- 1School of Electrical and Electronic Engineering, Universiti Sains Malaysia (USM), Penang, Malaysia
- 2Department of Mechanical Engineering, Sharif University of Technology, Tehran, Iran
- 3College of Engineering and Technology, American University of the Middle East, Egaila, Kuwait
- 4Sustainable and Renewable Energy Engineering Department,, University of Sharjah, Sharjah, United Arab Emirates
Organic Rankine Cycles (ORCs) are promising approaches for generating power from medium or low temperature heat sources. In this regard, ORCs can be used to indirectly produce power from solar energy. Due to intermittent nature of solar energy, storage unit should be coupled with solar ORCs to improve the output power and operating hours. In this article, studies on solar ORCs integrated with various types of storage units were reviewed; the main findings of such studies were extracted and provided. Based on the findings, several factors such as the temperature and pressure at the inlet of the turbine, as well as the operating condition affect the performance of solar ORCs with thermal storage unit just like the conventional ORCs. In addition, the optimum size of the storage unit in the solar ORCs was found to depend on the operating condition. From the financial perspective, it can be noted that the storage unit affects the corresponding indicators. Moreover, the improvement rate in the ORCs by applying storage units could be affected by the configuration of the system.
Introduction
Industrialization and population growth are the main drivers of the increase in electricity demand. Regarding the increment in power demand, limitations in the quantity of conventional fossil fuels such as oil, coal, and natural gas have necessitated the search for renewable energy sources for power generation (Amin et al., 2015; Khanlari and Alhuyi Nazari, 2021). For this purpose, hydropower, geothermal, wind, and solar energies are currently the main alternatives (Stark et al., 2015; Singh et al., 2018). Solar energy, with respect to its availability and huge amount, can be harnessed in different regions of the world for clean power production. Different technologies have been developed for power production from solar in indirect or direct modes (Klein et al., 2015; Alizadeh et al., 2020). Generally, photovoltaic cells are employed for direct power generation while thermal configurations are utilized for indirect power production from solar. To generate power indirectly using solar energy, different technologies and cycles can be used (Grange et al., 2014; Ahmadi et al., 2018; Wang et al., 2019); however, Organic Rankine Cycles (ORCs) are one of the most feasible ones due to its ability to produce power using medium or low-temperature heat sources (Castelli et al., 2019; Haghighi et al., 2020).
The operating principles of ORCs are similar to steam Rankine cycles; however, different working fluids are used. In the conventional Rankine cycles, water is used as the working fluid while different organic fluids are applicable in ORCs (Tian and Shu, 2017). The utilization of organic fluids with lower boiling temperatures compared to water makes it possible to generate power from the medium or low temperature heat sources (Tchanche et al., 2009). Consequently, ORCs are suitable choices for power generation with different heat sources, such as geothermal, waste heat of industries, and solar (Stijepovic et al., 2017; Mirzaei et al., 2018). Similar to conventional Rankine cycles, the economic and working performance of the ORCs are affected by different elements, such as the configuration of the system, the operating conditions, and the used equipment (Zarogiannis et al., 2017; Petrollese and Cocco, 2019). For instance, Stanciu et al. (Dragomir-Stanciu et al., 2020) investigated the impact of condensing temperature on the efficiency of a solar ORC system and found that decreases in the temperature of condensing would improve the efficiency. In another work by (Baccioli et al., 2017), an ORC integrated with compound parabolic collectors was investigated; the study found that higher concentration ratios cause increase in specific production. In addition, the type of operating fluid can remarkably influence the output of ORCs (Stijepovic et al., 2017). According to a work done by Desai and Bandyopadhyay (2016), it was concluded that the efficiency and cost of power block were dependent on the working fluid of the cycle.
Despite several benefits of solar energy, its intermittent nature is one of its main disadvantages. To solve this defect, storage units are suggested to be applied. Conventionally, electricity storage technologies are used to overcome this situation by storing the produced power in off-peak conditions or day hours and used in peak or night hours. In addition, thermal storage units can be used to improve the reliability and working hours of solar thermal systems. Thermal energy can be stored in several forms, such as latent heat, sensible heat, and reversible thermochemical reactions (Kalaiselvam and Parameshwaran, 2014; Das et al., 2021). Depending on the desired temperature for storing the thermal energy, the choice of suitable material would be different. In this regard, it is necessary to consider the operating condition and the applications of the discharged thermal energy to have a proper storage. Thermal storage units have a wide range of applications in solar energy systems, such as solar preheaters and desalinations (Faegh and Shafii, 2017). In addition, latent heat thermal storage units have been used for thermal management of the PV cells and have shown efficient performance for this purpose (Salari et al., 2020). Furthermore, thermal storage units would be attractive for solar ORCs to increase the operating hours and the output power.
Despite the existence of some review papers on the working fluids of ORCs, there is no comprehensive review work on the solar ORCs with storage units. The current work focused on the applications of thermal storage units in solar ORCs for the first time. For this purpose, different scientific databases, including GoogleScholar and Scopus were searched for the references related to the subject of this review. Afterwards, their main findings were extracted and provided in this paper to help researchers in the relevant fields in their future works. In the following section, the contents of the reviewed studies were provided and discussed.
Solar Energy for Power Generation
As previously mentioned, solar energy is suitable for both direct and indirect power generation. In this section, the main technologies used for power generation using solar energy are briefly discussed and explained.
PV Cells
For te direct conversion of solar radiation into electricity, PV cells are applied. In the PV cells, special types of semiconductors are employed. The application of solar radiation on the semiconductor provides energy for electron transfer that give rise to electric current (Assad et al., 2021). Crystalline and thin film are the mostly used types of PV technologies; however, there are some other technologies, such as organic cells which have been used in recent years (Assad et al., 2021). The efficiencies of some of the PV cells, which indicates the ratio of the generated electricity to the solar incidence on the cell under standard condition, are provided in Table 1. According to this table, the efficiencies of the PV cells are relatively low, which restricts adequate power generation in small spaces. Hence, concentrators are used to reach higher solar radiation on the surface of the PV cells by employing optical tools and mediums. In this condition, efficient thermal management approaches can be used to prevent efficiency degradation due to temperature increment (Wang et al., 2020; Wu et al., 2020). From the economic point of view, the feasibility of the solar cells for power generation has improved in recent years. According to the report of the International Renewable Energy Agency (IRENA), the cost of utility-scale electricity generation by PV cells decreased by 85% between 2010 and 2020 (International Renewable Energy Agency IRENA, 2021). This report suggests that over 707 GW of PV systems had been installed by the end of 2020, meaning that more than 16-fold growth has been achieved since 2010.
