- 1Energy Technology Program, Faculty of Engineering, Prince of Songkla University, Hat Yai, Thailand
- 2Department of Mechanical Engineering, Institute of Aerospace and Avionics, Air University, Islamabad, Pakistan
- 3Department of Mechanical and Manufacturing Engineering, Universiti Tun Hussein Onn Malaysia, Parit Raja, Malaysia
- 4Department of Mechanical and Mechatronics Engineering, Faculty of Engineering, Prince of Songkla University, Hat Yai, Thailand
- 5Department of Mechanical Engineering, King Fahd University of Petroleum and Minerals, Dhahran, Saudi Arabia
The current study is an economic evaluation and feasibility study of hybrid microgrid Net Zero Energy Buildings in Pakistan. A Net Zero Energy approach is proposed for a two-story hospital building situated in Taxila, Pakistan. Consequently, to design a hybrid Net Zero Energy Building, the solar radiation potential of the subject location and electricity usage of the hospital are estimated. The proposed hybrid microgrid Net Zero Energy Building comprises photovoltaic modules and converters. However, the thermal load is computed as a grid-connected hybrid system. Economic evaluation is performed by using economic indicators of the net present cost and the payback period. Also, initial and operational costs are determined, to determine the profitability of the project. Results show that the analysis is a cost-effective approach and has a payback period of 2.53y. Additionally, the per-unit cost of electricity is reduced to 0.12 USD/kWh. Moreover, the energy produced by a hybrid system is 10.24% more economical than that of the pre-working grid system. Results explain the reduction in the cost of energy and profit margin in electricity generation and the increase in electricity production and feasibility of hybrid Net Zero Energy Buildings in Pakistan. Research will help to develop an approach toward an IEA task 47 in Pakistan with the possible development of simulation-based installations of Net Zero Energy Buildings in the health sector in Pakistan.
Introduction
In the past few decades, energy consumption in buildings has been increased to 30% worldwide (Ji et al., 2017; Si et al., 2018). Renewable energy is not a new concept to work with. Many researchers are already working on various renewable energy systems to reduce capital costs and increase energy production. In a study, the water management of PEM fuel is inspected, and, according to the results, the effectiveness of PEM fuel cells is at maximum at 80°C with the removal of wastewater periodically (Taner, 2015a). In another research, the importance of simulation and optimization techniques is highlighted by working on PEM fuel cells in the energy sector. Results conclude that by the simulation of operating pressures and voltage parameters as working parameters, the energy and exergy efficiencies are 47.6 and 50.4%, respectively (Taneer, 2018). In a study of the PEM electrolyzer, the payback period for the hydrogen generation systems is observed. Operating parameters were the current and voltage. According to the results, the simple payback period was thought to be 2.32y (Taneer et al., 2018). In another research, a techno economic analysis was performed for sugar production processes. The main aim of the research was to reduce capital cost with an increase in the production of turbine power. So, by way of a well-structured analysis, the unit cost ought to be 3.142 [$/kW] with a payback period of 4.32 years (Taneer and Sivrioglu, 2017).
In recent times, China has emerged as a major global energy consumer in terms of the building sector (Wang et al., 2014). However, the energy consumption of hospitals is more than double that of other ordinary buildings in society. Energy use in hospitals is important due to the high heating, cooling, and ventilation thermal loads, critical medical equipment, and operational requirements (Balaras et al., 2007). Hence, energy-efficient designs for hospitals can play a vital role in sustainable development in society (Meegoda et al., 2012). In the past years, many studies have been conducted in different countries especially for hospitals. For example, Ahmed H·S (Sherif, 1999) proposed in 1999 that cost-effective and technical aspects should be considered for the designing of hospitals in developing nations, particularly for low-income groups. In a study in the United Kingdom in 2007 (Shu et al., 2007), primary energy consumption was reduced by 15% by simply implementing sustainable healthcare goals. Also, a sensitivity analysis for the building energy consumption and energy-saving potential optimization of the hospital energy supply system was conducted by Japanese researchers in Asia (Schweber, 2013). According to the research, there is little available data for energy consumption related to hospitals, especially energy data for climate diversity in different regions of Asia. Moreover, for the designing of NZEBs (Alfano et al., 2014), two characteristics have to be measured, namely, indoor environmental quality (IEQ) and energy reduction per capita. The new regulation demands that the NZEB goal must be achieved with an acceptable IEQ level for residents, especially for medical institutions, i.e., hospital buildings. Moreover, with the help of two different groups of people, i.e., medical staff and patients, the IEQ demand can be standardized. Therefore, a lot of literature is available on IEQ in hospital buildings (Skoog et al., 2005; Han et al., 2007; Yau and Chew, 2009; Pourshaghaghy and Omidvari, 2012; Del Ferraro et al., 2015; Golbazi and Aktas, 2016; Alfano et al., 2017; Khalid et al., 2017; Sattayakorn et al., 2017). One of the best researches in the area of indoor environmental quality is Fanger's research, which describes various factors regarding human thermal comfort and indoor air quality in the hospitals (Skoog et al., 2005).
Presently, non-residential buildings are major power consumers in developed countries, consuming 20–40% of the total primary energy (PE). For example, in 2010, the Dutch environment consumed 41% of the total energy consumption in the Netherlands (Vanhoutteghem et al., 2015). For these reasons, the European Union issued the “Energy Performance Directive for Buildings” (EPBD) in May 2010 (EIA, 2011). The directive sets forth requirements to accomplish the goals of the NZEBs regulations and EU member states (MS) legislation by 2020. Generally, from January 1, 2019, new buildings under the government must be NZEBs, and the rest of the new buildings be they either residential or public utilities must meet the NZEB requirements from January 1, 2020. As there is in the Netherlands no clear definition of NZEBs for 2020, the Dutch administration has issued a public plan to encourage NZEBs (Dutch governments, 2012), which illustrates different schemes to decrease overall energy consumption. In another research (REHVA, 2010), the total energy consumption by the Dutch healthcare sector accounts for 1.64%. There are 120 hospitals in the Netherlands, 8 of which are academic hospitals (Ministerie van Volkshuisvesting, 2010; Zurghulpatias, 2015a,b).
