- Science and Technology on Reactor System Design Technology Laboratory, Nuclear Power Institute of China, Chengdu, China
The fast reactor is gradually being paid attention to in nuclear power due to a better breeding effect, and its economy is also directly followed. Due to the restriction of the core power density, the core power level is directly related to the volume of the core active area. When the power level and the volume of the core gradually increase, the mass of uranium and 235U in the core at the beginning of life will gradually increase on the basis of a critical uranium inventory. At the same time, due to the gradual increase in the breeding effect, the utilization rate of uranium resources at the end of life will gradually increase. Due to the characteristics of the large critical uranium mass and high breeding effect, the mass of uranium and 235U in the low power level core at the beginning of life cannot be further reduced because of the critical uranium inventory. The lifetime of the high power level core should be extended to use the wealthy reactivity of the core because of the improvement in the breeding effect. However, due to the requirements of the nuclear power refuel time and the limitation to fuel consumption, the breeding effect brought by the improvement in the core power level and the size of the active zone may be less effective. In this article, the power level and economy of lead-based modular nuclear power are analyzed, and the economy of different power level fast reactors with 2,000 EFPD lifetime such as 100 MWt, 300 MWt, 500 MWt, 700 MWt, and 1000 MWt thermal power is designed and analyzed. By analyzing the invention of uranium and 235U and the fuel utilization of different core schemes, the best economic analysis of the single reactor power of the current lead-based modular reactor is proposed and the foundation of the lead-based modular nuclear power is provided.
Introduction
As one of the fourth-generation nuclear energy system reactor types, the lead-based fast reactor is economical, secure, and sustainable. A lead-based coolant not only has the characteristics of high thermal conductivity in metals and the boiling point being higher than 1,000° under normal pressure but is also more chemically inert than some other lively metals such as sodium, and the lead-based coolant would hardly incur chemical reactions with water and air. The lead-based coolant can not only improve the inherent safety of the reactor core but can also use two circuit designs of the reactor system, so the lead coolant and water directly use the steam generator to use heat, which greatly improves the economy of the reactor (Zrodnikov et al., 2001; Zrodnikov et al., 2008).
The neutron spectrum of a fast reactor is harder than that of the thermal reactor. Fissile materials such as 235U and 239Pu have large fission cross sections in the thermal neutron zone but small fission cross sections in the fast neutron zone; however, the fertile material such as 238U is different; it has large capture cross sections in the fast neutron zone but relatively small capture cross sections in the thermal neutron zone, and the 238U material will capture fast neutrons and become 239Pu, which is the fissile material. The fast neutron spectrum can use 238U, so it can improve the utilization rate of uranium resources. At the same time, because the thermal neutron fraction of a fast reactor is much lower than that of a thermal reactor, so the critical uranium inventory of the fast reactor is far greater than that of the thermal reactor. Also, the analysis of the economy of the core must not only consider the utilization rate of uranium resources but also the inventory amount of uranium and 235U at the beginning of life.
At present, the fuel types of a lead-based fast reactor are commonly UO2 and U-10Zr. UO2 has rich experience and radiation data in PWR, and U-10Zr has the advantages of having more uranium amount in the unit volume. At the same time, the neutron spectrum of the core loaded with U-10Zr is relatively harder than that of UO2, so the fuel breeding performance is better. Therefore, this article will also analyze the characteristics of the cores loaded with UO2 and U-10Zr fuels at different power levels.
There are many factors that need to be considered in the installation capacity of the core in the nuclear power plant. Among them, from reactor’s physical perspective, the economy about a single core power level should be considered and then, the conceptual design of a modular nuclear power plant is adopted according to multiple cores so as to fully improve its economy.
Based on the characteristics and needs of a lead-based modular nuclear power plant, this article conducts analysis and research on the core power levels and economy. It mainly analyzes the initial uranium inventory of different power levels and fuel utilization rate at the end of life. The characteristics of different power levels of the lead-based fast reactor core design are given, and recommended lead-based modular nuclear power levels are given.
