420. doi:10.3390/membranes12040420
de Wilt, A., Butkovskyi, A., Tuantet, K., Leal, L. H., Fernandes, T. V., Langenhoff, A., et al. (2016). Micropollutant removal in an algal treatment system fed with source separated wastewater streams. J. Hazard. Mat. 304, 84–92. doi:10.1016/j.jhazmat.2015.10.033
Delorenzo, M. E., and Fleming, J. (2008). Individual and mixture effects of selected pharmaceuticals and personal care products on the marine phytoplankton species Dunaliella tertiolecta. Arch. Environ. Contam. Toxicol. 54, 203–210. doi:10.1007/s00244-007-9032-2
Do, J. M., Jo, S. W., Kim, I. S., Na, H., Lee, J. H., Kim, H. S., et al. (2019). A feasibility study of wastewater treatment using domestic microalgae and analysis of biomass for potential applications. Water 11, 2294. doi:10.3390/w11112294
Do, J. M., Jo, S. W., Yeo, H. T., Shin, D. H., Oh, H., Hong, J. W., et al. (2021). Biological treatment of reverse osmosis concentrate by microalgae cultivation and utilization of the resulting algal biomass. J. Water Process Eng. 42, 102157. doi:10.1016/j.jwpe.2021.102157
Doll, T. E., and Frimmel, F. H. (2005). Removal of selected persistent organic pollutants by heterogeneous photocatalysis in water. Catal. Today 101, 195–202. doi:10.1016/j.cattod.2005.03.005
Domínguez, J. R., González, T., Palo, P., and Sánchez-Martín, J. (2010). Electrochemical advanced oxidation of carbamazepine on boron-doped diamond anodes. Influence of operating variables. Ind. Eng. Chem. Res. 49, 8353–8359. doi:10.1021/ie101023u
Domínguez-Rebolledo, Á. E., Martínez-Pastor, F., Fernández-Santos, M. R., Del Olmo, E., Bisbal, A., Ros-Santaella, J. L., et al. (2010). Comparison of the TBARS assay and BODIPY C11 probes for assessing lipid peroxidation in red deer spermatozoa. Reprod. Domest. Anim. 45, e360–e368. doi:10.1111/j.1439-0531.2009.01578.x
Du, H., Huque, Z., and Kommalapati, R. R. (2018). Impacts of biodiesel applied to the transportation fleets in the Greater Houston Area. J. Renew. Energy 2018, 1–9. doi:10.1155/2018/7350715
Ebele, A. J., Abdallah, M. A. E., and Harrad, S. (2017). Pharmaceuticals and personal care products (PPCPs) in the freshwater aquatic environment. Emerg. Contam. 3, 1–16. doi:10.1016/j.emcon.2016.12.004
Ellis, J. B. (2008). Assessing sources and impacts of priority PPCP compounds in urban receiving waters. Edinburgh, Scotland, UK: Proceedings of the 11th International Conference on Urban Drainage.
Emmanuel, E., Keck, G., Blanchard, J. M., Vermande, P., and Perrodin, Y. (2004). Toxicological effects of disinfections using sodium hypochlorite on aquatic organisms and its contribution to AOX formation in hospital wastewater. Environ. Int. 30, 891–900. doi:10.1016/j.envint.2004.02.004
Escapa, C., Coimbra, R. N., Paniagua, S., García, A. I., and Otero, M. (2017). Paracetamol and salicylic acid removal from contaminated water by microalgae. J. Environ. Manage. 203, 799–806. doi:10.1016/j.jenvman.2016.06.051
Esquerdo, A. A., Galvañ, P. J. V., Gadea, I. S., and Rico, D. P. (2021). Carbamazepine and diclofenac removal double treatment: Oxidation and adsorption. Int. J. Environ. Res. Public Health 18, 7163. doi:10.3390/ijerph18137163
Francisco, É. C., Neves, D. B., Jacob-Lopes, E., and Franco, T. T. (2010). Microalgae as feedstock for biodiesel production: Carbon dioxide sequestration, lipid production and biofuel quality. J. Chem. Technol. Biotechnol. 85, 395–403. doi:10.1002/jctb.2338
Gantar, M., and Svircev, Z. (2008). Microalgae and cyanobacteria: Food for thought. J. Phycol. 44, 260–268. doi:10.1111/j.1529-8817.2008.00469.x
Gebhardt, W., and Schroder, H. F. (2007). Liquid chromatography–tandem mass spectrometry for the follow up of the elimination of persistent pharmaceuticals during wastewater treatment applying biological wastewater treatment and advanced oxidation. J. Chromatogr. A 1160, 34–43. doi:10.1016/j.chroma.2007.05.075
Given, P. H., Weldon, D., and Zoeller, J. H. (1986). Calculation of calorific values of coals from ultimate analyses: Theoretical basis and geochemical implications. Fuel 65, 849–854. doi:10.1016/0016-2361(86)90080-3