Similarly, in the past decade, NZEBs in the United States have received increasing attention from different organizations, i.e., ASHRAE and the American Architectural Institute (AIA). ASHRAE has set a target to develop a tool by 2020 that will facilitate the building community to develop a viable NZEB approach in the market by 2030 (AIA, 2011). The AIA 2030 has the goal of attaining new carbon-neutral buildings all over the country (Entrop and Brouwers, 2019). In previous literature, only a few studies were based on the actual measured performance and efficiency of NZEBs. For example (AlAjmi et al., 2016; Zhou et al., 2016; Kneifel and Webb, 2018; Wu and Skye, 2018), worked on NZEBs. AlAjmi et al. (2016) worked on simulation-based studies to examine the possibility of converting existing institutional buildings to NZEB in the hot and humid climate of Kuwait. In the study, solar PV and thermal cooling systems were installed in Kuwait, and feasibility analysis was performed for NZEBs.
Also, there are many established healthcare regulations available in Europe, the USA, and the UK. Building Research Establishment Environmental Assessment Method (BREEAM) is the earliest green building rating system, established in the UK in 1990 (Schweber and Haroglu, 2014; Alhorr et al., 2015). Among BREEAM products, the BREEAM healthcare version was developed for green hospital buildings in 2008 (Ferreira et al., 2014). Leadership in Energy and Environmental Design (LEED) is another green building rating system, and it was launched by the US Green Building Council in 1998. In the LEED product family, the U.S. Green Building Council (USGBC) released LEED for healthcare in 2011. In addition, the US healthcare industry steering committee has also formulated the Green Healthcare Guidelines (GGHC) (Azhar et al., 2011; Wu et al., 2017). The rating systems of these developed countries set an example for other countries. However, different regions have different energy consumption attributes and impact methods, and the evaluation method must thus be suitable for the real situation of the country. Therefore, in 2006, China formulated the “Evaluation Standard for Green Buildings” (ESGB), which was the first assessment standard for the “environmental performance rating” of green buildings in China (Younger et al., 2008; Pradinuk, 2009).
In developed nations, there are many international communities that are already working on NZEBs. However, in underdeveloped nations like Pakistan, the energy consumption of buildings was 44% from 2016 to 2017. The degree of energy consumed in the building sector will be doubled in the year 2030 (Liu et al., 2014). Similarly, buildings also account for 43% of greenhouse gas emissions and 75% of the electricity usage in Pakistan (Jian et al., 2017; Zhang et al., 2017). More than 38% of the total building energy is consumed in heating, ventilation, and air conditioning. The energy needs of commercial buildings account for a considerable part of the total energy consumption (Chen et al., 2008). In Pakistan, hospitals account for 18% of the energy use and they occupy 17% of the total non-residential area [Pakistan Integrated Energy Model (Pak-IEM), Ministry of Planning Development Government of Pakistan, 2011].
In order to solve this problem, one of the two solutions can be used: minimizing the building energy usage or adapting the renewable energy resources. Analysts believe that it is possible to achieve 70% financial savings by applying better design strategies and efficient tools during the building design phase (Rafique and Rehmanb, 2017). Energy shortage in Pakistan is attributed to an ever-increasing population size, followed by consumers being subjected to frequent load shedding. Pakistan, being the sixth most populous country in the world, makes up about 2.56% of the total worldwide population (Khan and Pervaiz, 2013; Raheem et al., 2016). Pakistan relies on fossil fuels to fulfill its energy demands and has to spend 20% on crude oil imports (Haves, 2019). Additionally, energy demand is growing by 8–10% annually, in contrast to an annual supply growth of only 7% (Farooqui, 2014).
By reviewing the literature, it can be inferred that the development of NZEBs is not a common practice in underdeveloped countries. Likewise, there are no set regulations and targets related to renewable energy usage in the building sector. Also, only a few studies were based on the actual measurement performance and efficiency of NZE hospitals in Asia and none in Pakistan. Furthermore, the previous studies and pre-existing energy planning modules neglect the importance of renewable energy for the commercial sector in Pakistan. Therefore, the ensuing renewable energy solutions are actually deterministic. Furthermore, the International Energy Agency (IEA) has outlined 13 tasks for the development, performance, and analysis of NZEBs in (OECD) countries. Pakistan is not an associated member of the IEA. Therefore, there is a dire need for research on energy-efficient non-residential buildings as per task 47, which is not available in Pakistan (Fritjof and Asplan Viak, 2014). Hence, the current research will be a feasibility analysis of an efficient and effective grid-connected NZEB model for a hospital using efficient optimization techniques along with intelligent simulation and modeling methods in accordance with the IEA standards in Pakistan.
The developed model of the NZE hospital would be used during the building and community project planning stages. Also, it will guide decision-makers toward much more efficient energy options and will attract investors toward a better adaptation of RE at the community level in Pakistan.
Key objectives of current research are as follows:
➢ To develop an approach for NZEB in a non-RE building in Pakistan
➢ Analysis of economic indicators and design solutions that will influence non-RE NZEBs
➢ Finding of an optimized NZEB design
➢ Feasibility of non-RE NZEBs in Pakistan.
This section is followed by six subsections as follows: building design and site selection, estimation of thermal load of the hospital building, description of the HOMER program, design of hybrid microgrid NZEB, NZEB microgrid feasibility, and optimization step.
Methodology
In this particular research, a hybrid microgrid NZEB is proposed for the hospital building situated in Taxila, Pakistan. A complete methodology is given in Figure 1. The aim of the current research is to minimize the electricity consumption expenditure of the hospital by implementing RE techniques as well as to check the feasibility of NZEBs for the Pakistani climate. So, the total thermal load is calculated for a hospital located in Taxila, Pakistan, using ASHRAE thermal load equations. Also, an energy-efficient model is developed in HOMER Pro software by using variables of NPC, operating cost, and payback period. Later on, sensitivity analysis and optimization of the parameters are performed.
