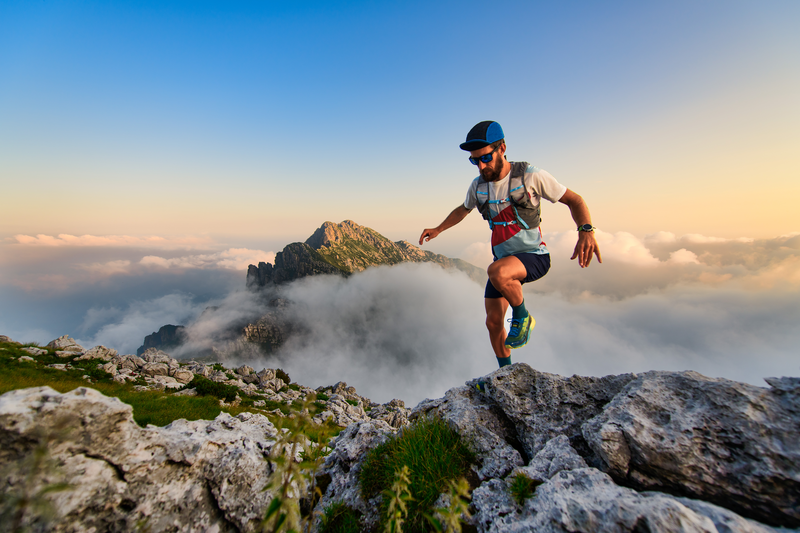
95% of researchers rate our articles as excellent or good
Learn more about the work of our research integrity team to safeguard the quality of each article we publish.
Find out more
ORIGINAL RESEARCH article
Front. Energy Res. , 23 June 2021
Sec. Process and Energy Systems Engineering
Volume 9 - 2021 | https://doi.org/10.3389/fenrg.2021.710626
This article is part of the Research Topic Enhancing Heat Transfer by Using Nanofluid to Improve the Efficiency of Thermal Systems View all 7 articles
In the Jeddah climate region, a lot of energy is assigned to the air handling unit (AHU) sector, which should be reduced by using energy-efficient solutions. As the air passes through the cooling coil, a lot of energy is consumed to reduce the temperature along with humidity so that if the air is precooled in the previous stages, energy consumption in this energy-intensive section will be diminished. Using the coldness of the return air in the heat recovery unit (HRU), the incoming air is precooled. Based on the thermodynamic calculations, in June, July, and August, the cooling coil power demand reduces by 11.6, 13.3, and 12%, respectively. In summer, owing to using HRU, an energy-saving by 76.08 MWh is achieved (12.34% reduction in energy demand). By the incorporation of the solar collectors in the AHU, heating coil demand diminishes by 1,206, 1,399, and 1,367 kWh in June, July, and August, respectively. To improve the solar-assisted AHU effectiveness, the MWCNT nanoparticles are injected into the collectors, and it is found that the saving-energy capability improves by 17.7% using MWCNT-water at 0.1 vol.%.
Residential buildings, along with commercial buildings, are heavily involved in
Almitani et al. (2021) added an energy recovery unit
Kalbasi et al. (2020a), through developing a program in EES software, examined the sensitivity of ERU to the extent of humidity in ambient. They added an ERU to the AHU and considered different scenarios. In the first scenario, they increased
In another study (Kalbasi et al., 2020b) the authors studied the exergy performance of an AHU + ERU. The major irreversibility corresponded to the heating coil (11.47 kW) while the minimum irreversibility (1.067 kW) was through the mixing box. Owing to using ERU, irreversibility diminished by 2.87 kWh. This is very acceptable because an 8.7-percent reduction in irreversibility improves the second efficiency by 4.6%.
In a numerical/experimental study, Ribé et al. (2019) examined the effects of relative humidity on the performance of ERU. Since in the heat recovery unit
In a similar study, Yari et al. (2019) examined the effects of
Zheng et al. (2020) added two ERUs in an AHU and focused on
Controllers with the apparent commands can reduce
In this study, two strategies are used to reduce AHU energy consumption
The description of the solar system integrated with AHU is shown in Figure 1. In general, AHUs have two main purposes: 1—providing the suitable temperature
As shown in Figure 1, the ambient fresh air enters the AHU with the thermodynamic properties of
If the task of the AHU is only to supply
where
where
After mixing, the air enters CC. After leaving CC, the properties are determined as follows:
Because through passing CC, both humidity and temperature decrease, so
The conditions for the supply air depend on
Equations 9 and 10 reveal that the supply air properties are influenced by
In this study, using a solar hot water supply
where
FIGURE 4. The efficiency of the solar collector by using MWCNT nanoparticles (Eltaweel and Abdel-Rehim, 2019).