Gonçalves, A. L., Pires, J. C. M., and Simões, M. (2017). A review on the use of microalgal consortia for wastewater treatment. Algal Res. 24, 403–415. doi:10.1016/j.algal.2016.11.008
Gong, C., Zhai, J., Wang, X., Zhu, W., Yang, D., Luo, Y., et al. (2022). Synergistic improving photo-Fenton and photo-catalytic degradation of carbamazepine over FeS2/Fe2O3/organic acid with H2O2in-situ generation. Chemosphere 307, 136199. doi:10.1016/j.chemosphere.2022.136199
Guo, Q., Zhu, W., Yang, D., Wang, X., Li, Y., Gong, C., et al. (2021). A green solar photo-Fenton process for the degradation of carbamazepine using natural pyrite and organic acid with in-situ generated H2O2. Sci. Total Environ. 784, 147187. doi:10.1016/j.scitotenv.2021.147187
Hassaan, M. A., El Nemr, A., and Hassaan, A. (2017). Health and environmental impacts of dyes: Mini review. Am. J. Environ. Sci. Eng. 1, 64–67. doi:10.11648/j.ajese.20170103.11
Hayat, S., Hayat, Q., Alyemeni, M. N., Wani, A. S., Pichtel, J., and Ahmad, A. (2012). Role of proline under changing environments: A review. Plant Signal. Behav. 7, 1456–1466. doi:10.4161/psb.21949
Hena, S., Gutierrez, L., and Croué, J. P. (2021). Removal of pharmaceutical and personal care products (PPCPs) from wastewater using microalgae: A review. J. Hazard. Mat. 403, 124041. doi:10.1016/j.jhazmat.2020.124041
Heye, K., Becker, D., Lütke Eversloh, C., Durmaz, V., Ternes, T. A., Oetken, M., et al. (2016). Effects of carbamazepine and two of its metabolites on the non-biting midge Chironomus riparius in a sediment full life cycle toxicity test. Water Res. 98, 19–27. doi:10.1016/j.watres.2016.03.071
Hoppe, P. D., Rosi-Marshall, E. J., and Bechtold, H. A. (2012). The antihistamine cimetidine alters invertebrate growth and population dynamics in artificial streams. Freshw. Sci. 31, 379–388. doi:10.1899/11-089
Huang, J., Ding, J., Jiang, H., Wang, Z., Zheng, L., Song, X., et al. (2022). Pharmaceuticals and personal care products across different water bodies in Taihu Lake basin, China: Occurrence, source, and flux. Int. J. Environ. Res. Public Health 19, 11135. 10.3390/ijerph191711135.
Humphrey, I., Chendo, M. A. C., Njah, A. N., and Nwankwo, D. I. (2021). Optimization of microalgae growth for biofuel production using a new empirical dynamic model. Biofuels 12, 1209–1224. doi:10.1080/17597269.2019.1608012
Im, J. K., Cho, I. H., Kim, S. K., and Zoh, K. D. (2012). Optimization of carbamazepine removal in O3/UV/H2O2 system using a response surface methodology with central composite design. Desalination 285, 306–314. doi:10.1016/j.desal.2011.10.018
Im, J. K., Hwang, M. Y., Lee, E. H., Noh, H. R., and Yu, S. J. (2020). Pharmaceutical compounds in tributaries of the Han River watershed, South Korea. Environ. Res. 188, 109758. doi:10.1016/j.envres.2020.109758
Islam, M. A., Magnusson, M., Brown, R. J., Ayoko, G. A., Nabi, M. N., and Heimann, K. (2013). Microalgal species selection for biodiesel production based on fuel properties derived from fatty acid profiles. Energies 6, 5676–5702. doi:10.3390/en6115676
Jelic, A., Cruz-Morató, C., Marco-Urrea, E., Sarrà, M., Perez, S., Vicent, T., et al. (2012). Degradation of carbamazepine by Trametes versicolor in an air pulsed fluidized bed bioreactor and identification of intermediates. Water Res. 46, 955–964. doi:10.1016/j.watres.2011.11.063
Jeyakumar, B., Asha, D., Varalakshmi, P., and Kathiresan, S. (2020). Nitrogen repletion favors cellular metabolism and improves eicosapentaenoic acid production in the marine microalga Isochrysis sp. CASA CC 101. Algal Res. 47, 101877. doi:10.1016/j.algal.2020.101877
Jo, S. W., Do, J. M., Na, H., Hong, J. W., Kim, I. S., and Yoon, H. S. (2020a). Assessment of biomass potentials of microalgal communities in open pond raceways using mass cultivation. PeerJ 8, e9418. doi:10.7717/peerj.9418
Jo, S. W., Kang, N. S., Lee, J. A., Kim, E. S., Kim, K. M., and Yoon, M. (2020b). Characterization of MABIK microalgae with biotechnological potentials. J. Mar. Biosci. Biotechnol. 12, 40–49. doi:10.15433/ksmb.2020.12.1.040
Kaiser, E., Prasse, C., Bröder, K., and Ternes, T. (2013). Transformation of three human metabolites of carbamazepine during sand filtration. Glasgow: SETAC Europe 23rd Annual Meeting.
