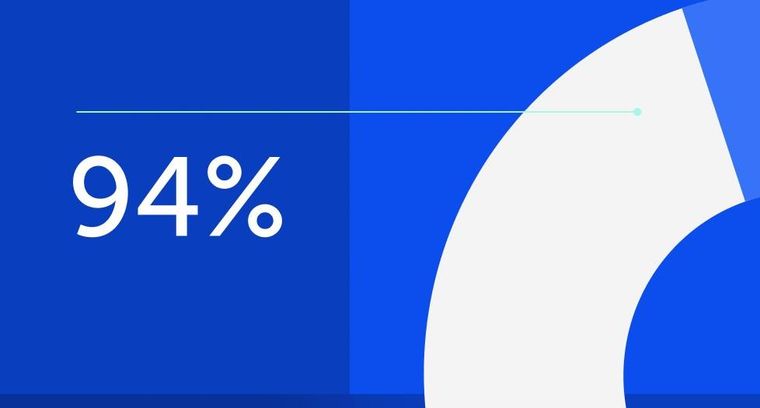
94% of researchers rate our articles as excellent or good
Learn more about the work of our research integrity team to safeguard the quality of each article we publish.
Find out more
ORIGINAL RESEARCH article
Front. Energy Res., 17 December 2021
Sec. Sustainable Energy Systems
Volume 9 - 2021 | https://doi.org/10.3389/fenrg.2021.701719
This article is part of the Research TopicSustainable Energy Systems with Policies in ChinaView all 23 articles
Coal is a major source of energy in China. Quantifying China’s coal supply sustainability is essential to track China’s efforts towards sustainable development and achieve carbon neutrality goals. In this research, in addition to availability, economic sustainability, environmental sustainability and technological sustainability, we specially considered health and security, and transport sustainability of China’s coal supply. We select 19 indicators from the above six dimensions to build a coal supply sustainability index and construct a novel optimized comprehensive evaluation model with level difference maximization to evaluate China’s coal supply sustainability. The results showed that the policies issued by the Chinese government have effectively improved coal supply sustainability. China’s coal supply sustainability level has improved significantly, with the figure nearly doubling from 0.338 in 2000 to 0.7004 in 2019. To improve the sustainability of China’s coal supply further fundamentally, it is still necessary to improve energy diversification. Since phasing out China’s coal reliance requires considerable time, the Chinese government needs to introduce more positive and effective policies to such as increase the research and development support for carbon capture, utilization and storage technology, etc. to improve the sustainability of coal supply. The results of this research presented in this paper will have reference value for both promoting the sustainable development of China and other coal-consuming countries in the world.
Climate change is regarded as one of the greatest challenges that human society is facing in the 21st century. Facing increasingly severe climate situation, the Paris Agreement in 2016 proposed to control the global temperature rise within 2°C comparing with the level before industrialization, and do utmost to limit within 1.5°C (Wei et al., 2020). China aims to reach CO2 emissions peak before 2030 and achieve carbon neutrality before 2060, Chinese President Xi Jinping said to United Nations General Assembly on September 22nd in 2020 (Wang and Zhang, 2020). Carbon neutrality has great significance in improving the ecological environment, coping with climate change, and promoting high-quality development.
Coal is the foundation of China’s energy security, as well as the key to achieve carbon neutrality. Up to now, the quantity of China’s carbon emissions accounts for 30% of global total and China generates more than 50% global coal-fired power (Oberschelp et al., 2019; Duan et al., 2021; Cui et al., 2021; Oberschelp et al., 2019; Cui et al., 2021; Duan et al., 2021). Although many policies has adopted to limit coal consumption for years, Chinese government has also imposed stricter requirements on coal consumption control after the carbon neutral target was put forward. However, China will not eliminate coal in the short term and coal will still play an important role in ensuring China’s energy security for a long period of time in the future (Zhang et al., 2020). Over the years, the Chinese government has put forward a number of measures such as the Guidance on Deepening the Reform of the Coal Marke to ensure the safety of coal supply (Yang et al., 2018). In July 2021, the National Development and Reform Commission held a special meeting on the establishment of a long-term coal supply guarantee mechanism, in this meeting the Chinese government required all localities and central enterprises to stick to the bottom line thinking, focus on building a long-term coal supply guarantee mechanism, continue to accelerate the construction of government coal reserve facilities, and promote the formation of a coal reserve system with flexible adjustments and strong guarantees. This fully reflects the importance the Chinese government attaches to ensuring the balance of coal supply and demand in China. China has more than 200 new coal-fired power stations planned or under construction that coal-fired power is an important strategic reserve in China’s energy transition (Mallapaty, 2020). To achieve the goal of carbon neutrality, it is necessary to transform the development model of coal industry in order to achieve low-carbon, decarbonized, and clean development, as well as safe and sustainable development. Therefore, studying the sustainability of China’s coal supply is significant in achieving the goal of carbon neutrality to China as well as to the world.
Since the concept of sustainable development was introduced, the issue of sustainable energy has attracted the attention of many organizations and scholars. It has also achieved rich and systematic research results. Coal is an important component of the energy supply system in China and in the world, and has also been the focus of many scholars’ interest over the years. However, compared with the study of energy sustainability, coal supply sustainability research is still relatively lacking. Therefore, this section presents a systematic review of energy sustainability research as well as research on the sustainability issues of coal.
From the perspective of energy sustainability research, the term “sustainable energy” derives from the concept of sustainable development used in the Brantland Commission report (Our Common Future, 1987). “Sustainable development” and “resources” have become the two most common keywords related to the concept of green economy in scientific literature from 1990 (Merino-Saum et al., 2018). Munasinghe (1994) introduced the concept of sustainable energy development. They believe that the implementation of a series of energy supply and demand management policies can ultimately lead to the realization of the sustainable development of energy (sustainable Energy Development, 1995). Following these seminal work, organizations and scholars started focusing on the environmental sustainability of energy security and the relationship between energy security and energy sustainability. The European Commission (2001) stressed the importance of sustainability and environmental concerns related to energy security (Green, 2001). In 2004, the “Global Energy Assessment” published by the United Nations introduced the concept of sustainability into energy security, emphasizing environmental sustainability (Meghan, 2013). (Sovacool et al., 2011) points out that energy security is almost synonymous with energy sustainability (Sovacool et al., 2011). According to the World Energy Council (2013) (Wyman, 2013) energy security, energy equity, and environmental sustainability are the three major challenges to global energy sustainability. With more and more in-depth and extensive research, scholars construct different energy sustainability indexes that incorporate various dimensions to evaluate the sustainability of the energy system in recent years (listed in Table 1).
Table 1 shows that the comprehensive evaluation method is a representative method for scholars to study the sustainability of the energy system. In addition to its environmental sustainability, factors such as equity, efficiency, economy, and society are also included in the study of energy sustainability. Energy technology sustainability, energy ecurity sustainability, and energy development sustainability have all been studied thoroughly by scholars. However, few scholars have thus far considered factors such as health, security, and transportation. Moreover, there is a lack of in-depth research on the sustainability of specific energy systems, such as coal, oil, or natural gas.