In this study, it is tried to reduce
In summer, outdoor air energy
Figure 7 illustrates the cooling coil power changes for AHU and AHU + HRU. It is clear that in June, the values of
A lower
In the second scenario, the focus is on the heating coil. Many studies showed the effectiveness of using solar energy (Ahmadi et al., 2017a; Ahmadi et al., 2017b; Ahmadi et al., 2018; Dabiri et al., 2018; Gholipour et al., 2020). In the heating coil, the temperature rises during a process in which the humidity ratio remains constant. The inlet temperature in the heating coil is preset according to the cooling coil outlet. On the other hand, the outlet temperature of the heating coil is also determined according to the room conditions using Eq. 9. Therefore, its input and output are predetermined. Generally, in AHUs, the heating coil supplies its energy through either a boiler or an electric heater. In this study, the heating coil power demand is supplied from a solar system. As mentioned in the previous section, the solar system consists of several collectors and a storage tank equipped with an electric heater. If solar energy cannot heat the water in the tank, the auxiliary heater must heat the water. In this study, in addition to the auxiliary heater, the tank is equipped with two sensors. The first sensor is located exactly at the top of the tank and the second sensor is located in the middle of the tank. The sensors function in such a way that they can keep the temperature in the whole tank in the range of 35–40°C. Since the power of the heating coil is obtained from
Owing to the temperature range of
For comparison, it is assumed that AHU provides heating power in the heating coil through an electric heater. Thermodynamic calculations affirm that accumulated heating power
Owing to integrating a solar system with AHU,
It seems that by increasing the number of collectors in parallel arrangement (increasing the collector area), it is possible to strengthen the ability of energy-saving. As the area increases (as shown in Figure 12), the saving energy intensifies. In June, the saving energy increases by 41.48% (from 850 kWh to 1,206 kWh) as the area rises from 5 to 10 m2. This figure for July and August is 44.22 and 43.9%. This implies that there is no linear relationship between the collector area and energy-saving content. Note that this figure in summer is 43.4% (with increasing area from 5 to 10
Nanoparticles are another technique for improving the collectors’ efficiency (Qu et al., 2019; Mahmoudi et al., 2020; Alawi et al., 2021; Sadeghi et al., 2021). These materials have widely been examined by many researchers (Osman et al., 2019; Yan et al., 2020; Aghakhani et al., 2020; Eshgarf et al., 2021; Mahdavi et al., 2020; Nguyen et al., 2020; Yan et al., 2020). Nanoparticles can improve heat transfer within the collector (Muhammad et al., 2016; Tong et al., 2019; Elcioglu et al., 2020; Yan et al., 2020; Gholipour et al., 2021; Mustafa et al., 2021). As shown in Figure 5, the efficiency for the MWCNT-based collector is higher. Therefore, it is expected that MWCNTs will increase the energy-saving content. In Figure 13, it can be seen that for a collector filled with water, the energy-saving is 3,972 kWh. This figure for collector filled with MWCNTs (at 0.01 vol.%) is 4,170 kWh. This indicates that using MWCNT is recommended owing to a 5% enhancement in energy-saving.
The positive effects of MWCNT increase as the nanoparticle concentration intensifies. Adding further MWCNTs leads to more enhancement in energy-saving. At 0.05 and 0.1 vol.%, the energy-saving is 4,520 and 4,675 kWh which is equivalent to 13.8 and 17.7% enhancement.
Considering the 40 and 35% contributions of the building sector in energy consumption and greenhouse gas emissions, two techniques were used to reduce both the parameters. In the first technique, a unit heat recovery (HRU) was used to reduce energy demand in the cooling coil, while in the second technique, the energy consumption in the heating coil was reduced by focusing on solar energy. By developing energy and continuity equations in individual sections, transient thermal analysis of the AHU was performed. The main results were:
❖ First technique (installing HRU):
1. By installing HRU, the hotness from the fresh air was transferred to the return air and consequently, the fresh air was precooled. Owing to entering with a lower temperature into the cooling coil, the power demand reduced by 76.09 MWh in summer which is equivalent to a 12.34% reduction.
