- 1Department of Civil Engineering, College of Engineering, Najran University, Najran, Saudi Arabia
- 2Department of Mechanical Engineering, College of Engineering, Najran University, Najran, Saudi Arabia
- 3Technische Universität Braunschweig, Braunschweig, Germany
The high share of buildings in energy consumption and carbon dioxide emission has led researchers to seek techniques to reduce energy consumption in this sector. In this study, considering a hot and arid climate region, the wall’s heat gain was investigated. To reduce energy demand, three techniques of adding PCM, combining absorption chiller with a solar system and dispersing nanoparticles were used and the results were evaluated transiently. In July, the addition of PCM to the building's walls reduced the heat exchange between interior and exterior spaces up to 21%. To cool the interior spaces, the combination of absorption chiller + fan coil was used and several flat plate collectors were integrated with it to reduce energy demand. By collecting energy in solar collectors and using a stratified tank, energy consumption in the generator section was reduced by 450 kWh. Nanoparticles were used to improve the solar system performance and it was found that loading ZnO and Al2O3 nanoparticles is useful. Dispersing ZnO into water increased the energy-saving by 9.5% while the second nanoparticle improved it by 14.5%.
Introduction
Buildings contribute a lot to pollution production and energy consumption (Jahangiri et al., 2016; Mostafaeipour et al., 2020; Kalbasi et al., 2021; Parsa, 2021; Song et al., 2021). Many solutions have been recommended by various researchers to reduce energy consumption (Ahmadi et al., 2017; Ahmad et al., 2018; Nwaji et al., 2019; Sarafraz et al., 2019; Azimi Fereidani et al., 2021; Nundy et al., 2021). Techniques include installing PCM (Ahangari and Maerefat, 2019; Lizana et al., 2019; Ziasistani and Fazelpour, 2019; Ben Romdhane et al., 2020; Miansari et al., 2020; Saxena et al., 2020), heat recovery (Liu et al., 2020; Shahsavar Goldanlou et al., 2020), using solar energy (Toghraie et al., 2018; Gagliano et al., 2019; Parsa et al., 2019; Gholipour et al., 2020; Menni et al., 2020; Parsa et al., 2020; Poon et al., 2020; Gholipour et al., 2021), other renewables such as wind (Jahangiri et al., 2019; Mostafaeipour et al., 2019; Kalbasi et al., 2021), geothermal sources (Kang et al., 2013; Palmero-Marrero et al., 2020) and finally using nanofluid (Kulkarni et al., 2009; Strandberg and Das, 2010; Moradi et al., 2019; Soltani et al., 2020; Mustafa et al., 2021). Considering the hot and dry climate, Li et al. (2019) by conducting a numerical study examined the effect of adding RT-27 PCM to a wall with 20 cm thickness. This material undergoes a phase change at 28–30
In this study, considering the hot and arid climate for Najran region (17.56°N, 44.22°E), a suitable PCM is selected and then by performing a transient analysis, the thermal behavior of this building is investigated. To cool the building in July, an absorption chiller with several solar collectors is combined to reduce energy demand. Then, nanofluids of
Problem Description
In this research, the main goal is to reduce energy demand in buildings, which is examined using two scenarios. In the first scenario, by reducing HGTW, the energy usage in HVAC sector reduces. In the second scenario, an absorption chiller is used to cool the building. For meeting the energy usage in the generator section, a solar collector filled with ZnO/water is used. Figure 1 shows that by adding PCM to the walls, the thermal resistance of the building rises and thus HGTW reduces both in winter and summer. Figure 1 shows that an absorption chiller enters the circuit to provide cooling in summer. In the evaporator, cold water within the temperature of 6-7°C enters the fan coil and returns to the evaporator by taking heat from the building. An evaporative cooling tower is used to cool the condenser. In an absorption chiller in the generator section, a hot water flow can be used to supply thermal energy.
Mathematical Formulation
The governing equations are presented in two parts. In the first part, due to the PCM phase change inside the wall, the governing equations are solved in such a way that the temperature distribution inside the wall can be obtained. To obtain the temperature, it is required to solve the continuity, momentum and energy equation:
where
The boundary conditions in x-direction as shown in are written as follows:
where
In solar collectors, the main parameter is the heat gain
where I
Results
PCM Efficacy on Heat Gain Through Wall
PCMs are substances that can store energy and then release it. The process of storing/releasing energy depends on the phase change temperature range

TABLE 1. Properties of the construction materials and studied PCMs (ASHRAEAmerican Society of Heating and Engineers, 2016; Tian et al., 2020a).