From the perspective of coal sustainability research, the concept of coal sustainability rarely concerned scholars and social organisations before 2000. In 2000, while Joyce and Thomson clarified social permission, the broader concept of sustainable development started attracting attention in the mining industry Since then, sustainable development has become the main management objective of the global mining industry. With the deepening of energy sustainability research, scholars started paying attention to the relationship between coal and sustainable development from 2000 to 2010 (Breaking, 2002) (Botin, 2009). Many of these scholars proposed the importance of considering the factors related to sustainable development in the mining sector (Corder et al., 2010). After 2010, more in-depth research on risk management and the social impact on the process of coal mining was done. Risk management and accident prevention in coal mining became the foremost concerns of scholars. (Kowalska, 2014) and (Kemp et al., 2016) both assessed the social risks of the coal mining process and the operation mode of social risk in the coal industry based on a case studies method and a literature review, respectively (Kowalska, 2014; Kemp et al., 2016). (Wang et al., 2013a) used a modified curve-fitting model to forecast China’s coal production capacity (CPC), and analyzed its influence on China’s economy and CO2 emissions. (Yuan et al., 2016) and (Feng et al., 2018) quantify the rational capacity and potential investment of coal power in China, and analyzed their influence on China’s low-carbon energy transition. Wang et al. (2018a) proposes a system dynamic (SD) model to forecast the change of China’s coal production capacity CPC in three scenarios. (Chen et al., 2015) and (Wang et al., 2018b) respectively studied the phased evaluation framework of coal mine safety production and impact mechanism of safe mining (Chen et al., 2015; Wang et al., 2018b). In recent years, the environmental and health losses ascribable to coal mining have also been a foremost topic addressed by scholars. Li and Chen (2018) develops a 30-province energy system optimization model (China TIMES-30P) to simulate China’s Carbon emissions during coal transportation. (Liu et al., 2018) develop an optimization model based on an appropriate index system evaluated the carbon dioxide emissions during coal transportation in China. (Zhang et al., 2018) established Hicks-neutral and Solow-neutral models to assess the coal capacity considering the technical progress, and applied the decoupling index to analyze the effect of coal CU on China’s economic growth. (Liu et al., 2019) evaluated the ecological efficiency of coal mining areas in Shanxi Province based on a DEA model (Liu et al., 2019). (von der Goltz and Barnwal, 2019) used micro-data from approximately 800 mines in 44 developing countries to assess the impact of mining on health and wealth (von der Goltz and Barnwal, 2019). (Wang et al., 2020a) estimated and predicted the health loss of coal workers due to pneumoconiosis in China, while (Rauner et al., 2020) found that coal exports also have an impact on both people’s health and the environment (Wang et al., 2020a; Rauner et al., 2020). Liu X (2021) estimated the potential environmental benefits of the widespread adoption of ULE in the Jing-Jin-Ji Region used atmospheric model (Liu et al., 2021). (Yan et al., 2021) studied the gas temperature and different emission gas concentration in the main combustion zone under different coal mixing ratio (Yan et al., 2021). (Zhu et al., 2021) proposed a new alternative fuel YSI value prediction model (BMKL) by using bayesian multi-core learning method (Zhu et al., 2021).
Existing research mentioned above provides important reference for this study. However, compared with energy sustainability research, coal sustainability still lack of comprehensive and systematic study. First of all, the existing coal supply sustainability research is more focused on specialized research on one or more aspects in social, environmental, safety and health impacts of coal mining. Standard in coal supply sustainability has not been established yet that it is hard to fully reflect the sustainability of coal. Secondly, coal supply is the origin of coal-related sustainable development that there is no comprehensive evaluation for coal supply sustainability. Thus, it is difficult to provide a comprehensive and systematic reference in theory and data for the sustainable development of coal under the constraints of the carbon neutral target. Thirdly, the current evaluation method is also based on a single approach with certain limitations. Therefore, the research goal of this article is to develop a more sustainable methodology to explore the sustainability of China’s coal supply and to further comprehensively evaluate the policy effects and key issues of China’s coal industry, in order to provide scientific guidance for the further improvement of relevant policies of China’s coal industry under the constraints of carbon neutral targets in the future.
Accordingly, the remarkable contributions of this paper can be clearly illustrated as follows: First, we select coal, China’s least sustainable energy source, to evaluate its sustainability. This research perspective is highly innovative and the research results of this study will have important reference value for improving the sustainability of China’s coal system and achieving carbon neutrality goals. Second, we propose a comprehensive evaluation index for the sustainability of China’s coal supply that can reflect China’s coal industry policy objectives more comprehensively. This index could also provide a reference for other countries to evaluate their coal supply sustainability in the future. Third, contrary to the traditional comprehensive evaluation method, this study constructs a new optimized comprehensive evaluation model to assess China’s coal supply sustainability, which can comprehensively reflect the advantages of subjective and objective weights. This model can also serve to solve other multi-objective attribute problems.
China’s coal supply sustainability is a multi-attribute decision-making conundrum; therefore, a comprehensive evaluation index is an important tool to quantify this problem. When utilising an evaluation index, each indicator is first given a certain weight according to its perceived importance. They are then combined to create an index, using an appropriate aggregation technique. However, sustainable development indicators have high complexity and dynamic characteristics. This means that it is difficult to use an original indicator system to track the entire process of energy sustainable development. Further, directly embedding a set of original indicators flexibly into the coal system to assess the sustainability of China’s coal supply system would not be effective either. Therefore, proposing a new indicator system to construct a comprehensive evaluation index that is suitable for China’s coal supply sustainable development is crucial. To obtain more scientifically accurate evaluation results, different from previous scholars’ research, in this paper, we put forward an ‘policy objective analysis—index construction—comprehensive valuation model construction’ method with three stages to perform an analysis (Figure 1).
An index is an important tool for evaluating the effectiveness of policies. The purpose of building China’s coal supply sustainability index is to track the progress of China’s coal supply sustainable development policies in several aspects. It can also become an operational tool to improve China’s coal supply sustainability. Therefore, this study focuses on the consistency of the selected indicators and policy objectives. Considering the process of indicator selection, on the one hand, policy objectives are attached to specific indicators that can be measured by unbiased standards and are determined according to China’s coal industry development policy goals and the country’s actual coal supply process. The goals mentioned include “Guiding Opinions on the High-quality Development of China’s coal industry in the 14th Five-Year Plan,” “research on China’s medium and long-term carbon emission reduction strategy target” and so on. On the other hand, it is also necessary to ensure that international goals are considered when choosing indicators. These include “Transforming our World: The 2030 Agenda for Sustainable Development,” “World energy sustainable development index” and so on (Ang et al., 2015; Radovanović et al., 2017). Thus, the indicators are formulated considering global sustainable development goals. Ultimately, this study proposes the research idea of “overall goal-detailed goals -implementation path-specific indicators.” As part of this research idea, in addition to availability, economic sustainability, environmental sustainability and technological sustainability, we specially considered health and security, and transport sustainability, subdivide the overall goal into six detailed goals: availability, economic sustainability, environmental sustainability, technological sustainability, health and security, transport sustainability. These six detailed goals are summarised into 17 implementation paths to design the China’s coal supply sustainability index (CCSSI) (See Figure 2).
