- State Key Laboratory of Oil and Gas Reservoir Geology and Exploitation, School of Oil and Natural Gas Engineering, Southwest Petroleum University, Chengdu, China
The malignant leakage of complex formations seriously restricts the drilling time and has become a major drilling technical problem that needs to be solved urgently at this stage. In particular, the malignant leakage of large fractures and fractured formations is difficult to construct, and the success rate of plugging is low. In this paper, according to the above formation conditions, by analyzing the leakage mechanism of the malignant leakage formation, the method of plugging the large fractures and broken fractured formations is summarized. The adiabatic copolymerization co-hydrolysis method was used as the gel synthesis method, 2-methacrylic acid amide and acrylamide (AM) were used as the main raw materials, and the redox initiation system composed of sodium sulfate and sodium bisulfite was used as the initiator. Using its redox initiation characteristics, high-strength bonding between organic polymer covalent bonds was achieved by a self-made cross-linking agent; using the thermoplasticity of hot-melt adhesive, the initiator is wrapped in hot-melt adhesive particles to achieve high-temperature slow-release initiation, and finally, a gel plugging agent with good compatibility with drilling fluid, controllable gel formation time, stable gel formation, and good gel breaking performance was synthesized, which is suitable for severe lost circulation under high-temperature and high-pressure conditions. A compatibility study of gel and common drilling fluid systems, the effect of temperature on gel formation, gel rheological properties under high temperature and high pressure, gel start-up pressure, and gel breakability tests were evaluated under laboratory conditions. The experimental results show that the gel has good compatibility with common drilling fluid systems; with the increase of temperature, the gel time of the gel plugging agent gradually decreases, and the gel time can be adjusted from 1 to 3 h at a high temperature of 150°C; when the concentration of the gel in the experimental slurry exceeds 2%, its rheological properties change abruptly; under certain conditions, the starting pressure of the gel can reach up to 6.5 MPa; and under the condition of 150°C, the gel breaking rate was over 98% after 24 h. Various experiments show that the gel’s performance meets the requirements for plugging malignant leakage formations, and the effect is good. Compared with traditional gel materials, the gel plugging agent synthesized in this paper has the characteristics of a simple configuration process and superior performance with drilling fluid, which provides a new idea for malignant leakage plugging.
Introduction
Loss of circulation is a phenomenon where various working fluids (including drilling fluid, cement slurry, completion fluid, and other fluids) leak into the formation under the action of pressure differences during various downhole operations such as drilling, cementing, and testing (Fan et al., 2018). The “vicious leakage” mainly refers to the phenomenon where the mud pumped into the wellbore has no circulation and no return during the drilling process. It has the characteristics of high leakage speed, huge leakage, difficult leakage processing, and easy recurrence of leakage. Malignant leakage mainly occurs in karst formations and fractured formations with large widths (Zhou et al., 2012). Half of the widths of wide fractures are more than millimeters, and even some fractures can reach about 10 mm in width; the underground voids of karst strata are much larger than wide fractures malignant leakage will seriously restrict the drilling speed during the drilling process. In particular, the malignant leakage that occurs in complex formations often requires long-term, multiple times, multiple methods, and multiple leakage plugging construction, which is inconsistent with the drilling goal of “safety, high quality, high efficiency, and economy” (Li and Xiang, 2020). The malignant leakage in complex formations has become a major drilling technology problem that has not been fully solved in the current stage. The study believes that for micro and narrow fractures, the use of irregular rigid and flexible plugging materials has a better effect; for large fractures and fractured formations, inorganic plugging materials are difficult to construct, the plugging success rate is low, it is difficult to significantly improve the pressure bearing capacity of the formation, the cost of plugging is high, and it is difficult to deal with the plugging of malignant leakage in complex formations (Du et al., 2019).