❖ Second technique (using solar collectors + stratified storage tank):
1. In summer, the task of the heating coil is to adjust the thermodynamic conditions of the supply air. With the installation of the solar system, energy consumption was reduced by 3,972 kWh in June + July + August which is equivalent to a 6.41-percent reduction.
2. To boost the solar collector effectiveness, MWCNT nanoparticles were loaded into the water and it was found that the energy-saving potential increased by 17.7% (from 3,972 to 4,675 kWh).
The original contributions presented in the study are included in the article/Supplementary Material, further inquiries can be directed to the corresponding author.
YK: Writing, Methodology, Software.
This project was funded by the Deanship of Scientific Research (DSR) at King Abdulaziz University, Jeddah, under Grant Nos. (135-007-D1433).
The author declares that the research was conducted in the absence of any commercial or financial relationships that could be construed as a potential conflict of interest.
The author, therefore, acknowledge with thanks DSR technical and financial support.
Aghakhani, S., Pordanjani, A. H., Afrand, M., Sharifpur, M., and Meyer, J. P. (2020). Natural Convective Heat Transfer and Entropy Generation of Alumina/water Nanofluid in a Tilted Enclosure with an Elliptic Constant Temperature: Applying Magnetic Field and Radiation Effects. Int. J. Mech. Sci. 174, 105470. doi:10.1016/j.ijmecsci.2020.105470
Ahmadi, G., Toghraie, D., and Akbari, O. A. (2017). Efficiency Improvement of a Steam Power Plant through Solar Repowering. Ijex 22 (2), 158–182. doi:10.1504/ijex.2017.083015
Ahmadi, G., Toghraie, D., and Akbari, O. A. (2017). Solar Parallel Feed Water Heating Repowering of a Steam Power Plant: A Case Study in Iran. Renew. Sustain. Energ. Rev. 77, 474–485. doi:10.1016/j.rser.2017.04.019
Ahmadi, G., Toghraie, D., and Akbari, O. A. (2018). Technical and Environmental Analysis of Repowering the Existing CHP System in a Petrochemical Plant: A Case Study. Energy 159, 937–949. doi:10.1016/j.energy.2018.06.208
Ahmadi, M. H., Alhuyi Nazari, M., Sadeghzadeh, M., Pourfayaz, F., Ghazvini, M., Ming, T., et al. (2019). Thermodynamic and Economic Analysis of Performance Evaluation of All the thermal Power Plants: A Review. Energy Sci Eng 7 (1), 30–65. doi:10.1002/ese3.223
Alawi, O. A., Kamar, H. M., Mallah, A. R., Mohammed, H. A., Kazi, S. N., Che Sidik, N. A., et al. (2021). Nanofluids for Flat Plate Solar Collectors: Fundamentals and Applications. J. Clean. Prod. 291, 125725. doi:10.1016/j.jclepro.2020.125725
Almitani, K. H., Abu-Hamdeh, N. H., Golmohammadzadeh, A., and Javaheran Yazd, M. (2021). The Effect of Various Forms of the Tube Cross on the Energetic and Exergetic Analysis of Helical Tube in Tube Heat Exchangers of an AHU with Energy Recovery Unit in Heating Mode: Injection of Vapor/water Particles. J. Therm. Anal. Calorim. doi:10.1007/s10973-020-10546-9
ASHRAE American Society of Heating, R., and Engineers, A-C. (2016). ASHRAE handbook-HVAC Systems and Equipment. IP Edition. Georgia: ASHRAE.
Attia, M. E. H., Karthick, A., Manokar, A. M., Driss, Z., Sathyamurthy, R., and Sharifpur, M. (2020). Sustainable Potable Water Production from Conventional Solar Still during the winter Season at Algerian Dry Areas: Energy and Exergy Analysis. J. Therm. Anal. Calorim., 1–11.