Tracking or interpreting changes in such a vast number of policy goals can be a problem. Furthermore, the choice of the final indicators depends upon both their complexity and ease of use. First, from the perspective of availability, coal resource endowment and coal exploration ability are the key factors affecting the sustainable supply of coal (Stefanova, 2012). The greater the reserve-production ratio, the longer the sustainable supply of coal resources and the stronger the ability to deal with coal supply risks. Additionally, the higher the self-sufficiency rate, the stronger the self-protection ability of coal resources. Coal supply per capita represents the equity of China’s coal supply.
Second, from the perspective of economic sustainability, the stability of the coal price and market concentration is very important to ensure the stability of the coal supply market (Iddrisu and Bhattacharyya, 2015). Specifically, referring to the increase in China’s coal imports in recent years, the influence of the international market on China’s coal market cannot be ignored. Therefore, this study also takes into account the indicator of external coal dependence.
Third, we address environmental sustainability. This dimension aims to reduce the negative impact of coal production and coal use on society and to increase the positive impacts. Coal resources have adverse effects on the environment during both the mining and the consumption process (Wirl, 1995). Both coal intensity and the proportion of coal supply in primary energy consumption determine the impact of coal on the environment (Laponche and Tillerson, 2001). This impact is specifically reflected by indicators such as carbon dioxide emissions, nitrogen oxide emissions, and sulphur dioxide emissions.
Fourth, from the perspective of technological sustainability, successful achievement of the United Nations’ sustainable development goals requires the full use of mineral technologies (Ali et al., 2017). On a national level, government support for sustainability and investment in science and technology can effectively promote national sustainable development (Xu et al., 2020). Moreover, good infrastructure is a prerequisite for stabilising coal supply. Improved coal supply technology, systems, and practices can reduce coal demand, reduce coal intensity, and increase the sustainability level of coal supply. Technologies that improve coking efficiency and reduce the use of coal are regarded as the main policies for improving coal supply sustainability (Kemmler and Spreng, 2007; Hughes, 2009; Wei et al., 2018).
Fifth, regarding health and safety, this dimension emphasises the impact of the coal mining process on the health of workers, or production safety. Representative indicators are: proportion of pneumoconiosis patients, deaths in mines, and mortality per million tons. Finally, from the perspective of transport sustainability, the obvious imbalance of China’s coal transportation market and the distribution, production, and consumption characteristics of coal resources determine the national transportation pattern of “transferring coal from the west to the east” and “transporting coal from the north to the south.” As a result, transportation has become another key factor restricting China’s coal supply sustainability. China’s coal is mainly transported by railway. Therefore, coal transportation distance and railway coal transportation volume are typical representative indicators. Based on the analysis mentioned above and to fully consider the availability of data and refer to relevant expert opinions in the field, 19 indicators are constructed. The source of each indicator and operation process of some complex indicators are shown in Table 2.
Among the numerous weighting methods in sustainability assessment, ANP(Analytic Network Process)and the entropy method (EM) are most commonly used weighting methods (Wang et al., 2009; Zhao et al., 2020). ANP is an effective, accurate and practical subjective evaluation method, which can effectively use the hypermatrix to analyze the influencing factors and synthesize the relationship between them. The entropy method (EM) can directly use the data information of the indicators themselves to determine their weight, completely avoided the deviation caused by subjective factors. This method does not have high requirements on the amount of sample data, it has a good calculation effect in the statistical analysis of small sample data, and it is a commonly used objective weighting method in the research of economics, energy, and other fields (Liu and Lin, 2019; Wu et al., 2019; Gong et al., 2021). Both methods have achieved good results in solving the weights of comprehensive evaluation indicators. However, subjective weight is better than objective weight in reflecting the importance of the indicator itself, and the objective weight is better than the subjective weight in reflecting the indicator data information level. If we only use one method for weighting, it is likely to lead to the problem of index weight bias due to the selected weight calculation methods are different. Therefore, after seriously considered the attribute of indicators for CCSSI, we develop a optimal combination weight model of level difference maximization.
This model is a subjective and objective combination weighting model which takes single index as combination unit. The method is to determine the reasonable value range of combination weight according to the subjective and objective weights, taking the interval as the constraint and the maximum discrimination of the evaluated object as the objective function, the optimization model is established, the optimal solution of the optimization model is the combination weight. The advantages of this model are mainly reflected in the following two aspects: the first advantage is this method maximizes the variance of the evaluation results of each evaluation indicator, thereby effectively highlighting the difference between each indicator. The second advantage is it can avoid the inconsistency of evaluation scores and rankings obtained by a single evaluation method. The specific steps are as below:
Step 1: Data normalization treatment.
In this paper, the evaluation indicators of China’s coal supply sustainability can be divided into two categories: The first category is indicators that has a positive impact on the China’s coal supply sustainability, that is, positive indicator; the other category is indicators that has a negative impact on the China’s coal supply sustainability, that is, negative indicator. When the indicator is a positive index, Formula 1 is used for normalization, otherwise, when the indicator is a negative indicator Formula 2 is used for normalization.
(t = 1.2, … ,k; i = 1.2, …
Step 2: Determine the combination weight matrix.
Suppose that in the
Where,
Step 3: Determine the reasonable value range of combination weight.
The reasonable interval range of combination weight can be determined by matrix A. Firstly, the following three definitions are given.
Definition 1:
Definition 2:
Definition 3: hypothesis
Step 4: Build a combinatorial optimization model.
Define the comprehensive evaluation result of coal supply sustainability in China is CCSSI, the standardized matrix of the
Define
Define
Step 4: Solving the combinatorial optimization model.
Take the maximum of
The combination weight
This study analyzes the China’s coal supply sustainability from 2000 to 2019. The dadas for indicators as coal supply per capita, coal price, coal consumption, CO2 emissions, SO2 emissions, clean energy consumption, coal mining mechanization degree, annual fixed assets investment in coal industry, coking efficiency, proportion of pneumoconiosis patients in the total number of occupational diseases, total number of deaths per year due to mine accidents, mortality per million tons of China coal mine, average railway transportation distance of coke and daily average railway loading vehicles were got from China Statistics Yearbook (2000–2020), China Energy Statistical Yearbook (2000–2020), BP World energy statistical database, China coal industry yearbook (2000–2020), the Wind database and Kwah Big Data Center. However, the data for reserve production ratio, coal self sufficiency rate, coal dependence, coal Market concentration and coal strength are not publicly available, so we have to calculate these indicators on our own. The details of how we estimate them are provided in Table 2.
After analyzing the characteristics of each indicator for CCSSI and the applicability of the comprehensive evaluation model, we utilize the data of each indicator for CCSSI from 2000 to 2019 as the sample, normalize them by Formula 1 or Formula 2, and calculate the subjective and objective weights of each indicator based on the ANP method and the entropy method. We then use these weights to determine a reasonable value range for the combination weight of each indicator. Lastly, we determine the optimal combination weight of each indicator by solving the combination weighting optimization model (7). The subjective weight, objective weight, combination weight and rank of each indicator for CCSSI are shown in Table 3. In order to further present the effect of the combined optimization model in this paper, we made a chart of the weights for each indicator of CCSSI, as shown in Figure 3. Figure 3 shows clearly that, compared with the single weighting method of the ANP method and the entropy method, the optimal combination weight have better distinguishing ability, which can better reflect the effect of each indicator on CSSI. This also verifies the rationality of the combinatorial optimization model constructed in this paper.
