- 1Faculty of Chemical Engineering, Kunming University of Science and Technology, Kunming, China
- 2School of Mechanical Engineering and Automation, Harbin Institute of Technology, Shenzhen, China
- 3Academy of Building Energy Efficiency, School of Civil Engineering, Guangzhou University, Guangzhou, China
With the increasing of global energy requirements and environmental problems, the use of solar thermal energy has attracted widespread attention. The selective solar absorption coating is the most important part of a solar thermal conversion device. At present, most of the coatings work well in a vacuum at a high temperature, while not stably in the air environment. Based on the high-temperature resistant and infrared-reflective properties of ITO, a multilayer film of SiO2/Si3N4/SiO2/ITO/Cr has been designed as a selective solar absorber. The genetic algorithm is applied to optimize the material and thickness selection for each layer. The results show that the optimized multilayer film could achieve a high solar absorptance up to 90% while keeping a relatively low infrared emittance around 50% for temperature change between 600°C and 900°C. All the materials composing this film have been tested before to be chemically stable at a high temperature up to 900°C in the air environment. It is also adaptive to different incident angles from 0° to 60°. The finite-difference time-domain method was also adopted to plot the energy density distribution for different wavelengths, which provided the underlying mechanism for the selective emission spectrum. The findings in this study would provide valuable guidance to design a low-cost selective solar absorption coating without the need for vacuum generation.
Introduction
Compared with traditional fossil energy, solar energy has the advantages of abundant stock, clean use, and direct development and utilization (Modi et al., 2017; Sansaniwal et al., 2018). The representative application of solar energy fields including solar water heating system, refrigeration, seawater desalination (Choi, 2017; Li et al., 2018), solar industrial heat (Geete, 2020; Kumar and Mylsamy, 2020) and concentrated thermal power generation (Cojocaru et al., 2020; Teffah and Zhang, 2017; Caccia et al., 2018; Wilberforce et al., 2019), which have formed an all-round utilization at low, medium and high temperatures. Especially, solar thermal electricity (STE) has become a driving force that cannot be ignored for “carbon peak” and “carbon neutrality”. There are still many urgent problems to be solved in the demonstration projects (Geete, 2020). The solar selective absorption coating (Seraphin, 1976; Craighead and Buhrman, 1977) is the cornerstone of solar photothermal utilization and the guarantee for efficient collection of solar energy to maintain good optical efficiency. Scientific researchers have conducted extensive research on selective absorption coatings (Cao et al., 2017; Gao et al., 2019). The materials for absorption coatings are mainly composite materials composed of transition metals such as Co, Ti, Cr, Ni, and their compounds. The structures mainly include intrinsic absorption type, surface texture type, multilayer gradient type, cermet composite type, etc. Each structure has some shortcomings, so the current coating structure is composed of a variety of structures. The typical solar spectrum selective absorption coating mainly has three layers, the base layer, the intermediate absorption layer (single layer or multi-layer composite structure) and the anti-reflection layer.
After decades of research, the absorption rate of the coating currently working in the medium and low-temperature environment can reach about 0.95, while the reflectivity is only 0.04, for which the comprehensive performance and stability have reached an ideal level (Baneshi et al., 2012; Grosjean et al., 2018; Long et al., 2019). A typical product is the super blue film-TiNOX produced by German Almeco Company (Almeco-TiNOX., 2013), which is based on SiO2-TiNxOy-Cu cermet coating with an absorption rate of 0.95 and reflectivity of 0.04 (100°C). Bayón et al. (Bayón et al., 2010) prepared Al/CuMnOx/SiO2 absorption coating by sol-gel extraction method, and the absorption rate of the coating is as high as 0.95. The absorption rate and reflectance of the large area (30 cm × 30 cm) coating are 0.935 and 0.04 respectively, and the film system has good thermal stability and humidity stability after heating at 250°C for 200 h.
