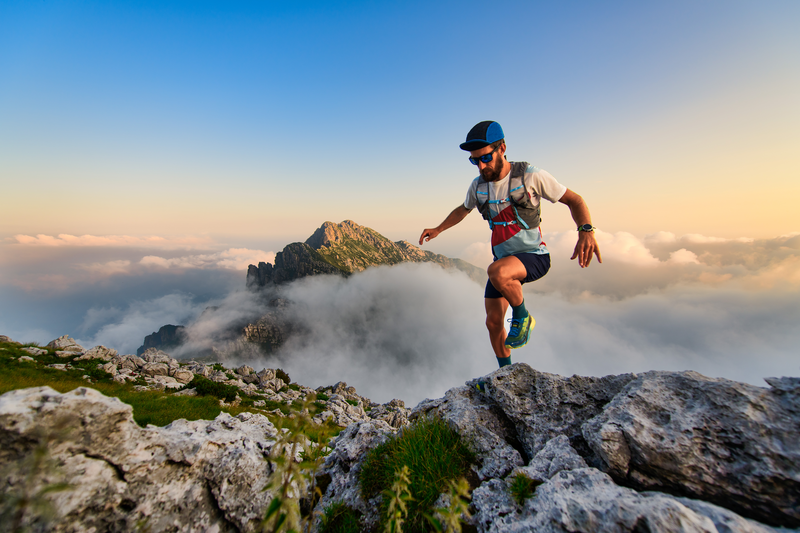
95% of researchers rate our articles as excellent or good
Learn more about the work of our research integrity team to safeguard the quality of each article we publish.
Find out more
ORIGINAL RESEARCH article
Front. Energy Res. , 20 August 2021
Sec. Process and Energy Systems Engineering
Volume 9 - 2021 | https://doi.org/10.3389/fenrg.2021.737915
This article is part of the Research Topic Enhancing Heat Transfer by Using Nanofluid to Improve the Efficiency of Thermal Systems View all 7 articles
This paper investigates the thermophysical properties and heat transfer performance of graphene nanoplatelet (GNP) and alumina hybrid nanofluids at different mixing ratios. The electrical conductivity and viscosity of the nanofluids were obtained at temperatures between 15–55°C. The thermal conductivity was measured at temperatures between 20–40°C. The natural convection properties, including Nusselt number, Rayleigh number, and heat transfer coefficient, were experimentally obtained at different temperature gradients (20, 25, 30, and 35°C) in a rectangular cavity. The Mouromtseff number was used to theoretically estimate all the nanofluids’ forced convective performance at temperatures between 20–40°C. The results indicated that the thermal conductivity and viscosity of water are increased with the hybrid nanomaterial. On the other hand, the viscosity and thermal conductivity of the hybrid nanofluids are lesser than that of mono-GNP nanofluids. Notwithstanding, of all the hybrid nanofluids, GNP-alumina hybrid nanofluid with a mixing ratio of 50:50 and 75:25 were found to have the highest thermal conductivity and viscosity, enhancing thermal conductivity by 4.23% and increasing viscosity by 15.79%, compared to water. Further, the addition of the hybrid nanomaterials improved the natural convective performance of water while it deteriorates with mono-GNP. The maximum augmentation of 6.44 and 10.48% were obtained for Nuaverage and haverage of GNP-Alumina (50:50) hybrid nanofluid compared to water, respectively. This study shows that hybrid nanofluids are more effective for heat transfer than water and mono-GNP nanofluid.
Heat transfer enhancement is essential towards reducing the energy consumption of numerous thermal systems, including nuclear cooling, automobile engine cooling, refrigeration, air conditioning systems, etc. Most of these thermal systems use conventional working fluids such as water, engine oil, glycols, etc. Over the last decade, the thermophysical properties of these fluids have been improved for thermal transport with the addition of nanomaterials to form a nanofluid (She and Fan, 2018; Borode et al., 2019). Nanofluids have been extensively studied and shown to exhibit enhanced thermophysical properties compared to conventional working fluids (Yazid et al., 2017; Irandoost Shahrestani et al., 2021). Numerous nanomaterials have been used to develop a nanofluid. However, hybrid nanomaterials are currently attracting more attention for the creation of advanced nanofluids with better thermophysical properties. A hybrid nanofluid is a suspension of two or more nanomaterials in a base fluid, which indicates it is an extension of single or mono nanofluids (Hussein, 2017; Nisar et al., 2020). Numerous studies (Chopkar et al., 2007; Jha and Ramaprabhu, 2009; Suresh et al., 2011; Aravind and Ramaprabhu, 2013; Munkhbayar et al., 2013; Senthilraja et al., 2015; Megatif et al., 2016) have reported a higher thermal conductivity for hybrid nanofluids compared to mono nanofluids, while other studies (Jana et al., 2007; Baghbanzadeh et al., 2012) also reported otherwise. Similarly, some authors observed a reduction in the viscosity of hybrid nanofluids compared to the mono nanofluids, while few studies reported a higher viscosity (Kazemi et al., 2020; Kumar and Sarkar, 2020). This shows that hybrid nanofluids can either increase or decrease the thermophysical properties of mono nanofluids depending on the compatibility of the nanomaterials.
A host of studies have explored the natural convective heat transfer application of hybrid nanofluids and mono nanofluids. Parvin et al. (2012) assessed the natural convection flow of alumina nanofluid in an annulus. They reported a thermal performance augmentation, which is attributed to the presence of alumina in water. This enhancement was further intensified with an increase in the concentration of the nanomaterial. Nasrin et al. (2020) conducted a numerical investigation of the heat transfer performance of single and hybrid nanofluids of Cu with other nanomaterials, including TiO2, CuO, alumina and carbon nanotube (CNT), in a cavity. They reported an increase of 8.1, 9.1, 10.2, 11.4, and 13.6% in the Nu value of nanofluids of Cu, Cu-TiO2, Cu-CuO, Cu-alumina and Cu-CNT, respectively, compared to water. This indicates that all the hybrid nanofluids exhibit superior convective heat transfer performance than the single Cu-based nanofluid and water.