Dabiri, S., Khodabandeh, E., Poorfar, A. K., Mashayekhi, R., Toghraie, D., and Abadian Zade, S. A. (2018). Parametric Investigation of thermal Characteristic in Trapezoidal Cavity Receiver for a Linear Fresnel Solar Collector Concentrator. Energy 153, 17–26. doi:10.1016/j.energy.2018.04.025
Dodoo, A. (2020). Primary Energy and Economic Implications of Ventilation Heat Recovery for a Multi-Family Building in a Nordic Climate. J. Building Eng. 31, 101391. doi:10.1016/j.jobe.2020.101391
Eades, W. G. (2018). Energy and Water Recovery Using Air-Handling Unit Condensate from Laboratory HVAC Systems. Sustain. Cities Soc. 42, 162–175. doi:10.1016/j.scs.2018.07.006
EIA, U. (2019). International Energy Outlook 2019: With Projections to 2050 Washington, DC: Office of Energy Analysis.
Elcioglu, E. B., Genc, A. M., Karadeniz, Z. H., Ezan, M. A., and Turgut, A. (2020). Nanofluid Figure-Of-Merits to Assess thermal Efficiency of a Flat Plate Solar Collector. Energ. Convers. Manag. 204, 112292. doi:10.1016/j.enconman.2019.112292
Eltaweel, M., and Abdel-Rehim, A. A. (2019). Energy and Exergy Analysis of a Thermosiphon and Forced-Circulation Flat-Plate Solar Collector Using MWCNT/Water Nanofluid. Case Stud. Therm. Eng. 14, 100416. doi:10.1016/j.csite.2019.100416
Eshgarf, H., Kalbasi, R., Maleki, A., and Shadloo, M. S. (2021). A Review on the Properties, Preparation, Models and Stability of Hybrid Nanofluids to Optimize Energy Consumption. J. Thermal Anal. Calorim. 144 (5), 1959–1983.
Gholipour, S., Afrand, M., and Kalbasi, R. (2020). Improving the Efficiency of Vacuum Tube Collectors Using New Absorbent Tubes Arrangement: Introducing Helical Coil and Spiral Tube Adsorbent Tubes. Renewable Energy 151, 772–781.
Gholipour, S., Afrand, M., and Kalbasi, R. (2021). Introducing Two Scenarios to Enhance the Vacuum U-Tube Solar Collector Efficiency by Considering Economic Criterion. J. Taiwan Inst. Chem. Eng. doi:10.1016/j.jtice.2021.04.015
Hashemi-Tilehnoee, M., Dogonchi, A. S., Seyyedi, S. M., and Sharifpur, M. (2020). Magneto-fluid Dynamic and Second Law Analysis in a Hot Porous Cavity Filled by Nanofluid and Nano-Encapsulated Phase Change Material Suspension with Different Layout of Cooling Channels. J. Energ. Storage 31, 101720. doi:10.1016/j.est.2020.101720
Homod, R. Z. (2014). Assessment Regarding Energy Saving and Decoupling for Different AHU (Air Handling Unit) and Control Strategies in the Hot-Humid Climatic Region of Iraq. Energy 74, 762–774. doi:10.1016/j.energy.2014.07.047
Jamei, M., Ahmadianfar, I., Olumegbon, I. A., Asadi, A., Karbasi, M., Said, Z., et al. (2021). On the Specific Heat Capacity Estimation of Metal Oxide-Based Nanofluid for Energy Perspective - A Comprehensive Assessment of Data Analysis Techniques. Int. Commun. Heat Mass Transfer 123, 105217. doi:10.1016/j.icheatmasstransfer.2021.105217
Jokar, M. A., Ahmadi, M. H., Sharifpur, M., Meyer, J. P., Pourfayaz, F., and Ming, T. (2017). Thermodynamic Evaluation and Multi-Objective Optimization of Molten Carbonate Fuel Cell-Supercritical CO 2 Brayton Cycle Hybrid System. Energ. Convers. Manag. 153, 538–556. doi:10.1016/j.enconman.2017.10.027
Kalbasi, R., Ruhani, B., and Rostami, S. (2020a). Energetic Analysis of an Air Handling Unit Combined with Enthalpy Air-To-Air Heat Exchanger. J. Therm. Anal. Calorim. 139 (4), 2881–2890. doi:10.1007/s10973-019-09158-9
Kalbasi, R., Izadi, F., and Talebizadehsardari, P. (2020b). Improving Performance of AHU Using Exhaust Air Potential by Applying Exergy Analysis. J. Therm. Anal. Calorim. 139 (4), 2913–2923. doi:10.1007/s10973-019-09198-1
Kalbasi, R., Shahsavar, A., and Afrand, M. (2020c). Reducing AHU Energy Consumption by a New Layout of Using Heat Recovery Units. J. Therm. Anal. Calorim. 139 (4), 2811–2820. doi:10.1007/s10973-019-09070-2