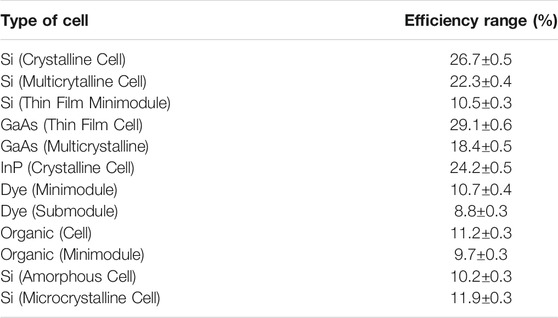
TABLE 1. The efficiencies of different types of solar cells (Green et al., 2019).
Solar Thermal Power Plants
In addition to direct power generation from solar energy, electricity can be produced indirectly by using solar thermal systems. In these technologies, thermal energy of the Sun is used as the heat source to drive thermal power plants. To increase the generated power in a constant space, solar concentrators must be employed similar to concentrated PV systems. In general, there are three kinds of concentrated solar power systems, which are linear parabolic collectors, parabolic dish collectors, and solar towers. Linear parabolic collectors are composed of a linear concentrator with a parabolic cross-sectional geometry. The surface of the concentrator follows the Sun path on a single axis (Assad et al., 2021). This type of concentrator is fixed on a structure and is appropriate for unfavorable operating conditions, such as windy weather. The received sunlight by these systems is concentrated on a tube located along the focal point. The working fluid inside the tube receives heat which increases its temperature and internal energy. In parabolic dishes, reflecting panels are used to follow the path of Sun through rotation around two axes. Sunlight is concentrated on the receiver and installed on the focal point. Using these systems, thermal energy in high temperature condition is transferred to the applied working fluid. In solar towers, heliostats, which are flat-surface reflecting panels, are applied to concentrate the sunlight. The used panels rotate on two axes and concentrate the received solar irradiance on a receiver installed at the top of tower in the central region of the systems. Similar to the previous systems, the concentrated solar radiation increases the temperature of the fluid inside the receiver (Assad et al., 2021). Concentrated solar power plant’s total installation cost reduced by 50% between 2010 and 2020 (International Renewable Energy Agency IRENA, 2021). Different working fluids, such as Brayton, ORC, and supercritical CO2 (Yan et al., 2011; Sánchez-Orgaz et al., 2015; Gao et al., 2019), can be applied on the collected thermal energy for power generation. According to the data provided by the International Energy Agency, the global concentrated solar power generation in 2019 was 15.6 TWh and has been predicted to reach 53.8 TWh by the year 2030 (Solar, 2020).
The Operating Principles of Solar ORCs
In this section, the operating principles of the ORCs and the selection of the working fluid were discussed.
Thermodynamic of the ORCs
As shown in Figure 1, the structure of ORCs is similar to the conventional steam Rankine Cycles; the main components of a simple ORC are turbine (expander), evaporator, condenser, and pump. The operating fluid of the cycle is sent to the evaporator using a pump. In this stage, heat is applied to the working fluid to evaporate it at approximately constant pressure. In solar ORCs, the required thermal energy in this stage is supplied by solar energy. In some cases, a superheater is applied to superheat the fluid. Afterwards, the provided saturated or superheated fluid will be expanded in the turbine. Finally, the turbine drives the generator for power generation (Javanshir et al., 2017). In the expander, the pressure and temperature of the operating fluid are decreased; subsequently, the fluid is condensed in the condenser to become saturated or slightly subcooled. Afterwards, the pump is used to increase the fluid pressure and complete the cycle (Javanshir et al., 2017). Adding some components such as regenerator units to the simple ORCs can improve the power output of the systems; however, the cost of the system will be increased (Haghighi et al., 2020). When using regenerative ORCs, a heat exchanger is installed at the outlet of the turbine, which makes it possible to preheat the fluid entering the evaporator by transferring heat from the outlet fluid of the turbine and the fluid entering the evaporator as shown in Figure 2. It should be noted that the selection of the operating fluid plays a key role in the design of solar ORCs. The properties of the working fluid affect the operation range and efficiency of the cycle (Mirzaei et al., 2018).
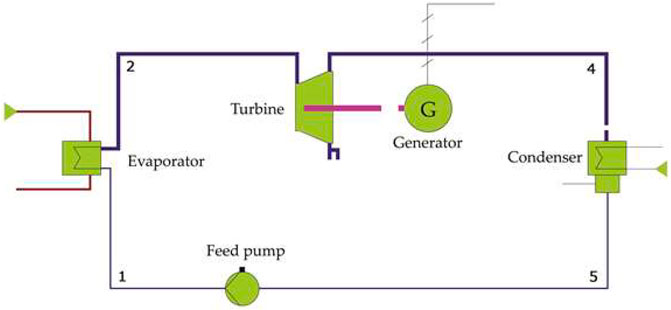
FIGURE 1. Schematic of a simple ORC (Javanshir et al., 2017).
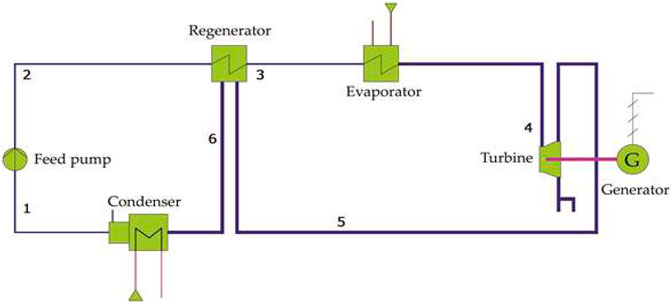
FIGURE 2. Schematic of regenerative ORC (Tchanche et al., 2010).
Despite the similarity in the working principles of ORCs and conventional Rankine cycles, ORCs have some advantages, such as applicability for lower temperatures, lower operation and maintenance costs, no requirement for water, more compact sizes, expanders with lower sizes and higher rotational speeds, quiet working and simple procedures for start and stop (Darvish et al., 2015). The main applications of ORCs are geothermal energy, biomass, heat recovery, and solar (Darvish et al., 2015; Markides, 2015). In addition, ORCs can be integrated with other cycles (Pantaleo et al., 2020) mainly as the bottoming of Brayton cycles to reach higher overall efficiencies and generated power. In this paper, the solar ORCs with thermal storage units are considered.