Building Design
To design the building, the most important task was the selection of a site in order to perform a feasibility analysis of NZEBs in Pakistan.
Site Selection
On the basis of climate condition, Pakistan is divided into five zones as shown in Figure 2A. However, the highest temperatures range, i.e., 25–127°F exists in zone D (Salma et al., 2012). Therefore, in this study, a hospital building is selected for the conversion into NZEB in zone D in Taxila, and it has a latitude of 33.43°N and longitude 72.8397°E. In Figure 2B, the solar potential of Pakistan for different cities is highlighted. It can be clearly seen that the selected zone has a high potential for energy production and development of NZEBs in Pakistan (Solar resource maps of Pakistan, 2019).
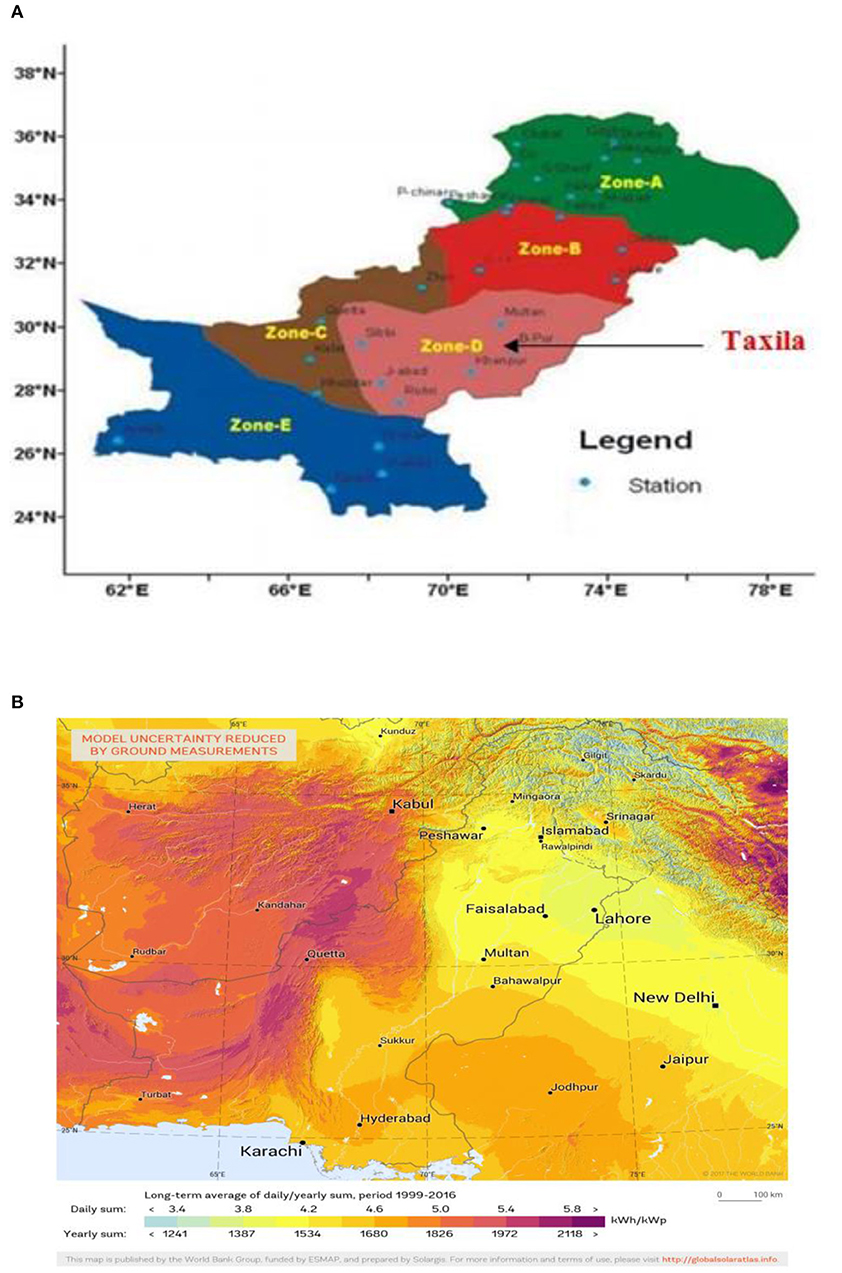
Figure 2. Zones classification of Pakistan. (A) Classification of zones on the basis of climate in Pakistan. (B) Classification of zones on the basis of solar potential in Pakistan.
Zone Identification and 3D Modeling
After selection of building type and site, a 3D model is designed in Heat Cad software as shown in Figures 3A,B. Also, the real building is illustrated in Figures 4A,B. In the current research, the hospital building is comprised of five offices, a patient ward, ICU, radiology, two laboratories, operation theater, pharmacy, and washrooms. However, identification of zones is dependent on the type of work, thermal conditions, and human comfort.
Thermal Load Assessment
The current hybrid on-grid system is designed to meet the electricity needs of a hospital building in Pakistan using solar energy as an alternative energy resource in addition to a conventional grid. The estimation of the thermal load is carried out carefully, taking into consideration the load requirements of the hospital building for the peak summer season. Design parameters for the building are given in Table 1.
July is selected as a peak month due to the high thermal load demand in the building. Design temperatures are selected on the basis of set regulation of ASHRAE for hospitals. Also, for estimation of thermal load of the building, external as well as internal loads are calculated using ASHRAE standard equations (Delisle, 2011; Lenoir et al., 2011; J, 2013) and the equation given below (Sandeep, 2015).
Internal Load
i) Occupants load
Where;
Qsensible is the sensible heat gain/person (W).
Qlatent is the latent heat gain/person (W).
N is the total number of people present in the conditioned space.
SHG is the sensible heat gain per person.
LHG is the latent heat gain per person.
ii) Heat gain from lighting
Thermal load is estimated due to lighting is given in Equation (3):
Where;
P is power provided per unit area.