Kaiser, E., Prasse, C., Wagner, M., Bröder, K., and Ternes, T. A. (2014). Transformation of oxcarbazepine and human metabolites of carbamazepine and oxcarbazepine in wastewater treatment and sand filters. Environ. Sci. Technol. 48, 10208–10216. doi:10.1021/es5024493
Kang, S. I., Kang, S. Y., and Hur, H. G. (2008). Identification of fungal metabolites of anticonvulsant drug carbamazepine. Appl. Microbiol. Biotechnol. 79, 663–669. doi:10.1007/s00253-008-1459-5
Keen, O. S., Baik, S., Linden, K. G., Aga, D. S., and Love, N. G. (2012). Enhanced biodegradation of carbamazepine after UV/H2O2 advanced oxidation. Environ. Sci. Technol. 46, 6222–6227. doi:10.1021/es300897u
Kesar, S., and Bhatti, M. S. (2022). Chlorination of secondary treated wastewater with sodium hypochlorite (NaOCl): An effective single alternate to other disinfectants. Heliyon 2022, e11162. doi:10.1016/j.heliyon.2022.e11162
Kim, I., and Tanaka, H. (2009). Photodegradation characteristics of PPCPs in water with UV treatment. Environ. Int. 35, 793–802. doi:10.1016/j.envint.2009.01.003
Klamerth, N., Malato, S., Maldonado, M. I., Aguera, A., and Fernandez-Alba, A. R. (2010). Application of photo-Fenton as a tertiary treatment of emerging contaminants in municipal wastewater. Environ. Sci. Technol. 44, 1792–1798. doi:10.1021/es903455p
Kolmakova, A. A., and Kolmakov, V. I. (2019). Amino acid composition of green microalgae and diatoms, cyanobacteria, and zooplankton (review). Inland Water Biol. 12, 452–461. doi:10.1134/S1995082919040060
König, A., Weidauer, C., Seiwert, B., Reemtsma, T., Unger, T., and Jekel, M. (2016). Reductive transformation of carbamazepine by abiotic and biotic processes. Water Res. 101, 272–280. doi:10.1016/j.watres.2016.05.084
Kosma, C. I., Lambropoulou, D. A., and Albanis, T. A. (2014). Investigation of PPCPs in wastewater treatment plants in Greece: Occurrence, removal and environmental risk assessment. Sci. Total Environ. 466–467, 421–438. doi:10.1016/j.scitotenv.2013.07.044
Koul, B., Sharma, K., and Shah, M. P. (2022). Phycoremediation: A sustainable alternative in wastewater treatment (WWT) regime. Environ. Technol. Innov. 25, 102040. doi:10.1016/j.eti.2021.102040
Kousoulidou, M., Fontaras, G., Ntziachristos, L., and Samaras, Z. (2010). Biodiesel blend effects on common-rail diesel combustion and emissions. Fuel 89, 3442–3449. doi:10.1016/j.fuel.2010.06.034
Laera, G., Chong, M. N., Jin, B., and Lopez, A. (2011a). An integrated MBR-TiO2 photocatalysis process for the removal of carbamazepine from simulated pharmaceutical industrial effluent. Bioresour. Technol. 102, 7012–7015. doi:10.1016/j.biortech.2011.04.056
Laera, G., Jin, B., Zhu, H., and Lopez, A. (2011b). Photocatalytic activity of TiO2 nanofibers in simulated and real municipal effluents. Catal. Today 161, 147–152. doi:10.1016/j.cattod.2010.10.037
Lee, Y., and Gunten, U. V. (2010). Oxidative transformation of micropollutants during municipal wastewater treatment: Comparison of kinetic aspects of selective (chlorine, chlorine dioxide, ferrateVI, and ozone) and non-selective oxidants (hydroxyl radical). Water Res. 44, 555–566. doi:10.1016/j.watres.2009.11.045
Lei, H., and Snyder, S. A. (2007). 3D QSPR models for the removal of trace organic contaminants by ozone and free chlorine. Water Res. 41, 4051–4060. doi:10.1016/j.watres.2007.05.010
Levasseur, M., Thompson, P. A., and Harrison, P. J. (1993). Physiological acclimation of marine phytoplankton to different nitrogen sources. J. Phycol. 29, 587–595. doi:10.1111/j.0022-3646.1993.00587.x
Li, W., Nanaboina, V., Zhou, Q., and Korshin, G. V. (2012). Effects of Fenton treatment on the properties of effluent organic matter and their relationships with the degradation of pharmaceuticals and personal care products. Water Res. 46, 403–412. doi:10.1016/j.watres.2011.11.002