In this study, considering that the building is an office, so the schedule was chosen in such a way that between 8 a.m and 8 p.m, parameters of occupancy, lighting and equipment affect.
The only parameter that is affected by the presence of PCM is HGTW. The variations in HGTW for simple building and building + RT-27 are illustrated in Figure 6.
The amount of thermal energy entering the building interior through the wall depends on the number of thermal layers of the wall as well as thermal resistance of the layers. Less thermal conductivity is more desirable for layers because thermal energy faces more barriers to entering the interior space. However, as shown in Figure 6, for a building that uses RT-27 inside walls/ceiling, less thermal energy is exchanged which is very acceptable.
Although Figure 6 proved that RT-27, as a thermal barrier, reduces the thermal energy, but the amount of thermal energy reduction is more important. In the previous section, it was mentioned that PCM does not affect the thermal energy caused by occupancy, lighting and equipment and only changes HGTW. Figure 7A reports the effect of RT-27 on HGTW and it is clear that this parameter decreased by 22.06%.
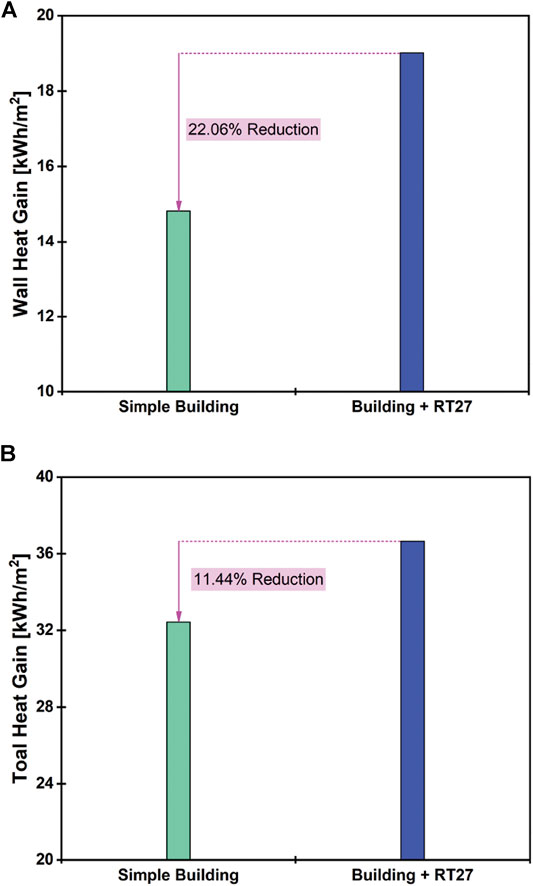
FIGURE 7. PCM efficacy on building energy usage in two conditions. (A): wall heat gain, (B): total heat gain.
If the total entering thermal energy to the building is examined, it can be seen that the amount of reduction is changed from 22.06 to 11.44%.
The incoming thermal energy rises the building temperature over time. An air conditioning unit must be used to regulate the temperature. In this study, as shown in Figure 1, the system of fan coil + absorption chiller is used to cool the building. Absorption chillers have low electrical power consumption and instead require a lot of thermal energy. In this study, chilled water is produced at 6.6°C. Chilled water enters the building through the fan coil and absorbs the room's thermal energy to cool it. The mass flow rate of the chilled water depends on the total amount of room thermal energy and obtained from
The power exchanged in the evaporator is determined by the total heat gain. Energy consumption in the absorber is also determined according to COP value. Neglecting the energy consumption in the pumps, the energy consumption in the condenser can be obtained. The changes in energy consumption are shown in Figure 9.
A cooling tower is needed to dissipate energy in the condenser. Of course, the wet-bulb ambient temperature should always be taken into account in the calculations to ensure the accuracy of the results. The cooling tower outlet water temperature should be greater than the ambient wet-bulb temperature. Figure 10 shows that this criterion meets in this study.