External Load
iii) Heat transfer through roof (Qroof)
Similarly, heat transfer through the roof is,
iv) Heat transfer due to infiltration (Qin)
For estimation of sensible and latent heat transfer due to infiltration, firstly, the mass of infiltration is estimated by Equation (5), and, using Equations (6, 7), the total heat transfer due to infiltration is then calculated.
where
To is the outdoor dry bulb temperature
Ti is the indoor dry bulb temperature
Cp,m is the Specific heat of the moist, infiltrated air
hfg is the latent heat of vaporization of water
Wo is the outdoor humidity ratio
Wi is the indoor humidity ratio.
v) Heat transfer due to walls (Qwall)
Heat transfer by the walls is estimated by ASHRAE Equation (8). The Qwall is dependent on the contact temperature of the outer and inner environment and wall material. In Pakistan, walls are usually made of a number of layers. In the current buildings, walls are composed of plaster and 255 mm face brick. The U value is used in the overall summation of thermal resistance of each layer of the wall where
and
Uwall is the overall heat transfer coefficient.
Awall is the area of the wall.
To − Tii is the temperature difference between the outside and inside of the wall.
vi) Heat transfer through glasses (Qg)
Heat transfer by glass is high due to sun rays. Diffused or radiated sunrays play a major role in heat transfer. However, the heat transmitted is dependent on the wavelength of ray and compositional properties of glass. Qg is calculated by Equation (9)
where
Uglass is the overall heat transfer coefficient
Aglass is the area of the wall
To − Ti is the temperature difference between the outside and inside of the wall
SHGF is the solar heat gain factor
SC is the shading coefficient.
The sensible load, latent load, heat transfer by walls, doors, infiltration, appliances, and ventilation of each zone of the hospital is tabulated in Table 2.
So, the estimated total thermal load of the hospital building ought to be 51TR. Furthermore, the NZEB model of the selected hospital buildings will be developed in HOMER Pro software to check the feasibility and profitability of the hybrid NZEBs. In Figure 5, a proposed schematic for the conversion of the selected hospital into a hybrid NZE hospital is illustrated.
The proposed hybrid system consists of PV modules, batteries, and a grid. The solar system will supply power as an alternative power resource. Power generated by solar modules will be stored by batteries. In case of a shortage of power, to meet the load demand, the required power will be fulfilled by the grid. Similarly, in the case of excess power generation, power will be transmitted to the grid.
Homer Program
HOMER is one of the best tools for designing renewable-energy-based systems. Moreover, it can simulate the operations of a hybrid system for an entire year in time steps from 1 min to 1 h. It also enables us to compare and evaluate the disadvantages and advantages of economic and technical features. In HOMER, the design process is performed in three steps: simulation, optimization, and sensitivity analysis. In this paper, all three processes are followed to get the best overview of the feasibility of NZEBs in Pakistan.
Model Development of Hybrid NZEB Hospital Building
NZEB model development of the current hospital building in HOMER is illustrated in Figure 6. The yearly thermal load and solar irradiation potential of the building are the input parameters for HOMER.
Available Solar Radiations
For the NZEB feasibility analysis, the available solar radiations are estimated for the selected location in Pakistan. For the location of latitude 33.43°N and longitude 72.8397°E, maximum solar radiation and average solar radiation were estimated to be 5.5 and 5 kWh/m2/day, respectively. The current position has excellent solar potential for producing energy using PV for the development of NZEB. Figure 7 shows solar radiation and a clearness index profile by using HOMER Pro.
In this study, the first step was to estimate yearly thermal load and defining peak load season. So, using statistical data obtained by Solarimeter and performing simulation in HOMER Pro, the daily load profile of the hospital in Taxila, Pakistan, was obtained and is shown in Figure 8. According to estimated thermal data, in the month of July, the energy demand is highest due to high usage of air-conditioning. The monthly profile of thermal data is given in Figure 8. Figure 8 also presents the highest and lowest energy consumption in every month of the entire year.
Additionally, it can be seen that the hospital building reaches an optimum energy consumption of about 177 kW in the summer months, i.e., June, July, and August. Figure 8 shows the thermal load profile of each month simulated in HOMER Pro. The total thermal load estimated for the hospital building in Taxila is 53 Ton. This is the required need of air-conditioning of the building.
Hourly Load Demand Curves
The thermal load profile is generated for 24 h, 365 days a year, in HOMER Pro. Thermal load profile depends on calculated thermal data and input solar data of the selected location. There are various types of thermal loads, i.e., fan, CFL, printers, computers, ECG machine, BP apparatus, X-ray machine, and TV, and they are used in hospital buildings. The electrical load consumption of the building for 24 h is calculated. Figure 9 shows scaled thermal load data 24 h per day for the entire year.
PV System Design
Solar radiation is converted directly into DC by using PV modules. So, the deviation of the output of the electrical power produced by PV modules is estimated using Equation (10). The output energy of a PV module is dependent on several parameters, including the following: the structure of cell type, nominal performance of the PV panel, solar radiation, derating factor, solar cell temperature, climatic conditions at the panel area, temperature coefficient, and weather conditions (Fritjof and Asplan Viak, 2014).
where;
YPV is the rated capacity of the PV array, meaning its power output under standard test conditions (kW),
fpv is the PV derating factor (%),
is the solar radiation incident on the PV array in the current time step (kW/m2),
GT,STC is the incident radiation at standard test conditions (1 kW/m2),
αP is the temperature coefficient of power (%/°C),
TC is the PV cell temperature in the current time step (°C), and
TC,STC is the PV cell temperature under standard test conditions (25°C).
Grid Information
In this study, PV panels and converters are two main components of the system. A proposed system is designed, and it consists of 220 kW PV modules and 226 kW converters shown in Figure 9. According to the results, for the hospital building, a peak load of 319.2 kW is observed. However, the average electricity consumption is 3336.07 kWh/d.
Design of Solar Module
In this study, 220 kW solar modules are used in combination with multiple PV solar panels. PV modules are polycrystalline and have a maximum rated power of 250 W, a nominal tension of 29.8 V, and an operating current of 8.71 A. Also, the installation cost of solar modules in Pakistan is thought to be 76,154 USD/kW with a life of 25 years. In Table 3, all the design parameters for the solar system are given.