Liang, L., Bai, X., and Hua, Z. (2022). Enhancement of the immobilization on microalgae protective effects and carbamazepine removal by Chlorella vulgaris. Environ. Sci. Pollut. Res. 29, 79567–79578. doi:10.1007/s11356-022-21418-0
Liang, X., Zhang, L., Natarajan, S. K., and Becker, D. F. (2013). Proline mechanisms of stress survival. Antioxid. Redox Signal. 19, 998–1011. doi:10.1089/ars.2012.5074
Liu, N., Zheng, M., Sijak, S., Tang, L., Xu, G., and Wu, M. (2015). Aquatic photolysis of carbamazepine by UV/H2O2 and UV/Fe(II) processes. Res. Chem. Intermed. 41, 7015–7028. doi:10.1007/s11164-014-1795-2
Loos, R., Marinov, D., Sanseverino, I., Napierska, D., and Lettieri, T. (2018). Review of the 1st watch list under the water framework directive and recommendations for the 2nd watch list. Luxembourg: EUR 29173 EN, Publications Office of the European Union. 10.2760/614367, JRC111198.
Ma, L., Liu, Y., Yang, Q., Jiang, L., and Li, G. (2022). Occurrence and distribution of pharmaceuticals and personal care products (PPCPs) in wastewater related riverbank groundwater. Sci. Total Environ. 821, 153372. doi:10.1016/j.scitotenv.2022.153372
Maldonado-Torres, S., Gurung, R., Rijal, H., Chan, A., Acharya, S., Rogelj, S., et al. (2018). Fate, transformation, and toxicological impacts of pharmaceutical and personal care products in surface waters. Environ. Health Insights 12, 117863021879583. doi:10.1177/1178630218795836
Mariotti, F., Tomé, D., and Mirand, P. P. (2008). Converting nitrogen into protein - beyond 6.25 and Jones’ factors. Crit. Rev. Food Sci. Nutr. 48, 177–184. doi:10.1080/10408390701279749
Matamoros, V., Gutiérrez, R., Ferrer, I., García, J., and Bayona, J. M. (2016a). Capability of microalgae-based wastewater treatment systems to remove emerging organic contaminants: A pilot-scale study. J. Hazard. Mat. 288, 34–42. doi:10.1016/j.jhazmat.2015.02.002
Matamoros, V., Uggetti, E., García, J., and Bayona, J. M. (2016b). Assessment of the mechanisms involved in the removal of emerging contaminants by microalgae from wastewater: A laboratory scale study. J. Hazard. Mat. 301, 197–205. doi:10.1016/j.jhazmat.2015.08.050
McDowell, D. C., Huber, M. M., Wagner, M., Von Gunten, U., and Ternes, T. A. (2005). Ozonation of carbamazepine in drinking water: Identification and kinetic study of major oxidation products. Environ. Sci. Technol. 39, 8014–8022. doi:10.1021/es050043l
Mecozzi, M. (2005). Estimation of total carbohydrate amount in environmental samples by the phenol-sulphuric acid method assisted by multivariate calibration. Chemom. Intell. Lab. Syst. 79, 84–90. doi:10.1016/j.chemolab.2005.04.005
Meena, M., Divyanshu, K., Kumar, S., Swapnil, P., Zehra, A., Shukla, V., et al. (2019). Regulation of L-proline biosynthesis, signal transduction, transport, accumulation and its vital role in plants during variable environmental conditions. Heliyon 5, e02952. doi:10.1016/j.heliyon.2019.e02952
Miranda-Garcia, N., Suarez, S., Sanchez, B., Coronado, J. M., Malato, S., and Maldonado, M. I. (2011). Photocatalytic degradation of emerging contaminants in municipal wastewater treatment plant effluents using immobilized TiO2 in a solar pilot plant. Appl. Catal. B Environ. 103, 294–301. doi:10.1016/j.apcatb.2011.01.030
Mishra, S. K., Suh, W. I., Farooq, W., Moon, M., Shrivastav, A., Park, M. S., et al. (2014). Rapid quantification of microalgal lipids in aqueous medium by a simple colorimetric method. Bioresour. Technol. 155, 330–333. doi:10.1016/j.biortech.2013.12.077
Mohapatra, D. P., Brar, S. K., Tyagi, R. D., Picard, P., and Surampalli, R. Y. (2013). A comparative study of ultrasonication, Fenton’s oxidation and ferro-sonication treatment for degradation of carbamazepine from wastewater and toxicity test by yeast estrogen screen (YES) assay. Sci. Total Environ. 447, 280–285. doi:10.1016/j.scitotenv.2012.12.072
Mohapatra, D. P., Brar, S. K., Tyagi, R. D., Picard, P., and Surampalli, R. Y. (2014). Analysis and advanced oxidation treatment of a persistent pharmaceutical compound in wastewater and wastewater sludge-carbamazepine. Sci. Total Environ. 470–471, 58–75. doi:10.1016/j.scitotenv.2013.09.034