Calculation program
Analyses of fast reactors can be accomplished by either deterministic methods or stochastic methods. Deterministic codes that are established on the neutron transport theory can provide both accurate and efficient solutions to fast reactor problems (Zhang et al., 2022). In addition, the Monte Carlo method is flexible in geometrical modeling and is suited for high-fidelity and accurate computations. The calculation of this article is completed by the reactor Monte Carlo code (RMC) (Wang et al., 2013) program, which was independently developed by the Department of Engineering Physics of Tsinghua University and is a three-dimensional transportation Monte Carlo program used for reactor core calculation analysis. The RMC program is developed in accordance with the basic needs of the reactor calculation analysis, such as complicated geometric structures and a complicated neutron spectrum in combination with the design of the new concept reactor system. Using continuous energy points to describe the complex energy spectrum and materials, it can be calculated based on the actual problems of a multiplier factor and fuel burnup problems.
Research route
In order to analyze the economics of lead-based modular nuclear power levels from the reactor physical perspective, this article will choose a fixed fuel assembly structure type, and five different power levels core schemes, such as 100 MWt, 300 MWt, 500 MWt, 700 MWt,, and 1000 MWt, are designed under the nearly same power density of the core and the lifetime of 2,000 equivalent full power day (EFPD) (Zrodnikov et al., 2009), as shown in Table 1. The lifetime of 2000EFPD refers to the Russian SVBR core (Zrodnikov et al., 2009) and the SLBR-50 core (Zhao et al., 2021) from the Nuclear Power Institute of China (NPIC). The core power is to analyze the economics of the core at different power levels. Therefore, several typical power points from 100 MWt to 1,000 MWt were selected for studies. The reason for choosing UO2 in this paper is its rich operating experience and irradiation data, and the reason for choosing U-10Zr is it has a higher mass percentage of uranium; therefore, it has a higher fuel breeding performance. UN and UC are also compounds of uranium. Their characteristics when used as fuel are similar to UO2 fuel. However, these two fuel types are less used in engineering than UO2. Therefore, this paper only selects UO2 as the representative of ceramic fuel for analysis. The amounts of 235U and uranium of cores with different power levels at the beginning and end of life will be given and analyzed.
Assembly design
The assembly type in the core is referred to as the assembly design of the lead-based research reactor SLBR-50 (Zhao et al., 2021) (small lead-based reactor—50 MWt) of the Nuclear Power Institute of China (NPIC). The assembly diagram is shown in Figure 1, and the main design parameters of the assembly are shown in Table 2.
Core design and calculation analysis
According to the research route and assembly design results, the assembly type selected previously is used for the core arrangement design with different power levels. In order to ensure that the core has a corresponding reactivity control ability, the corresponding control assembly positions are reserved during the core arrangement. In order to simplify the core design, the control rod assembly design is not performed and the core burn-up calculation is performed with all rods out of the core, and at the end of the life, keff of the core is greater than 1.005, which is the standard of judgment during core life. The arrangement diagrams of the 10 schemes are shown in Figures 2–6, and the design parameters of all the schemes are shown in Table 3. Here, the irradiation effect of the U-10Zr alloy is considered in its fuel density. The theoretical density of 75% is used in this paper, and the feasibility of metal fuel irradiation swelling is fully considered. Also, there are two main reasons why the height of the active section of the core is 1 m. One is to better compare the core performance under different core powers and core sizes, and the other is to obtain a relatively short and fat core design. The short and fat core design is beneficial to obtain a negative core feedback coefficient, so the core height should not be too high. To sum up, the core height is set to 1 m in this paper.
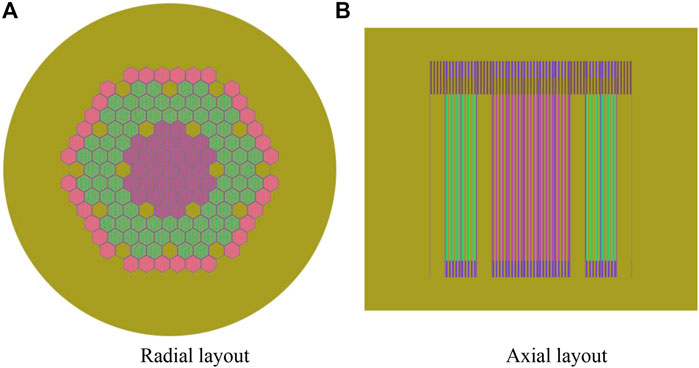
FIGURE 2. 100-MWt core arrangement diagrams of UO2 and U-10Zr: (A) radial layout and (B) axial layout.