The natural convection of alumina-multi-walled carbon nanotube (MWCNT) hybrid nanofluids with mixing ratios of 95:5 and 90:10 were experimentally investigated by (Giwa et al., 2018). They observed an improvement in the convective heat transfer performance of the hybrid nanofluids compared to distilled water and mono-alumina nanofluid. The research group (Giwa et al., 2020a) conducted further studies on the natural convection of alumina-MWCNT hybrid nanofluids with different mixing ratios (80:20, 60:40, 40:60, and 20:80) in a square cavity. They reported an enhancement in the free convection properties of all the hybrid nanofluids compared to water. Alumina-MWCNT hybrid nanofluid with a 60:40 ratio exhibited the highest convective performance at different temperature gradients. Estellé et al. (2017) assessed the free convection of mono-CNT nanofluid in a square cavity. They found that the addition of CNT reduces the Nusselt number (Nu) of the base fluid. Kouloulias et al. (2016) also reported a deterioration in the natural convection of a base fluid with the addition of mono-alumina. This was majorly attributed to nanofluid sedimentation. In contrast to the study by Kouloulias et al. (2016) and Moradi et al. (2020) reported an improvement in the heat transfer with the application of alumina nanofluids. Furthermore, numerous authors (Ghodsinezhad et al., 2016) observed an optimal enhancement in heat transfer using 0.1 vol% nanofluids, after which it starts depreciating at a higher concentration.
The literature reviewed shows a deterioration in the free convection heat transfer of some mono-particle nanofluids. However, hybrid nanofluids with concentrations lesser or equal to 0.1 vol% were found to improve heat transfer compared to the base fluid. Also, heat transfer studies on graphene-based hybrid nanofluids are limited despite the remarkable properties of the nanomaterial. Graphene has been identified to possess outstanding thermal conductivity and low density, making it an exceptional nanomaterial for the preparation of nanofluids (Borode et al., 2019). Furthermore, much like other nanomaterials, suspension of graphene in an aqueous solution tends to increase the viscosity of the base fluid (Rasheed et al., 2016). The viscosity of nanofluids is one of the significant factors that limits or reduces the thermal performance of nanofluids. Thus, compatible hybridization of nanomaterials can produce a nanofluid with exceptional heat transfer performance.
In this study, the comparative effect of different mixing ratios on the thermophysical properties and heat transfer performance of mono-graphene nanoplatelet (GNP) nanofluids and GNP-alumina hybrid nanofluids at the same volume concentration of 0.1 vol% was investigated. To the best of our knowledge, there is little to no study on the thermophysical properties and free convective heat transfer performance of GNP-alumina nanofluids. Mono-GNP and GNP-alumina hybrid with mixing ratios of 25:75, 50:50, and 75:25 with volume concentration of 0.1 vol% were loaded into distilled water. The thermal conductivity and viscosity of the prepared nanofluids and distilled water were measured at different temperatures. The natural convective heat transfer of all the thermo-fluids was assessed in a differentially heated cavity at different temperature gradients. Finally, the efficacy of the fluids for forced convection heat transfer was theoretically evaluated using the Mouromtseff number.
In addition, it is essential to note that there are limited experimental studies on the natural convection of nanofluids based on the available literature, with the majority of studies focused on numerical analysis. Hence, this study is significant because it is one of the limited peer-reviewed articles to experimentally evaluate the free convection performance of nanofluids. Also, to the best of our knowledge, this study is one of the first research articles to focus on the thermo-convection performance of GNP-alumina hybrid nanofluids. Furthermore, this study theoretically considers the forced convection performance of the hybrid nanofluids.
The materials and methods required to fulfil the aim and objectives of this study are presented in this section.
The mono GNP and GNP-alumina hybrid nanofluids used in this study were prepared using a two-step technique. The GNP (15 nm thickness and 50–80 m2/g specific surface area) and gamma-alumina (20–30 nm diameter, 180 m2/g specific surface area) were purchased from Sigma Aldrich (Germany) and Nanostructured and Amorphous Materials Inc. (United States), respectively. Sodium dodecyl sulfate obtained from Sigma Aldrich (Germany) was used as surfactants to suspend the nanomaterials in distilled water stably. The hybrid nanofluids with a volume concentration of 0.1 vol% were prepared with different GNP and alumina (Al2O3) mixing ratios (25:75, 50:50, and 75:25). The weight of the nanomaterials was calculated using Eq. 1
All measurements were done using Radwag AS 220. R2 digital weighing balance (±0.01 g accuracy, Poland). The sodium dodecyl sulfate surfactant at a nanomaterial-surfactant ratio of 1:1 was first added to the distilled water, and the mixture was agitated using a magnetic stirrer for 5 min. The mono or hybrid nanomaterial was then added, followed by further agitation for 10 min. Finally, the agitated nanofluid mixture was further sonicated for 45 min using a Q-700 Qsonica ultrasonicator (700 W, 20 kHz). To prevent overheating and evaporation of the nanofluid during sonication, the temperature of the nanofluid was maintained at a constant temperature of 20°C using a LAUDA ECO RE1225 water bath.