Working Fluids of ORCs
As noted earlier, the working fluid of the ORCs must have some special properties. Generally, organic fluids are proper choices for power generation from low grade heat sources due to their lower specific vaporization heat compared to water (Kang, 2012). A proper working fluid for these cycles must have appropriate chemical, physical and environmental properties. In addition, it is crucial to be safe and have feasible cost. The specific volume of the fluid should be low (Linke et al., 2015). Low condensation and high vaporization latent heat, in addition to high critical temperature, are among the features of the working fluid that positively affect the performance of ORCs. The fluids with lower vapor density would increase the volumetric flow rate, leading to increase in the pressure drop and expander size (Desai and Bandyopadhyay, 2016). The selection of a proper working fluid for ORC is dependent on the heat source temperature. Table 2 provides the evaporation temperatures of some fluids used in ORCs.
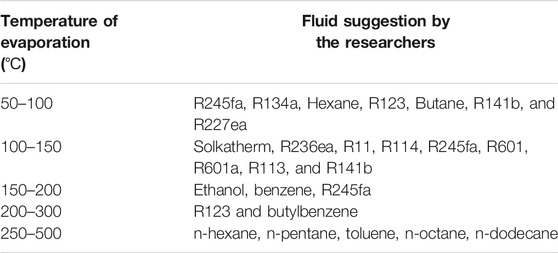
TABLE 2. Selection of the working fluid for ORCs based on the literature (Bao and Zhao, 2013; Thurairaja et al., 2019).
Thermal Storage Units in Solar ORCs
Solar ORCs are categorized into two main groups–direct vapor generation (DVG) and indirect solar ORCs. In DVG types, the intermediate heat exchanger is removed, and solar collectors are directly applied as the evaporator of the cycle. In the conventional types, a heat exchanger is used to transfer the collected solar energy to the operating fluid of the cycle using a heat transfer fluid (Alvi et al., 2021). In both types of solar ORCs, the performance of the system is improvable by utilizing thermal storage unit. Using storage units in the solar ORCs extend the time of power generation; for instance, Manfrida et al. (2016) used phase Change Material (PCM) in solar ORC and found that the proposed configuration can produce power in 78.5% of time with an average efficiency of 13.4% for ORC. Based on these findings, it could be concluded that the addition of a storage unit will extend the operating hour. In another work, Kutlu et al. (2018) applied pressurized hot water as the storage unit in a solar ORC. It was found that the adjustment of the mass flow rate of water in the evaporator section of the ORC gave a power output range of 4.3–5.7 kW in the daytime and 4.7 and 4.3 kW at late night. This finding showed that the integration of a storage unit with the solar ORC can facilitate the operation of the system even in the absence of solar radiation.
The performance of the solar ORCs using storage unit is affected by different factors, and the configuration of the system is one of the most important factors. For instance, Alvi et al. (2020) compared the performance of both direct and indirect solar ORCs by applying the same collector, operating fluid, and storage unit. For the considered case study (Islam Abad in Pakistan), their model revealed that the annual system efficiency of the direct solar ORC was 71.96% higher compared to the corresponding value of the indirect solar ORC. Furthermore, they found that the capacity of the systems increased by 21.71 and 17% for the indirect and direct solar ORCs, respectively. In another work, Wang et al. (2014) investigated the impacts of different factors on the performance of a solar ORC with compound parabolic collector. They found that decreases in the ambient temperature and increases in the mass flow rate of thermal oil (as storage unit) could enhance the performance of the cycle in off-design conditions. Other parameters can also influence the performance of the simple or regenerative solar ORCs; for instance, a regenerative solar ORC with thermal storage unit was analyzed by Wang et al. (2013). It was found that increment in the turbine inlet temperature and pressure leads to performance improvement of the system. Due to the dependency of the performance on the operating conditions, the optimal size of the storage unit is dependent on these factors. For instance, He et al. (2012) investigated the performance of a solar ORC with parabolic solar trough and found that the optimal volume of the storage unit highly depends on the solar irradiation, and the highest size is required in summer solstice. Operating conditions affect the economic indicators of the solar ORCs with thermal storage units in addition to the technical aspects. According to the findings of a study by (Baral, 2020), solar source temperature remarkably influences the electricity generation cost. Based on their case study, the cost of electricity generation in cases of solar heat sources of 90
The type of storage unit can remarkably affect the overall performance of the solar ORCs using storage. As an example, Bellos et al. (2020) investigated the performance of a regenerative solar ORC shown in Figure 3 by applying three storage types, including pure thermal oil, thermal oil in addition to rocks, and finned PCM. As seen in Figure 4, the use of PCM and thermal oil + rock improved the efficiency as defined by the ratio of net electricity generation to the heat input in the system, compared to the use of pure oil as storage. The Net Present Value (NPV) of the system was compared for different types of storage units and it was found that applying pure oil results in the lowest NPV for all the considered collecting areas. According to the findings of this study, it can be determined that system performance in terms of technical and financial criteria is under the influence of storage material and type since the stored heat in the thermal storage unit is dependent on the properties of the applied material, such as the latent and sensible heat capacities, and thermal conductivity of the material (Iasiello et al., 2017). In this regard, it would be beneficial to assess different storage types and materials to find the most appropriate choice.
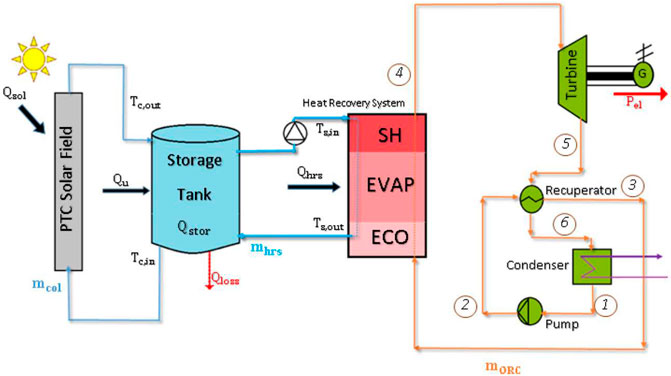
FIGURE 3. Schematic of regenerative solar ORC with different types of storage units (Bellos et al., 2020).