With the continuous emergence of new materials and new technologies in the petroleum industry, the gel plugging materials have been improved and upgraded for the malignant leakage of the complex formation. Jianshan (2019) takes the rigid aromatic ring as the main body of the structural unit and develops the SMSHIELD intelligent gel plugging agent with the carbon–nitrogen bond, carbon–carbon bond, and other chemical bonds with high stability and outstanding rigidity as the main chain. Such gel materials are characterized by strong deformation and easy formation, and they could effectively bond the components in the mud cake and fully fill all spatial positions of the mud cake to make it denser. Such intelligent gel can be combined with other treating agents, but they were not compatible with the drilling fluid of other systems. Suming et al. (2007) have developed a pressure-bearing delay plugging agent, OC L-GYDL. Such a plugging agent has a strong pressure bearing capacity, and the gel time is adjustable. However, it could not be consistent with high temperature; therefore, it could not be applied to high-temperature formations.
In view of the deficiency of the above gel plugging materials that need the cooperation of a mud system and are not resistant to high temperatures, a new gel plugging material, ZND-6, is developed. The gel is compatible with the general water-based drilling fluid, and it can be mixed with the drilling fluid on the ground to maintain the relatively low viscosity. It may enter the target formation with the drill pipe pump and utilize the temperature of the formation within a specified time to form a gel polymer with certain strength so as to block the formation. Its performance is superior to that of the general gel, and it has features such as a controlled gelling time, high temperature resistance of 150°C, good pressure bearing capacity, etc. It solves the problem of the compatibility of the general loss-stopping agent with the leakage fracture size and can effectively block the malignant leakage caused by large fractures and broken formations.
Gel synthesis
Reagent and main instruments
Acrylamide (AM), analytically pure (Elsharafi and Bai, 2017); initiator (self-made); 2-methacrylic acid amide, analytically pure; sodium hydroxide (NaOH), analytically pure; deionized water; sodium persulfate (Na2S2O8), analytically pure (Zhao et al., 2018); sodium bisulfite (NaHSO3), analytically pure; sodium sulfate (Na2SO4), analytically pure; sodium formate, analytically pure; surfactant OP-10, analytically pure.
FFD-50 adiabatic reactor; 85-1 magnetic stirrer; HHS-11-6 electric water bath; GJD-B12K variable-frequency and high-speed mixer; Granulator.
Synthesis
The synthesis method of gel is the adiabatic copolymerization co-hydrolysis method. This method does not require a separate hydrolysis operation, which not only reduces the production cost but also saves the production cycle. The steps of preparing gel by adiabatic copolymerization co-hydrolysis are as follows.
First, 2-methacrylic acid amide and acrylamide were added to an adiabatic reactor according to a certain feed ratio. According to the requirements of gel fluidity, the acrylate monomer was added, deionized water and instant additives were added, and an appropriate amount of alkali was added to adjust the pH value of the system. After stirring, a monomer solution with a concentration of 35% was formed. Then the solution was controlled to the polymerization temperature (40°C) (Kumar et al., 2014), and an initiator was added after removing oxygen by nitrogen. After adiabatic polymerization for 8 h (Barreira and Negrão, 2017), the colloid was taken out for granulation, drying, crushing, and packaging to obtain the gel sample.
The initiator is a redox initiation system composed of sodium persulfate and sodium bisulfite, and its addition amount is 2% of the total weight of the monomer solution (Quiroz et al., 2022). The instant additive is composed of sodium sulfate, sodium formate, and surfactant OP-10, and the dosage is 3% of the total weight of the monomer solution (Liu et al., 2018).
Evaluation of gel performance
Reagent and main instrument
KPS, analytical reagent (Gregolin et al., 2010); HV-CMC, analytical reagent; SPNH, analytical reagent; the formula for the experimental pulp is as follows:
H2O + bentonite + barytes, +1%HV-CMC+3%SPNH, the experimental mud density is 1.5.
M7500 high-temperature and high-pressure rheometer; QD-2 test device on plugging materials; XGRL-4 aging furnace.
Microstructure characterization
According to the experimental pulp formula, we add 3% gelatin ZND-6 to the configured experimental pulp, stir for 10 min at low speed, and then put it into the aging tank. After aging for 10 h at 150°C, gel particles are gelatinized. The sample status before and after the experiment is shown in Figure 1. It could be found that the sample has a strong thixotropy after adding the gel particles. Before aging, the gel particles in the sample are distributed independently in the experimental slurry, and there is no cementation between them. After aging, the gel particles agglomerate with each other and have a strong structural force. We used a scanning electron microscope to observe the microstructure of the samples after aging, as shown in Figure 2. It was found that the gel particles were completely gelled under the conditions of 10 h and high temperature, and a dense three-dimensional network structure is formed between the gel molecules. The reticular formation of the gel molecular closely combines with other gel molecules that are independently distributed to form the large gel mass so that the sample has strong structural strength (Wang et al., 2020).