Liu, W., Kalbasi, R., and Afrand, M. (2020). Solutions for Enhancement of Energy and Exergy Efficiencies in Air Handling Units. J. Clean. Prod. 257, 120565. doi:10.1016/j.jclepro.2020.120565
Mahdavi, M., Sharifpur, M., Meyer, J. P., and Chen, L. (2020). Thermal Analysis of a Nanofluid Free Jet Impingement on a Rotating Disk Using Volume of Fluid in Combination with Discrete Modelling. Int. J. Therm. Sci. 158, 106532. doi:10.1016/j.ijthermalsci.2020.106532
Mahmoudi, A., Fazli, M., Morad, M. R., and Gholamalizadeh, E. (2020). Thermo-hydraulic Performance Enhancement of Nanofluid-Based Linear Solar Receiver Tubes with Forward Perforated Ring Steps and Triangular Cross Section; a Numerical Investigation. Appl. Therm. Eng. 169, 114909. doi:10.1016/j.applthermaleng.2020.114909
Muhammad, M. J., Muhammad, I. A., Che Sidik, N. A., and Muhammad Yazid, M. N. A. W., "Thermal Performance Enhancement of Flat-Plate and Evacuated Tube Solar Collectors Using Nanofluid: A Review," Int. Commun. Heat Mass Transfer, vol. 76, pp. 6–15. (2016). doi:10.1016/j.icheatmasstransfer.2016.05.009
Mustafa, J., Alqaed, S., and Kalbasi, R. (2021). Challenging of Using CuO Nanoparticles in a Flat Plate Solar Collector- Energy Saving in a Solar-Assisted Hot Process Stream. J. Taiwan Inst. Chem. Eng. doi:10.1016/j.jtice.2021.04.003
Nguyen, Q., Bahrami, D., Kalbasi, R., and Karimipour, A. (2020). Functionalized Multi‐Walled Carbon Nano Tubes Nanoparticles Dispersed in Water through an Magneto Hydro Dynamic Nonsmooth Duct Equipped with Sinusoidal‐wavy wall: Diminishing Vortex Intensity via Nonlinear Navier-Stokes Equations. Math. Meth Appl. Sci.. doi:10.1002/mma.6528
Noussan, M., Carioni, G., Degiorgis, L., Jarre, M., and Tronville, P. (2017). Operational Performance of an Air Handling Unit: Insights from a Data Analysis. Energ. Proced. 134, 386–393. doi:10.1016/j.egypro.2017.09.579
Osman, S., Sharifpur, M., and Meyer, J. P. (2019). Experimental Investigation of Convection Heat Transfer in the Transition Flow Regime of Aluminium Oxide-Water Nanofluids in a Rectangular Channel. Int. J. Heat Mass Transfer 133, 895–902. doi:10.1016/j.ijheatmasstransfer.2018.12.169
Pacak, A., Jedlikowski, A., Karpuk, M., and Anisimov, S. (2019). Analysis of Power Demand Calculation for Freeze Prevention Methods of Counter-flow Heat Exchangers Used in Energy Recovery from Exhaust Air. Int. J. Heat Mass Transfer 133, 842–860. doi:10.1016/j.ijheatmasstransfer.2018.12.144
Qu, J., Zhang, R., Wang, Z., and Wang, Q. (2019). Photo-thermal Conversion Properties of Hybrid CuO-Mwcnt/h2o Nanofluids for Direct Solar thermal Energy Harvest. Appl. Therm. Eng. 147, 390–398. doi:10.1016/j.applthermaleng.2018.10.094
Ribé, O., Ruiz, R., Quera, M., and Cadafalch, J. (2019). Analysis of the Sensible and Total Ventilation Energy Recovery Potential in Different Climate Conditions. Application to the Spanish Case. Appl. Therm. Eng. 149, 854–861. doi:10.1016/j.applthermaleng.2018.12.076
Sadeghi, G., Pisello, A. L., Nazari, S., Jowzi, M., and Shama, F. (2021). Empirical Data-Driven Multi-Layer Perceptron and Radial Basis Function Techniques in Predicting the Performance of Nanofluid-Based Modified Tubular Solar Collectors. J. Clean. Prod. 295, 126409. doi:10.1016/j.jclepro.2021.126409
Shahsavar Goldanlou, A., Kalbasi, R., and Afrand, M. (2020). Energy Usage Reduction in an Air Handling Unit by Incorporating Two Heat Recovery Units. J. Building Eng. 32, 101545. doi:10.1016/j.jobe.2020.101545
Thalib, M. M., Vimala, M., Manokar, A. M., Sathyamurthy, R., Sadeghzadeh, M., and Sharifpur, M. (2021). Energy, Exergy and Economic Investigation of Passive and Active Inclined Solar Still: Experimental Study. J. Therm. Anal. Calorim., 1–12.