However, with the promotion of solar high-temperature heat utilization, the working temperature of the coating has been greatly increased. According to Planck’s Law of black body radiation, the radiation heat loss of the coating itself has also increased significantly, resulting in a decrease in the thermal performance of the system. In order to reduce the self-emission heat loss, its emissivity should be reduced. In addition, when the coating works in a high-temperature environment, the comprised materials would be oxidized in air environment, resulting in worse performance in thermal stability, and be suggested to only work in a vacuum environment. For this reason, the cost of the coating working at high-temperature would be greatly raised. This problem may also exist for medium-temperature heat utilization, because the temperature of the concentrated solar absorber after photothermal conversion is usually much higher than that of the working medium (Wang et al., 2020a; Wang et al., 2020b), and the coating would still reach the high-temperature working temperature zone. Therefore, in the medium and high-temperature solar heat utilization, it is necessary to further improve the high-temperature stability of the coating and maintain low emissivity and high absorptivity.
In the coating industry, many studies have been carried out to enhance the high-temperature coating performance, but most of them stay in the vacuum environment. Wang et al. (Xinkang et al., 2008) prepared a double-layer gradient Mo-Al2O3 spectral coating on stainless steel (SS) substrate. Under the high-temperature vacuum environment of 350∼1,000°C, the absorption rate can reach 0.91∼0.93 and the thermal emissivity is 0.19∼0.27. After annealing the coating for 2∼5 h, the coating can still maintain good optical properties, but with the extension of annealing time and the increase of annealing temperature, the absorption coating has serious cracking and falling off, which indicates that the thermal stability of the coating needs to be improved. Wang et al. (Wang et al., 2017) proposed the alloying of W-NPs and Ti to inhibit the thermal diffusion of W atoms and the agglomeration of nanoparticles (NPs) in the cermet membrane, which significantly improved the thermal stability of the WTi-Al2O3 cermet membrane. Based on the WTi-Al2O3 cermet with good performance, a solar selective absorption coating with SS substrate / W infrared reflection layer / ultra-thin Al2O3 layer / WTi-Al2O3 HMVF (high metal volume fraction) layer / W-Al2O3 LMVF (low metal volume fraction) layer / Al2O3 anti-reflection layer structure is also developed. After annealing at 600°C in a vacuum for 840 h, it shows a high solar absorptivity of about 0.93 and a thermal emissivity as low as 0.1 at 500°C. A. Antonaia and S. Esposito et al. (Antonaia et al., 2010) fabricated the W/W-Al2O3/Al2O3 coating with stainless steel (SS) as the substrate from the magnetron sputtering technology, of which W, W-Al2O3 and Al2O3 was the infrared reflection, light absorption and anti-reflection layer, respectively. Under the high-temperature vacuum environment of 580°C, the light absorption rate of the coating reached 0.94 and the emissivity reached 0.04. Guo et al. (Guo, 2016) used the magnetron sputtering technology to deposit a layer of metal Mo as the light absorption layer of the absorption coating on the SS substrate, and continued to deposit a layer of Al2O3 as the anti-reflection layer. The light absorption rate of the coating at room temperature reached 0.90, and the thermal emissivity was only 0.08. After annealing in a high-temperature vacuum environment at 600°C, the absorption coating can still maintain good optical properties. Ma et al. (Ma, 2012) used electrochemical copper plating technology to prepare a Mo-Cu composite on the surface of nano Mo powder. The composite was used as the absorption layer, and then the Al2O3 and Mo-Cu composite powder were prepared to be suitable for plasma spraying micron-sized composite powder, and finally, a coating with a thickness of about 2.5 μm was sprayed on the pre-polished copper substrate by plasma spraying method. The thermal stability test results show that, when the annealing temperature is lower than 600°C, the light absorption rate (0.868) and thermal emissivity (0.347) of the coating has no obvious change, and it has good thermal stability at 900°C, but the optical performance itself still has great potential for improvement.