Compatibility study of gel and common drilling fluid systems
In order to explore the effect of gel ZND-6 mixed into drilling fluid on the performance of drilling fluid, the compatibility experiment of gel with NaCl, polysulfonic acid, and polymer drilling fluid systems was carried out in the laboratory. The drilling fluid before and after aging after adding gel was mainly investigated for changes in rheology and dehydration wall-building. The experimental results are shown in Tables 1, 2, 3.
It can be seen from Table 1 that adding the gel ZND-6 to the NaCl drilling fluid can enhance the static shear force of the system, reduce the filtration loss, and enhance its water loss and wall-building ability without affecting the fluidity of the system. Gel ZND-6 has good compatibility with NaCl drilling fluid.
It can be seen from Table 2 that the effect of gel ZND-6 on the system is generally favorable after adding the polysulfonic drilling fluid, which can improve the rheological properties of the system and improve the ability of water loss and wall-building. Gel ZND-6 has good compatibility with polysulfonic drilling fluid.
It can be seen from Table 3 that ZND-6 gel can improve the fluidity of the drilling fluid after adding the polymer drilling fluid, while maintaining the static shear force of the system, and the fluid loss of the drilling fluid is low. The performance after aging also meets the general requirements for drilling fluids and has good compatibility with polymer drilling fluids.
To sum up, the gel ZND-6 has good compatibility with drilling fluids such as NaCl, polysulfonic acid, and polymers and can improve the water loss and wall-building properties as well as the viscosity and static shear force of the drilling fluid. The compatibility between the gel and the commonly used drilling fluid system is sufficient to meet the requirements of solving the malignant loss of large fractures and broken formations.
Influence of temperature on the gelling time
The effect of temperature on the gel formation time is obvious: the half-life of the gel polymer initiator decreases exponentially with the increase in temperature. The gelling times of gelatin in water and the experimental slurry at 80°C–150°C are studied, respectively, to determine the relevant time. The experimental results are shown in Tables 4, 5.
It can be seen from the experimental results that the gel-forming time of the gel ZND-6 gradually decreases with an increase in the experimental temperature. The reasons and results may be that ① the half-life of the initiator decreases with the increase of temperature, which shortens the gel formation time (Li et al., 2015) and ② with the increase of the experimental temperature, the melting rate of the hot-melt adhesive capsule in the gel increases, resulting in a faster release of the initiator from the hot-melt adhesive and a shortening of the gel formation time (Li, X et al., 2022).
Evaluation of the gel’s rheological property
To determine the rheological data of the gel ZND-6 under the downhole high-temperature and high-pressure conditions, the rheological property of the gel ZND-6 is tested for 10H in a high-temperature and high-pressure environment with the M7500 high-temperature and high-pressure rheometer produced by Grace Company. The time length includes the time before and after the gelation of gel ZND-6 and the period after gelation so as to determine the viscosity change before gelation and the stability after gelation.
Add the gel ZND-6 with a concentration of 1.5% to the experimental pulp and increase the temperature to 150°C. Then, test the sample viscosity every 20 min under the environment at a pressure of 20 MPa, with the experimental results shown in Figure 3. The samples are gelatinized at 60 min, and the highest viscosity reaches 19000 mPa s. Besides, the sample viscosity basically remains stable in the remaining experiment time.
According to Figures 4, 5, the rheological properties of the samples only increase linearly with the gel concentration when the gel concentration is 0.5%, 1.0%, or 1.5%. After the gel concentration reaches 2%, all rheological data of the sample show sudden changes. When the gel concentration is relatively low, the gel particles in the sample are far away; therefore, it is not easy for them to contact each other and form a three-dimensional network structure between the particles. Actually, they just change the rheological property of the samples through the swelling and gelation of each individual gel particle. After the gel concentration reaches or exceeds 2%, not only the individual gel particle has an influence on the rheological property of the samples. Besides, with the increase of gel particles per unit volume, the distances between the gel particles are reduced so as to form a network structure among the particles and create a larger gel mass. Such large gel masses could obviously affect the rheological properties of samples more than single gel particles.