Another important parameter is to check the actual power of the chiller, which is usually expressed in terms of refrigeration ton. By selecting a chiller with a nominal refrigeration ton of 40, the actual power of the chiller changes as shown in Figure 11. The parameter of “f” is a variable that indicates how the actual power of the chiller is changing relative to the nominal power. Given the appropriate range of f parameter, a 40 ton of refrigeration is acceptable.
Effects of Nanofluid
Nanoparticles are materials that can improve the thermophysical properties if well dispersed in the fluid and provided they are stable (Esfahani et al., 2018; Keyvani et al., 2018; Asadi et al., 2019; Ranjbarzadeh et al., 2019; Li et al., 2020; Wei et al., 2020). Nanofluids have been studied in many studies (Jahangir et al., 2018; Mahdavi et al., 2019a; Mahdavi et al., 2019b; Giwa et al., 2020a; Giwa et al., 2020b; Tian et al., 2020b; Yan et al., 2020). In this section, the results of two nanoparticles of ZnO and Al2O3 were used to evaluate the effect of nanoparticles. In a study by Arıkan et al. (2018) it was shown that the efficiency increases in the presence of nanoparticles. In the presence of ZnO the efficiency increases but to a lesser extent than that of Al2O3. Figure 12 shows that both nanoparticles can increase the rate of heat absorption by improving efficiency.
Using a solar system reduces energy consumption in the absorption chiller. Since the generator section requires a lot of thermal energy, a part of which can be provided by the solar system. Figure 13 shows the amount of energy-saving by using the solar system over time. Note that in this case, the inside of the collector is filled with water. The oscillation of the amount of saving energy is attributed to the solar energy oscillation.
To examine the effect of
Conclusion
In this study, the thermal behavior of a building impregnated with PCM was investigated. In July, with a temperature range of 25–40°C, PCM of RT-27 was added to the walls. An absorption chiller + fan coil system was utilized to cool the building. Nanofluid-filled collectors were used to provide thermal energy in the absorption chiller. The most important results were as follows:
• The PCM acted like a heat sink when installed in the wall and reduced the heat transfer through the walls by 22.06%. Taking into account the heat gains of occupancy, lighting and equipment, it was found that the total heat gain decreased by 11.44%.
• The combination of solar collector and absorption chiller reduced energy demand by 428 kWh.
• The energy-saving was affected by the addition of ZnO and
Data Availability Statement
The original contributions presented in the study are included in the article/Supplementary Material, further inquiries can be directed to the corresponding author.
Author Contributions
AA, AM, JM, and GC wrote the manuscript. AA, AM, JM, and G.C. provided critical feedback and helped shape the research, analysis, and manuscript. All authors discussed the results and commented on the manuscript.
Funding
The authors would like to express their gratitude to the Ministry of Education and Deanship of Scientific Research, Najran University, Najran, Kingdom of Saudi Arabia for their financial and technical support under code number NU/ ESCI / 17/ 053. We acknowledge support by the the German Research Foundation (DFG).
Conflict of Interest
The authors declare that the research was conducted in the absence of any commercial or financial relationships that could be construed as a potential conflict of interest.
References
Ahangari, M., and Maerefat, M. (2019). An Innovative PCM System for thermal comfort Improvement and Energy Demand Reduction in Building under Different Climate Conditions. Sust. Cities Soc. 44, 120–129. doi:10.1016/j.scs.2018.09.008
Ahmad, T., Chen, H., Guo, Y., and Wang, J. (2018). A Comprehensive Overview on the Data Driven and Large Scale Based Approaches for Forecasting of Building Energy Demand: A Review. Energy and Buildings 165, 301–320. doi:10.1016/j.enbuild.2018.01.017
Ahmadi, G., Toghraie, D., and Akbari, O. A. (2017). Efficiency Improvement of a Steam Power Plant through Solar Repowering. Ijex 22, 158–182. doi:10.1504/ijex.2017.083015
Arıkan, E., Abbasoğlu, S., and Gazi, M. (2018). Experimental Performance Analysis of Flat Plate Solar Collectors Using Different Nanofluids. Sustainability 10, 1794.