It can be concluded that the optical efficiency and thermal stability of a selective absorption coating are the most important properties for high-temperature applications. However, most of the coatings are still tested in the vacuum environment, and they will face a bigger challenge when applied in an air environment due to the possible oxidization and cracking. The present study aims at designing a coating with desired optical selectivity and chemical stability at high temperatures and in the air environment. Although the present work is a theoretical one, the materials composing the coating, which is a multilayer film, have already been experimentally tested to be chemically stable before for the conditions of high temperature and air environment. As a result, combined with the genetic algorithm and transfer matrix method, four kinds of common high-temperature resistant materials, i.e., SiO2, Si3N4, ITO, and Cr, are selected to compose a five-layer membrane. The designed multilayer structure has optimal spectral selectivity with a high solar absorptance and a relatively low infrared emittance at high-temperature ambient conditions. The proposed design method and obtained results in the present study could provide valuable guidance for the coating design of solar applications.
Results and Discussion
Optimized Structural Design of Multilayer Films
Wang et al. (Wang et al., 2018) of Lawrence Berkeley National Laboratory designed a coating structure made of a three-layer film structure formed by alternating deposition of Cr, ITO, and SiO2, and the structure can maintain pretty good thermal stability for 120 h in high-temperature air up to 900°C. The absorption rate of the coating in the solar spectrum can reach above 0.9, but its emissivity in the infrared spectrum is also beyond 0.6. The overall performance still has a large room for improvement. The relatively high infrared reflectivity and outstanding thermal stabilitity in air of the material ITO provides us a baseline to design a selective solar absorber.
Figure 1 presents a schematic diagram of the multi-layer thin film structure proposed in present study. The coating is a multilayer film structure formed by the alternating deposition of Cr, ITO, SiO2, and Si3N4. The main function of Cr is to improve the solar absorption, and that of ITO is to provide high infrared reflectance. The additional dielectric layers are combined to achieve anti-reflection and spectrum selectivity. Figure 1B shows the black body radiation energy and the solar radiation curve. It can be seen from the figure that the black body radiation intensity is higher than the solar radiation intensity above 1.5 μm. Therefore, in order to take into account both the solar absorption and thermal emission, and to ensure the absorbed net energy as much as possible, the research goal is to reduce its emissivity above 1.5 μm. The calculated spectral reflectivities of the proposed five-layer film and the reference structure of three layers are presented in Figure 1C as well. The ideal spectral reflectivity curve indicates that it should have zero reflectivity below 1.5 μm and unity beyond 1.5 μm. Both the reference structure and optimized one could get pretty low reflectivity, which is generally below 0.2. However, the optimized structure has a better performance in the infrared range with a higher infrared reflectivity, especially in the wavelength range of 1.5–5 μm where most of the thermal emission is focused at a high temperature of 600 ∼ 900°C.
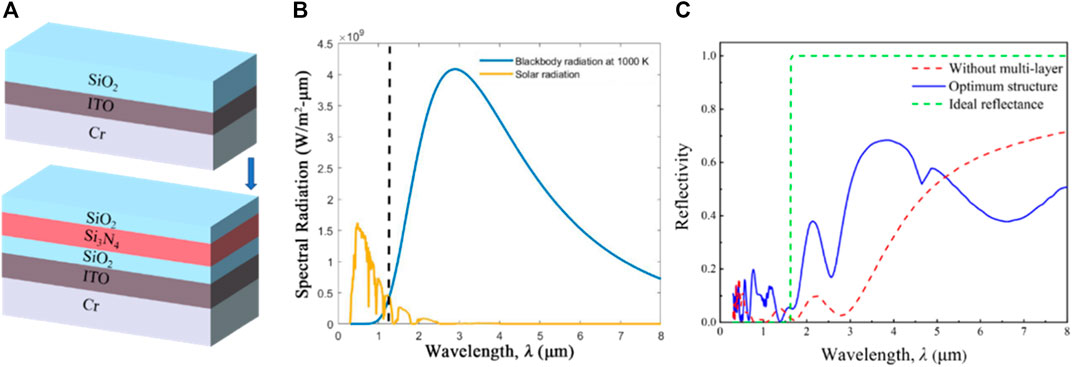
FIGURE 1. (A) Schematic diagram of the multilayer film structure formed by alternate deposition of Cr, ITO, SiO2, and Si3N4. (B) Solar and blackbody spectral radiation. (C) Spectral reflectance of the base structure, optimized and ideal one.