Mojiri, A., Baharlooeian, M., Kazeroon, R. A., Farraji, H., and Lou, Z. (2021). Removal of pharmaceutical micropollutants with integrated biochar and marine microalgae. Microorganisms 9, 4–20. doi:10.3390/microorganisms9010004
Monteoliva-García, A., Martín-Pascual, J., Muñío, M. M., and Poyatos, J. M. (2019). Removal of carbamazepine, ciprofloxacin and ibuprofen in real urban wastewater by using light-driven advanced oxidation processes. Int. J. Environ. Sci. Technol. 16, 6005–6018. doi:10.1007/s13762-019-02365-9
Morais, E. G., Cristofoli, N. L., Maia, I. B., Magina, T., Cerqueira, P. R., Teixeira, M. R., et al. (2021). Microalgal systems for wastewater treatment: Technological trends and challenges towards waste recovery. Energies 14, 8112. doi:10.3390/en14238112
Muir, D., Simmons, D., Wang, X., Peart, T., Villella, M., Miller, J., et al. (2017). Bioaccumulation of pharmaceuticals and personal care product chemicals in fish exposed to wastewater effluent in an urban wetland. Sci. Rep. 7, 16999. doi:10.1038/s41598-017-15462-x
Naddeo, V., Meric, S., Kassinos, D., Belgiorno, V., and Guida, M. (2009). Fate of pharmaceuticals in contaminated urban wastewater effluent under ultrasonic irradiation. Water Res. 43, 4019–4027. doi:10.1016/j.watres.2009.05.027
Nakada, N., Tanshima, T., Shinohara, H., Kiri, K., and Takada, H. (2006). Pharmaceutical chemicals and endocrine disrupters in municipal wastewater in Tokyo and their removal during activated sludge treatment. Water Res. 40, 3297–3303. doi:10.1016/j.watres.2006.06.039
Narayanan, M., El-sheekh, M., Ma, Y., Pugazhendhi, A., Natarajan, D., Kandasamy, G., et al. (2022). Current status of microbes involved in the degradation of pharmaceutical and personal care products (PPCPs) pollutants in the aquatic ecosystem. Environ. Pollut. 300, 118922. doi:10.1016/j.envpol.2022.118922
Nigam, H., Jain, R., Malik, A., and Singh, V. (2022). Effect of different polystyrene nano-plastic concentrations on Chlorella pyrenoidosa. Algal Res. 67, 102835. doi:10.1016/j.algal.2022.102835
Ohoro, C. R., Adeniji, A. O., Elsheikh, E. A. E., Otim, M., Okoh, O. O., et al. (2022). Influence of physicochemical parameters on PPCP occurrences in the wetlands. Environ. Monit. Assess. 194, 339. doi:10.1007/s10661-022-09990-xAl-Marzouqi
Oluwole, A. O., Omotola, E. O., and Olatunji, O. S. (2020). Pharmaceuticals and personal care products in water and wastewater: A review of treatment processes and use of photocatalyst immobilized on functionalized carbon in AOP degradation. BMC Chem. 14, 62. doi:10.1186/s13065-020-00714-1
Oulton, R. L., Kohn, T., and Cwiertny, D. M. (2010). Pharmaceuticals and personal care products in effluent matrices: A survey of transformation and removal during wastewater treatment and implications for wastewater management. J. Environ. Monit. 12, 1956–1978. doi:10.1039/c0em00068j
Özengin, N., and Elmaci, A. (2016). Removal of pharmaceutical products in a constructed wetland. Iran. J. Biotechnol. 14, 221–229. doi:10.15171/ijb.1223
Peng, F. Q., Ying, G. G., Yang, B., Liu, S., Lai, H. J., Liu, Y. S., et al. (2014). Biotransformation of progesterone and norgestrel by two freshwater microalgae (Scenedesmus obliquus and Chlorella pyrenoidosa): Transformation kinetics and products identification. Chemosphere 95, 581–588. doi:10.1016/j.chemosphere.2013.10.013
Pohl, J., Golovko, O., Carlsson, G., Eriksson, J., Glynn, A., Örn, S., et al. (2020). Carbamazepine ozonation byproducts: Toxicity in zebrafish (Danio rerio) embryos and chemical stability. Environ. Sci. Technol. 54, 2913–2921. doi:10.1021/acs.est.9b07100
Rao, K. S., and Belorkar, N. (2010). Development and validation of a specific stability indicating liquid chromatographic method for carbamazepine in bulk and pharmaceutical dosage forms. J. Adv. Pharm. Res. 1, 36–47.