As shown in Table 3, the weight of each indicator is arranged in order: proportion of alternative energy consumption (C11), coal supply proportion in primary energy (C8), reserve production ratio (C1), coal supply per capita (C3), CO2 emissions (C9), coal strength (C7), investment in fixed assets (C13), proportion of pneumoconiosis patients (C15), SO2 emissions (C10), coal self-sufficiency rate (C2), deaths in mines (C16), coking efficiency (C14), coal transportation volume (C19), coal transportation distance (C18), market concentration (C6), coal mining mechanization degree (C12), coal degree of dependence (C5), coal price (C4), and mortality per million tons of China coal mine (C17). The weights represent the impact of each indicator on the CCSSI. These results are consistent with the policy objectives for the sustainable development of China’s coal industry. It also fully demonstrates the rationality of the research methodology used in this study.
Of the six different dimensions, the top three were environmental sustainability (0.2707), technological sustainability (0.2444), and availability (0.2073); the bottom three were therefore health loss (0.1205), economic sustainability (0.0792), and transport sustainability (0.0779). This result is consistent with the actual coal supply situation in China. Although it is an energy resource with a high degree of pollution, coal has the highest consumption in China. The environmental sustainability of China’s coal supply not only determines China’s coal supply sustainability, but also has great significance for China’s overall sustainable development. Therefore, environmental sustainability ranks first. China’s coal technology not only determines the amount of coal supply, but it also plays a role in the environmental impact and health loss related to coal supply. Therefore, technological sustainability ranks second. In this dimension, the proportion of alternative energy consumption ranks first. This shows that if Chinese government want to fundamentally improve their coal supply sustainability, they still need to rely on renewable energy. But coal is the main energy resource in China. Therefore, the availability of coal is not only a basic requirement to ensure China’s coal supply sustainability, but it is also an important indicator of China’s energy supply sustainability. Therefore, the availability of coal resources ranks the third. China’s coal resources rely mainly on the country’s self-sufficiency. China’s coal prices are becoming more and more concentrated in the market and the degree of dependence on foreign coal is low; therefore, China’s coal has strong economy sustainability. Although China’s coal is unevenly distributed from east to west, the coal supply has never been interrupted due to unsustainable transportation. In recent years, China’s increasing coal imports have further eased the pressure of coal transportation to coastal areas in China and reduced the coal price from inland to coastal cities (Rioux et al., 2016). Therefore, compared with economic sustainability and transportation sustainability, the health loss related to China’s coal supply has a greater impact on its sustainability.
The results here are presented in terms of dimensions to determine the strengths and weaknesses of China’s coal supply sustainability. Figure 4 clearly shows the change characteristics of the three indicators in the coal availability dimension. We find that both reserve production ratio and the coal self-sufficiency rate indicators showed a significant downward trend during the study period. Within this period, the coal supply per capita remained virtually unchanged; except for a slight increase from 2003 to 2007, it remained at approximately 68 kg per capita. The decrease of the availability dimension from 2000 to 2010 is caused by the decreasing of reserve production ratio and coal self-sufficiency, whereas the increase of availability dimension from 2011 to 2016 is caused by the improvement of coal self-sufficiency.
Figure 5 shows the change characteristics of the three indicators in the economic sustainability dimension. During the sample interval, China’s coal price was very volatile. Coal dependence showed an inverted U-shaped trend before 2012 and started increasing continuously after 2012. Market concentration dropped sharply in the sample interval, which reflects the increasing concentration of China’s coal market. The economic sustainability dimension decreased from 2000 to 2019. It decreased rapidly from 2000 to 2004 mainly due to the increase of coal price and coal dependence. And after 2004, the economic sustainability dimension is around 0.03, and it declined mainly due to the sharp change of coal price.
FIGURE 5. Economic sustainability results from 2000 to 2019: (A) indicator value; (B) dimension value.
Figure 6 shows the change characteristics of the four indicators in the environmental sustainability dimension. Except the CO2 emissions indicator, all indicators—coal strength, coal supply, and SO2 emissions—showed a downward trend. Among them, the coal strength indicator declined the fastest, indicating that the unit output of coal consumption per capita in China is decreasing significantly. Environmental sustainability dimension decreased from 2000 to 2005 mainly because the increase of coal strength coal consumption and SO2 emissions. After 2005, it increased rapidly, this fully demonstrates the effectiveness of the environmental policies adopted by the Chinese government.
FIGURE 6. Environmental sustainability results from 2000 to 2019: (A) indicator value; (B) dimension value.
Figure 7 presents the change characteristics of the four indicators in the technological sustainability dimension. China’s coal investment showed a rapid upward trend before 2012, after which it showed a significant V-shaped trend from 2013 to 2019. The indicators for clean energy consumption and coal mining mechanization degree increased slightly; however, the coking efficiency indicator decreased slightly in recent years. Technological sustainability dimension increased sharply from 2000 to 2019 mainly because the increase of coal mining mechanization degree coal investment.
FIGURE 7. Technological sustainability results from 2000 to 2019: (A) indicator value; (B) dimension value.
Figure 8 shows clearly the change characteristics of the three indicators in the health and safety dimension. Within the sample interval, the deaths in mines and mortality rate per million tons dropped significantly. However, the proportion of pneumoconiosis patients increased slightly after 2010. This does not explain why the degree of absenteeism in China’s coal mines is getting worse and worse, despite safety protocols. The reason for the increase in diagnosed miners is that the Chinese government increased its efforts to detect pneumoconiosis as a reason for absenteeism after 2010. Coal miners who had not been tested for pneumoconiosis before 2010 were gradually tested in these years. In addition, this is also because pneumoconiosis itself is a chronic disease with a longer period of onset. Health and security dimension is lower than 0.4 from 2000 to 2003 mainly because the increase of pneumoconiosis patients and sharp increase of deaths in mines, especially the sharp increase of deaths in mines. After 2009, with the sharp decrease of deaths in mines, health and security dimension become increased.
Figure 9 shows clearly the change characteristics of the two indicators in the transport sustainability dimension. China’s coal transportation distance has been increasing from 2000 to 2019; however, the coal transportation volume declined after 2011 and then increased again. It is very interesting that the overall trends of coal transportation volume and the coal investment curve is very consistent. It reflects that China’s coal transportation volume is closely related to coal industry investment. It shows clearly that the trend of transport sustainability is very similar to the trend of coal transportation volume, which fully proved that coal transportation volume is the main determinant of coal transport sustainability.
FIGURE 9. Transport sustainability results from 2000 to 2019: (A) indicator value; (B) dimension value.
The average value of availability, economic sustainability, environmental sustainability, technological sustainability, health and security and transport sustainability dimensions between 2000 and 2019 are 0.0736, 0.0388, 0.1209, 0.1045, 0.0580 and 0.0445, respectively. The highest average dimension value is environmental sustainability, while the lowest is economic sustainability. The dimensions that increases more quickly from 2000 to 2019 are environmental sustainability and technological sustainability, whereas availability and economic sustainability dimensions are tend to decrease. Thus, the environmental sustainability and technological sustainability should be improved immediately to create a more sustainable coal supply (see Figure 10).