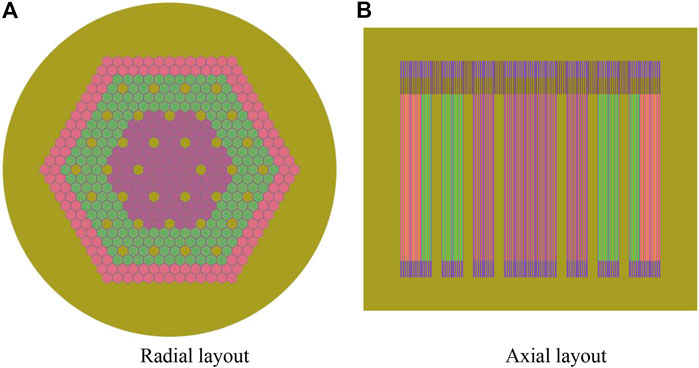
FIGURE 3. 300-MWt core arrangement diagrams of UO2 and U-10Zr: (A) radial layout and (B) axial layout.
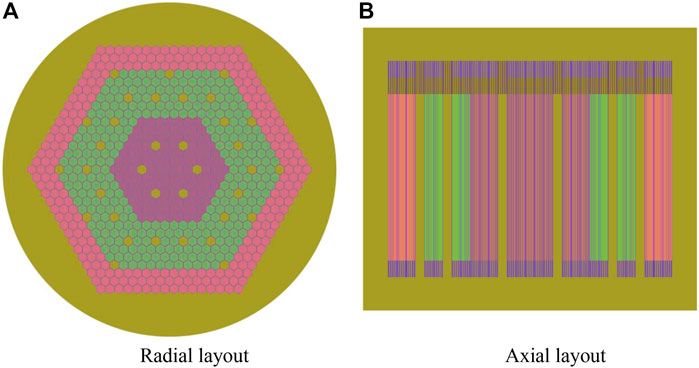
FIGURE 4. 500-MWt core arrangement diagrams of UO2 and U-10Zr: (A) radial layout and (B) axial layout.
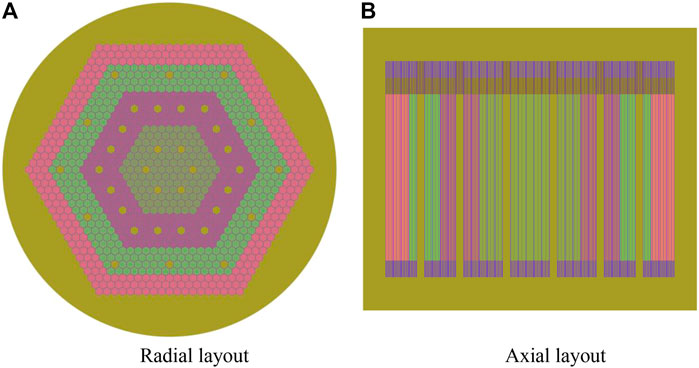
FIGURE 5. 700-MWt core arrangement diagrams of UO2 and U-10Zr: (A) radial layout and (B) axial layout.
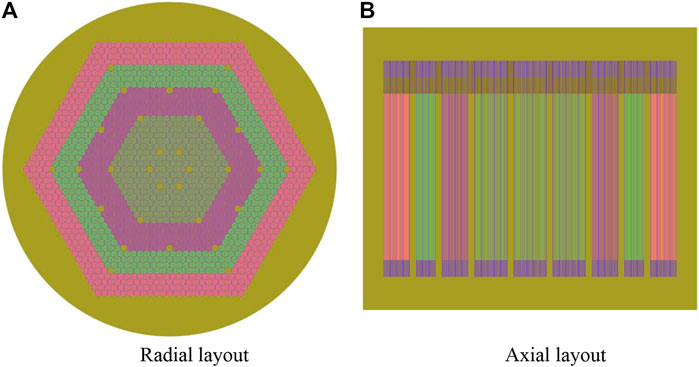
FIGURE 6. 1000-MWt core arrangement diagrams of UO2 and U-10Zr: (A) radial layout and (B) axial layout.
The amount of uranium and 235U of each core scheme changing along the core powers is shown in Figure 7. It can be seen that the amount of uranium and 235U have linear growth characteristics along the core powers. This is because each core scheme maintains a short and fat core design, and the overall leakage rates of the cores are not much different. The critical uranium inventory of each core scheme is basically the same. In order to maintain the energy output of a given power level and core life, they all need to increase the corresponding uranium and 235U amounts on the basis of critical uranium inventory. So the amount of uranium and 235U of all the core schemes with different power levels but the same fuel assembly structure presents a linear growth trend.