Asadi, A., Pourfattah, F., Miklós Szilágyi, I., Afrand, M., Żyła, G., Seon Ahn, H., et al. (2019). Effect of Sonication Characteristics on Stability, Thermophysical Properties, and Heat Transfer of Nanofluids: A Comprehensive Review. Ultrason. Sonochem. 58, 104701. doi:10.1016/j.ultsonch.2019.104701
ASHRAEAmerican Society of Heating, R., and Engineers, A-C. (2016). ASHRAE Handbook-HVAC Systems and Equipment. IP Edition. ASHRAE.
Azimi Fereidani, N., Rodrigues, E., and Gaspar, A. R. (2021). A Review of the Energy Implications of Passive Building Design and Active Measures under Climate Change in the Middle East. J. Clean. Prod. 305, 127152. doi:10.1016/j.jclepro.2021.127152
Ben Romdhane, S., Amamou, A., Ben Khalifa, R., Saïd, N. M., Younsi, Z., and Jemni, A. (2020). A Review on thermal Energy Storage Using Phase Change Materials in Passive Building Applications. J. Building Eng. 32, 101563. doi:10.1016/j.jobe.2020.101563
Esfahani, N. N., Toghraie, D., and Afrand, M. (2018). A New Correlation for Predicting the thermal Conductivity of ZnO–Ag (50%–50%)/water Hybrid Nanofluid: An Experimental Study. Powder Tech. 323, 367–373.
Gagliano, A., Aneli, S., and Nocera, F. (2019). Analysis of the Performance of a Building Solar thermal Facade (BSTF) for Domestic Hot Water Production. Renew. Energ. 142, 511–526. doi:10.1016/j.renene.2019.04.102
Ghaffarkhah, A., Afrand, M., Talebkeikhah, M., Sehat, A. A., Moraveji, M. K., Talebkeikhah, F., et al. (2020). On Evaluation of Thermophysical Properties of Transformer Oil-Based Nanofluids: A Comprehensive Modeling and Experimental Study. J. Mol. Liquids 300, 112249. doi:10.1016/j.molliq.2019.112249
Gholipour, S., Afrand, M., and Kalbasi, R. (2020). Improving the Efficiency of Vacuum Tube Collectors Using New Absorbent Tubes Arrangement: Introducing Helical Coil and Spiral Tube Adsorbent Tubes. Renew. Energ. 151, 772–781. doi:10.1016/j.renene.2019.11.068
Gholipour, S., Afrand, M., and Kalbasi, R. (2021). Introducing Two Scenarios to Enhance the Vacuum U-Tube Solar Collector Efficiency by Considering Economic Criterion. J. Taiwan Inst. Chem. Eng. doi:10.1016/j.jtice.2021.04.015
Giwa, S. O., Sharifpur, M., Ahmadi, M. H., and Meyer, J. P. (2020). A Review of Magnetic Field Influence on Natural Convection Heat Transfer Performance of Nanofluids in Square Cavities. J. Therm. Anal. Calorim. doi:10.1007/s10973-020-09832-3
Giwa, S. O., Sharifpur, M., Ahmadi, M. H., and Meyer, J. P. (2020). Magnetohydrodynamic Convection Behaviours of Nanofluids in Non‐square Enclosures: A Comprehensive Review. Math. Methods Appl. Sci. doi:10.1002/mma.6424
Jahangir, M. H., Ghazvini, M., Pourfayaz, F., Ahmadi, M. H., Sharifpur, M., and Meyer, J. P. (2018). Numerical Investigation into Mutual Effects of Soil thermal and Isothermal Properties on Heat and Moisture Transfer in Unsaturated Soil Applied as thermal Storage System. Numer. Heat Transfer, A: Appl. 73, 466–481. doi:10.1080/10407782.2018.1449518
Jahangiri, M., Ghaderi, R., Haghani, A., and Nematollahi, O. (2016). Finding the Best Locations for Establishment of Solar-Wind Power Stations in Middle-East Using GIS: A Review. Renew. Sust. Energ. Rev. 66, 38–52. doi:10.1016/j.rser.2016.07.069