Effect of Incident Angle on Spectral Emissivity
The effect of incident angle on the spectral emissivity of the proposed multilayer film is also investigated. As shown in Figure 2, when the incident angle increases from 0 to 60°, the spectral emissivity cuve hardly changes, which is advantageous especially in energy related applications. As the incident angle increases above 60°, the spectral emissivity in the spectral range of 0.3∼1.5 μm only changes very slightly, while there is a relatively obvious increase of the spectral emissivity in the spectral range of 1.5∼5 μm. This is possibly due to large phase differences at a large incident angle.
Optimal Design of Thin Films Based on Genetic Algorithms
To obtain the best spectrum selectivity, the genetic algorithm (Shi et al., 2017) is used to optimize the overall parameters of the multilayer film. The optimization process includes determining the material used, the number of layers required, and the thickness of each layer. Figure 3 shows the optimization process combining the genetic algorithm and the framework of transfer matrix theory, which is applied to calculate the optical properties of a multilayer film. Following this genetic algorithm, it is necessary to define a value function of F (n, d) at first, which represents the residual between the emissivity spectrum of each case and the target spectrum, and the optimization goal is minimizing this function.
Where n represents the number of layers of the film, d represents the thickness of each layer of film, θ is the incident angle, λ is the wavelength,
The directional emissivity and spectral emissivity of the multilayer films were calculated by the transfer matrix method. At the beginning of the genetic algorithm optimization process, a total of 61 five layers film structures formed by the random arrangement of Cr, ITO, SiO2, and Si3N4 were generated, the emissivity of the thin film in the wavelength range of 0.3∼12 μm was calculated by transfer matrix method. Through genetic algorithm optimization, the nth generation is optimized on the basis of the calculated emission degree, and the n+1-th generation is obtained. In the (n+1) generation, the best 90% of the value function of the n generation is maintained. The convergence criterion is that the optimal emissivity curve of each generation does not change within 8 generations. After optimization calculation, the optimized multilayer film structure is obtained, and its parameters are shown in Table 1.

TABLE 1. The material selection and thickness setup assigned for each layer of the proposed film after genetic algorithm optimization process.
In order to evaluate the overall performance of the proposed solar absorber before and after optimizaiton, the parameters of total solar absorptance α, thermal emittance ε and quality factor Q are defined as follows (Zhang et al., 2017):
Here λ1, λ2 are 0.3 and 5 μm, respectively. Esol(λ) is the spectral solar radiation, R(λ) is the spectral reflectivity of the film. The total thermal emittance is expressed as (Zhang et al., 2017):
where Eb (T, λ) is the spectral blackbody radiation at a wavelength λ and a temperature T. Moreover, the quality factor is calcuated as (Zhang et al., 2017):
The physical meaning of the quality factor represents the absorbed energy efficiency of the film, and the ultimate goal of the optimization process is to obtain a largest Q. Note that a high-temperature condition with 600°C–900°C is consided when comparing the performance of each film.
As shown in Figure 4, as the temperature rises, both the quality factors before and after optimization tend to decrease, which is because of the blueshift of the radiant energy of the film as its temperature increases. The shorter wavelength corresponds to a smaller reflectivity, which would increase the total thermal emittance and decrease the quality factor. However, the quality factor of the proposed film after optimization is always higher than that before optimization at the temperature range of 600°C∼900°C, and the relative improvement is 13∼23%, which is even larger at a higher temperature.