Fuel utilization rates of all the schemes are shown in Table 3, from which it can be seen that, with the increase in the power level of the schemes, the fuel utilization rate of the schemes with the same fuel type is linearly increased. This is because the fuel utilization rate is similar to the ratio of fuel consumption and the sum of fuel consumption and critical fuel mass, and with the increase in the power level of the core, the amount of fuel consumption increased during the core life and the critical fuel mass has basically maintained, so the fuel utilization rate is increasing linearly.
Core keff curves of all the schemes with UO2 and U-10Zr fuel along with the core life are shown in Figures 8, 9. The keff curves of all the UO2 schemes have no big difference except for that of the 100 MWt core. This is because all the schemes adopt a short and fat core design, and the ratio of height and diameter of all the schemes is far less than 1 except that of the 100 MWt core is nearly 1. Although the size of the UO2 fuel core increases the fuel utilization rate, the breeding performance has not changed much, so the trends of the keff have basically remained unchanged. The keff cures of U-10Zr schemes show increasing trend along the increases of the core size, such as the cores with 500 MWt, 700 MWt, and 1000 MWt. In the 500 MWt core, keff reaches the maximum value at 1,000 EFPD, and then, it gradually decreases along the increasing fuel burn-up, and at the end of life, keff is basically the same as the beginning of life. In the 700 MWt and 1,000 MWt cores, keff increases from the beginning of life and does not reach the maximum value at 2,000 EFPD. From the perspective of evaluating the reactivity of the core, it can be seen that the core has not reached the end of life, and it is estimated that it can also operate more than 2,000 EFPD or more, and the life period will reach 4,000 EFPD or longer.
The main reason why the breeding performance of U-10Zr is greater than that of UO2 is that U-10Zr fuel has a larger proportion of uranium per unit volume than UO2, and at the same time, there is no oxygen element in metal fuel and the energy spectrum is harder. All these reasons make the breeding performance of U-10Zr fuel stronger.
It can be seen by comparing the core schemes with UO2 and U-10Zr fuels at the same power level that, at low power levels, because the total energy output of the core is relatively small, the size of the core and the amount of uranium are relatively small, which saves uranium resources and more economical.
The breeding performance and the fuel utilization rate of the UO2 core will gradually enhance with the increase in the core power level and core size, so the high power level core, such as 700 MWt and 1,000 MWt, is more suitable for loading with the UO2 fuel.
The breeding performance of the U-10Zr core is far higher than that of the UO2 fuel core with the increase in the core power level and core size. For example, keff of the 700 MWt core shows the upward trend, and the core life period of 2,000 EFPD is not long enough to release the breeding capacity of the core. Therefore, it is necessary to appropriately reduce the power level and size of the core to make the breeding effect of the core match the core life period. So uranium fuel is suitable for the core of the 500 MWt class power level.
The utilization rates of 235U and 238U along with the core power level are shown in Figures 10, 11. It can be seen that the core fuel utilization rate gradually increases with the increase in core power, but with the increase in the core power level to a certain extent and the core size being increased to a certain degree, the increase rate of the core fuel utilization rate will gradually become gentle. At the same time, the fuel utilization rate of the UO2 core is generally slightly lower than that of the U-10Zr fuel core.
It should be noted that the main reason for pursuing an approximate level of the reactivity curve of the modular core is to reduce the difficulty of reactivity control. In a fast reactor, the reactivity value of a single control rod is generally small in order to avoid a prompt supercritical accident, and the low residual reactivity of the core can be used to reduce the number of control rods in the core. On the other hand, the flatter reactivity curve of the core means that the reactivity loss during the core burn-up matches with the reactivity increase in the breeding so that the breeding performance of the core can be more fully utilized.
Conclusion
This article is based on the same fuel assembly structure type to design core schemes with different power levels and different fuel types and analyzes the amount of uranium and 235U of each core scheme and the fuel utilization rate at the end of life. The following conclusion can be obtained:
(1) In the case of maintaining the leakage and life of the core, the amount of uranium linearly increases with the power level of the core, and the fuel utilization rate increases with the increase in the power level and size of the core.
(2) The UO2 fuel type is more suitable for the core arrangement of the low power level such as 100 MWt and high power level such as 1000 MWt. As in the low power level core, the amount of uranium with UO2 fuel is less, and in the high power level core, the core breeding performance matches the core energy output, which is more suitable for the core reactivity control.