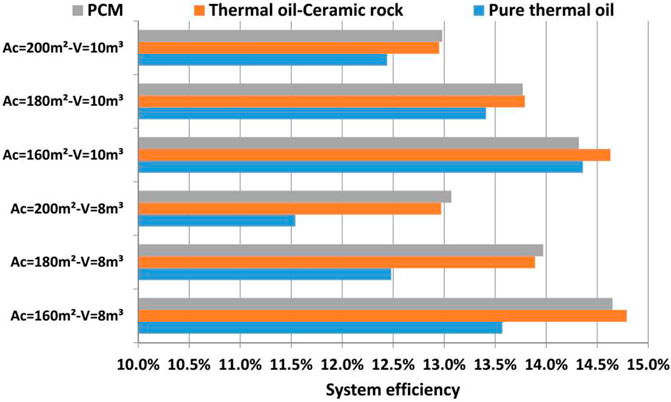
FIGURE 4. Efficiency of the system in different collecting areas (Bellos et al., 2020).
Solar ORCs with thermal storage unit have been optimized by considering different objective functions; for instance, Yu et al. (2021) optimized a solar ORC with and without recuperator as shown in Figure 5 by considering the overall efficiency of the cycle as the objective function. In their study, the decision variables of the optimization were temperatures of the hot and cold tanks, evaporation pressure, operating fluid mass flow rate, heat load of the recuperator, and cold tank temperature (recuperative ORC). They found that the thermal efficiency of the cycle with recuperator was around 11.2–18.7% higher than the systems without it. Other criteria can also be used to optimize solar ORCs with thermal storage; for instance, Bellos and Tzivanidis (2020) financially optimized a solar ORC with recuperator as shown in Figure 6 by considering the volume of the storage tank and the area of collecting as the variables. The nominal power generation was set 10 kW and for this system, the minimum payback period was found to be 8.37 years. They also found that there is an optimum value for the size of the storage tank as higher values were not beneficial from the financial point of view. Increment in the size more than the optimal value would not be useful since the storage capacity is limited and higher that it cannot be possible or utilizable for the system.
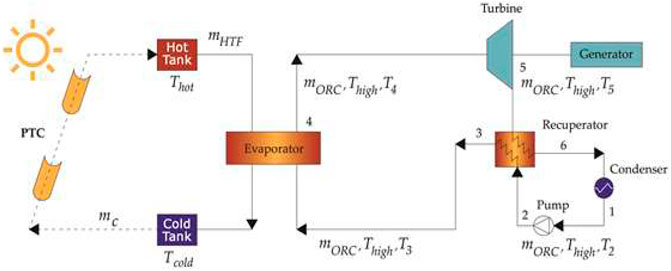
FIGURE 5. Schematic of a solar ORC with two-tank thermal storage and recuperator (Yu et al., 2021).
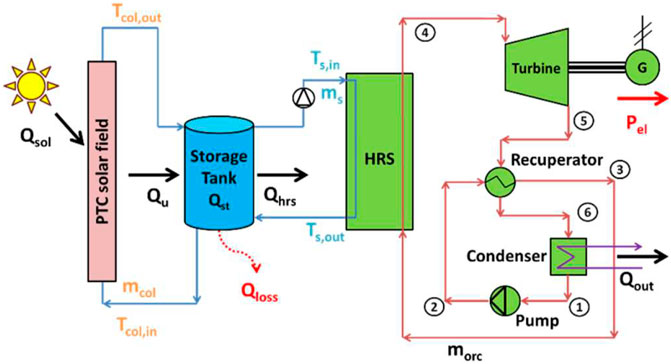
FIGURE 6. Schematic of solar ORC with storage tank and recuperator (Bellos and Tzivanidis, 2020).
Solar ORCs can be integrated with other systems to supply other energy-related output in addition to electrical power (Tarique et al., 2014; Kutlu et al., 2019). For instance, Ramos et al. (2018) investigated two configurations, as shown in Figure 7, to investigate their performance. The proposed configurations were used to generate power and supply domestic hot water (DHW). Different working fluids were used in the proposed configurations to find the optimal performance. It was observed that in term of electricity prioritization, using evacuated tube solar collector is preferred. Furthermore, in case of using the best fluid for each configuration, the investment cost of the configuration using flat plate collector was much higher than the one using evacuated tube. In another study, a solar combined heat and power system using ORC and thermal storage was investigated by Freeman et al. (2017) as shown in Figure 8. The study compared the performance of the system using different PCMs and sensible thermal energy storage. They found that using inorganic PCM as the storage led to the highest outlet work of ORC. Utilizing inorganic PCM resulted in 20 and 41% higher daily output in comparison to the cases of using the best considered sensible storage and the best solid thermal energy storage, respectively.
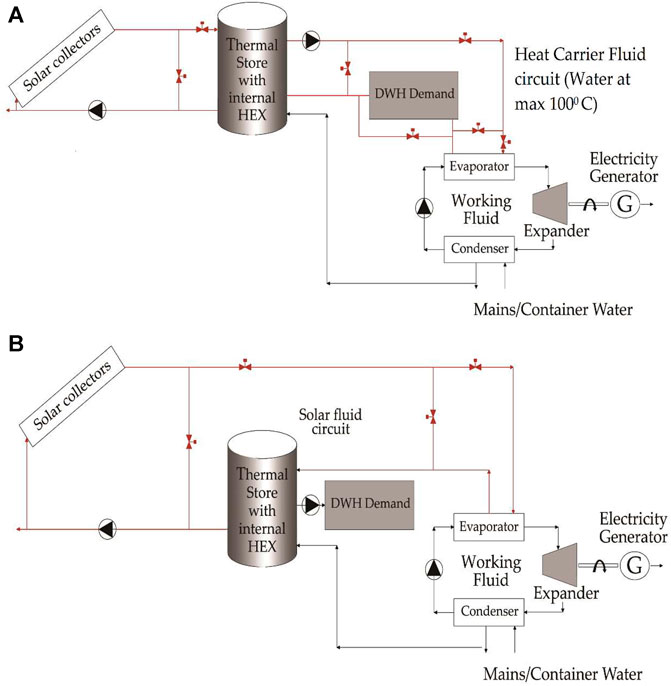
FIGURE 7. Solar ORCs with (A) flat-plate collector, (B) evacuated collector (Ramos et al., 2018).