Rempel, A., Gutkoski, J. P., Nazari, M. T., Biolchi, G. N., Cavanhi, V. A. F., Treichel, H., et al. (2021). Current advances in microalgae-based bioremediation and other technologies for emerging contaminants treatment. Sci. Total Environ. 772, 144918. doi:10.1016/j.scitotenv.2020.144918
Reyes, N. J. D. G., Geronimo, F. K. F., Yano, K. A. V., Guerra, H. B., and Kim, L. H. (2021). Pharmaceutical and personal care products in different matrices: Occurrence, pathways, and treatment processes. Water 13, 1159. doi:10.3390/w13091159
Richmond, E. K., Grace, M. R., Kelly, J. J., Reisinger, A. J., Rosi, E. J., and Walters, D. M. (2017). Pharmaceuticals and personal care products (PPCPs) are ecological disrupting compounds (EcoDC). Elem. Sci. Anth. 5, 66. doi:10.1525/elementa.252
Rizzo, L., Meric, S., Guida, M., Kassinos, D., and Belgiorno, V. (2009). Heterogenous photocatalytic degradation kinetics and detoxification of an urban wastewater treatment plant effluent contaminated with pharmaceuticals. Water Res. 43, 4070–4078. doi:10.1016/j.watres.2009.06.046
Rosario-Ortiz, F. L., Wert, E. C., and Snyder, S. A. (2010). Evaluation of UV/H2O2 treatment for the oxidation of pharmaceuticals in wastewater. Water Res. 44, 1440–1448. doi:10.1016/j.watres.2009.10.031
Rosi-Marshall, E. J., Kincaid, D. W., Bechtold, H. A., Royer, T. V., Rojas, M., and Kelly, J. J. (2013). Pharmaceuticals suppress algal growth and microbial respiration and alter bacterial communities in stream biofilms. Ecol. Appl. 23, 583–593. doi:10.1890/12-0491.1
Rosi-Marshall, E. J., and Royer, T. V. (2012). Pharmaceutical compounds and ecosystem function: An emerging research challenge for aquatic ecologists. Ecosystems 15, 867–880. doi:10.1007/s10021-012-9553-z
Ross, A. B., Jones, J. M., Kubacki, M. L., and Bridgeman, T. (2008). Classification of macroalgae as fuel and its thermochemical behaviour. Bioresour. Technol. 99, 6494–6504. doi:10.1016/j.biortech.2007.11.036
Saeid, S., Kråkström, M., Tolvanen, P., Kumar, N., Eränen, K., Mikkola, J. P., et al. (2020). Advanced oxidation process for degradation of carbamazepine from aqueous solution: Influence of metal modified microporous, mesoporous catalysts on the ozonation process. Catalysts 10, 90. doi:10.3390/catal10010090
Sakthivel, R., Ramesh, K., Purnachandran, R., and Mohamed Shameer, P. (2018). A review on the properties, performance and emission aspects of the third generation biodiesels. Renew. Sustain. Energy Rev. 82, 2970–2992. doi:10.1016/j.rser.2017.10.037
Salvi, B. L., and Panwar, N. L. (2012). Biodiesel resources and production technologies–A review. Renew. Sustain. Energy Rev. 16, 3680–3689. doi:10.1016/j.rser.2012.03.050
Schoenell, E. K., Otto, N., Rodrigues, M. A. S., and Metzger, J. W. (2022). Removal of organic micropollutants from treated municipal wastewater by O3/UV/H2O2 in a UVA-LED reactor. Ozone Sci. Eng. 44, 172–181. doi:10.1080/01919512.2021.1900716
Seo, C. D., Yoom, H. S., Song, M. J., Kim, K. A., Kim, S. Y., and Son, H. (2020). Occurrence and behavior of residual pharmaceuticals in the Nakdong River basin. J. Korean Soc. Environ. Eng. 42, 177–187. (in Korean). doi:10.4491/KSEE.2020.42.4.177
Snyder, S. A. (2008). Occurrence, treatment, and toxicological relevance of EDCs and pharmaceuticals in water. Ozone Sci. Eng. 30, 65–69. doi:10.1080/01919510701799278
Somathilake, P., Dominic, J. A., Achari, G., Langford, C. H., and Tay, J. H. (2019). Influence of UV dose on the UV/H2O2 process for the degradation of carbamazepine in wastewater. Environ. Technol. 40, 3031–3039. doi:10.1080/09593330.2018.1464065
Sun, S., Hu, Y., Xu, M., Cheng, F., Zhang, H., and Li, Z. (2022). Photo-Fenton degradation of carbamazepine and ibuprofen by iron-based metal-organic framework under alkaline condition. J. Hazard. Mat. 424, 127698. Part C. doi:10.1016/j.jhazmat.2021.127698