Jahangiri, M., Haghani, A., Mostafaeipour, A., Khosravi, A., and Raeisi, H. A. (2019). Assessment of Solar-Wind Power Plants in Afghanistan: A Review. Renew. Sust. Energ. Rev. 99, 169–190. doi:10.1016/j.rser.2018.10.003
Kalbasi, R., Jahangiri, M., Mosavi, A., Jalaladdin Hosseini Dehshiri, S., Shahabaddin Hosseini Dehshiri, S., Ebrahimi, S., et al. (2021). Finding the Best Station in Belgium to Use Residential-Scale Solar Heating, One-Year Dynamic Simulation with Considering All System Losses: Economic Analysis of Using ETSW. Sustainable Energ. Tech. Assessments 45, 101097. doi:10.1016/j.seta.2021.101097
Kang, E. C., Riederer, P., Yoo, S. Y., and Lee, E. J. (2013). New Approach to Evaluate the Seasonal Performance of Building Integrated Geothermal Heat Pump System. Renew. Energ. 54, 51–54. doi:10.1016/j.renene.2012.08.067
Keyvani, M., Afrand, M., Toghraie, D., and Reiszadeh, M. (2018). An Experimental Study on the thermal Conductivity of Cerium Oxide/ethylene Glycol Nanofluid: Developing a New Correlation. J. Mol. Liquids 266, 211–217. doi:10.1016/j.molliq.2018.06.010
Kulkarni, D. P., Das, D. K., and Vajjha, R. S. (2009). Application of Nanofluids in Heating Buildings and Reducing Pollution. Appl. Energ. 86, 2566–2573. doi:10.1016/j.apenergy.2009.03.021
Li, Z., Kalbasi, R., Nguyen, Q., and Afrand, M. (2020). Effects of Sonication Duration and Nanoparticles Concentration on thermal Conductivity of Silica-Ethylene Glycol Nanofluid under Different Temperatures: An Experimental Study. Powder Tech. 367, 464–473. doi:10.1016/j.powtec.2020.03.058
Li, Z. X., Al-Rashed, A. A. A. A., Rostamzadeh, M., Kalbasi, R., Shahsavar, A., and Afrand, M. (2019). Heat Transfer Reduction in Buildings by Embedding Phase Change Material in Multi-Layer walls: Effects of Repositioning, Thermophysical Properties and Thickness of PCM. Energ. Convers. Manag. 195, 43–56. doi:10.1016/j.enconman.2019.04.075
Liu, W., Kalbasi, R., and Afrand, M. (2020). Solutions for Enhancement of Energy and Exergy Efficiencies in Air Handling Units. J. Clean. Prod. 257, 120565. doi:10.1016/j.jclepro.2020.120565
Lizana, J., de-Borja-Torrejon, M., Barrios-Padura, A., Auer, T., and Chacartegui, R. (2019). Passive Cooling through Phase Change Materials in Buildings. A Critical Study of Implementation Alternatives. Appl. Energ. 254, 113658. doi:10.1016/j.apenergy.2019.113658
Mahdavi, M., Garbadeen, I., Sharifpur, M., Ahmadi, M. H., and Meyer, J. P. (2019). Study of Particle Migration and Deposition in Mixed Convective Pipe Flow of Nanofluids at Different Inclination Angles. J. Therm. Anal. Calorim. 135, 1563–1575. doi:10.1007/s10973-018-7720-y
Mahdavi, M., Sharifpur, M., Ahmadi, M. H., and Meyer, J. P. (2019). Aggregation Study of Brownian Nanoparticles in Convective Phenomena. J. Therm. Anal. Calorim. 135, 111–121. doi:10.1007/s10973-018-7283-y
Menni, Y., Ghazvini, M., Ameur, H., Kim, M., Ahmadi, M. H., and Sharifpur, M. (2020). Combination of Baffling Technique and High-thermal Conductivity Fluids to Enhance the Overall Performances of Solar Channels. Eng. Comput., 1–22.