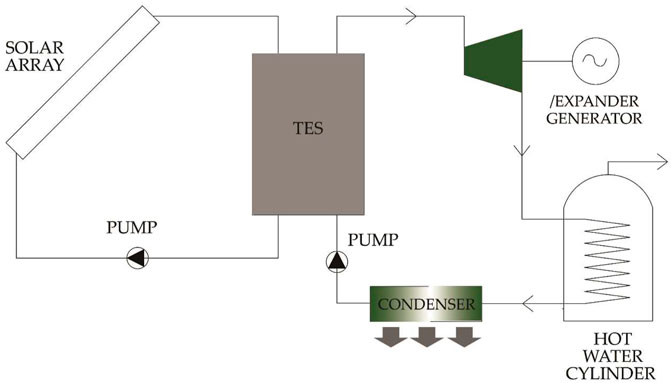
FIGURE 8. Schematic of a CHP system using ORC and thermal storage (Freeman et al., 2017).
Table 3 summarized the findings of the works on the applications of the thermal storage units in the solar ORCs.
Existing Challenges for Using Thermal Storage Units in Solar ORCs
In the previous sections of this article, the applications of thermal storage units in solar ORCs have been reviewed. According to the outcomes of the research studies in this field, using storage unit in these systems would be useful in terms of power generation, operating hour extension, and reliability of the plants; however, there are some challenges in this area. First, the integration of thermal storage units will increase the cost of the system, which may cause higher levelized cost of electricity generation. In addition, applying storage units require some accessories that will increase the complexity of the systems and the maintenance cost. The use of storage units may also necessitate some changes in the configuration of the system. Besides the mentioned challenges, the selection of thermal storage material and type is another issue that must be considered in the design of solar ORCs with storage units. To properly integrate storage units with solar ORCs, it is crucial to consider different factors, such as the required volume of storage material, the operating temperature of the system, and the working fluid.
Recommendations for Future Works
The performance of solar ORCs has been investigated from different perspectives in recent years; however, there are some suggestions that can be considered for the future works. First, there is not enough experimental work on the solar ORCs, and it is highly recommended to perform experimental works to reach more realistic results. In the experimental works, the effects of different parameters must be considered by varying the operating conditions, such as the ambient temperature and pressure, and solar irradiation. In addition, it would be useful to consider exergy and exergoeconomic analysis more widely in future to get better insight into the system performance (Kerme and Orfi, 2015). By conducting exergy analysis and determining the exergy destruction of the components, the potentials of performance improvement could be better distinguished. Furthermore, the storage unit of the solar ORC can be improved by using different tools and approaches, such as the incorporation of nanotechnology (Alhuyi Nazari et al., 2021), and conductive heat transfer mediums such as heat pipes and thermosyphons. The idea of using heat pipes has been considered in some other energy-related systems and have shown efficient performance; this approach can be applied in solar ORCs with thermal storage units to accelerate and improve heat transfer and consequently modify the overall performance (Jung and Boo, 2014; Wu et al., 2018). Moreover, the thermal performance of the system can be enhanced by dispersing nanoparticles in the PCMs or heat transfer fluids used for sensible storage due to the modification of the thermophyscial properties (Ramezanizadeh and Alhuyi Nazari, 2019). For the modeling and forecasting of the performance of the solar ORCs with thermal storage units, intelligent methods such as artificial neural networks can be applied due to their ability in modeling complex systems. By employing these methods and using proper inputs, the outputs of the solar ORCs can be predicted in a fast and accurate way; furthermore, the obtained models would be useful for the optimization of the systems.
In addition to the modeling and performance improvement of solar ORCs with thermal storage components, there are some suggestions for optimization of these systems. For instance, it is highly suggested to apply more novel algorithms to optimize solar ORCs with storage units. In addition, hybrid optimization approaches obtained by integrating different algorithms would be useful for the future works. Moreover, it is recommended to apply optimization with more objective functions. The components of the storage units, such as the dimension and number of fins (Shinde et al., 2017), can also be optimized to reach the maximum efficiency and output of the systems. Finally, the ORCs using different renewable energies as heat source can be coupled with thermal storage units, analyzed and optimized by considering different objective functions.
Conclusion
ORCs are promising technologies for power generation from solar energy due to their ability in power generation using low or medium temperature heat sources. To extend the operating hours and increase the output power of solar ORCs, different thermal energy storage units can be applied. In this work, the studies on solar ORCs with thermal storage units were reviewed and based on the review, the most important findings are as follows:
• The type and material of the storage unit significantly influences the performance and output of the system.
• In addition to technical features, the type of storage unit affects the economic aspect of solar ORCs.
• The performance improvement of the solar ORCs with thermal storage units is dependent on the system configuration.
• Different thermal storage types such as sensible and latent heat are applicable for solar ORCs.
• The operating hours of the solar ORCs could be extended by using thermal storage units to make them applicable in night hours.
• The optimal size of the storage unit is dependent on some factors such as solar irradiance and demand.
• Like the solar ORCs without storage units, using additional equipment such as recuperator can improve the performance.
The performance of the solar ORCs with storage unit is dependent on the operating conditions and the employed technologies, such as the type of collector.
• Solar ORCs with thermal storage units can be integrated with other technologies to supply other energy-related demand, such as heating load.
• There is an optimum size for storage units of solar ORCs and further increase would not be beneficial.
Author Contributions
MS and MF have designed the work and contributed in writing and implementation of the work. IM and OA have contributed in implementation of the work and edition. ME edited the manuscript and contributed in writing.
Funding
This work was partially supported by Universiti Sains Malaysia under Short-term grant No. 304/PELECT/6315330.
Conflict of Interest
The authors declare that the research was conducted in the absence of any commercial or financial relationships that could be construed as a potential conflict of interest.
Publisher’s Note
All claims expressed in this article are solely those of the authors and do not necessarily represent those of their affiliated organizations, or those of the publisher, the editors and the reviewers. Any product that may be evaluated in this article, or claim that may be made by its manufacturer, is not guaranteed or endorsed by the publisher.
Abbreviations
IRENA, International Renewable Energy Agency; DHW, Domestic Hot Water; DVG, Direct Vapor Generation; NPV, Net Present Value; ORC, Organic Rankine Cycle; PCM, Phase Change Material; PV, Photovoltaic.