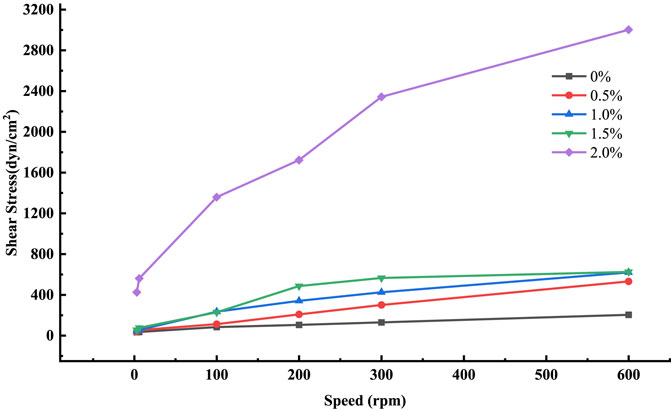
FIGURE 4. Comparison of shearing stress at different rotating speeds under different concentrations of gel.
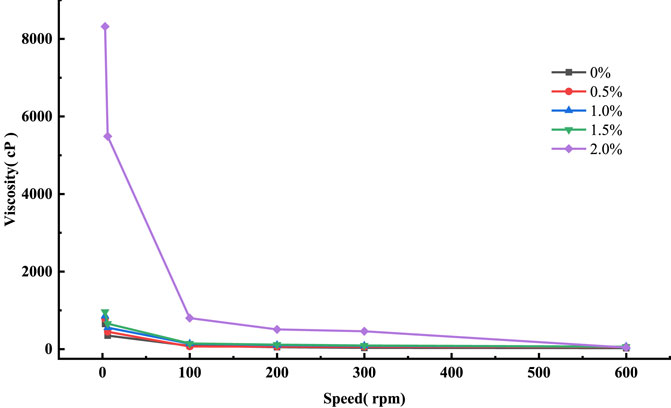
FIGURE 5. Comparison of viscosity at different rotating speeds under different concentrations of gel.
It could be judged from the above experiments that the rheological property of gel could basically maintain stability under the downhole high-temperature and high-pressure conditions, and it could block the broken formation stably for a long time, which could prolong the safe operation time effectively and strengthen the safety of well control (Li, Z et al., 2022). Besides, the rheological property of the slurry will change suddenly when the concentration of the gel ZND-6 exceeds 2%. Hence, the optimal dosage concentration of gel ZND-6 should not be less than 2%.
Starting pressure test of gel
After reaching the position of lost circulation and penetrating into large fractures and fractured formations, the gel swells into glue in the well slurry to block large pores and fractures. After the gel plugging, there is a pressure difference inside and outside the wellbore. After the gelatinization, the pressure difference that the plugging material can withstand is the start-up pressure of the gel, and it is a key metric to evaluate its plugging performance (Yokoi et al., 2022).
The QD-2 test device on plugging materials is adopted to simulate leakage layer cracks with different seam widths (1, 2, 3, 4, and 5 mm). We add the gel to the experimental pulp and investigate the plugging pressure-bearing capacity of the gel plugging materials on the large pores and fractures causing the malignant leakage under the high-temperature and high-pressure conditions.
The specific experimental method is as follows: add the gel ZND-6 to the experimental pulp and put it into the plugging material test device after mixing evenly, pressurize the upper valve rod by 0.5 MPa, close the lower valve rod, and let it stand for several hours at a certain temperature. After the gel in the samples seals the joint board, open the foot valve and increase the pressure with 0.5 MPa as the gradient to observe whether there is a sample extrusion. After the samples are extruded, stop the experiment and record the pressure, which is the start-up pressure of the gel. The start-up pressures vary at different temperatures, gel concentrations, and high-preservation standing times. The experimental results are as shown in Table 6.