The optimal combination weight of each CCSSI indicator in Figure 11 is substituted into Formula 11 to obtain the CCSSI from 2000 to 2019. The evolution trend and change rate of CCSSI are shown in Figure 12. Figure 12 shows that within the sample interval, China’s energy supply sustainability improved significantly, from 0.3102 in 2000 to 0.7004 in 2019. However, it is obvious that the overall level of China’s energy supply sustainability is not high.
From 2000 to 2005, China’s coal supply sustainability level fluctuated greatly. Compared with the year 2000, China’s coal supply sustainability began to decline from the year 2001, the decline rate reached as high as 8.59% in the year 2002. In the year 2003, China’s coal supply sustainability started increasing rapidly. In the year 2003 and the year 2004, the growth rate reached 17.26 and 12.32% respectively. However, in the year 2005, there was a decline of 7.58%. Because of the rapid development of China’s economy from 2000 to 2005, the proportion of coal in primary energy consumption increased from 71.5% in 2000 to 75.4% in 2005. However, for this period, China’s.
Coal technology was relatively unsophisticated and coal mine safety accidents were also very frequent. According to statistics, there were 18,514 accidents in China’s coal mines from 2001 to 2005, which caused 31,064 deaths. The average annual number of coal mine safety accidents of all kinds was 3,702, and the death toll of mine accidents reached 6,213 in this period.
Since 2006, China’s coal supply sustainability index has been increasing, especially during the period from 2009 to 2012 and during 2018. This is because after 2007, the Chinese government increased investment in coal mine safety, continuously improved equipment and the level of safety technology, and paid attention to safety technology training and safety education among coal mine workers. Since then, China’s coal production has increased continuously, the mortality rate per million tons has gradually decreased, and the coal mine supply safety situation has obviously improved. From 2008, the Chinese government started paying attention to the importance to renewable energy. More than 20 related supporting policies—including supporting electricity price and investment subsidies—have been successively issued. This has effectively promoted the industrial progress of renewable energy. Considering the emission standard of air pollutants for thermal power plants, in 2011, a new version of “the emission standard of air pollutants for thermal power” was issued by the Chinese government. This regulation greatly increased the emission concentration requirements of sulphur dioxide, nitrogen oxides, smoke, and dust, some of these regulations are even stricter than the European Union standards, which greatly urging thermal power plants to carry out energy-saving and emission-reducing transformation to reduce emissions and to add waste gas treatment and disposal facilities to improve the environmental impact of coal supply. With the support of the series of policies mentioned above, the supply sustainability level of coal in China improved significantly, from 0.3849 in 2009 to 0.5293 in 2012.
After 2013, China’s coal supply sustainability index increased steadily at a speed of about 0.2% per year; however, in 2018, the growth rate suddenly increased to 12.45%. This is because in this period, China’s carbon emissions increased rapidly (Wang et al., 2020b). Coal, as China’s main energy source, still produces a lot of environmental pollution and health losses. China’s coal technology is still less developed than many developed countries, and in recent years, the import of coal has also increased significantly. In 2018, China’s coal consumption fell below 60% for the first time. The significant increase of CCSSI in 2018 than 2017 shows clearly that the promotion speed for CCSSI brought by clean energy substitution is much higher than that of other coal sustainable development policies issued by China. Interestingly, the evolution trend of China’s coal supply sustainability is almost consistent with the functional curve of China’s energy security. This result is also consistent with China’s actual coal supply situation. As the main energy source in China, the sustainable supply of coal determines China’s energy security; this also reflects the important role of coal for China’s energy security.
As referring to the Li et at (Li and Zhang, 2019) about China’s energy supply sustainability (ESSI), here we get the comparison results of China’s energy supply sustainability (ESSI) and China’s coal supply sustainability (CSSI) as seen in Figure 12. From the change trend of CSSI and ESSI, ESSI is a little bit more volatile, because ESSI is influenced not only by the sustainability of China’s coal supply, but also by oil, gas, renewable energy, and complex factors both at home and abroad. In Figure 11, it is very clear that the economic crisis of 2008, the economic crisis has reduced energy consumption in most countries around the world while maintaining large supplies, and thus CSSI and ESSI all increased (Erahman et al., 2016). This also explains the linear relationship between economic sustainability and energy supply sustainability. As refer to (Gong et al., 2021) measured the energy security levels of 30 provinces in China from 2004 to 2017 the entropy weight method, they found that the energy security level of Inner Mongolia, Shanxi, and Shaanxi ranked in the top three in China. These three provinces are very rich in coal resources in China. Once again verified the important role of coal for China’s energy security. It shows that the entropy method is suitable for the study of small sample and multi-index attribute problems such as energy security and coal supply sustainability.
Based on the United Nations’ sustainable development agenda, focuses on the Chinese carbon neutrality goals, and according to the characteristics of coal supply in China, this paper designs the research framework of ‘policy objective analysis—index system construction—model construction—empirical research’ taking China’s theme energy coal as the research object. On the basis of previous studies, aiming at the practical problems and difficulties in China’s current coal supply process, and in particular, the health and security, and transport sustainability indicators are taken into consideration, we proposed a China’s coal supply sustainability index based on six aspects: availability, economic sustainability, environmental sustainability, technological sustainability, health and security, and transport sustainability. Then fully considered advantages of ANP and EM models, we constructed a novel optimized comprehensive evaluation model with level difference maximization, and assessed the sustainability of China’s coal supply over the past 20 years from 2000 to 2019.
This study found that the sustainability of China’s coal supply has been greatly improved from 2000 to 2019, indicating that China’s coal supply is becoming more and more sustainable. Mainly credit to the greatly improved environmental sustainability and technological sustainability. Among them, the substitution of renewable energy, the improvement of coal mechanization and the implementation of related environmental policies are the fundamental reasons. Economic efficiency has a negative effect on the sustainability of coal supply, this result is consistent with the World Energy Trilemma Index and also in line with the reality of the Chinese coal market.
Although China will continue to develop renewable energy and take more measures to reduce coal consumption in the process of achieving the carbon neutral goal, China’s coal consumption may increase in the short term, especially in the next 5 years. In the long run, even if renewable energy develops vigorously, coal will play an important role in ensuring the flexibility of the power system in the future. Improving the sustainability of coal supply is of great significance to ensuring the security of China’s energy supply.
According to the research results of this paper, the optimal coal supply system in China should be an advanced and intelligent supply system with zero emissions of CO2, SO2 and other pollutants, zero death, stable market price and sufficient supply. In the future, coal power plants have strong flexibility, which can flexibly adjust the peak, make up for the instability of renewable power such as solar power and wind power, improve the current situation of insufficient power supply in some areas in some years, and ultimately promote the adequate supply and clean supply of the power system. Therefore, in addition to continuing to promote technological innovation in the coal field, we must also vigorously promote the application of blockchain technology, big data and other technologies in the energy system to improve the efficiency of coal mining and use, and ensure that the supply of coal is available. Persistent (Weng and Huang, 2021). Moreover, there is an urgent need to increase policy flexibility, focus on building a new development pattern of domestic and international double cycles and mutual promotion, organically combine domestic development with strengthening international cooperation, and make better use of both domestic and foreign markets and resources. To promote a win-win relationship between carbon peaking and coal sustainability in the process of opening up to the outside world, so as to achieve high-quality development of the coal industry and sustainable economic and social development.