References
Ahmadi, M. H., Alhuyi Nazari, M., Ghasempour, R., Pourfayaz, F., Rahimzadeh, M., and Ming, T. (2018). A Review on Solar‐Assisted Gas Turbines. Energy Sci Eng 6, 658–674. doi:10.1002/ese3.238
Alhuyi Nazari, M., Maleki, A., Assad, M. E. H., Rosen, M. A., Haghighi, A., Sharabaty, H., et al. (2021). A Review of Nanomaterial Incorporated Phase Change Materials for Solar thermal Energy Storage. Solar Energy 228, 1–19. doi:10.1016/J.SOLENER.2021.08.051
Alizadeh, H., Alhuyi Nazari, M., Ghasempour, R., Shafii, M. B., and Akbarzadeh, A. (2020). Numerical Analysis of Photovoltaic Solar Panel Cooling by a Flat Plate Closed-Loop Pulsating Heat Pipe. Solar Energy 206, 455–463. doi:10.1016/j.solener.2020.05.058
Alvi, J. Z., Feng, Y., Wang, Q., Imran, M., and Alvi, J. (2020). Modelling, Simulation and Comparison of Phase Change Material Storage Based Direct and Indirect Solar Organic Rankine Cycle Systems. Appl. Therm. Eng. 170, 114780. doi:10.1016/J.APPLTHERMALENG.2019.114780
Alvi, J. Z., Feng, Y., Wang, Q., Imran, M., and Pei, G. (2021). Effect of Working Fluids on the Performance of Phase Change Material Storage Based Direct Vapor Generation Solar Organic Rankine Cycle System. Energ. Rep. 7, 348–361. doi:10.1016/J.EGYR.2020.12.040
Amin, T. E., Roghayeh, G., Fatemeh, R., and Fatollah, P. (2015). Evaluation of Nanoparticle Shape Effect on a Nanofluid Based Flat-Plate Solar Collector Efficiency. Energy Exploration & Exploitation 33, 659–676. doi:10.1260/0144-5987.33.5.659
Assad, M. E. H., Nazari, M. A., and Rosen, M. A. (2021). “Applications of Renewable Energy Sources,” in Design and Performance Optimization of Renewable Energy Systems. Cambridge: Academic Press, 1–15. doi:10.1016/B978-0-12-821602-6.00001-8
Baccioli, A., Antonelli, M., and Desideri, U. (2017). Dynamic Modeling of a Solar ORC with Compound Parabolic Collectors: Annual Production and Comparison with Steady-State Simulation. Energ. Convers. Manage. 148, 708–723. doi:10.1016/J.ENCONMAN.2017.06.025
Bao, J., and Zhao, L. (2013). A Review of Working Fluid and Expander Selections for Organic Rankine Cycle. Renew. Sustain. Energ. Rev. 24, 325–342. doi:10.1016/J.RSER.2013.03.040
Baral, S. (2020). A Study of Effects on Economic Indicators for Different Heat Source Temperature on thermal Storage‐Based Solar Organic Rankine Cycle System. Eng. Rep. 2, e12102. doi:10.1002/ENG2.12102
Bellos, E., Sarakatsanis, I., and Tzivanidis, C. (2020). Investigation of Different Storage Systems for Solar-Driven Organic Rankine Cycle. Appl. Syst. Innov. 3 (3), 52. doi:10.3390/ASI3040052
Bellos, E., and Tzivanidis, C. (2020). Financial Optimization of a Solar-Driven Organic Rankine Cycle. Appl. Syst. Innov. 3, 23. doi:10.3390/ASI3020023
Castelli, A. F., Elsido, C., Scaccabarozzi, R., Nord, L. O., and Martelli, E. (2019). Optimization of Organic Rankine Cycles for Waste Heat Recovery from Aluminum Production Plants. Front. Energ. Res. 7, 44. doi:10.3389/FENRG.2019.00044
Darvish, K., Ehyaei, M., Atabi, F., and Rosen, M. (2015). Selection of Optimum Working Fluid for Organic Rankine Cycles by Exergy and Exergy-Economic Analyses. Sustainability 7, 15362–15383. doi:10.3390/SU71115362
Das, L., Rubbi, F., Habib, K., Aslfattahi, N., Saidur, R., Baran Saha, B., et al. (2021). State-of-the-Art Ionic Liquid & Ionanofluids Incorporated with Advanced Nanomaterials for Solar Energy Applications. J. Mol. Liquids 336, 116563. doi:10.1016/J.MOLLIQ.2021.116563
Desai, N. B., and Bandyopadhyay, S. (2016). Thermo-Economic Analysis and Selection of Working Fluid for Solar Organic Rankine Cycle. Appl. Therm. Eng. 95, 471–481. doi:10.1016/J.APPLTHERMALENG.2015.11.018
Dragomir-Stanciu, D., Saghebian, S. M., and Kurchania, A. (2020). The Influence of Condensing Temperature on the Efficiency of Solar Power Systems with ORC. Proced. Manufacturing 46, 359–363. doi:10.1016/J.PROMFG.2020.03.052
Faegh, M., and Shafii, M. B. (2017). Experimental Investigation of a Solar Still Equipped with an External Heat Storage System Using Phase Change Materials and Heat Pipes. Desalination 409, 128–135. doi:10.1016/j.desal.2017.01.023
Freeman, J., Guarracino, I., Kalogirou, S. A., and Markides, C. N. (2017). A Small-Scale Solar Organic Rankine Cycle Combined Heat and Power System with Integrated thermal Energy Storage. Appl. Therm. Eng. 127, 1543–1554. doi:10.1016/J.APPLTHERMALENG.2017.07.163
Gao, W., Yao, M., Chen, Y., Li, H., Zhang, Y., and Zhang, L. (2019). Performance of S-CO2 Brayton Cycle and Organic Rankine Cycle (ORC) Combined System Considering the Diurnal Distribution of Solar Radiation. J. Therm. Sci. 28 (3), 463–471. doi:10.1007/S11630-019-1114-8
Grange, B., Dalet, C., Falcoz, Q., Siros, F., and Ferrière, A. (2014). Simulation of a Hybrid Solar Gas-Turbine Cycle with Storage Integration. Energ. Proced. 49, 1147–1156. doi:10.1016/J.EGYPRO.2014.03.124
Green, M. A., Hishikawa, Y., Dunlop, E. D., Levi, D. H., Hohl-Ebinger, J., Yoshita, M., et al. (2019). Solar Cell Efficiency Tables (Version 53). Prog. Photovolt Res. Appl. 27, 3–12. doi:10.1002/pip.3102
Haghighi, A., Pakatchian, M. R., Assad, M. E. H., Duy, V. N., and Alhuyi Nazari, M. (2020). A Review on Geothermal Organic Rankine Cycles: Modeling and Optimization. J. Therm. Anal. Calorim. 144, 1799–1814. doi:10.1007/s10973-020-10357-y
He, Y.-L., Mei, D.-H., Tao, W.-Q., Yang, W.-W., and Liu, H.-L. (2012). Simulation of the Parabolic Trough Solar Energy Generation System with Organic Rankine Cycle. Appl. Energ. 97, 630–641. doi:10.1016/J.APENERGY.2012.02.047
Iasiello, M., Braimakis, K., Andreozzi, A., and Karellas, S. (2017). Thermal Analysis of a Phase Change Material for a Solar Organic Rankine Cycle. J. Phys. Conf. Ser. 923, 012042. doi:10.1088/1742-6596/923/1/012042
International Renewable Energy Agency IRENA (2021). Renewable Power Generation Costs in 2020. Abu Dhabi: International Renewable Energy Agency (IRENA).