Data in Table 6 indicates that under the same concentration, the start-up pressure increases with the rise in temperature, and the maximum start-up pressure is 6.5 MPa in the temperature range of 80°C–150°C; when the temperature exceeds 150°C, the sample’s start-up pressure decreases with the rise in temperature. Under the same temperature, the ZND-6 start-up pressure increases as the gel concentration increases. At 150°C and a concentration of 5%, the gel will form a tight and elastic air blockage, as shown in Figure 6.
This phenomenon occurs mainly because the hydrophobic group in gel ZND-6 will form a network structure in the slurry. This network structure not only makes the gel combine into an air blockage but also cements with the tank externally. With the rise in temperature, the strength of the network structure will increase. Once it exceeds the limiting temperature of gel, the strength of the structure will decrease. Therefore, the start-up pressure of gel will increase with the rise in temperature in the range from 80°C to 150°C. After it exceeds 150°C, the start-up pressure will decrease with the rise in temperature. Similarly, the strength of the gel’s network structure will be enhanced with the concentration of gel in the experimental pulp.
Gel breaking test
The core material of the oxidation breaker is potassium persulfate or ammonium persulfate. The principle is that the breaker can be dissolved into a highly active, solid strong oxidant in contact with water (Guo et al., 2016). The advantage of oxidative gel breakers is that they reduce the effect of gel breakers on the rheological properties of fracturing fluid.
After the reservoir leakage plugging, it is necessary to reverse the gel breaking in the lost circulation plugging slurry to ensure the downhole production capacity. Hence, the gel-breaking test shall be carried out. The calculation formula of the gel breaking rate is:
D is the gel breaking rate; m is the gel quality before gel breaking, g; and m0 is the remaining gel quality after gel breaking, g.
Add 3% gel to the experimental pulp, and then add 1% KPS. After mixing evenly, pour it in the aging tank and take out the sample after rolling it in the roller furnace for a certain time at 150°C. Then, screen out the remaining gel with the screen and weigh it to calculate the gel breaking rate. The experimental results are as shown in Figure 7.
It can be seen from Figure 7 that the gel breaking rate could reach about 50% after 18 h at 150°C; after 24 h, the gel breaking rate could reach 98%. At this time, the gel breaking of gel is basically complete, meeting the requirements of the reverse drainage of the general reservoir.
Applications in the field
Well Shuangmiao 1
Well Shuangmiao 1 in Dazhou is a key vertical exploration well in southwest China. There are six leakage points in the 2083–3,573 m well section. A high-pressure gas layer was drilled at a depth of 3,446–3,448 m, and the bottom hole overflowed. In order to seal the leakage layer and protect the gas layer, the on-site technicians have successively adopted bridge plug plugging, nine times of cement plugging, and bridge plug and cement composite plugging methods, all of which have not been effective, taking up to 2 months, and the phenomenon of simultaneous leakage and leakage has not been controlled. Wellhead two single ram blowout preventers in the upper ram damage failure, four anti-nozzle lines in three damaged, throttling blowout, and casing pressure are extremely difficult to reduce. The leakage layer of 3,446–3,448 m is the main factor to induce complex downhole conditions.
In order to effectively plug the three leakage layers at a depth of 3,483, 3,446, and 3,436 m, the special gel plugging technology is tried. A total of 70 m3 of gel was injected into the three layers, and then 25 m3 of cement was added. The construction was successful. After pressure relief, the casing pressure drops to 12 MPa and then shuts down. The standpipe pressure is zero, and the casing pressure remains unchanged. Shut-in observation riser pressure is still zero, the casing pressure is still zero, to achieve the purpose of blocking the annulus, cut off the bottom hole high-pressure gas layer. The logging results show that the cement sheath is in the well depth of 2,250–3,200 m, the cement return height is in the well depth of 2,355 m, the ZND-6 gel column in the annulus of the leakage layer is nearly 100 m, and the plugging is successful.
Well Liu 67–72
Well Liu 67–72 in Changqing leakage occurred at a depth of 1,629 m. The bridge slurry (the particle size of the bridging material is 3 ∼ 5 mm) and the cement plug failed nine times. In order to plug the leakage point, ZND-6 gel plugging technology is adopted. Drill down the drill pipe a depth of 1,500 m, pump 34 m3 concentration of 1.2% gel ZND-6, and then inject 12 m3 water; next, pull out the drill, and allow it to stand for 6 h. Down to the bottom of the well, open the pump return, normal displacement. After circulating the drilling fluid for 15 min, drilling 8 m at 150 kN and 65 r/min, the pump pressure displacement is normal and the plugging is successful.