Miansari, M., Nazari, M., Toghraie, D., and Akbari, O. A. (2020). Investigating the thermal Energy Storage inside a Double-wall Tank Utilizing Phase-Change Materials (PCMs). J. Therm. Anal. Calorim. 139, 2283–2294. doi:10.1007/s10973-019-08573-2
Moradi, A., Toghraie, D., Isfahani, A. H. M., and Hosseinian, A. (2019). An Experimental Study on MWCNT-Water Nanofluids Flow and Heat Transfer in Double-Pipe Heat Exchanger Using Porous media. J. Therm. Anal. Calorim. 137, 1797–1807. doi:10.1007/s10973-019-08076-0
Mostafaeipour, A., Jahangiri, M., Haghani, A., Dehshiri, S. J. H., Dehshiri, S. S. H., Issakhov, A., et al. (2020). Statistical Evaluation of Using the New Generation of Wind Turbines in South Africa. Energ. Rep. 6, 2816–2827. doi:10.1016/j.egyr.2020.09.035
Mostafaeipour, A., Rezaei, M., Jahangiri, M., and Qolipour, M. (2019). Feasibility Analysis of a New Tree-Shaped Wind Turbine for Urban Application: A Case Study. Energ. Environ. 31, 1230–1256. doi:10.1177/0958305x19888878
Mustafa, J., Alqaed, S., and Kalbasi, R. (2021). Challenging of Using CuO Nanoparticles in a Flat Plate Solar Collector- Energy Saving in a Solar-Assisted Hot Process Stream. J. Taiwan Inst. Chem. Eng. doi:10.1016/j.jtice.2021.04.003
Nariman, A., Kalbasi, R., and Rostami, S. (2020). Sensitivity of AHU Power Consumption to PCM Implementation in the wall-considering the Solar Radiation. J. Therm. Anal. Calorim.
Nundy, S., Mesloub, A., Alsolami, B. M., and Ghosh, A. (2021). Electrically Actuated Visible and Near-Infrared Regulating Switchable Smart Window for Energy Positive Building: A Review. J. Clean. Prod. 301, 126854. doi:10.1016/j.jclepro.2021.126854
Nwaji, G. N., Okoronkwo, C. A., Ogueke, N. V., and Anyanwu, E. E. (2019). Hybrid Solar Water Heating/nocturnal Radiation Cooling System I: A Review of the Progress, Prospects and Challenges. Energy and Buildings 198, 412–430. doi:10.1016/j.enbuild.2019.06.017
Palmero-Marrero, A. I., Gomes, F., Sousa, J., and Oliveira, A. C. (2020). Energetic Analysis of a thermal Building Using Geothermal and Solar Energy Sources. Energ. Rep. 6, 201–206. doi:10.1016/j.egyr.2020.11.268
Parsa, S. M., Javadi Y, D., Rahbar, A., Majidniya, M., Aberoumand, S., Amidpour, Y., et al. (2019). Experimental Assessment on Passive Solar Distillation System on Mount Tochal at the Height of 3964 m: Study at High Altitude. Desalination 466, 77–88. doi:10.1016/j.desal.2019.05.010
Parsa, S. M., Javadi Y, D., Rahbar, A., Majidniya, M., Salimi, M., Amidpour, Y., et al. (2020). Experimental Investigation at a summit above 13,000 Ft on Active Solar Still Water Purification Powered by Photovoltaic: A Comparative Study. Desalination 476, 114146. doi:10.1016/j.desal.2019.114146
Parsa, S. M. (2021). Reliability of thermal Desalination (Solar Stills) for Water/wastewater Treatment in Light of COVID-19 (Novel Coronavirus "SARS-CoV-2") Pandemic: What Should Consider? Desalination 512, 115106. doi:10.1016/j.desal.2021.115106
Poon, K. H., Kämpf, J. H., Tay, S. E. R., Wong, N. H., and Reindl, T. G. (2020). Parametric Study of URBAN Morphology on Building Solar Energy Potential in Singapore Context. Urban Clim. 33, 100624. doi:10.1016/j.uclim.2020.100624
Ranjbarzadeh, R., Moradikazerouni, A., Bakhtiari, R., Asadi, A., and Afrand, M. (2019). An Experimental Study on Stability and thermal Conductivity of Water/silica Nanofluid: Eco-Friendly Production of Nanoparticles. J. Clean. Prod. 206, 1089–1100. doi:10.1016/j.jclepro.2018.09.205
Sarafraz, M. M., Shadloo, M. S., Tian, Z., Tlili, I., Alkanhal, T. A., Safaei, M. R., et al. (2019). Convective Bubbly Flow of Water in an Annular Pipe: Role of Total Dissolved Solids on Heat Transfer Characteristics and Bubble Formation. Water 11, 1566. doi:10.3390/w11081566