Different instruments were used to measure the thermophysical properties of the prepared nanofluids at different temperatures. The temperature of the nanofluids was controlled using the LAUDA ECO RE1225 water bath. All the instruments were first calibrated before the collection of data. The electrical conductivity of the nanofluids was measured using CON700 EUTECH electrical conductivity meter (±1% accuracy). The pH of the nanofluids was obtained using Jenway 3510 pH meter (±0.003 accuracy). SV-10 Vibro-viscometer (A and D, Japan; ±3% accuracy) was employed to determine the viscosity of the nanofluids. Finally, the thermal conductivity of the nanofluids was obtained using the DECAGON KD2 Pro thermal meter (±5% accuracy) with the aid of a KS-1 hot wire needle sensor.
The free convection heat transfer of GNP-alumina hybrid nanofluids was studied in a 99.7 mm × 113.2 mm × 120.8 mm rectangular cavity at different temperature gradients (20°C, 25°C, 30°C, and 35°C). The set-up for the study is presented in Figure 1. The set-up includes two PR20R Polyscience digital-controlled water baths (0.005°C accuracy) and isothermal shell and tube heat exchangers to achieve the cavity’s differential heating by maintaining the temperature of the cold and hot walls. In addition, Burkert 8,081 flow meter (accuracy ±0.01%) was employed to obtain the flow rate of water flowing through the heat exchangers. The temperatures in the cavity were measured using T-type thermocouples (Omega Engineering, United States, accuracy of 0.1°C) connected to Data Logger (SCXI-1303 National instrument).
The experimental data for the natural convection were collected after the nanofluids prepared were charged into the cavity and allowed to reach a steady-state after 1 h at different temperature gradients.
The average heat transfer rate, average heat transfer coefficient, Rayleigh number, and Nusselt number were calculated by measuring the flow rates, internal and external temperatures of the cavity. The model for the experimental values of the viscosity and thermal conductivity for the examined nanofluids were used in the calculations. The density, specific heat capacity, and coefficient of thermal expansion of the different nanofluid samples were estimated using Eqs 2, 3, 4. The thermophysical properties of the base fluid and nanomaterials are presented in Table 1.
TABLE 1. Values of the density, specific heat capacity, and coefficient of thermal expansion of distilled water and GNP.
The Rayleigh number, Ra, was estimated using Eq. 5
After that, the average heat transfer rate, Q, and average convection heat transfer coefficient, h, was calculated using Eqs 6, 7, respectively.
Where
The average Nusselt number, Nu, was evaluated using Eq. 8.
The experimental result was validated by examining the Nu of distilled water in the cavity as a function of Ra at different temperature gradients of 20°C, 25°C, 30°C, and 35°C. Furthermore, the results obtained were compared with that of the model proposed by Berkovsky and Polevikov (1977) and Leong et al. (1998). The Berkovsky model and Leong model are presented in Eqs. 9, 10.
Where Prandtl number,
Uncertainty analysis of Q, h, and Nu was done to quantify the data’s reliability due to the inputs’ variability. The inputs, which are a source of error, include temperature and flow rates. The values of uncertainty were obtained using Eqs. 11, 12, 13 (Giwa et al., 2020a).
The maximum uncertainty for Q, h, and Nu are 5.96, 6.03, and 6.33%, respectively.
This section covers the results obtained with the application of the materials and methods. Also, keys findings of the study were discussed.
The stability of the nanofluid samples used for this study was studied using a transmission electron microscope, viscosity measurement, and visual technique. The transmission electron microscope images of the mono-GNP nanofluid and hybrid GNP-alumina (50:50) nanofluid are presented in Figure 2. The alumina particles can be observed on the surface of the GNP, which indicates the stability of the hybrid nanofluid. The stability of the nanofluids was further analyzed by taking the viscosity of the nanofluids over 24 h, which is more than the total time taken to carry out the experiments. The viscosity of all the nanofluids as a function of time is illustrated in Figure 3. The almost linear measurements of all the nanofluids indicate that the nanofluids remain relatively stable for at least 24 h. Also, the visual analysis displayed in Figure 4 shows that the nanofluids are stable for at least 3 weeks without any visible sedimentation.
The effects of temperature on the electrical conductivity (
FIGURE 5. (A) Electrical conductivity and (B) the relative electrical conductivity of the GNP-alumina hybrid nanofluids for various mixing ratios at different temperatures.
The measured pH of water, mono-GNP nanofluid, GNP-alumina nanofluids with mixing ratios of 75:25, 50:50, and 25:75 were observed to range from 7.69–7.46, 8.06–7.01, 7.10–5.95, 8.19–6.87, and 8.25–7.41, respectively as the temperatures increase 15 °C–55 °C. This indicates that the pH of all the samples reduces at elevated temperatures. Further observation revealed that the hybrid GNP-alumina nanofluids have a lesser pH than mono GNP nanofluids. This shows that the addition of alumina causes a reduction in the H+ concentration of the GNP nanofluids.
The effects of temperature on the viscosity (
FIGURE 6. (A) Viscosity and (B) the relative viscosity of the GNP-alumina hybrid nanofluids for various mixing ratios at different temperatures.
The effects of temperature on the thermal conductivity (
FIGURE 7. Thermal conductivity of the GNP-alumina hybrid nanofluids for different mixing ratios as a temperature function.
A new correlation for the electrical conductivity (
Where R, which is the ratio of the weight of GNP to the total weight of GNP-alumina, ranges from 0.25 to 1. Figures 8A–C shows that the developed correlation for the predicted values of
FIGURE 8. Variation of (A) predicted electrical conductivity with experimental electrical conductivity, (B) predicted viscosity with experimental viscosity, and (C) predicted thermal conductivity with experimental thermal conductivity.