Conclusion
1. The high-temperature gel plugging agent ZND-6 is synthesized with a controlled gelling time, and the free radical is aggregated to form the high-strength gel with dense cross-linking.
2. The gel plugging materials ZND-6 could penetrate the broken formation to form the air blockage. When the gel concentration is 1.5% at 150°C, the sample viscosity reaches 19,000 mPa s and the stable time is greater than 10 h.
3. It could be found from the experiment that after the concentration of gel reaches 2% in the slurry, it is easier to form the three-dimensional network structure and become the larger gel mass, and the sample performance may change suddenly. In view of the actual construction and economic benefits, it is suggested to control the gel concentration at 3%–5%.
4. The temperature resistance of the gel ZND-6 could reach 150°C. When the temperature exceeds 150°C, the gel strength would decrease.
5. Under the condition of 150°C, the gel breaking rate is over 98% after 24 h, which meets the requirement of general reservoir reverse flow.
6. According to field application, the innovation of gel plugging agent ZND-6 is that it can be used alone with drilling fluid and has a good effect on plugging malignant leakage layers. It can well solve the leakage problems that cannot be solved by methods such as fractured, porous, and broken formations; bridge plugging; and plugging while drilling. It is unique in dealing with the leakage problems of aquifers and the same layer of leakage.
Data availability statement
The original contributions presented in the study are included in the article/supplementary material; further inquiries can be directed to the corresponding author.
Author contributions
YB: ideas and suggestions; LW: data collection and drafting of papers; PL: approval of the final version of the paper for publication; and DL: made important revisions to the paper.
Conflict of interest
The authors declare that the research was conducted in the absence of any commercial or financial relationships that could be construed as a potential conflict of interest.
Publisher’s note
All claims expressed in this article are solely those of the authors and do not necessarily represent those of their affiliated organizations or those of the publisher, the editors, and the reviewers. Any product that may be evaluated in this article or claim that may be made by its manufacturer is not guaranteed or endorsed by the publisher.
References
Barreira, E. M., and Negrão, C. O. R. (2017). Performance of pressure relief devices on flow start-up of gelled waxy crude oils in pipelines. J. Petroleum Sci. Eng. 158, 158234–158242. doi:10.1016/j.petrol.2017.08.049
Du, D., Pu, W., Tan, X., and Liu, R. (2019). Experimental study of secondary crosslinking core-shell hyperbranched associative polymer gel and its profile control performance in low-temperature fractured conglomerate reservoir. J. Petroleum Sci. Eng. 179, 179912–179920. doi:10.1016/j.petrol.2019.05.006
Elsharafi, M. O., and Bai, B. (2017). Experimental work to determine the effect of load pressure on the gel pack permeability of strong and weak preformed particle gels. Fuel 188, 188332–188342. doi:10.1016/j.fuel.2016.10.001
Fan, X., Zhao, P., Zhang, Q., Zhang, T., Zhu, K., and Zhou, C. (2018). A polymer plugging gel for the fractured strata and its application. Materials 11 (5), 856. doi:10.3390/ma11050856
Gregolin, M. T., Chiari, B. G., Ribeiro, H. M., and Isaac, V. L. B. (2010). Rheological characterization of hydrophylic gels. J. dispersion Sci. Technol. 31 (6), 820–825. doi:10.1080/01932690903333655
Guo, X., Deng, Z., Li, Z., Zhang, X., Zhang, L., and Zhao, Q. (2016). The effect of different activators on the performance of an inorganic solidifiable gel plugging fluid. J. Nat. Gas Sci. Eng. 34, 34253–34264. doi:10.1016/j.jngse.2016.05.045
Jianshan, L. (2019). Drilling fluid technology for borehole wall stabilization and mud loss control in block Hangjinqi. Drill. FLUID & Complet. FLUID 36 (03), 308–314.