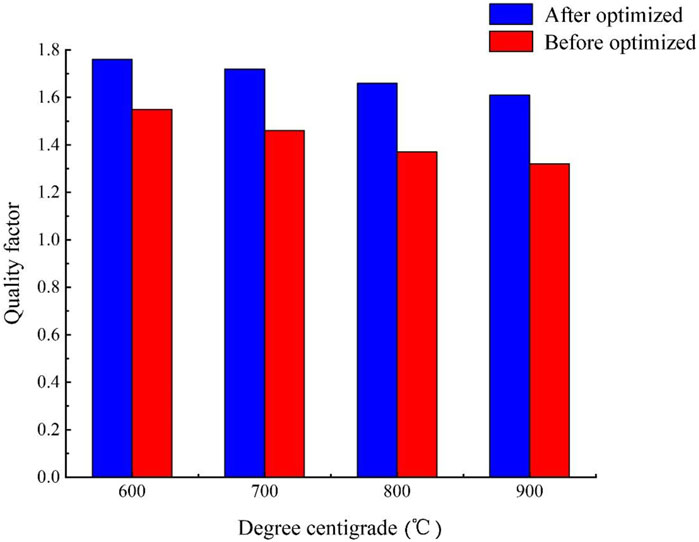
FIGURE 4. The quality factors of the coatings before and after optimization were compared at 600°C∼900°C.
Energy Density Distribution
In order to further understand the underlying mechanism for the spectrum selectivity of the proposed multilayer film, the energy density distributions at different wavelengths are obtained by using the finite difference time domain method (Meagher et al., 2020) to solve the Maxwell equations (Liu et al., 2016) in three-dimensional space. The contour plot of the Poynting vector distribution, which represents the energy transport, are shown in Figure 5 for wavelengths of 1 and 3.57 μm, which exactly correspond to the two wavelength ranges of 0.3∼1.5 μm and 1.5 ∼5 μm, respectively. It is worth noting that the substrate is at the lowest end, and the upper surface of the film is on the top. Due to the interference effect, some of the radiative energy is confined at specific layers. At the wavelength of 1 μm, there is still a large value transmitted to the substrate, which indicates that there is a large part of radiative energy transported into the substrate, which indicates a large energy absorption. For the wavelength of 3.57 μm, most of its energy is limited to the outer surface of the film, which brings a high reflectivity.
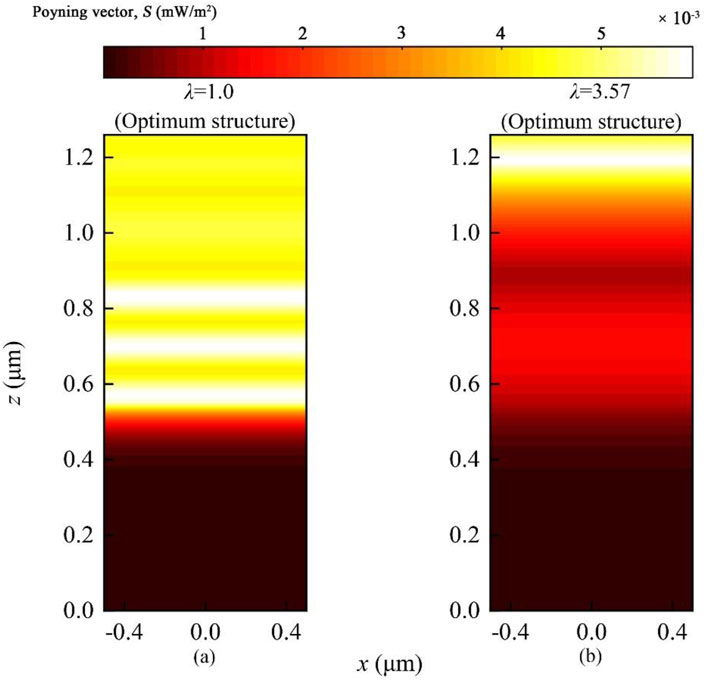
FIGURE 5. The Poynting vector distribution of the proposed multilayer film at different wavelengths of 1 and 3.57 µm.
Conclusion
In summary, a multilayer film of SiO2/Si3N4/SiO2/ITO/Cr is theoretically designed as a selective solar absorber in present study. All the materials composing the film are high-temperature resistant, giving the film great potential to be applied at ambient conditions. Combining the transfer matrix theory and genetic optimization algorithm, the proposed film could occupy an optimal selective emission/absorption with a high solar absorptance up to 90% while keeping a relatively low infrared emittance around 50% for temperature change between 600°C and 900°C. The effect of different incident angle is also explored, and it shows a pretty good consistence when the incident angle increases from 0 to 60 degrees. The energy distribution obtained from the FDTD simulation indicates that the reason for spectrum selectivity comes from the inteference effect between multiple layers. With a relatively simple structure and good spectral selectivity, the proposed selective solar absorber would find great value in solar enegy utilization at ambient conditions.