Javanshir, A., Sarunac, N., and Razzaghpanah, Z. (2017). Thermodynamic Analysis of ORC and its Application for Waste Heat Recovery. Sustainability 9, 1974. doi:10.3390/su9111974
Jung, E. G., and Boo, J. H. (2014). Thermal Analytical Model of Latent Thermal Storage with Heat Pipe Heat Exchanger for Concentrated Solar Power. Solar Energy 102, 318–332. doi:10.1016/J.SOLENER.2013.11.008
Kalaiselvam, S., and Parameshwaran, R. (2014). Thermal Energy Storage Technologies for Sustainability: Systems Design, Assessment and Applications. Amsterdam, Netherlands: Elsevier, 1–430. doi:10.1016/C2013-0-09744-7
Kang, S. H. (2012). Design and Experimental Study of ORC (Organic Rankine Cycle) and Radial Turbine Using R245fa Working Fluid. Energy 41, 514–524. doi:10.1016/J.ENERGY.2012.02.035
Kerme, E., and Orfi, J. (2015). Exergy-Based Thermodynamic Analysis of Solar Driven Organic Rankine Cycle. J. Therm. Eng. 1, 192–202. doi:10.18186/JTE.25809
Khanlari, A., and Alhuyi Nazari, M. (2021). A Review on the Applications of Multi-Criteria Decision-Making Approaches for Power Plant Site Selection. J. Therm. Anal. Calorim. 2021, 1–17. doi:10.1007/S10973-021-10877-1
Klein, P., Roos, T. H., and Sheer, T. J. (2015). Parametric Analysis of a High Temperature Packed Bed Thermal Storage Design for a Solar Gas Turbine. Solar Energy 118, 59–73. doi:10.1016/J.SOLENER.2015.05.008
Kutlu, C., Erdinc, M. T., Li, J., Wang, Y., and Su, Y. (2019). A Study on Heat Storage Sizing and Flow Control for a Domestic Scale Solar-Powered Organic Rankine Cycle-Vapour Compression Refrigeration System. Renew. Energ. 143, 301–312. doi:10.1016/J.RENENE.2019.05.017
Kutlu, C., Li, J., Su, Y., Pei, G., and Riffat, S. (2018). Off-Design Performance Modelling of a Solar Organic Rankine Cycle Integrated with Pressurized Hot Water Storage Unit for Community Level Application. Energ. Convers. Manage. 166, 132–145. doi:10.1016/J.ENCONMAN.2018.04.024
Linke, P., Papadopoulos, A., and Seferlis, P. (2015). Systematic Methods for Working Fluid Selection and the Design, Integration and Control of Organic Rankine Cycles-A Review. Energies 8, 4755–4801. doi:10.3390/EN8064755
Manfrida, G., Secchi, R., and Stańczyk, K. (2016). Modelling and Simulation of Phase Change Material Latent Heat Storages Applied to a Solar-Powered Organic Rankine Cycle. Appl. Energ. 179, 378–388. doi:10.1016/J.APENERGY.2016.06.135
Markides, C. N. (2015). Low-Concentration Solar-Power Systems Based on Organic Rankine Cycles for Distributed-Scale Applications: Overview and Further Developments. Front. Energ. Res. 3, 47. doi:10.3389/FENRG.2015.00047
Mirzaei, M., Ahmadi, M. H., Mobin, M., Nazari, M. A., and Alayi, R. (2018). Energy, Exergy and Economics Analysis of an ORC Working with Several Fluids and Utilizes Smelting Furnace Gases as Heat Source. Therm. Sci. Eng. Prog. 5, 230–237. doi:10.1016/j.tsep.2017.11.011
Pantaleo, A. M., Camporeale, S. M., Sorrentino, A., Miliozzi, A., Shah, N., and Markides, C. N. (2020). Hybrid Solar-Biomass Combined Brayton/organic Rankine-Cycle Plants Integrated with thermal Storage: Techno-Economic Feasibility in Selected Mediterranean Areas. Renew. Energ. 147, 2913–2931. doi:10.1016/J.RENENE.2018.08.022
Petrollese, M., and Cocco, D. (2019). Robust Optimization for the Preliminary Design of Solar Organic Rankine Cycle (ORC) Systems. Energ. Convers. Manage. 184, 338–349. doi:10.1016/J.ENCONMAN.2019.01.060
Ramezanizadeh, M., and Alhuyi Nazari, M. (2019). Modeling Thermal Conductivity of Ag/Water Nanofluid by Applying a Mathematical Correlation and Artificial Neural Network. Int. J. Low-Carbon Tech. 14, 468–474. doi:10.1093/ijlct/ctz030
Ramos, A., Chatzopoulou, M. A., Freeman, J., and Markides, C. N. (2018). Optimisation of a High-Efficiency Solar-Driven Organic Rankine Cycle for Applications in the Built Environment. Appl. Energ. 228, 755–765. doi:10.1016/J.APENERGY.2018.06.059
Salari, A., Kazemian, A., Ma, T., Hakkaki-Fard, A., and Peng, J. (2020). Nanofluid Based Photovoltaic thermal Systems Integrated with Phase Change Materials: Numerical Simulation and Thermodynamic Analysis. Energ. Convers. Manage. 205, 112384. doi:10.1016/j.enconman.2019.112384
Sánchez-Orgaz, S., Pedemonte, M., Ezzatti, P., Curto-Risso, P. L., Medina, A., and Calvo Hernández, A. (2015). Multi-Objective Optimization of a Multi-Step Solar-Driven Brayton Plant. Energ. Convers. Manage. 99, 346–358. doi:10.1016/J.ENCONMAN.2015.04.077
Shinde, A., Arpit, S., Km, P., Rao, P. V. C., and Saha, S. K. (2017). Heat Transfer Characterization and Optimization of Latent Heat Thermal Storage System Using Fins for Medium Temperature Solar Applications. J. Solar Energ. Eng. 139, 031003-1–031003-10. doi:10.1115/1.4035517
Singh, A., Jamasb, T., Nepal, R., and Toman, M. (2018). Electricity Cooperation in South Asia: Barriers to Cross-Border Trade. Energy Policy 120, 741–748. doi:10.1016/j.enpol.2017.12.048
Solar (2020). International Energy Agency. Available at: https://www.iea.org/fuels-and-technologies/solar (Accessed November 2020).