Ternes, T. A., Stüber, J., Herrmann, N., McDowell, D., Ried, A., Martin Kampmann, M., et al. (2003). Ozonation: A tool for removal of pharmaceuticals, contrast media and musk fragrances from wastewater?. Water Res. 37, 1972–1982. doi:10.1016/S0043-1354(02)00570-5
Thanekar, P., Panda, M., and Gogate, P. R. (2018). Degradation of carbamazepine using hydrodynamic cavitation combined with advanced oxidation processes. Ultrason. Sonochem. 40, Part A, 567–576. doi:10.1016/j.ultsonch.2017.08.001
Tripathi, R., Singh, J., and Thakur, I. S. (2015). Characterization of microalga Scenedesmus sp. ISTGA1 for potential CO2 sequestration and biodiesel production. Renew. Energy 74, 774–781. doi:10.1016/j.renene.2014.09.005
Um, B. H., and Kang, C. H. (2019). An economical analysis on fuel switching model of coal power plant using herbaceous biomass. JKSAE 61, 89–99. (in Korean). doi:10.5389/KSAE.2019.61.3.089
Villota, N., Ferreiro, C., Qulatein, H. A., Lomas, J. M., and Lombraña, J. I. (2021). Turbidity changes during carbamazepine oxidation by photo-Fenton. Catalysts 11, 894. doi:10.3390/catal11080894
Vogna, D., Marotta, R., Andreozzi, R., Napolitano, A., and d’Ischia, M. (2004). Kinetic and chemical assessment of the UV/H2O2 treatment of antiepileptic drug carbamazepine. Chemosphere 54, 497–505. doi:10.1016/S0045-6535(03)00757-4
Wang, Q., Liu, W., Li, X., Wang, R., and Zhai, J. (2020a). Carbamazepine toxicity and its co-metabolic removal by the cyanobacteria Spirulina platensis. Sci. Total Environ. 706, 135686. doi:10.1016/j.scitotenv.2019.135686
Wang, S., and Zhou, N. (2016). Removal of carbamazepine from aqueous solution using sono-activated persulfate process. Ultrason. Sonochem. 29, 156–162. doi:10.1016/j.ultsonch.2015.09.008
Wang, Y., Jing, B., Wang, F., Wang, S., Liu, X., Ao, Z., et al. (2020b). Mechanism insight into enhanced photodegradation of pharmaceuticals and personal care products in natural water matrix over crystalline graphitic carbon nitrides. Water Res. 180, 115925. doi:10.1016/j.watres.2020.115925
Wee, S. Y., Aris, A. Z., Yusoff, F. M., and Praveena, S. M. (2020). Occurrence of multiclass endocrine disrupting compounds in a drinking water supply system and associated risks. Sci. Rep. 10, 17755. doi:10.1038/s41598-020-74061-5
Wellburn, A. R. (1994). The spectral determination of chlorophylls a and b, as well as total carotenoids, using various solvents with spectrophotometers of different resolution. J. Plant Physiol. 144, 307–313. doi:10.1016/S0176-1617(11)81192-2
Wert, E. C., Rosario-Ortiz, F. L., and Snyder, S. A. (2009). Using ultraviolet absorbance and color to assess pharmaceutical oxidation during ozonation of wastewater. Environ. Sci. Technol. 43, 4858–4863. doi:10.1021/es803524a
Xia, K., Bhandari, A., Das, K., and Pillar, G. (2005). Occurrence and fate of pharmaceuticals and personal care products (PPCPs) in biosolids. J. Environ. Qual. 34, 91–104. doi:10.2134/jeq2005.0091
Xie, J., Li, H., Wang, S., Chen, H., Jiang, W., Zhang, L., et al. (2022). Removal and fate of carbamazepine in the microbial fuel cell coupled constructed wetland system. Environ. Eng. Res. 27, 210119. doi:10.4491/eer.2021.119
Xiong, J. Q., Kurade, M. B., Abou-Shanab, R. A. I., Ji, M. K., Choi, J., Kim, J. O., et al. (2016). Biodegradation of carbamazepine using freshwater microalgae Chlamydomonas mexicana and Scenedesmus obliquus and the determination of its metabolic fate. Bioresour. Technol. 205, 183–190. doi:10.1016/j.biortech.2016.01.038
Xiong, J. Q., Kurade, M. B., and Jeon, B.-H. (2018). Can microalgae remove pharmaceutical contaminants from water? Trends Biotechnol. 36, 30–44. doi:10.1016/j.tibtech.2017.09.003
Xiong, J. Q., Kurade, M. B., and Jeon, B. H. (2017). Biodegradation of levofloxacin by an acclimated freshwater microalga, Chlorella vulgaris. Chem. Eng. J. 313, 1251–1257. doi:10.1016/j.cej.2016.11.017