Data Availability Statement
The original contributions presented in the study are included in the article/Supplementary Material, further inquiries can be directed to the corresponding authors.
Author Contributions
KS: Software, Formal Analysis, Writing-Original Draft HL and LW: Software, Writing-Review and Editing YB: Supervision, Formal Analysis, and Project administration YY: Conceptualization, Supervision, and Funding acquisition.
Funding
This work was supported by the National Natural Science Foundation, China (No. 51866005), and Science, Technology and Innovation Commission of Shenzhen Municipality under a college supporting project under grant number of GXWD20201230155427003-20200731100557008 and Guangdong Science and Technology Program (Nos 2021A0505030077, 2020A1414010300).
Conflict of Interest
The authors declare that the research was conducted in the absence of any commercial or financial relationships that could be construed as a potential conflict of interest.
Publisher’s Note
All claims expressed in this article are solely those of the authors and do not necessarily represent those of their affiliated organizations, or those of the publisher, the editors and the reviewers. Any product that may be evaluated in this article, or claim that may be made by its manufacturer, is not guaranteed or endorsed by the publisher.
Abbreviations
b, black-body radiation; Esol, spectral solar irradiance; Eb, spectral blackbody irradiance; HMVF, high metal volume fraction; LMVF, low metal volume fraction; NPs, nanoparticles; R, the spectral reflectivity; Sol, solar absorptance; STE, solar thermal electricity; SS, stainless steel; T, temperature, °C; θ, incident angle, °; λ, wavelength, μm; α, absorptivity; ε, emissivity; 1,2, the initial state and the final state.
References
Almeco-TiNOX (2013). Solar Absorber [EB/OL]. Available at: http://www.almecosolar.com/cn/products/solar_absorber (01 09, 2013).
Antonaia, A., Castaldo, A., Addonizio, M. L., and Esposito, S. (2010). Stability of W-Al2O3 Cermet Based Solar Coating for Receiver Tube Operating at High Temperature. Solar Energ. Mater. Solar Cell 94 (10), 1604–1611. doi:10.1016/j.solmat.2010.04.080
Baneshi, M., Maruyama, S., and Komiya, A. (2012). The Effects of TiO2 Pigmented Coatings Characteristics on Temperature and Brightness of a Coated Black Substrate. Solar Energy 86 (1), 200–207. doi:10.1016/j.solener.2011.09.019
Bayón, R., San Vicente, G., and Morales, Á. (2010). Durability Tests and Up-Scaling of Selective Absorbers Based on Copper-Manganese Oxide Deposited by Dip-Coating. Solar Energ. Mater. Solar Cell 94 (6), 998–1004. doi:10.1016/j.solmat.2010.02.006
Caccia, M., Tabandeh-Khorshid, M., Itskos, G., Strayer, A. R., Caldwell, A. S., Pidaparti, S., et al. (2018). Ceramic-metal Composites for Heat Exchangers in Concentrated Solar Power Plants. Nature 562 (7727), 406–409. doi:10.1038/s41586-018-0593-1
Cao, F., Tang, L., Yang, L., and Litvinchuk, A. P. (2017). A High-Temperature Stable Spectrally-Selective Solar Absorber Based on Cermet of Titanium Nitride in SiO2 Deposited on Lanthanum Aluminate. Solar Energ. Mater. Solar Cell 160, 12–17. doi:10.1016/j.solmat.2016.10.012