Kumar, L., Lawrence, C., and Sjöblom, J. (2014). Mechanism of pressure propagation and weakly compressible homogeneous and heterogeneous thixotropic gel breakage to study flow restart. RSC Adv. 4 (52), 27493–27501. doi:10.1039/c4ra02753a
Li, X., Fu, M., Liu, J., Xiao, Q., Tang, W., and Yang, G. (2022). Synthesis and performance evaluation of a novel heat and salt-resistant gel plugging agent. Polymers 14 (18), 3894. doi:10.3390/polym14183894
Li, Z., Deng, Z., Guo, X., Zhang, L., and Zhi, Y. (2015). Study of the impact of solidifier inorganic components on the performance of a solidifiable gel plugging fluid. J. Nat. Gas Sci. Eng. 23, 23450–23457. doi:10.1016/j.jngse.2015.02.010
Li, Z., and Xiang, C. (2020). Experimental investigation of a new weak-gel-type clay-free and water-based drilling fluid with high-temperature and high-salt resistance for determining its optimized formulation. Energy Sources Part A Recovery Util. Environ. Eff. 107, 1–18. doi:10.1080/15567036.2020.1763516
Li, Z., Zhao, G., and Xiang, C. (2022). Synthesis and properties of a gel agent with a high salt resistance for use in weak-gel-type water-based drilling fluid. Arab. J. Sci. Eng. 47, 12045–12055. doi:10.1007/s13369-022-06826-1
Liu, S., Zhang, H., Qiu, X., Fang, B., Lu, Y., Zhai, W., et al. (2018). Rheokinetic study of gel-breaking process of xanthan gum solution. J. dispersion Sci. Technol. 39 (2), 270–274. doi:10.1080/01932691.2017.1312433
Quiroz, A., Chavira, E., Palomino-Merino, R., Guzmán, J., and Flores, C. (2022). Polymorphic synthesis and structural characterization of NaSbO3 prepared by sol–gel acrylamide polymerization. J. Solid State Chem. 315, 315123478. doi:10.1016/j.jssc.2022.123478
Suming, L., Xiangdong, L., Guangchang, M., Wei, S., and Youcheng, Z. (2007). Research and evaluation of new anti-high pressure plugging agent. Drill. FLUID & Complet. FLUID 04, 24–27+93. doi:10.2112/SI83-011.1
Wang, G., Fan, H., Jiang, G., Li, W., Ye, Y., Liu, J., et al. (2020). Rheology and fluid loss of a polyacrylamide-based micro-gel particles in a water-based drilling fluid. Mat. express 10 (5), 657–662. doi:10.1166/mex.2020.1687
Yokoi, T., Kuzuya, A., Nakajima, T., Kurokawa, T., Gong, J. P., and Ohya, Y. (2022). Synthesis of degradable double network gels using a hydrolysable cross-linker. Polym. Chem. 13, 3756–3762. doi:10.1039/D2PY00360K
Zhao, W., Liu, H., Wang, J., Zhang, H., Yao, C., Wang, L., et al. (2018). Investigation of restarting pressure gradient for preformed particle gel passing through pore-throat. J. Petroleum Sci. Eng. 168, 16872–16880. doi:10.1016/j.petrol.2018.05.005
Keywords: gel synthesis, delay, anti-high temperature gel, malignant leakage plugging, drilling engineering
Citation: Bai Y, Wu L, Luo P and Li D (2022) Synthesis and evaluation of delayed anti-high temperature gel plugging agent. Front. Energy Res. 10:1003473. doi: 10.3389/fenrg.2022.1003473
Received: 26 July 2022; Accepted: 31 October 2022;
Published: 14 November 2022.
Edited by:
Xianbin Huang, China University of Petroleum (East China), ChinaReviewed by:
Hanyi Zhong, China University of Petroleum, Huadong, ChinaHui Mao, Chengdu University of Technology, China
Yb He, China University of Petroleum, China
Copyright © 2022 Bai, Wu, Luo and Li. This is an open-access article distributed under the terms of the Creative Commons Attribution License (CC BY). The use, distribution or reproduction in other forums is permitted, provided the original author(s) and the copyright owner(s) are credited and that the original publication in this journal is cited, in accordance with accepted academic practice. No use, distribution or reproduction is permitted which does not comply with these terms.
*Correspondence: Yang Bai, YmFpeWFuZ2h5cUBmb3htYWlsLmNvbQ==