Stark, C., Pless, J., Logan, J., Zhou, E., and Arent, D. J. (2015). Renewable electricity: Insights for the coming decade (No. NREL/TP-6A50-63604). Golden, CO (United States): National Renewable Energy Lab.(NREL). doi:10.2172/1176740
Stijepovic, M. Z., Papadopoulos, A. I., Linke, P., Stijepovic, V., Grujic, A. S., Kijevčanin, M., et al. (2017). Targeting and Design of Organic Rankine Cycle Systems for Multiple Heat Sources with Simultaneous Working Fluid Selection. Comput. Aided Chem. Eng. 40, 769–774. doi:10.1016/B978-0-444-63965-3.50130-6
Tarique, A., Dincer, I., and Zamfirescu, C. (2014). “Energy and Exergy Analyses of Solar-Driven ORC Integrated with Fuel Cells and Electrolyser for Hydrogen and Power Production,” in Progress in Exergy, Energy, and the Environment. Editors I. Dincer, A. Midilli, and H Kucuk (Cham: Springer), 81–89. doi:10.1007/978-3-319-04681-5_7
Tchanche, B. F., Lambrinos, G., Frangoudakis, A., and Papadakis, G. (2010). Exergy Analysis of Micro-Organic Rankine Power Cycles for a Small Scale Solar Driven Reverse Osmosis Desalination System. Appl. Energ. 87, 1295–1306. doi:10.1016/J.APENERGY.2009.07.011
Tchanche, B. F., Papadakis, G., Lambrinos, G., and Frangoudakis, A. (2009). Fluid Selection for a Low-Temperature Solar Organic Rankine Cycle. Appl. Therm. Eng. 29, 2468–2476. doi:10.1016/J.APPLTHERMALENG.2008.12.025
Thurairaja, K., Wijewardane, A., Jayasekara, S., and Ranasinghe, C. (2019). Working Fluid Selection and Performance Evaluation of ORC. Energ. Proced. 156, 244–248. doi:10.1016/J.EGYPRO.2018.11.136
Tian, H., and Shu, G. Q. (2017). “Organic Rankine Cycle Systems for Large-Scale Waste Heat Recovery to Produce Electricity,” in Organic Rankine Cycle (ORC) Power Systems: Technologies and Applications. Sawston: Woodhead Publishing, 613–636. doi:10.1016/B978-0-08-100510-1.00017-X
Wang, J., Yan, Z., Zhao, P., and Dai, Y. (2014). Off-Design Performance Analysis of a Solar-Powered Organic Rankine Cycle. Energ. Convers. Manage. 80, 150–157. doi:10.1016/J.ENCONMAN.2014.01.032
Wang, M., Wang, J., Zhao, Y., Zhao, P., and Dai, Y. (2013). Thermodynamic Analysis and Optimization of a Solar-Driven Regenerative Organic Rankine Cycle (ORC) Based on Flat-Plate Solar Collectors. Appl. Therm. Eng. 50, 816–825. doi:10.1016/J.APPLTHERMALENG.2012.08.013
Wang, R., Jiang, L., Ma, Z., Gonzalez-Diaz, A., Wang, Y., and Roskilly, A. (2019). Comparative Analysis of Small-Scale Organic Rankine Cycle Systems for Solar Energy Utilisation. Energies 12, 829. doi:10.3390/EN12050829
Wang, Y., Kamari, M. L., Haghighat, S., and Ngo, P. T. T. (2020). Electrical and thermal Analyses of Solar PV Module by Considering Realistic Working Conditions. J. Therm. Anal. Calorim. 144, 1925–1934. doi:10.1007/s10973-020-09752-2
Wu, G., Liu, Q., Wang, J., and Sun, B. (2020). Thermal Analysis of Water-Cooled Photovoltaic Cell by Applying Computational Fluid Dynamics. J. Therm. Anal. Calorim. 144, 1741–1747. doi:10.1007/s10973-020-10283-z
Wu, W., Dai, S., Liu, Z., Dou, Y., Hua, J., Li, M., et al. (2018). Experimental Study on the Performance of a Novel Solar Water Heating System with and without PCM. Solar Energy 171, 604–612. doi:10.1016/j.solener.2018.07.005
Yan, Q., Hu, E., Yang, Y., and Zhai, R. (2011). Evaluation of Solar Aided Thermal Power Generation with Various Power Plants. Int. J. Energ. Res. 35, 909–922. doi:10.1002/ER.1748
Yu, H., Helland, H., Yu, X., Gundersen, T., and Sin, G. (2021). Optimal Design and Operation of an Organic Rankine Cycle (ORC) System Driven by Solar Energy with Sensible thermal Energy Storage. Energ. Convers. Manage. 244, 114494. doi:10.1016/J.ENCONMAN.2021.114494
Keywords: solar energy, thermal storage, organic rankine cycles, renewable energy, energy storage
Citation: Salem M, Fahim Alavi M, Mahariq I, Accouche O and El Haj Assad M (2021) Applications of Thermal Energy Storage in Solar Organic Rankine Cycles: A Comprehensive Review. Front. Energy Res. 9:766292. doi: 10.3389/fenrg.2021.766292
Received: 28 August 2021; Accepted: 27 September 2021;
Published: 13 October 2021.
Edited by:
Navid Aslfattahi, University of Malaya, MalaysiaReviewed by:
Likhan Das, University of Technology Petronas, MalaysiaSilvia Lasala, Université de Lorraine, France
Copyright © 2021 Salem, Fahim Alavi, Mahariq, Accouche and El Haj Assad. This is an open-access article distributed under the terms of the Creative Commons Attribution License (CC BY). The use, distribution or reproduction in other forums is permitted, provided the original author(s) and the copyright owner(s) are credited and that the original publication in this journal is cited, in accordance with accepted academic practice. No use, distribution or reproduction is permitted which does not comply with these terms.
*Correspondence: Mohamed Salem, c2FsZW1tQHVzbS5teQ==