Xu, J., Li, L., Guo, C., Zhang, Y., and Meng, W. (2013). Photocatalytic degradation of carbamazepine by tailored BiPO4: Efficiency, intermediates and pathway. Appl. Catal. B Environ. 130–131, 285–292. doi:10.1016/j.apcatb.2012.11.013
Yacouba, Z. A., Lesage, G., Mendret, J., Zaviska, F., Petit, E., and Brosillon, S. (2021). Fate and toxicity of carbamazepine and its degradation by-products during coupling of ozonation and nanofiltration for urban wastewater reuse. Front. Environ. Chem. 2, 798785. doi:10.3389/fenvc.2021.798785
Yan, Z., Xu, L., Zhang, W., Yang, G., Zhao, Z., Wang, Y., et al. (2021). Comparative toxic effects of microplastics and nanoplastics on Chlamydomonas reinhardtii: Growth inhibition, oxidative stress, and cell morphology. J. Water Process Eng. 43, 102291. doi:10.1016/j.jwpe.2021.102291
Yang, L., Liang, L., Wang, L., Zhu, J., Gao, S., and Xia, X. (2019). Accelerated photocatalytic oxidation of carbamazepine by a novel 3D hierarchical protonated g-C3N4/BiOBr heterojunction: Performance and mechanism. Appl. Surf. Sci. 473, 527–539. doi:10.1016/j.apsusc.2018.12.180
Yang, W. (2016). Status and perspective of biomass co-firing to pulverized coal power plants. KEPCO J. Electr. Power Energy 2, 525–529. (in Korean). doi:10.18770/KEPCO.2016.02.04.525
Yang, Y., Ok, Y. S., Kim, K. H., Kwon, E. E., and Tsang, Y. F. (2017). Occurrences and removal of pharmaceuticals and personal care products (PPCPs) in drinking water and water/sewage treatment plants: A review. Sci. Total Environ. 596–597, 303–320. doi:10.1016/j.scitotenv.2017.04.102
Yin, L., Wang, B., Yuan, H., Deng, S., Huang, H., Wang, Y., et al. (2017). Pay special attention to the transformation products of PPCPs in environment. Emerg. Contam. 3, 69–75. doi:10.1016/j.emcon.2017.04.001
Zhai, J., Wang, Q., Li, Q., Shang, B., Rahaman, M. H., Liang, J., et al. (2018). Degradation mechanisms of carbamazepine by δ-MnO2: Role of protonation of degradation intermediates. Sci. Total Environ. 640, 981–988. doi:10.1016/j.scitotenv.2018.05.368
Zhang, P., Zhou, H., Li, K., Zhao, X., Liu, Q., Li, D., et al. (2018). Occurrence of pharmaceuticals and personal care products, and their associated environmental risks in Guanting Reservoir and its upstream rivers in north China. RSC Adv. 8, 4703–4712. doi:10.1039/C7RA12945A
Zhang, Y., Geißen, S. U., and Gal, C. (2008). Carbamazepine and diclofenac: Removal in wastewater treatment plants and occurrence in water bodies. Chemosphere 73, 1151–1161. doi:10.1016/j.chemosphere.2008.07.086
Keywords: microalgae, emerging micropollutant, carbamazepine, sodium hypochlorite, water treatment, biomass
Citation: Do J-M, Yeo H-T, Do G-S, Hong JW and Yoon H-S (2022) Enhancement of carbamazepine removal rate using Tetradesmus obliquus KNUA061 and NaOCl and utilization of the resulting biomass. Front. Energy Res. 10:1004613. doi: 10.3389/fenrg.2022.1004613
Received: 27 July 2022; Accepted: 11 November 2022;
Published: 28 December 2022.
Edited by:
Blake Hovde, Los Alamos National Laboratory (DOE), United StatesReviewed by:
Karmegam Natchimuthu, Government Arts College (Autonomous), IndiaKseniya Sorokina, Boreskov Institute of Catalysis (RAS), Russia
Copyright © 2022 Do, Yeo, Do, Hong and Yoon. This is an open-access article distributed under the terms of the Creative Commons Attribution License (CC BY). The use, distribution or reproduction in other forums is permitted, provided the original author(s) and the copyright owner(s) are credited and that the original publication in this journal is cited, in accordance with accepted academic practice. No use, distribution or reproduction is permitted which does not comply with these terms.
*Correspondence: Ji Won Hong, andob25nQGtudS5hYy5rcg==; Ho-Sung Yoon, aHN5QGtudS5hYy5rcg==