(3) The U-10Zr fuel type is more suitable for the core arrangement of the middle power level such as 500 MWt. As in the middle power level core, the core breeding performance nearly matches the core energy output, which can give full play to the breeding performance of U-10Zr fuel.
The aforementioned conclusion is applicable to the power density, life period, and the ratio of grid distance and rod diameter specified in this article. If the aforementioned parameters change, the core power level of different fuel types will be appropriately shifted, but the basic laws will be constant and appropriate.
Data availability statement
The raw data supporting the conclusion of this article will be made available by the authors, without undue reservation.
Author contributions
LL: conceptualization, methodology, and software. WL: conceptualization. ZuB: methodology and conceptualization. ZCh: methodology and conceptualization. ZgB: visualization and investigation. YM: funding acquisition and conceptualization. ZCe: funding acquisition and supervision. XH: funding acquisition and supervision. CY: methodology and visualization. WX: visualization and software. ZZ: funding acquisition and supervision. ZN: funding acquisition and supervision. LJ: funding acquisition and supervision.
Conflict of interest
The authors declare that the research was conducted in the absence of any commercial or financial relationships that could be construed as a potential conflict of interest.
Publisher’s note
All claims expressed in this article are solely those of the authors and do not necessarily represent those of their affiliated organizations, or those of the publisher, the editors, and the reviewers. Any product that may be evaluated in this article, or claim that may be made by its manufacturer, is not guaranteed or endorsed by the publisher.
References
Wang, K, Li, Z G, She, D, Liang, J., Xu, Q., Qiu, A., et al. (2013). RMC-A Monte Carlo code for reactor physics analysis [C], Joint International Conference on Supercomputing in Nuclear Applications and Monte Carlo. Paris: France.
Zrodnikov, A. V., Toshinskii, G. I., Grigor'ev, O. G., Dragunov, Y. G., Stepanov, V. S., Klimov, N. N., et al. (2001). SVBR-75/100 multipurpose modular low-power fast reactor with lead–bismuth coolant. At. Energy 91 (6), 528–533. doi:10.1023/b:aten.0000047677.16291.f2
Zrodnikov, A.V., Toshinsky, G.I., Komlev, O.G., Stepanov, V.S., Klimov, N.N., Kudryavtseva, A.V., et al. (2009, Fuel Cycle of Reactor SVBR-100 [J], Proceedings of GlobalSeptember, May 2009).. Paris, France. 6-11.
Zrodnikov, A.V., Toshinsky, G., Komlev, O., Dragunov, Y., Stepanov, V., Klimov, N., et al. (2008). Innovative nuclear technology based on modular multi-purpose lead–bismuth cooled fast reactors. Progress in Nuclear Energy 50, 170–178. doi:10.1016/j.pnucene.2007.10.025
Zhao, C, Lou, L., Peng, X., Zhang, B., and Wang, L. (2021). Application of the Spectral-Shift Effect in the Small Lead-Based Reactor SLBR-50. Front. Energy Res. 9, 756106. doi:10.3389/fenrg.2021.756106
Keywords: lead-based modular reactor, power level, economy, proliferation effect, fuel utilization
Citation: Lei L, Lianjie W, Bingyan Z, Chen Z, Bin Z, Mingyu Y, Ce Z, Hongzhi X, Yun C, Xingbo W, Zifan Z, Nan Z and Jiayi L (2022) Research and analysis of the effect between the power level and economy on the lead-based modular nuclear power reactor. Front. Energy Res. 10:1002077. doi: 10.3389/fenrg.2022.1002077
Received: 24 July 2022; Accepted: 24 October 2022;
Published: 08 November 2022.
Edited by:
Jingang Liang, Tsinghua University, ChinaReviewed by:
Tengfei Zhang, Shanghai Jiao Tong University, ChinaQian Zhang, Harbin Engineering University, China
Copyright © 2022 Lei, Lianjie, Bingyan, Chen, Bin, Mingyu, Ce, Hongzhi, Yun, Xingbo, Zifan, Nan and Jiayi. This is an open-access article distributed under the terms of the Creative Commons Attribution License (CC BY). The use, distribution or reproduction in other forums is permitted, provided the original author(s) and the copyright owner(s) are credited and that the original publication in this journal is cited, in accordance with accepted academic practice. No use, distribution or reproduction is permitted which does not comply with these terms.
*Correspondence: Lou Lei, 371682011@qq.com; Wang Lianjie, mcd2264@126.com