Jinko solar manufacturers are the top-rated solar component providers in Pakistan. Moreover, flat-plate PV modules can be installed after the designing phase. The estimated capital cost is $63,462, the replacement cost is $3,173, the O&M cost is $1,7628/y, the hours of operation are 4,385/y, the PV penetration is 29%, and the total lifespan of the modules is 25 years, as shown in Figure 10. Also, Figure 11 explains the mean output of solar modules (40.3 kW), maximum output (174 kW), and the capacity factor of energy production (21.8%).
Converter
For the conversion of DC to AC, a converter is used. This function is executed using a bidirectional energy converter within a microgrid. As per the generated output, usage, and storage factors of electrical energy in microgrid, the converter functions as both a rectifier and an inverter. Usually, a converter has 80% efficiency and is evaluated on the basis of solar module selection.
A hybrid NZEB system is proposed when undergoing research in order to share Peak thermal load. Moreover, nine converters of 26 kW capacities were selected for the PV field of 226 kW. The initial cost of one converter is 18.35 USD/kW, and it provides an efficiency of 90%.
Optimization Process
The aim of the current research was to obtain an optimized hybrid NZE system for a hospital building. HOMER performs several optimizations under a range of input variables to determine the effect of uncertainty. Optimization estimates the optimal value of variables on which the system has the best performance (Fritjof and Asplan Viak, 2014). HOMER gives the best possible combination of modules that will fulfill particular electrical and thermal loads (Jaman et al., 2010). Figure 12 shows a flowchart of the optimization process for obtaining an optimized NZEB system for hospital building using HOMER in this study.
For optimum results using HOMER software, the selected variables are thermal load and solar data. For calculation of discount factor, the discount rate is 15%,the rate of inflation is 6.8%, the fuel cost escalation rate is 4.10%, and the interest rate is 11% (Giyler and Lilienpthal, 2005; Tahir Masood and Shah, 2012; Singh et al., 2015) in Pakistan. Solar panels generally have a life between 25 and 30 years (Sen and Bhattacharyya, 2014). Table 4 shows constraints used in optimization.
Results and Discussion
Economic evaluation for NZEB conversion of a hospital building is performed by three main operations of HOMER software: simulation, optimization, and sensitivity analysis. In the current section, different obtained results will be discussed.
Cost and Production Details of PV Panels and Converters
By way of careful analysis, the price of the 325 W panel in Pakistan is found to be $43.66USD. Additionally, the inverter mean output is 37.4 kW, the capital cost $0.082 million, and the production by PV panels ranged from 280,498 to 344,733 kWh for different solar irradiances given in Table 5.
Influence of Economic Indicators on Feasibility of Non-re NZEBs
In Table 6, the NPC, operating costs, initial capital costs, and sell-back rate are given. NPC ranges from $20.41 to $21.63 million. The operating cost for the whole installation is $0.151 million. However, initial capital cost is $0.111 million. The sell-back rate after adaptation of the NZEB system is 0.11 USD/kWh.
Optimized NZEB Design
Table 7 shows the best optimal values for the solar-powered optimal system for the selected buildings in terms of the NPC, operating cost, and capital cost.
The optimized NZEB system has a capital cost of $0.082 million, operating cost of $0.15 million, and an optimized NPC value of $2.04 million. Additionally, the sell-back rate is 0.11 USDkW.
Electricity Generation Comparison Between PV and Grid
Figure 13 is a representation of the monthly electricity production by PV and grid. The green bar shows the power generated by PV, whereas the orange bar represents power produced by the grid. In the proposed system, 70.7% of the production is due to PV and 28.3% is produced by the grid, as shown in Figure 12. Total operational hours are 4,385 in a year. In Pakistan, the unit price of electricity from the grid is 0.14 USD/kWh; however, the unit price of electricity obtained by hybrid PV is thought to be 0.12 USD/kWh. Additionally, the obtained total cost of production of electricity by the hybrid system is ~$0.102 million. Though, initially, when the system was operating only by grid, the annual cost of production of electricity was $0.114 million. So, by installing the hybrid NZEB system, an annual profit of 10.24% can be obtained. Similarly, in another study of the optimization process for the estimation of energy efficiency of drying plant. The use of a thermodynamics laws analysis was 35.40% efficient, which is 10.11% more efficient (Taner, 2015b).
Feasibility of Commercial NZEBs in Pakistan
The feasibility of any project depends on the percentage of the profit and payback period. This section describes the feasibility of commercial NZEBs in Pakistan.
Cost of Electricity (COE)
Figure 14 shows the surface plots of the cost of electricity (COE) in HOMER Pro. The cost of energy is reduced with the increase in the intensity of solar radiation. Also, the COE is reduced from $0.135 to $0.128 million for selected buildings.
Payback Period
After the optimization of the system, the basic objective of the research was to check the feasibility and profitability of the proposed hybrid system. HOMER Pro software works as a black box and simulates several input data. According to the results as shown in Figure 15. the payback period of the proposed system is 2.53y after the installation of the 185 kW hybrid PV system, while the return on investment is 38.9%.
Conclusion
The current study is an economic evaluation and feasibility analysis of hybrid microgrid NZEB. A hybrid PV power system is created to supply energy to the hospital building located in Taxila, Pakistan. The objectives were to decrease the total electricity price and feasibility analysis of hybrid NZEBs in Pakistan for non-residential buildings. To attain maximum advantage from the proposed hybrid NZEB system, the designing of the building, calculation of thermal load, simulation, and optimization of the suggested hybrid microgrid NZEB were all performed in HOMER Pro. An optimal range of design parameters was acquired using thermal data and solar irradiance as input. Additionally, an economic analysis was performed to evaluate the feasibility of the proposed hybrid system over the duration of 25y. The main findings of the current system were as follows: solar radiation potential, operating cost, initial capital cost, the energy produced by PV, payback period, and net present cost. The optimal NPC of the proposed NZEB is $20.49 million, the operating cost for the whole installation of a hybrid NZEB system is $0.151 million, and the initial capital cost is $0.111 million. By adaptation of the hybrid NZEB system, the electricity unit price has dropped from 0.14 to 0.12 USD/kWh. The maximum electricity generation by PV panels is 344,733 kWh. Consequently, the proposed hybrid NZEB system is economically 10.24% more profitable than the grid system only. Moreover, the proposed NZEB system has a payback period of 2.53y, thereby making it a cost-efficient and economically feasible method. Primarily, on the basis of the analysis of all the above-stated variables, it may be concluded that the current design is able to satisfy the aim and objectives of the current research by making renewable energy a viable solution to the energy shortages in Pakistan. The developed framework will assist in the decision-making of feasibility analyses and economic evaluation of NZEBs in Pakistan. Also, it will facilitate municipal and provincial planners in NZE community planning. This work will be further supported by the implementation of a federal renewable energy plan, which could act as a long-term roadmap for sustainable community planning.