Natural thermo-convection are employed for numerous applications where heat transfer without forced or external motion is required. This section focused on the experimental results of the study on the natural convection of GNP-based nanofluids in a cavity.
The validation of the cavity was done with the experimental values of Nu as a function of Ra. The experimentally obtained Nu values of the distilled water were compared with the Nu values estimated using the Berkovsky model (Berkovsky and Polevikov, 1977) and the model from Leong et al. (1998), as presented in Figure 9. It was found that the two models cannot accurately predict the experimental values of the Nu in the cavity with the Ra values estimated in this study. The Berkovsky model overestimates the Nu values while the Leong Model underestimates the Nu values. This observation agrees with a host of a previous study (Ghodsinezhad et al., 2016; Giwa et al., 2020a) on the natural convection of nanofluids in a cavity.
The free convective heat transfer performance of GNP-alumina hybrid nanofluids was studied by evaluating the Ra, Nuaverage, and haverage. Figure 10A presents the Nuaverage of all the thermo-fluids as a function of Ra. The Ra of the base fluid ranges from 3.05 × 108–6.56 × 108, while that of the nanofluids ranges from 2.72 × 108–6.08 × 108. This shows that the addition of mono or hybrid nanomaterials causes a reduction in the Ra values of water. This could be ascribed to the changes in the thermophysical properties of water associated with the suspension of nanomaterials. Notwithstanding, despite the lower nanofluid’s Ra values, the addition of hybrid nanofluids augments the Nuaverage of water while that of mono-GNP nanofluid deteriorates. This observation is consistent with previous studies (Giwa et al., 2020b).
FIGURE 10. The average Nusselt number of all the samples at different (A) Rayleigh number and (B) temperature gradients.
The effects of the hybrid mixture ratios and temperature gradient on the Nuaverage are illustrated in Figure 10B. The figure shows that the Nuaverage increases as the temperature gradient is elevated for all the samples. Further observation reveals that the GNP-alumina (50:50) hybrid nanofluid has the highest Nuaverage, followed by GNP-alumina (75:25) and GNP-alumina (25:75) hybrid nanofluids. In addition, the Nuaverage of mono-GNP nanofluid was observed to be lower than that of water. This clearly shows that the addition of mono-GNP causes a deterioration in the convective heat transfer of water in a cavity. In contrast, the hybridization of GNP with alumina causes an enhancement in heat transfer. This enhancement could be attributed to the lower viscosity of the hybrid nanofluids compared to the mono-GNP’s viscosity. This indicates that the higher viscosity of mono-GNP nanofluid causes a reduction in the buoyant force-induced bulk fluid flow, which subsequently reduces heat transfer due to advection.
The Nuaverage of water is enhanced by 1.61–3.17%, 3.33–6.44%, and 3.23–5.43% with the addition of GNP-alumina with mixing ratios of 25:75, 50:50, and 75:25, respectively. In contrast, the addition of mono-GNP reduces the Nuaverage by 5.67–9.81% at the temperature gradients considered in this study.
The experimental data of the hybrid nanofluids were used to derive a correlation for the average Nusselt number as a function of Ra and R, as shown in Eq. 17. In addition, the developed correlation with a coefficient of determination (R2) of 96.36% is presented in Eq. 17.
The Nu predicted using the model conforms with the experimental results with a margin of deviation between -1.35 and 1.26%. The variation between the predicted and experimental Nu is illustrated in Figure 11. The comparison between the experimental value of Nuaverage with the developed correlation and the existing correlation by Giwa et al. (2020a) is illustrated in Figure 12. The figure confirms that the experimental values highly match the developed correlation. Furthermore, the developed correlation does not conform with the model by Giwa et al. (2020a), but they exhibit a similar trend.
The haverage of all the samples at different temperature gradients is illustrated in Figure 13. An increase in the temperature results in an enhancement in the haverage of all the samples examined in this study. Similar to the Nuaverage results, the maximum haverage was achieved with GNP-alumina (50:50) hybrid nanofluid. This was followed by GNP-alumina (75:25) and GNP-alumina (25:75) hybrid nanofluids. All the hybrid nanofluids exhibit a higher haverage than water, while the haverage of mono-GNP nanofluid is lesser than that of water. The haverage of water is enhanced by 4.79–5.96%, 7.58–10.48%, and 7.02–8.88% with GNP-alumina with mixing ratios of 25:75, 50:50, and 75:25, respectively. However, the addition of mono-GNP diminished the haverage of water by 0.78–5.30% at the temperature gradients considered in this study. Also, it is noteworthy to state that the optimum hybrid mixture ratio of GNP-alumina for maximum heat transfer augmentation is found at a ratio of 50:50. Also, the free convective heat transfer enhancement observed in this experimental study is consistent with numerous studies on the heat transfer performance of hybrid nanofluids (Giwa et al., 2020a; 2020b). The higher haverage of the hybrid nanofluids compared to water can be attributed to the higher thermal conductivity of the nanofluids, which improves heat transfer through conduction.
FIGURE 13. The average heat transfer coefficient of the different nanofluids at different temperature gradients.
On the other hand, the poor heat transfer performance of the mono-nanofluid is strongly linked to its higher viscosity compared to water and hybrid nanofluids. The higher viscosity of the mono-nanofluid lowers buoyant fluid flow from the hot side of the cavity to the cold side, which consequently reduces heat transfer through advection. The impact of this high viscosity coupled with high thermal conductivity causes the heat transfer with mono-nanofluid to be dependent on heat transfer through diffusion rather than advection. This resulted in a lower Nu value than water and hybrid nanofluids, as Nu is the ratio of heat transfer through advection (convection) to diffusion (conduction).