The raw data supporting the conclusion of this article will be made available by the authors, without undue reservation.
All authors contributed equally to this work. JZ proposed the original idea; JX modified and refined the manuscript; PL designed the research and wrote the manuscript; HY optimized the methodology and embellished the picture in this paper. MD provided most of the important data and edited the language. All authors read and approved the final manuscript.
This research was supported by the National Natural Science Foundation of China under grant nos. 71273206 and 72004181, by the National Social Science Foundation of China under grant nos. 18AZD005 and 20BJY076, and by the China Postdoctoral Science Foundation, grant no. 2019M663781.
The authors declare that the research was conducted in the absence of any commercial or financial relationships that could be construed as a potential conflict of interest.
All claims expressed in this article are solely those of the authors and do not necessarily represent those of their affiliated organizations, or those of the publisher, the editors and the reviewers. Any product that may be evaluated in this article, or claim that may be made by its manufacturer, is not guaranteed or endorsed by the publisher.
The authors would like to express their sincere thanks to the anonymous referees as well as the editors.
Ai De, C. (2008). Forecast and Early-Warning on Coal Supply and Demand Balance in China[D]. China: China University of Mining and Technology.
Ali, S. H., Giurco, D., Arndt, N., Nickless, E., Brown, G., Demetriades, A., et al. (2017). Mineral Supply for Sustainable Development Requires Resource Governance. Nature 543 (7645), 367–372. doi:10.1038/nature21359
Ang, B. W., Choong, W. L., and Ng, T. S. (2015). A Framework for Evaluating Singapore's Energy Security. Appl. Energ. 148, 314–325. doi:10.1016/j.apenergy.2015.03.088
Botin, J. A. M. (2009). Managing Project Feasibility and Construction: Sustainable Management of Mining Operations. Littleton, USA: Society for Mining, Metallurgy, and Exploration, 207–260.
Breaking, I. (2002). New Ground: Mining, Minerals and Sustainable Development (Report of the MMSD Project)[M]. Earthscan Publications Limited.
Brown, M. A., and Sovacool, B. K. (2007). Developing an 'energy Sustainability index' to Evaluate Energy Policy. Interdiscip. Sci. Rev. 32 (4), 335–349. doi:10.1179/030801807x211793
Chen, F., and Wu, C. (2020). A Novel Methodology for Forecasting Gas Supply Reliability of Natural Gas Pipeline Systems. Front. Energ. 14 (2), 213–223. doi:10.1007/s11708-020-0672-5
Chen, S.-S., Xu, J.-H., and Fan, Y. (2015). Evaluating the Effect of Coal Mine Safety Supervision System Policy in China's Coal Mining Industry: A Two-phase Analysis. Resour. Pol. 46, 12–21. doi:10.1016/j.resourpol.2015.07.004
Chen, X., and Zhou, D. (2010). An Empirical Study of Market Concentration of China’s Coal Industry[J]. Contemp. Finance Econ. (02), 80–89.
Corder, G. D., Mclellan, B. C., and Green, S. (2010). Incorporating Sustainable Development Principles into Minerals Processing Design and Operation: SUSOP. Minerals Eng. 23 (3), 175–181. doi:10.1016/j.mineng.2009.12.003
Cui, R. Y., Hultman, N., Cui, D., McJeon, H., Yu, S., Edwards, M. R., et al. (2021). A Plant-By-Plant Strategy for High-Ambition Coal Power Phaseout in China. Nat. Commun. 12 (1), 1468. doi:10.1038/s41467-021-21786-0
Duan, H., Zhou, S., Jiang, K., Bertram, C., Harmsen, M., Kriegler, E., et al. (2021). Assessing China's Efforts to Pursue the 1.5°C Warming Limit. Science 372, 378–385. doi:10.1126/science.aba8767
Erahman, Q. F., Purwanto, W. W., Sudibandriyo, M., and Hidayatno, A. (2016). An Assessment of Indonesia's Energy Security index and Comparison with Seventy Countries. Energy 111, 364–376. doi:10.1016/j.energy.2016.05.100
Fang, X., and Zhang, W. (2013). Sustainability of China's Coal Import and Adjustment of Import Country Structure -- Strategic Thinking on China Russia Coal Cooperation and Development and Utilization of Russian Far East Coal Resources [J]. Contemp. Econ. Res. 000 (011), 22–29.
Feng, Y., Wang, S., Sha, Y., Ding, Q., Yuan, J., and Guo, X. (2018). Coal Power Overcapacity in China: Province-Level Estimates and Policy Implications. Resour. Conserv. Recycl. 137, 89–100. doi:10.1016/j.resconrec.2018.05.019
Gong, X., Wang, Y., and Lin, B. (2021). Assessing Dynamic China's Energy Security: Based on Functional Data Analysis. Energy 217, 119324. doi:10.1016/j.energy.2020.119324
Green, E. C. (2001). Paper–Towards a European Strategy for the Security of Energy supply[J]. Luxembourg: Office for Official Publications of the European Communities.
Guo, J., and Wang, E. (2010). Measurement Indicator System and Comprehensive Appraisal for Coal Energy Security in China. [J]. China Saf. Sci. J. 11, 112–118.
Hughes, L. (2009). The Four 'R's of Energy Security. Energy Policy 37 (6), 2459–2461. doi:10.1016/j.enpol.2009.02.038
Iddrisu, I., and Bhattacharyya, S. C. (2015). Sustainable Energy Development Index: A Multi-Dimensional Indicator for Measuring Sustainable Energy Development. Renew. Sust. Energ. Rev. 50, 513–530. doi:10.1016/j.rser.2015.05.032
Jie, D., Xu, X., and Guo, F. (2021). The Future of Coal Supply in China Based on Non-fossil Energy Development and Carbon price Strategies. Energy 220, 119644. doi:10.1016/j.energy.2020.119644
Jing, Q., and Jiang, X. (2006). Study on Index System of Capability of Production Safety in Coal Mine Based on AHP[J]. China Saf. Sci. J. (09), 74–79.
Kemmler, A., and Spreng, D. (2007). Energy Indicators for Tracking Sustainability in Developing Countries. Energy Policy 35 (4), 2466–2480. doi:10.1016/j.enpol.2006.09.006
Kemp, D., Worden, S., and Owen, J. R. (2016). Differentiated Social Risk: Rebound Dynamics and Sustainability Performance in Mining. Resour. Pol. 50, 19–26. doi:10.1016/j.resourpol.2016.08.004
Kowalska, I. J. (2014). Risk Management in the Hard Coal Mining Industry: Social and Environmental Aspects of Collieries' Liquidation. Resour. Pol. 41, 124–134. doi:10.1016/j.resourpol.2014.05.002
Kumar, D., and Katoch, S. S. (2014). Sustainability Indicators for Run of the River (RoR) Hydropower Projects in Hydro Rich Regions of India. Renew. Sust. Energ. Rev. 35, 101–108. doi:10.1016/j.rser.2014.03.048
Laponche, B., and Tillerson, K. (2001). Green Paper: Towards a European Strategy for the Security of Energy Supply[J]. Vgb Powertech 1 (82), 27–29.