Data Availability Statement
The original contributions presented in the study are included in the article/supplementary material, further inquiries can be directed to the corresponding author/s.
Author's Note
By literature review it can be inferred that development of NZEBs is not a common practice in underdeveloped countries. Likewise, there is no set regulations and targets related to renewable energy usage in building sector. Also, only a few researches were based on the actual measurement performance and efficiency of NZE hospitals in Asia and none in Pakistan. Furthermore, the previous studies and pre existing energy planning modules neglect the importance of renewable energy for commercial sector in Pakistan. Therefore, the ensuing renewable energy solutions are actually deterministic. Moreover, IEA have outlined 13 tasks for the development, performance and analysis of NZEBs in (OECD) countries. Pakistan is not an associated member of the International Energy Agency (IEA). Therefore, there is a dire need of research on energy efficient non residential buildings as per task 47 which is not available in Pakistan. Hence, current research will be the feasibility analysis of an efficient and effective grid connected NZEB model for a hospital using efficient optimization technique along with intelligent simulation and modeling method in accordance with the International Energy Agency (IEA) standards in Pakistan.
Author Contributions
All authors listed have made a substantial, direct and intellectual contribution to the work, and approved it for publication.
Conflict of Interest
The authors declare that the research was conducted in the absence of any commercial or financial relationships that could be construed as a potential conflict of interest.
References
AIA (2011). The 2030 Commitment. Available online at: https://architecture2030.org/2030_challenges/2030-challenge/ (assessed October 11, 2019).
AlAjmi, A., Abou-Ziyan, H., and Ghoneim, A. (2016). Achieving annual and monthly net-zero energy of existing building in hot climate. Appl. Energy 165:511–521. doi: 10.1016/j.apenergy.2015.11.073
Alfano, F. R. D., Olesen, B. W., and Palella, B. I. (2017). Povl ole fanger's impact ten years later. Energy Build. 15, 243–249. doi: 10.1016/j.enbuild.2017.07.052
Alfano, F. R. D. A., Olesen, B. W., Palella, B. I., and Riccio, G. (2014). Thermal comfort: design and assessment for energy saving. Energy Build. 81, 326–336. doi: 10.1016/j.enbuild.2014.06.033
Alhorr, Y., Arif, M., Bano, T., Egbu, C., Mazroei, A., and Elsarrag, E. (2015). Creating a better healing environment in Qatari healthcare sector: exploring the research agenda for the future. Int. J. Sustain. Built. Environ. 4, 158–164. doi: 10.1016/j.ijsbe.2015.03.007
Azhar, S., Carlton, W. A., and Olsen, D. (2011). Ahmad, I. Building information modeling for sustainable design and LEED rating analysis. Autom. Constr. 20, 217–224. doi: 10.1016/j.autcon.2010.09.019
Balaras, C. A., Dascalaki, E., and Gaglia, A. (2007). HVAC and indoor thermal conditions in hospital operating rooms. Build. Environ. 39, 454–470. doi: 10.1016/j.enbuild.2006.09.004
Chen, S., Li, N., Guan, J., Xie, Y., Sun, F., and Ni, J. (2008). A statistical method to investigate national energy consumption in the residential building sector of China. Energy Build 40, 654–665. doi: 10.1016/j.enbuild.2007.04.022
Del Ferraro, S., Iavicoli, S., Russo, S., and Molinaro, V. (2015). A field study on thermal comfort in an Italian hospital considering differences in gender and age. Appl. Ergon. 50, 177–184. doi: 10.1016/j.apergo.2015.03.014
Delisle, V. (2011). “Net-zero energy homes: solar photovoltaic electricity scenario analysis based on current and future costs,” in ASHRAE Conference Montreal, 1–8. Available online at: http://www.nrcan.gc.ca/sites/www.nrcan.gc.ca/files/canmetenergy/files/2011-040.pdf
Dutch governments. (2012). Nationaal Plan voor het bevorderen van Bijna-EnergieNeutrale Gebouwen (BENG) in Nederland.
Entrop, A., and Brouwers, H. (2019). Assessing the sustainability of buildings using a framework of triad approaches. J. Build. Apprais. 5, 293–310. doi: 10.1057/jba.2009.36
Farooqui, S. K. (2014). Prospects of renewable penetration in the energy mix of Pakistan. Renew. Sustain. Energy Rev. 29, 693–700. doi: 10.1016/j.rser.2013.08.083
Ferreira, J., Pinheiro, M. D., and Brito, J. D. (2014). Portuguese sustainable construction assessment tools benchmarked with BREEAM and LEED: an energy analysis. Energy Build. 69, 451–463. doi: 10.1016/j.enbuild.2013.11.039
Fritjof, S., and Asplan Viak, A. S. (2014). SHC Task 47 Renovation of Non- Residential Buildings Towards Sustainable Standards. Avaialbel online at: https://task47.iea/shc.org/Data/Sites/1/publications/IEA/SHC/Task47/Highlights/2014.pdf (assessed December 10, 2019).
Giyler, T., and Lilienpthal, P. (2005). Using HOMER Software, NREL's Micro Power Optimization Model, to Explore the Role of Gen-Sets in Small Solar Power Systems Case Study: Pakistan. Technical Report NREL/TP-710-36774.