Choi, S.-H. (2017). Thermal Type Seawater Desalination with Barometric Vacuum and Solar Energy. Energy 141 (PT.1), 1332–1349. doi:10.1016/j.energy.2017.11.007
Cojocaru, E. G., Bravo, J. M., Vasallo, M. J., and Marin, D. (2020). A Binary‐regularization‐based Model Predictive Control Applied to Generation Scheduling in Concentrating Solar Power Plants. Optimal Control. Appl. Methods 41 (1), 215–238. doi:10.1002/oca.2498
Craighead, H. G., and Buhrman, R. A. (1977). Optical Properties of Selectively Absorbing Ni/Al2O3composite Films. Appl. Phys. Lett. 31, 423–425. doi:10.1063/1.89732
Gao, X.-H., Qiu, X.-L., Li, X.-T., Theiss, W., Chen, B.-H., Guo, H.-X., et al. (2019). Structure, thermal Stability and Optical Simulation of ZrB2 Based Spectrally Selective Solar Absorber Coatings. Solar Energ. Mater. Solar Cell 193, 178–183. doi:10.1016/j.solmat.2018.12.040
Geete, A. (2020). Performance Analyses of Coal-Fired thermal Power Plant Using Parabolic Solar Collectors for Feed Water Heaters. Aust. J. Mech. Eng. (4), 1–12. doi:10.1080/14484846.2019.1706226
Grosjean, A., Soum-Glaude, A., Neveu, P., and Thomas, L. (2018). Comprehensive Simulation and Optimization of Porous SiO2 Antireflective Coating to Improve Glass Solar Transmittance for Solar Energy Applications. Solar Energ. Mater. Solar Cell 182, 166–177. doi:10.1016/j.solmat.2018.03.040
Guo, Z. M. (2016). Preparation and Properties of SS/Mo/Al2O3 and SS/W/Al2O3 Solar Spectral Selective Absorbing Coatings for High Temperature Application. Lanzhou: Lanzhou Jiaotong University.
Kumar, P. M., and Mylsamy, K. (2020). A Comprehensive Study on thermal Storage Characteristics of Nano-CeO2 Embedded Phase Change Material and its Influence on the Performance of Evacuated Tube Solar Water Heater. Renew. Energ. 162, 662–676. doi:10.1016/j.renene.2020.08.122
Li, G., Law, W.-L., and Chan, K. C. (2018). Floating, Highly Efficient, and Scalable Graphene Membranes for Seawater Desalination Using Solar Energy. Green. Chem. 20, 3689–3695. doi:10.1039/c8gc01347k
Liu, H., Rondi, L., and Xiao, J. (2016). Mosco Convergence for H(curl) Spaces, Higher Integrability for Maxwell's Equations, and Stability in Direct and Inverse EM Scattering Problems. J. Eur. Math. Soc. 21, 2945–2993. doi:10.4171/jems/895
Long, L., Yang, Y., and Wang, L. (2019). Simultaneously Enhanced Solar Absorption and Radiative Cooling with Thin Silica Micro-grating Coatings for Silicon Solar Cells. Solar Energ. Mater. Solar Cell 197, 19–24. doi:10.1016/j.solmat.2019.04.006
Luque-Raigon, J. M., Halme, J., and Miguez, H. (2014). Fully Stable Numerical Calculations for Finite One-Dimensional Structures: Mapping the Transfer Matrix Method. J. Quantitative Spectrosc. Radiative Transfer 134, 9–20. doi:10.1016/j.jqsrt.2013.10.007
Ma, R. B. (2012). Preparation and Thermal Stability on Non-vacuum Medium-High Temperature Mo-Cu/Al2O3 Solar Absorber Coatings. Lanzhou: Lanzhou University of Technology.