Li, N., and Chen, W. (2018). Modeling China's Interprovincial Coal Transportation under Low Carbon Transition. Appl. Energ. 222, 267–279. doi:10.1016/j.apenergy.2018.03.103
Li, P., and Zhang, J. (2019). Is China's Energy Supply Sustainable? New Research Model Based on the Exponential Smoothing and GM(1,1) Methods. Energies 12 (2), 236. doi:10.3390/en12020236
Liu, F., Lv, T., Sajid, M., and Li, X. (2018). Optimization for China's Coal Flow Based on Matching Supply and Demand Sides. Resour. Conserv. Recycl. 129, 345–354. doi:10.1016/j.resconrec.2016.08.013
Liu, K., and Lin, B. (2019). Research on Influencing Factors of Environmental Pollution in China: A Spatial Econometric Analysis. J. Clean. Prod. 206, 356–364. doi:10.1016/j.jclepro.2018.09.194
Liu, X., Guo, P., and Guo, S. (2019). Assessing the Eco-Efficiency of a Circular Economy System in China's Coal Mining Areas: Emergy and Data Envelopment Analysis. J. Clean. Prod. 206, 1101–1109. doi:10.1016/j.jclepro.2018.09.218
Liu, X., Liu, Z., Jiao, W., Li, X., Lin, J., and Ku, A. (2021). Impact of "ultra Low Emission" Technology of Coal-Fired Power on PM2.5 Pollution in the Jing-Jin-Ji Region. Front. Energ. 15 (1), 235–239. doi:10.1007/s11708-017-0518-y
Mainali, B., and Silveira, S. (2015). Using a Sustainability index to Assess Energy Technologies for Rural Electrification. Renew. Sust. Energ. Rev. 41, 1351–1365. doi:10.1016/j.rser.2014.09.018
Mallapaty, S. (2020). How China Could Be Carbon Neutral by Mid-century. Nature 586 (586), 482–483. doi:10.1038/d41586-020-02927-9
Marquez-Ballesteros, M.-J., Mora-López, L., Lloret-Gallego, P., Sumper, A., and Sidrach-de-Cardona, M. (2019). Measuring Urban Energy Sustainability and its Application to Two Spanish Cities: Malaga and Barcelona. Sust. Cities Soc. 45, 335–347. doi:10.1016/j.scs.2018.10.044
Martín-Gamboa, M., Iribarren, D., García-Gusano, D., and Dufour, J. (2017). A Review of Life-Cycle Approaches Coupled with Data Envelopment Analysis within Multi-Criteria Decision Analysis for Sustainability Assessment of Energy Systems. J. Clean. Prod. 150, 164–174. doi:10.1016/j.jclepro.2017.03.017
Meghan, L. O. S. (2013). The Entanglement of Energy, Grand Strategy, and International security[Z]. England: John Wiley & Sons, 1–566.
Merino-Saum, A., Baldi, M. G., Gunderson, I., and Oberle, B. (2018). Articulating Natural Resources and Sustainable Development Goals through green Economy Indicators: A Systematic Analysis. Resour. Conserv. Recycl. 139, 90–103. doi:10.1016/j.resconrec.2018.07.007
Mondal, M. A. H., and Denich, M. (2010). Assessment of Renewable Energy Resources Potential for Electricity Generation in Bangladesh. Renew. Sust. Energ. Rev. 14 (8), 2401–2413. doi:10.1016/j.rser.2010.05.006
Munasinghe, M. (1994). Protected Area Economics and Policy: Linking Conservation and Sustainable Development. Economic and Policy Issues in Natural Habitats and Protected Areas, 15–49.
Narula, K., and Reddy, B. S. (2016). A SES (Sustainable Energy Security) index for Developing Countries. Energy 94, 326–343. doi:10.1016/j.energy.2015.10.106
Oberschelp, C., Pfister, S., Raptis, C. E., and Hellweg, S. (2019). Global Emission Hotspots of Coal Power Generation. Nat. Sustain. 2 (2), 113–121. doi:10.1038/s41893-019-0221-6
Pavlović, D., Banovac, E., and Vištica, N. (2018). Defining a Composite index for Measuring Natural Gas Supply Security - the Croatian Gas Market Case[J]. Energy Policy 114, 30–38.
Radovanović, M., Filipović, S., and Pavlović, D. (2017). Energy Security Measurement – A Sustainable Approach[J]. Renew. Sust. Energ. Rev. 68, 1020–1032.
Rauner, S., Bauer, N., Dirnaichner, A., Dingenen, R. V., Mutel, C., and Luderer, G. (2020). Coal-exit Health and Environmental Damage Reductions Outweigh Economic Impacts. Nat. Clim. Chang. 10 (4), 308–312. doi:10.1038/s41558-020-0728-x
Raza, S. S., Janajreh, I., and Ghenai, C. (2014). Sustainability index Approach as a Selection Criteria for Energy Storage System of an Intermittent Renewable Energy Source. Appl. Energ. 136, 909–920. doi:10.1016/j.apenergy.2014.04.080
Rioux, B., Galkin, P., Murphy, F., and Pierru, A. (2016). Economic Impacts of Debottlenecking Congestion in the Chinese Coal Supply Chain. Energ. Econ. 60, 387–399. doi:10.1016/j.eneco.2016.10.013
Sovacool, B. K., Mukherjee, I., Drupady, I. M., and D’Agostino, A. L. (2011). Evaluating Energy Security Performance from 1990 to 2010 for Eighteen Countries. Energy 36 (10), 5846–5853. doi:10.1016/j.energy.2011.08.040
Sovacool, B. K., and Walter, G. (2018). Major Hydropower States, Sustainable Development, and Energy Security: Insights from a Preliminary Cross-Comparative Assessment. Energy 142, 1074–1082. doi:10.1016/j.energy.2017.09.085
Stefanova, B. (2012). European Strategies for Energy Security in the Natural Gas Market. [J] 3 (5). doi:10.5038/1944-0472.5.3.4
Tian, S., and Zhao, P. (2015). Study on the Early-Warning Management of Daqing’s Overseas Oil Drilling Project Risks—Based on Fuzzy Comprehensive Evaluation Model. Nat. Resour. Econ. China (1), 29–33.
Tsai, W.-T. (2010). Energy Sustainability from Analysis of Sustainable Development Indicators: A Case Study in Taiwan. Renew. Sust. Energ. Rev. 14 (7), 2131–2138. doi:10.1016/j.rser.2010.03.027
von der Goltz, J., and Barnwal, P. (2019). Mines: The Local Wealth and Health Effects of mineral Mining in Developing Countries. J. Dev. Econ. 139, 1–16. doi:10.1016/j.jdeveco.2018.05.005
Wang, C., and Zhang, Y. (2020). Implementation Pathway and Policy System of Carbon Neutrality Vision[J]. Chin. J. Environ. Manag. 72 (06), 60–66.