Golbazi, M., and Aktas, C. B. (2016). Analysis of credits earned by LEED healthcare certified facilities. Proc. Eng. 145, 203–210. doi: 10.1016/j.proeng.2016.04.062
Han, J., Zhang, G., Zhang, Q., Zhang, J., Liu, J., Tian, L., et al. (2007). Field study on occupants' thermal comfort and residential thermal environment in a hot-humid climate of China. Build. Environ. 42, 4043–4050. doi: 10.1016/j.buildenv.2006.06.028
Haves, P. (2019). A study on energy savings and measure cost effectiveness of existing building commissioning.
Jaman, M. T., Rahman, M. M., Rahman, A. M., and Khulna, B. (2010). “Design of a direct gain passive solar heating system,” in Paper Presented At The Proceedings Of 2010 International Conference on Industrial Engineering and Operations Management (Dhaka).
Ji, R., Zhang, Z., He, Y., Liu, J., and Qu, S. (2017). Simulating the effects of anchors on the thermal performance of building insulation systems. Energy Build. 140, 501–507. doi: 10.1016/j.enbuild.2016.12.036
Jian, Z., Pullen, S., Rameezdeen, R., Bennetts, H., Wang, Y., Mao, G., et al. (2017). Green building evaluation from a life-cycle perspective in Australia: a critical review. Renew. Sustain. Energy Rev. 70, 358–368. doi: 10.1016/j.rser.2016.11.251
Khalid, W., Salim, S. A. Z. S., Rijal, H. B., and Yakub, F. (2017). “Thermal comfort in hospitals in hot and humid climates: a literature review,” in Proceedings of the 1st Green Environmental Energy and Building Science (GEEBS) International Conference (Johor Bahru), 16–17.
Khan, H. A., and Pervaiz, S. (2013). Technological review on solar PV in Pakistan: scope, practices and recommendations for optimized system design. Renew. Sustain. Energy Rev. 23, 147–154. doi: 10.1016/j.rser.2013.02.031
Kneifel, J., and Webb, D. (2018). Predicting energy performance of a net-zero energy building: a statistical approach. Appl. Energy. 178, 468–483. doi: 10.1016/j.apenergy.2016.06.013
Lenoir, A., Thellier, F., and Garde, F. (2011). “Towards net zero energy buildings in hot climate, part 2: experimental feedback,” in ASHRAE Transactions, Vol. 117.
Liu, Y., Guo, X., and Hu, F. (2014). Cost-benefit analysis on green building energy efficiency technology application: a case in China. Energy Build. 82, 37–46. doi: 10.1016/j.enbuild.2014.07.008
Meegoda, J., Hsieh, H. N., Rodriguez, P., and Jawidzik, J. (2012). Sustainable community sanitation for a rural hospital in Haiti. Sustainability 4, 3362–3376. doi: 10.3390/su4123362
Ministerie van Volkshuisvesting, Ruimtelijke Ordening en Milieubeheer. (2010). Available online at: https://www.parlement.com/id/vhnnmt7hw7e7/ministerie_van_volkshuisvesting (accessed November 20, 2020).
Ministry of Planning and Development Government of Pakistan. (2011). “Pakistan Integrated Energy Model (Pak-IEM),” in Policy Analysis Report Volume II (Islamabad).
Pourshaghaghy, A., and Omidvari, M. (2012). Examination of thermal comfort in a hospital using PMV-PPD model. Appl. Ergon. 43, 1089–1095. doi: 10.1016/j.apergo.2012.03.010
Pradinuk, R. (2009). Incentivizing the Daylit Hospital: the green guide for health care approach. HERD Health Environ. Res. Des. J. 2, 92–112. doi: 10.1177/193758670900200408
Rafique, M. M., and Rehmanb, S. (2017). National energy scenario of Pakistan: current status, future alternatives and institutional infrastructure: an overview. Renew. Sustain. Energy Rev. 69, 156–167. doi: 10.1016/j.rser.2016.11.057
REHVA. (2010). EU, Directive 2010/31/EU of the European Parliament and of the Council “REHVA EPBD recast, of 19 May 2010 on the energy performance of buildings,” in Official Journal of the European Union.
Raheem, S. A., Abbasi, A., Memon, A., Samo, S. R., Taufiq-Yap, Y. H., Danquah, M. K., et al. (2016). Renewable energy deployment to combat energy crisis in Pakistan,|| Energy. Sustain. Soc. 6:16. doi: 10.1186/s13705-016-0082-z
Salma, S., Rehman, S., and Shah, M. A. (2012). Pakistan Journal of Meteorology:Rainfall Trends in Different Climate Zones of Pakistan. Pakistan J. Meteorol. 9:39.
Sattayakorn, S., Ichinose, M., and Sasaki, R. (2017). Clarifying thermal comfort of healthcare occupants in tropical region: a case of indoor environment in Thai hospitals. Energy Build. 149, 45–57. doi: 10.1016/j.enbuild.2017.05.025
Schweber, L. (2013). The effect of BREEAM on clients and construction professionals. Build. Res. Inf. 41, 129–145. doi: 10.1080/09613218.2013.768495
Schweber, L., and Haroglu, H. (2014). Comparing the fit between BREEAM assessment and design processes. Build. Res. Inf. 42, 300–317. doi: 10.1080/09613218.2014.889490
Sen, R., and Bhattacharyya, S. (2014). Off-grid electricity generation with renewable energy technologies in India: an application of HOMER. Renew. Energy 62, 388–453. doi: 10.1016/j.renene.2013.07.028
Sherif, A. H. (1999). Hospitals of developing countries: design and construction economics. J. Archit. Eng. 5, 74–81. doi: 10.1061/(ASCE)1076-0431(1999)5:3(74)
Shu, Y., Ito, K., and Yokoyama, R. (2007). Sensitivity analysis in structure optimization of energy supply systems for a hospital. Energy Conv. Manag. 48, 2836–2843. doi: 10.1016/j.enconman.2007.06.045
Si, B., Tian, Z., Chen, W., Jin, X., Zhou, X., and Shi, X. (2018). Performance assessment of algorithms for building energy optimization problems with different properties. Sustainability 11:18. doi: 10.3390/su11010018
Singh, A., Baredar, P., and Gupta, B. (2015). Computational simulation & optimization of a solar, fuel cell and biomass hybrid energy system using HOMER Pro software. Proc. Eng. 127, 743–750. doi: 10.1016/j.proeng.2015.11.408
Skoog, J., Fransson, N., and Jagemar, L. (2005). Thermal environment in Swedish hospitals: summer and winter measurements. Energy Build. 37, 872–877. doi: 10.1016/j.enbuild.2004.11.003
Solar resource maps of Pakistan. (2019). Available online at: https://solargis.com/maps-and-gis-data/download/Pakistan (accessed March 11, 2021).