Furthermore, it is noteworthy to provide an insight into the difference in the heat transfer performance of the examined mono and hybrid nanofluids. To better comprehend the result of this study, Ra values exhibit an influence on the heat transfer performance of the nanofluids. The higher Ra and lower viscosity of the hybrid nanofluids has an effect on the augmentation of the Nuaverage and haverage compared to mono-nanofluid. This indicates that there is an intensification in the buoyant convective force and fluid flow from the hot side to the cold side of the cavity. An enhanced buoyant force causes an intensification in the motion of fluid particles and thermal transport to the boundary walls. This made heat transfer to be more dependent on advection rather than diffusion. Thus, resulting in a higher Nuaverage and haverage with the hybrid nanofluids compared to the mono-nanofluid.
Also, viscosity and thermal conductivity results show that these properties are strongly related to temperature and hybrid mixing ratio. The impact of lower viscosity and enhanced thermal conductivity at elevated temperatures was strongly pronounced in the heat transfer study. The haverage and Nuaverage were found to increase for the different nanofluids with increased temperature gradients.
In order to assess the forced convective heat transfer performance of the nanofluids in a thermal system, Mouromtseff Number (Mo) was employed. Mo is an indicator of the efficacy of a thermo-fluid in a thermal system. It is noteworthy to state that higher Mo values indicate higher thermal performance. The Mo of the samples was estimated using Eq. 18 (Minea and Moldoveanu, 2017).
Where the constants a = 0.8, b = 0.33, c = 0.67 and d = 0.47 for the nanofluids’ turbulent flow regime, while a = 0.8, b = 0.33, c = 0.8 and d = 0.47 for that of water (Huminic and Huminic, 2018; Leena and Srinivasan, 2018; Kumar et al., 2021).
Figure 14A shows Mo for the different hybrid nanofluids at different temperatures. All the nanofluids were found to display better heat transfer efficiency than water as the Mo of all the nanofluids is greater than water. It is noteworthy to state that all the GNP-alumina hybrid nanofluids exhibit better performance than the single GNP nanofluid. Also, the Mo results show that the nanofluid’s viscosity greatly influences the efficiency of a thermal system. This is evident as the hybrid nanofluids with the lowest viscosity exhibit the best performance. GNP-alumina (25:75) nanofluid displayed the best performance, followed by GNP-alumina (50:50) and GNP-alumina (75:25).
FIGURE 14. (A) Mouromtseff number and (B) pumping power ratio of the GNP-alumina hybrid nanofluids for different mixing ratios as a function of temperature.
It is important to note that viscosity significantly influences the pumping power of a thermal system. A higher viscosity is expected to increase the pumping power. Thus, the pumping power for the turbulent flow will be evaluated using Eq. 19 (Huminic and Huminic, 2018).
The pumping power ratio,
In this paper, the thermophysical properties and natural convection properties of 0.1 vol% of mono-GNP and hybrid GNP-alumina at different mixing ratios (25:75, 50:50, and 75:25) were experimentally studied. Also, the forced convection heat transfer was theoretically explored using the Mouromtseff number. The following conclusion can be deduced from the results of this study:
i. The electrical conductivity and thermal conductivity of all the samples (water, mono-GNP nanofluid, and hybrid nanofluids) are augmented at elevated temperatures while the viscosity and pH reduce.
ii. The electrical conductivity of water is improved with the addition of mono GNP and hybrid nanomaterials. Nanofluids with higher concentrations of alumina exhibit a higher electrical conductivity. GNP-alumina (25:75) hybrid nanofluid has the highest electrical conductivity of all the samples, with a maximum enhancement of 135.74%.
iii. With the addition of nanomaterials, the viscosity and thermal conductivity of water are augmented. The highest viscosity and thermal conductivity increase were obtained with the addition of mono-GNP. The maximum thermal conductivity enhancement of 5.62% was obtained for mono-GNP nanofluid at 40°C, while the maximum increase in viscosity is 17.54%.
iv. Among the GNP-alumina hybrid nanofluids, the highest thermal conductivity was recorded at a mixing ratio of 50:50. Also, hybrid nanofluids with a higher ratio of alumina tend to possess lower viscosity. This is evident as GNP-alumina hybrid nanofluids with a mixing ratio of 25:75 exhibit the lowest viscosity followed by that of 50:50.
v. Among all the samples, mono-GNP nanofluid is the least effective fluid regarding natural convective heat transfer performance, while GNP-alumina (50:50) hybrid nanofluid is the most effective. Compared to water, maximum enhancements of 3.17, 6.44, and 5.43% were obtained for Nuaverage of GNP-alumina hybrid nanofluid with mixing ratios of 25:75, 50:50 and 75:25, respectively. In a similar trend, the haverage is enhanced by 5.96, 10.48, and 8.88%. On the other hand, the Nuaverage and haverage deteriorated by 9.81 and 5.30% with mono-GNP nanofluid.
vi. Compared to water, the superior heat transfer performance of the hybrid nanofluids can be attributed to their superior thermal conductivity. However, a high viscosity can be ascribed to the poor thermal performance of mono-GNP nanofluids, which causes loss of buoyancy and made heat transfer dependent mainly on conduction.
vii. The theoretical analysis of the forced convection performance revealed that all the nanofluids (mono and hybrid) have a higher heat transfer efficiency than water. This shows that mono-GNP nanofluid is not suitable for heat transfer without an external motion.
viii. Further, in contrast to the free convection performance, GNP-alumina hybrid nanofluids with a mixing ratio of 25:75 have the best efficiency, followed by that of 50:50 and 75:25, while the mono-GNP nanofluid has the lowest efficiency.
ix. The correlation developed for the electrical conductivity, thermal conductivity, viscosity, and Nuaverage are in good agreement with the experimental data.