Meagher, T., Jiang, B., and Jiang, P. (2020). An Enhanced Finite Difference Time Domain Method for Two Dimensional Maxwell's Equations. Numer. Methods Partial Differential Equations 36, 1129–1144. doi:10.1002/num.22467
Modi, A., Bu ¨hler, F., Andreasen, J. G., and Haglind, F. (2017). A Review of Solar Energy Based Heat and Power Generation Systems. Renew. Sustain. Energ. Rev 67, 1047e64. doi:10.1016/j.rser.2016.09.075
Sansaniwal, S. K., Sharma, V., and Mathur, J. (2018). Energy and Exergy Analyses of Various Typical Solar Energy Applications: a Comprehensive Review. Renew. Sustain. Energ. Rev 82, 1576e601. doi:10.1016/j.rser.2017.07.003
Seraphin, B. O. (1976). Chemical Vapor Deposition of Thin Semiconductor Films for Solar Energy Conversion. Thin Solid Films 39, 87–94. doi:10.1016/0040-6090(76)90626-x
Shi, Y., Li, W., Raman, A., and Fan, S. (2017). Optimization of Multilayer Optical Films with a Memetic Algorithm and Mixed Integer Programming. ACS Photon. 5 (3), 684–691. doi:10.1021/acsphotonics.7b01136
Teffah, K., and Zhang, Y. (2017). Modeling and Experimental Research of Hybrid PV-Thermoelectric System for High Concentrated Solar Energy Conversion. Solar Energy 157 (nov), 10–19. doi:10.1016/j.solener.2017.08.017
Wang, H., Haechler, I., Kaur, S., Freedman, J., and Prasher, R. (2018). Spectrally Selective Solar Absorber Stable up to 900 °C for 120 H under Ambient Conditions. Solar Energy 174 (NOV), 305–311. doi:10.1016/j.solener.2018.09.009
Wang, K., He, Y., Liu, P., Kan, A., Zheng, Z., Wang, L., et al. (2020). Highly-efficient Nanofluid-Based Direct Absorption Solar Collector Enhanced by Reverse-Irradiation for Medium Temperature Applications. Renew. Energ. 159, 652–662. doi:10.1016/j.renene.2020.05.167
Wang, K., He, Y., Kan, A., Yu, W., Wang, L., Wang, D., et al. (2020). Enhancement of Therminol-Based Nanofluids with Reverse-Irradiation for Medium-Temperature Direct Absorption Solar Collection. Mater. Today Energ. 17, 100480. doi:10.1016/j.mtener.2020.100480
Wang, X., Gao, J., Hu, H., Zhang, H., Liang, L., Javaid, K., et al. (2017). High-temperature Tolerance in WTi-Al 2 O 3 Cermet-Based Solar Selective Absorbing Coatings with Low thermal Emissivity. Nano Energy 37, 232–241. doi:10.1016/j.nanoen.2017.05.036
Wilberforce, T., Baroutaji, A., El Hassan, Z., Thompson, J., Soudan, B., and Olabi, A. G. (2019). Prospects and Challenges of Concentrated Solar Photovoltaics and Enhanced Geothermal Energy Technologies. Sci. Total Environ. 659, 851–861. doi:10.1016/j.scitotenv.2018.12.257
Xinkang, D., Cong, W., Tianmin, W., Long, Z., Buliang, C., and Ning, R. (2008). Microstructure and Spectral Selectivity of Mo-Al2O3 Solar Selective Absorbing Coatings after Annealing. Thin Solid Films 516 (12), 3971–3977. doi:10.1016/j.tsf.2007.07.193
Keywords: multilayer film, selective solar absorber, high temperature resistant, genetic algorithm, solar application
Citation: Shi K, Liu H, Wang L, Bie Y and Yang Y (2021) Theoretical Design of a Multilayer Based Spectrally Selective Solar Absorber Applied Under Ambient Conditions. Front. Energy Res. 9:795261. doi: 10.3389/fenrg.2021.795261
Received: 14 October 2021; Accepted: 29 November 2021;
Published: 14 December 2021.
Edited by:
Yang Li, Hong Kong University of Science and Technology, Hong Kong SAR, ChinaReviewed by:
Muhammad Amjad, University of Engineering and Technology, Lahore, PakistanHaochun Zhang, Harbin Institute of Technology, China
Copyright © 2021 Shi, Liu, Wang, Bie and Yang. This is an open-access article distributed under the terms of the Creative Commons Attribution License (CC BY). The use, distribution or reproduction in other forums is permitted, provided the original author(s) and the copyright owner(s) are credited and that the original publication in this journal is cited, in accordance with accepted academic practice. No use, distribution or reproduction is permitted which does not comply with these terms.
*Correspondence: Yu Bie, YmlleXVAZ3podS5lZHUuY24=; Yue Yang, eWFuZ3l1ZTIwMTdAaGl0LmVkdS5jbg==