Saxena, R., Rakshit, D., and Kaushik, S. C. (2020). Experimental Assessment of Phase Change Material (PCM) Embedded Bricks for Passive Conditioning in Buildings. Renew. Energ. 149, 587–599. doi:10.1016/j.renene.2019.12.081
Shahsavar Goldanlou, A., Kalbasi, R., and Afrand, M. (2020). Energy Usage Reduction in an Air Handling Unit by Incorporating Two Heat Recovery Units. J. Building Eng. 32, 101545. doi:10.1016/j.jobe.2020.101545
Soltani, F., Toghraie, D., and Karimipour, A. (2020). Experimental Measurements of thermal Conductivity of Engine Oil-Based Hybrid and Mono Nanofluids with Tungsten Oxide (WO3) and MWCNTs Inclusions. Powder Tech. 371, 37–44. doi:10.1016/j.powtec.2020.05.059
Song, Y.-l., Darani, K. S., Khdair, A. I., Abu-Rumman, G., and Kalbasi, R. (2021). A Review on Conventional Passive Cooling Methods Applicable to Arid and Warm Climates Considering Economic Cost and Efficiency Analysis in Resource-Based Cities. Energ. Rep. 7, 2784–2820. doi:10.1016/j.egyr.2021.04.056
Strandberg, R., and Das, D. K. (2010). Influence of Temperature and Properties Variation on Nanofluids in Building Heating. Energ. Convers. Manag. 51, 1381–1390. doi:10.1016/j.enconman.2010.01.006
Tian, M.-W., Parikhani, T., Jermsittiparsert, K., and Ashraf, M. A. (2020). Exergoeconomic Optimization of a New Double-Flash Geothermal-Based Combined Cooling and Power (CCP) System at Two Different Cooling Temperatures Assisted by Boosters. J. Clean. Prod. 261, 120921. doi:10.1016/j.jclepro.2020.120921
Tian, X.-X., Kalbasi, R., Jahanshahi, R., Qi, C., Huang, H.-L., and Rostami, S. (2020). Competition between Intermolecular Forces of Adhesion and Cohesion in the Presence of Graphene Nanoparticles: Investigation of Graphene Nanosheets/ethylene Glycol Surface Tension. J. Mol. Liquids 311, 113329. doi:10.1016/j.molliq.2020.113329
Toghraie, D., Karami, A., Afrand, M., and Karimipour, A. (2018). Effects of Geometric Parameters on the Performance of Solar Chimney Power Plants. Energy 162, 1052–1061. doi:10.1016/j.energy.2018.08.086
Wei, H., Afrand, M., Kalbasi, R., Ali, H. M., Heidarshenas, B., and Rostami, S. (2020). The Effect of Tungsten Trioxide Nanoparticles on the thermal Conductivity of Ethylene Glycol under Different Sonication Durations: An Experimental Examination. Powder Tech. 374, 462–469. doi:10.1016/j.powtec.2020.07.056
Yan, S.-R., Kalbasi, R., Nguyen, Q., and Karimipour, A. (2020). Sensitivity of Adhesive and Cohesive Intermolecular Forces to the Incorporation of MWCNTs into Liquid Paraffin: Experimental Study and Modeling of Surface Tension. J. Mol. Liquids 310, 113235. doi:10.1016/j.molliq.2020.113235
Keywords: saving-energy, building, pcm, solar collector, nanofluids
Citation: Amirahmad A, Maglad AM, Mustafa J and Cheraghian G (2021) Loading PCM Into Buildings Envelope to Decrease Heat Gain-Performing Transient Thermal Analysis on Nanofluid Filled Solar System. Front. Energy Res. 9:727011. doi: 10.3389/fenrg.2021.727011
Received: 17 June 2021; Accepted: 01 July 2021;
Published: 20 July 2021.
Edited by:
Mohsen Sharifpur, University of Pretoria, South AfricaReviewed by:
Kashif Irshad, King Fahd University of Petroleum and Minerals, Saudi ArabiaBasharat Jamil, Rey Juan Carlos University, Spain
Copyright © 2021 Amirahmad, Maglad, Mustafa and Cheraghian. This is an open-access article distributed under the terms of the Creative Commons Attribution License (CC BY). The use, distribution or reproduction in other forums is permitted, provided the original author(s) and the copyright owner(s) are credited and that the original publication in this journal is cited, in accordance with accepted academic practice. No use, distribution or reproduction is permitted which does not comply with these terms.
*Correspondence: Goshtasp Cheraghian, goshtasp.cheraghian@tu-braunschweig.de