Tahir Masood, M., and Shah, F. (2012). Dilemma of third world countries – problems facing pakistan energy crisis a case-in-point. Int. J. Bus. Manag. 7:231. doi: 10.5539/ijbm.v7n5p231
Taneer, T. (2018). Energy and exergy analyze of PEM fuel cell: a case study of modeling and simulations. Energy 143, 284–294. doi: 10.1016/j.energy.2017.10.102
Taneer, T., Naqvi, S. A. H., and Ozkaymak, M. (2018). Techno-economic analysis of a more efficient hydrogen generation system prototype: a case study of PEM electrolyzer with Cr-C coated SS304 bipolar plates. Fuel Cells. 19, 19–26. doi: 10.1002/fuce.201700225
Taneer, T., and Sivrioglu, M. (2017). A techno-economic & cost analysis of a turbine power plant: a case study for sugar plant. Renew. Sustain. Energy Rev. 78, 722–730. doi: 10.1016/j.rser.2017.04.104
Taner, T. (2015b). Optimisation processes of energy efficiency for a drying plant: a case of study for Turkey. Appl. Therm. Eng. 80, 247–260. doi: 10.1016/j.applthermaleng.2015.01.076
Taner, T. (2015a). Alternative energy of the future: a technical note of PEM fuel cell water management. J. Fundament. Renew. Energy Appl. 5:3. doi: 10.4172/2090-4541.1000163
Vanhoutteghem, L., Skarning, G. C. J., Hviid, C. A., and Svendsen, S. (2015). Impact of façade window design on energy, day lighting and thermal comfort in nearly zero-energy houses. Energy Build. 102, 149–156. doi: 10.1016/j.enbuild.2015.05.018
Wang, Q., Zhou, P., Zhao, Z., and Shen, N. (2014). Energy efficiency and energy saving potential in China: a directional meta-frontier DEA approach. Sustainability 6, 5476–5492. doi: 10.3390/su6085476
Wu, P., Song, Y., Shou, W., Chi, H., and Chong, H. Y. (2017). Sutrisna, M. A comprehensive analysis of the credits obtained by LEED 2009 certified green buildings. Renew. Sustain. Energy Rev. 68, 370–379. doi: 10.1016/j.rser.2016.10.007
Wu, W., and Skye, H. M. (2018). Domanski PA. Selecting HVAC systems to achieve comfortable and costeffective residential net zero energy buildings. Appl. Energy 212, 577–591. doi: 10.1016/j.apenergy.2017.12.046
Yau, Y. H., and Chew, B. T. (2009). Thermal comfort study of hospital workers in Malaysia. Indoor Air 19, 500–510. doi: 10.1111/j.1600-0668.2009.00617.x
Younger, M., Morrow-Almeida, H. R., Vindigni, S. M., and Dannenberg, A. L. (2008). The built environment, climate change, and health: opportunities for co-benefits. Am. J. Prev. Med. 35, 517–526. doi: 10.1016/j.amepre.2008.08.017
Zhang, Y., Wang, J., Hu, F., and Wang, Y. (2017). Comparison of evaluation standards for green building in China, Britain, United States. Renew. Sustain. Energy Rev. 68 (Pt 1), 262–271. doi: 10.1016/j.rser.2016.09.139
Zhou, Z., Feng, L., Zhang, S., Wang, C., Chen, G., Du, T., et al. (2016). The operational performance of “net zero energy building”: a study in China. Appl. Energy 177, 716–728. doi: 10.1016/j.apenergy.2016.05.093
Zurghulpatias. (2015a). Overzicht Algemene Ziekenhuizen in Nederland. Available online at: http://www.zorghulpatlas.nl/zorginnederland/ziekenhuiszorg/algemeneenacademische/ziekenhuizen/overzicht/algemeneziekenhuizen/innederland/ (accessed December 17, 2017).
Zurghulpatias. (2015b). Academische Ziekenhuizen in Nederland. Available online at: http://www.zorghulpatlas.nl/zorg/innederland/ziekenhuiszorg/algemene/enacademische/ziekenhuizen/academischeziekenhuizen/in/nederland/ (accessed December 17, 2018).
Nomenclature
Keywords: Net Zero Energy Building, hospital, micro grid, feasibility analysis, payback period, cost of electricity
Citation: Arif S, Taweekun J, Ali HM and Theppaya T (2021) Techno Economic Evaluation and Feasibility Analysis of a Hybrid Net Zero Energy Building in Pakistan: A Case Study of Hospital. Front. Energy Res. 9:668908. doi: 10.3389/fenrg.2021.668908
Received: 17 February 2021; Accepted: 15 March 2021;
Published: 30 April 2021.
Edited by:
Mohammad Hossein Ahmadi, Shahrood University of Technology, IranCopyright © 2021 Arif, Taweekun, Ali and Theppaya. This is an open-access article distributed under the terms of the Creative Commons Attribution License (CC BY). The use, distribution or reproduction in other forums is permitted, provided the original author(s) and the copyright owner(s) are credited and that the original publication in this journal is cited, in accordance with accepted academic practice. No use, distribution or reproduction is permitted which does not comply with these terms.
*Correspondence: Juntakan Taweekun, Juntakantaweekun@yahoo.com