The raw data supporting the conclusions of this article will be made available by the authors, without undue reservation.
AB - Conceived and designed the experiments; Performed the experiments; Analyzed and interpreted the data; Wrote the paper. NA and PO - Conceived and designed the experiments; Contributed reagents, materials, analysis tools, Supervision, review and editing. MS, JM - review and editing, equipment, software, experimental design.
This work is based on the research supported by the National Research Foundation of South Africa (Grant Number: 132920).
The authors declare that the research was conducted in the absence of any commercial or financial relationships that could be construed as a potential conflict of interest.
All claims expressed in this article are solely those of the authors and do not necessarily represent those of their affiliated organizations, or those of the publisher, the editors and the reviewers. Any product that may be evaluated in this article, or claim that may be made by its manufacturer, is not guaranteed or endorsed by the publisher.
The authors acknowledge the support of Momin Modaser (Department of Mechanical and Aeronautical Engineering, University of Pretoria), who trained and assisted the first author on the use of the equipment at the Nanofluid Research Laboratory, University of Pretoria, South Africa.
CNT, Carbon nanotube; FOM, Figure-of-Merit; GNP, Graphene nanoplatelet; HTC, Heat transfer coefficient; MWCNT, Multi-walled carbon nanotubes
Aravind, S. S. J., and Ramaprabhu, S. (2013). Graphene-multiwalled Carbon Nanotube-Based Nanofluids for Improved Heat Dissipation. RSC Adv. 3 (13), 4199–4206. doi:10.1039/c3ra22653k
Baghbanzadeh, M., Rashidi, A., Rashtchian, D., Lotfi, R., and Amrollahi, A. (2012). Synthesis of Spherical Silica/multiwall Carbon Nanotubes Hybrid Nanostructures and Investigation of thermal Conductivity of Related Nanofluids. Thermochim. Acta 549, 87–94. doi:10.1016/j.tca.2012.09.006
Berkovsky, B., and Polevikov, V. (1977). Numerical Study of Problems on High-Intensive Free Convection. Available at: https://elib.bsu.by/handle/123456789/10278 (Accessed May 9, 2021).
Borode, A., Ahmed, N., and Olubambi, P. (2019). A Review of Solar Collectors Using Carbon-Based Nanofluids. J. Clean. Prod. 241, 118311. doi:10.1016/j.jclepro.2019.118311
Chopkar, M., Kumar, S., Bhandari, D. R., Das, P. K., and Manna, I. (2007). Development and Characterization of Al2Cu and Ag2Al Nanoparticle Dispersed Water and Ethylene Glycol Based Nanofluid. Mater. Sci. Eng. B 139 (2–3), 141–148. doi:10.1016/j.mseb.2007.01.048
Dezfulizadeh, A., Aghaei, A., Joshaghani, A. H., and Najafizadeh, M. M. (2021). An Experimental Study on Dynamic Viscosity and thermal Conductivity of Water-Cu-SiO2-MWCNT Ternary Hybrid Nanofluid and the Development of Practical Correlations. Powder Technol. 389, 215–234. doi:10.1016/J.POWTEC.2021.05.029
Estellé, P., Mahian, O., Maré, T., and Öztop, H. F. (2017). Natural Convection of CNT Water-Based Nanofluids in a Differentially Heated Square Cavity. J. Therm. Anal. Calorim. 128 (3), 1765–1770. doi:10.1007/s10973-017-6102-1
Ghodsinezhad, H., Sharifpur, M., and Meyer, J. P. (2016). Experimental Investigation on Cavity Flow Natural Convection of Al 2 O 3 -water Nanofluids. Int. Commun. Heat Mass Transfer 76, 316–324. doi:10.1016/j.icheatmasstransfer.2016.06.005
Giwa, S. O., Sharifpur, M., and Meyer, J. P. (2020b). Effects of Uniform Magnetic Induction on Heat Transfer Performance of Aqueous Hybrid Ferrofluid in a Rectangular Cavity. Appl. Therm. Eng. 170, 115004. doi:10.1016/j.applthermaleng.2020.115004
Giwa, S. O., Sharifpur, M., and Meyer, J. P. (2020a). Experimental Study of Thermo-Convection Performance of Hybrid Nanofluids of Al2O3-MWCNT/water in a Differentially Heated Square Cavity. Int. J. Heat Mass Transfer 148, 119072. doi:10.1016/j.ijheatmasstransfer.2019.119072
Giwa, S. O., Sharifpur, M., and Meyer, J. P. (2018). Heat Transfer Enhancement of Dilute Al2O3-MWCNT Water Based Hybrid Nanofluids in a Square Cavity. Int. Heat Transf. Conf., 5365–5372. doi:10.1615/ihtc16.hte.023927
Huminic, G., and Huminic, A. (2018). Heat Transfer Capability of the Hybrid Nanofluids for Heat Transfer Applications. J. Mol. Liquids 272, 857–870. doi:10.1016/j.molliq.2018.10.095