Tong, Y., Lee, H., Kang, W., and Cho, H., "Energy and Exergy Comparison of a Flat-Plate Solar Collector Using Water, Al2O3 Nanofluid, and CuO Nanofluid," Appl. Therm. Eng., vol. 159, p. 113959, (2019). doi:10.1016/j.applthermaleng.2019.113959
U.S. Energy Information Administration (). Available at: https://www.eia.gov/totalenergy/data/monthly/2021.
Yan, S.-R., Golzar, A., Sharifpur, M., Meyer, J. P., Liu, D.-H., and Afrand, M. (2020). Effect of U-Shaped Absorber Tube on thermal-hydraulic Performance and Efficiency of Two-Fluid Parabolic Solar Collector Containing Two-phase Hybrid Non-newtonian Nanofluids. Int. J. Mech. Sci. 185, 105832. doi:10.1016/j.ijmecsci.2020.105832
Yan, S.-R., Kalbasi, R., Nguyen, Q., and Karimipour, A. (2020). Sensitivity of Adhesive and Cohesive Intermolecular Forces to the Incorporation of MWCNTs into Liquid Paraffin: Experimental Study and Modeling of Surface Tension. J. Mol. Liq., 113235.
Yari, M., Kalbasi, R., and Talebizadehsardari, P. (2019). Energetic-exergetic Analysis of an Air Handling Unit to Reduce Energy Consumption by a Novel Creative Idea. Int. J. Numer. Methods Heat Fluid Flow 29 (10), 3959–3975. doi:10.1108/HFF-09-2018-0524
Keywords: air handling unit, solar collector, energy-saving, nanofluid, heat recovery
Citation: Khetib Y (2021) Transient Thermal Analysis of a Solar-Assisted AHU by Focusing on Heat Recovery and Nanoparticles: Jeddah Climate Zone. Front. Energy Res. 9:710626. doi: 10.3389/fenrg.2021.710626
Received: 16 May 2021; Accepted: 26 May 2021;
Published: 23 June 2021.
Edited by:
Mohsen Sharifpur, University of Pretoria, South AfricaReviewed by:
Houman Yarmand, Delft University of Technology, NetherlandsCopyright © 2021 Khetib. This is an open-access article distributed under the terms of the Creative Commons Attribution License (CC BY). The use, distribution or reproduction in other forums is permitted, provided the original author(s) and the copyright owner(s) are credited and that the original publication in this journal is cited, in accordance with accepted academic practice. No use, distribution or reproduction is permitted which does not comply with these terms.
*Correspondence: Yacine Khetib, eWtoZXRpYkB5YWhvby5jb20=, eWtoYXRlYkBrYXUuZWR1LnNh
Disclaimer: All claims expressed in this article are solely those of the authors and do not necessarily represent those of their affiliated organizations, or those of the publisher, the editors and the reviewers. Any product that may be evaluated in this article or claim that may be made by its manufacturer is not guaranteed or endorsed by the publisher.
Research integrity at Frontiers
Learn more about the work of our research integrity team to safeguard the quality of each article we publish.