Wang, D., Nie, R., Long, R., Shi, R., and Zhao, Y. (2018). Scenario Prediction of China's Coal Production Capacity Based on System Dynamics Model. Resour. Conserv. Recycl. 129, 432–442. doi:10.1016/j.resconrec.2016.07.013
Wang, J.-J., Jing, Y.-Y., Zhang, C.-F., and Zhao, J.-H. (2009). Review on Multi-Criteria Decision Analysis Aid in Sustainable Energy Decision-Making. Renew. Sust. Energ. Rev. 13 (9), 2263–2278. doi:10.1016/j.rser.2009.06.021
Wang, J., Feng, L., Davidsson, S., and Höök, M. (2013). Chinese Coal Supply and Future Production Outlooks. Energy 60, 204–214. doi:10.1016/j.energy.2013.07.031
Wang, L., Cao, Q., and Zhou, L. (2018). Research on the Influencing Factors in Coal Mine Production Safety Based on the Combination of DEMATEL and ISM. Saf. Sci. 103, 51–61. doi:10.1016/j.ssci.2017.11.007
Wang, Q., Li, S., and Pisarenko, Z. (2020). Modeling Carbon Emission Trajectory of China, US and India. J. Clean. Prod. 258, 120723. doi:10.1016/j.jclepro.2020.120723
Wang, W., Jia, H., and Lin, Y. (2013). Study on Research Framework of Effective Supply Capability of Regional Coal[J]. China Coal (12), 12–15.
Wang, Y., Chen, H., Long, R., and Yang, M. (2020). Health Economic Loss Measurement and Risk Assessment of New Cases of Coal Worker's Pneumoconiosis in China. Saf. Sci. 122, 104529. doi:10.1016/j.ssci.2019.104529
Wang, Z. H., and li, X. (2013). Study on the Efficiency Evaluation of green Investment of Coal enterprise Based on Entropy Method. [J] (05), 28–30.
Wei, Y.-M., Chen, H., Chyong, C. K., Kang, J.-N., Liao, H., and Tang, B.-J. (2018). Economic Dispatch Savings in the Coal-Fired Power Sector: An Empirical Study of China. Energ. Econ. 74, 330–342. doi:10.1016/j.eneco.2018.06.017
Wei, Y. M., Han, R., Wang, C., Yu, B., Liang, Q. M., Yuan, X. C., et al. (2020). Self-preservation Strategy for Approaching Global Warming Targets in the post-Paris Agreement Era. Nat. Commun. 11 (1), 1624. doi:10.1038/s41467-020-15453-z
Weng, S., and Huang, Z. (2021). Building a Modern Energy System in the Yangtze River Delta. Strateg. Study CAE 23 (01), 42–51.
Wirl, F. (1995). The Exploitation of Fossil Fuels under the Threat of Global Warming and Carbon Taxes: A Dynamic Game Approach. Environ. Resource Econ. 5 (4), 333–352. doi:10.1007/bf00691573
Wu, H., Hao, Y., and Weng, J.-H. (2019). How Does Energy Consumption Affect China's Urbanization? New Evidence from Dynamic Threshold Panel Models. Energy Policy 127, 24–38. doi:10.1016/j.enpol.2018.11.057
Wyman, O. (2013). World Energy Trilemma 2013 Energy Sustainability index. London: UK: World Energy Council.
Xu, X., and Wang, W. (2021). Vulnerability Assessment and Management Strategies of the Regional Coal Market Supply in China. [J].China Coal. 44 (09), 5–10.
Xu, Z., Chau, S. N., Chen, X., Zhang, J., Li, Y., Dietz, T., et al. (2020). Assessing Progress towards Sustainable Development over Space and Time. Nature 577 (7788), 74–78. doi:10.1038/s41586-019-1846-3
Yan, Y., Sun, L., Peng, Z., Qi, H., Liu, L., and Sun, R. (2021). Effects of Pyrolyzed Semi-char Blend Ratio on Coal Combustion and Pollution Emission in a 0.35 MW Pulverized Coal-Fired Furnace. Front. Energ. 15 (1), 78–90. doi:10.1007/s11708-020-0678-z
Yang, R. G., and Fan, Y. (2005). System Dynamics Analytic Model for Coal Supply and Investment[J]. J. Appl. Stat. Manage. (05), 6–12.
Yang, Y., Zeng, M., Xue, S., Wang, J., and Li, Y. (2018). Unifying the "Dual-Track" Pricing Mechanism for Coal in China: Policy Description, Influences, and Suggestions for Government and Generation Enterprises. Resour. Conserv. Recycl. 129, 402–415. doi:10.1016/j.resconrec.2016.04.004
Yuan, J., Li, P., Wang, Y., Liu, Q., Shen, X., Zhang, K., et al. (2016). Coal Power Overcapacity and Investment Bubble in China during 2015-2020. Energy Policy 97 (10), 136–144. doi:10.1016/j.enpol.2016.07.009
Zhang, C., Su, B., Zhou, K., and Sun, Y. (2020). A Multi-Dimensional Analysis on Microeconomic Factors of China's Industrial Energy Intensity (2000-2017). Energy Policy 147, 111836. doi:10.1016/j.enpol.2020.111836
Zhang, Y., Nie, R., Shi, R., and Zhang, M. (2018). Measuring the Capacity Utilization of the Coal Sector and its Decoupling with Economic Growth in China's Supply-Side Reform. Resour. Conserv. Recycl. 129, 314–325. doi:10.1016/j.resconrec.2016.09.022
Zhao, D., Li, C., Wang, Q., and Yuan, J. (2020). Comprehensive Evaluation of National Electric Power Development Based on Cloud Model and Entropy Method and TOPSIS: A Case Study in 11 Countries. J. Clean. Prod. 277, 123190. doi:10.1016/j.jclepro.2020.123190
Keywords: coal supply, sustainability, optimization, comprehensive evaluation, carbon neutral
Citation: Li P, Yu H, Zhang J, Du M and Xiong J (2021) Coal Supply Sustainability in China: A New Comprehensive Evaluation Methodology. Front. Energy Res. 9:701719. doi: 10.3389/fenrg.2021.701719
Received: 28 April 2021; Accepted: 29 October 2021;
Published: 17 December 2021.
Edited by:
You-hua Chen, South China Agricultural University, ChinaReviewed by:
Dongxiao Yang, Hunan University of Technology and Business, ChinaCopyright © 2021 Li, Yu, Zhang, Du and Xiong. This is an open-access article distributed under the terms of the Creative Commons Attribution License (CC BY). The use, distribution or reproduction in other forums is permitted, provided the original author(s) and the copyright owner(s) are credited and that the original publication in this journal is cited, in accordance with accepted academic practice. No use, distribution or reproduction is permitted which does not comply with these terms.
*Correspondence: Jinsuo Zhang, bWFyazU2emhhbmdAMTYzLmNvbQ==; Meiyang Du, bWVpeWFuZy5kdUBzanR1LmVkdS5jbg==; Jing Xiong, YmVhcm5lYXJAMTYzLmNvbQ==
Disclaimer: All claims expressed in this article are solely those of the authors and do not necessarily represent those of their affiliated organizations, or those of the publisher, the editors and the reviewers. Any product that may be evaluated in this article or claim that may be made by its manufacturer is not guaranteed or endorsed by the publisher.
Research integrity at Frontiers
Learn more about the work of our research integrity team to safeguard the quality of each article we publish.