Hussein, A. M. (2017). Thermal Performance and thermal Properties of Hybrid Nanofluid Laminar Flow in a Double Pipe Heat Exchanger. Exp. Therm. Fluid Sci. 88, 37–45. doi:10.1016/j.expthermflusci.2017.05.015
Irandoost Shahrestani, M., Houshfar, E., Ashjaee, M., and Allahvirdizadeh, P. (2021). Convective Heat Transfer and Pumping Power Analysis of MWCNT + Fe3O4/Water Hybrid Nanofluid in a Helical Coiled Heat Exchanger with Orthogonal Rib Turbulators. Front. Energ. Res. 9, 12. doi:10.3389/FENRG.2021.630805
Jana, S., Salehi-Khojin, A., and Zhong, W.-H. (2007). Enhancement of Fluid thermal Conductivity by the Addition of Single and Hybrid Nano-Additives. Thermochim. Acta 462 (1–2), 45–55. doi:10.1016/j.tca.2007.06.009
Jha, N., and Ramaprabhu, S. (2009). Thermal Conductivity Studies of Metal Dispersed Multiwalled Carbon Nanotubes in Water and Ethylene Glycol Based Nanofluids. J. Appl. Phys. 106 (8), 084317. doi:10.1063/1.3240307
Kazemi, I., Sefid, M., and Afrand, M. (2020). A Novel Comparative Experimental Study on Rheological Behavior of Mono & Hybrid Nanofluids Concerned Graphene and Silica Nano-Powders: Characterization, Stability and Viscosity Measurements. Powder Technol. 366, 216–229. doi:10.1016/j.powtec.2020.02.010
Kouloulias, K., Sergis, A., and Hardalupas, Y. (2016). Sedimentation in Nanofluids during a Natural Convection experiment. Int. J. Heat Mass Transfer 101, 1193–1203. doi:10.1016/j.ijheatmasstransfer.2016.05.113
Kumar, V., Pare, A., Tiwari, A. K., and Ghosh, S. K. (2021). Efficacy Evaluation of Oxide-MWCNT Water Hybrid Nanofluids: An Experimental and Artificial Neural Network Approach. Colloids Surf. A: Physicochemical Eng. Aspects 620, 126562. doi:10.1016/j.colsurfa.2021.126562
Kumar, V., and Sarkar, J. (2020). Particle Ratio Optimization of Al2O3-MWCNT Hybrid Nanofluid in Minichannel Heat Sink for Best Hydrothermal Performance. Appl. Therm. Eng. 165, 114546. doi:10.1016/j.applthermaleng.2019.114546
Leena, М., and Srinivasan, S. (2018). Experimental Investigation of the Thermophysical Properties of TiO2/Propylene Glycol-Water Nanofluids for Heat-Transfer Applications. J. Eng. Phys. Thermophy 91 (2), 498–506. doi:10.1007/s10891-018-1770-7
Leong, W. H., Hollands, K. G. T., and Brunger, A. P. (1998). Experimental Nusselt Numbers for a Cubical-Cavity Benchmark Problem in Natural Convection. Int. J. Heat Mass. Transf. 42 (11), 1979–1989. doi:10.1016/S0017-9310(98)00299-3
Megatif, L., Ghozatloo, A., Arimi, A., and Shariati-Niasar, M. (2016). Investigation of Laminar Convective Heat Transfer of a Novel Tio2-Carbon Nanotube Hybrid Water-Based Nanofluid. Exp. Heat Transfer 29 (1), 124–138. doi:10.1080/08916152.2014.973974
Mehrali, M., Sadeghinezhad, E., Latibari, S., Kazi, S., Mehrali, M., Zubir, M. N. B. M., et al. (2014). Investigation of thermal Conductivity and Rheological Properties of Nanofluids Containing Graphene Nanoplatelets. Nanoscale Res. Lett. 9 (1), 15. doi:10.1186/1556-276X-9-15
Minea, A. A., and Moldoveanu, M. G. (2017). Studies on Al2O3, CuO, and TiO2 Water-Based Nanofluids: A Comparative Approach in Laminar and Turbulent Flow. J. Engin. Thermophys. 26 (2), 291–301. doi:10.1134/S1810232817020114
Moradi, A., Zareh, M., Afrand, M., and Khayat, M. (2020). Effects of Temperature and Volume Concentration on thermal Conductivity of TiO2-MWCNTs (70-30)/eg-Water Hybrid Nano-Fluid. Powder Technol. 362, 578–585. doi:10.1016/j.powtec.2019.10.008
Munkhbayar, B., Tanshen, M. R., Jeoun, J., Chung, H., and Jeong, H. (2013). Surfactant-free Dispersion of Silver Nanoparticles into MWCNT-Aqueous Nanofluids Prepared by One-step Technique and Their thermal Characteristics. Ceramics Int. 39 (6), 6415–6425. doi:10.1016/j.ceramint.2013.01.069
Nasrin, R., Hossain, S., Zahan, I., Ahmed, K. F. U., and Fayaz, H. (2020). Performance Analysis of Hybrid/single Nanofluids on Augmentation of Heat Transport in Lid‐driven Undulated Cavity. Heat Transfer 49 (8), 4204–4225. doi:10.1002/HTJ.21823
Nisar, K. S., Khan, U., Zaib, A., Khan, I., and Baleanu, D. (2020). Numerical Simulation of Mixed Convection Squeezing Flow of a Hybrid Nanofluid Containing Magnetized Ferroparticles in 50%:50% of Ethylene Glycol-Water Mixture Base Fluids between Two Disks with the Presence of a Non-linear Thermal Radiation Heat Flux. Front. Chem. 8, 792. doi:10.3389/FCHEM.2020.00792
Nordell, P. (2011). Aluminium Oxide - Poly(ethylene-Co-Butylacrylate) Nanocomposites : Synthesis, Structure, Transport Properties and Long-Term Performance.
Parvin, S., Nasrin, R., Alim, M. A., Hossain, N. F., and Chamkha, A. J. (2012). Thermal Conductivity Variation on Natural Convection Flow of Water-Alumina Nanofluid in an Annulus. Int. J. Heat Mass Transfer 55 (19–20), 5268–5274. doi:10.1016/J.IJHEATMASSTRANSFER.2012.05.035
Rasheed, A. K., Khalid, M., Rashmi, W., Gupta, T. C. S. M., and Chan, A. (2016). Graphene Based Nanofluids and Nanolubricants - Review of Recent Developments. Renew. Sustain. Energ. Rev. 63, 346–362. doi:10.1016/j.rser.2016.04.072
Said, Z., Saidur, R., Sabiha, M. A., Rahim, N. A., and Anisur, M. R. (2015). Thermophysical Properties of Single Wall Carbon Nanotubes and its Effect on Exergy Efficiency of a Flat Plate Solar Collector. Solar Energy 115, 757–769. doi:10.1016/j.solener.2015.02.037
Senthilraja, S., Vijayakumar, K., and Gangadevi, R. (2015). A Comparative Study on thermal Conductivity of Al2O3/water, CuO/water and Al2O3 – CuO/water Nanofluids. Dig. J. Nanomater. Biostructures 10 (4), 1449–1458.
She, L., and Fan, G. (2018). Numerical Simulation of Flow and Heat Transfer Characteristics of CuO-Water Nanofluids in a Flat Tube. Front. Energ. Res. 6 (JUN), 57. doi:10.3389/FENRG.2018.00057
Suresh, S., Venkitaraj, K. P., Selvakumar, P., and Chandrasekar, M. (2011). Synthesis of Al2O3-Cu/water Hybrid Nanofluids Using Two Step Method and its Thermo Physical Properties. Colloids Surf. A: Physicochemical Eng. Aspects 388 (1–3), 41–48. doi:10.1016/j.colsurfa.2011.08.005
Taherian, H., Alvarado, J. L., and Languri, E. M. (2018). Enhanced Thermophysical Properties of Multiwalled Carbon Nanotubes Based Nanofluids. Part 2: Experimental Verification. Renew. Sustain. Energ. Rev. 82, 4337–4344. doi:10.1016/j.rser.2017.05.117
Wang, J., Li, G., Li, T., Zeng, M., and Sundén, B. (2021). Effect of Various Surfactants on Stability and Thermophysical Properties of Nanofluids. J. Therm. Anal. Calorim. 143 (6), 4057–4070. doi:10.1007/s10973-020-09381-9
Wu, H., and Drzal, L. T. (2014). Effect of Graphene Nanoplatelets on Coefficient of thermal Expansion of Polyetherimide Composite. Mater. Chem. Phys. 146 (1–2), 26–36. doi:10.1016/j.matchemphys.2014.02.038
Xiao, W., Zhai, X., Ma, P., Fan, T., and Li, X. (2018). Numerical Study on the thermal Behavior of Graphene Nanoplatelets/epoxy Composites. Results Phys. 9, 673–679. doi:10.1016/j.rinp.2018.01.060
Yazid, M. N. A. W. M., Sidik, N. A. C., and Yahya, W. J. (2017). Heat and Mass Transfer Characteristics of Carbon Nanotube Nanofluids: A Review. Renew. Sustain. Energ. Rev. 80, 914–941. doi:10.1016/j.rser.2017.05.192
A cavity area (m2)
Cp specific heat capacity (J/Kg.K)
g acceleration due to gravity (9.8 m/s)
h convection heat transfer coefficient (W/m2.K)
L length of cavity (m)
M weight of nanoparticle (g)
ṁ mass flow rate per unit width (kg/m-s)
Mo Mouromtseff number
Nu Nusselt number
Q heat transfer rate (W)
R hybrid mixing ratio
Ra Rayleigh Number
W pumping power
vol% volume fraction of nanomaterials
β coefficient of thermal expansion (K−1)
θ temperature gradient (°C)
λ thermal conductivity (W/m.K)
μ viscosity (mPa.S)
ρ density (Kg/m3)
σ electrical conductivity (μS/cm)
φ volume concentration (vol%)
ω weight percent of nanoparticle
BF base fluid
c cold
h hot
HNF hybrid nanofluid
NF nanofluid
Keywords: graphene nanoplatelets, hybrid nanofluids, heat transfer, alumina nanoparticle, natural convection, thermal efficacy
Citation: Borode AO, Ahmed NA, Olubambi PA, Sharifpur M and Meyer JP (2021) Investigation of the Thermal Conductivity, Viscosity, and Thermal Performance of Graphene Nanoplatelet-Alumina Hybrid Nanofluid in a Differentially Heated Cavity. Front. Energy Res. 9:737915. doi: 10.3389/fenrg.2021.737915
Received: 07 July 2021; Accepted: 09 August 2021;
Published: 20 August 2021.
Edited by:
Valerie Eveloy, Khalifa University, United Arab EmiratesReviewed by:
Alina Adriana Minea, Gheorghe Asachi Technical University of Iași, RomaniaCopyright © 2021 Borode, Ahmed, Olubambi, Sharifpur and Meyer. This is an open-access article distributed under the terms of the Creative Commons Attribution License (CC BY). The use, distribution or reproduction in other forums is permitted, provided the original author(s) and the copyright owner(s) are credited and that the original publication in this journal is cited, in accordance with accepted academic practice. No use, distribution or reproduction is permitted which does not comply with these terms.
*Correspondence: Adeola O. Borode, aGFkZXlvbGEyMDAzQHlhaG9vLmNvbQ==; Mohsen Sharifpur, TW9oc2VuLnNoYXJpZnB1ckB1cC5hYy56YQ==
Disclaimer: All claims expressed in this article are solely those of the authors and do not necessarily represent those of their affiliated organizations, or those of the publisher, the editors and the reviewers. Any product that may be evaluated in this article or claim that may be made by its manufacturer is not guaranteed or endorsed by the publisher.
Research integrity at Frontiers
Learn more about the work of our research integrity team to safeguard the quality of each article we publish.