1 Introduction
Since stone age, humans use fuels, defined as any energy carriers intended for energy conversion (Food and Agriculture Organization of the United Nations, 2004; International Organization for Standardization, 2014). First evidence of the use of domesticated fire was established in 790,000 B.C. (Alperson-Afil and Goren-Inbar, 2010). Thus, biomass has been the first fuel used by the human kind for security, cooking and heating. Nowadays, most of the used energy sources are fossil fuels. In 2019, oil, coal and gas represented respectively 31%, 25%, and 23% of the global primary energy consumption (Our World in Data, 2021). Despite their great advantage, high energy density, these fuels have a major drawback: their combustion releases huge quantities of carbon dioxide (35 Gt of CO2 in 2019) mainly responsible for climate change (International Energy Agency, 2020b). The biggest challenge of the energy transition is to secure the energy supply while reducing the greenhouse gas emissions. In practice, this means finding alternatives to fossil fuels.
First and foremost, in the context of the energy transition, fuels will keep playing a major role in the global energy system (Ahlgren, 2012). Even if electricity gains shares through the electrification of the energy demand, it will not entirely displace fuels for three main reasons: storage, infrastructure compatibility and cross-sectorial links. Because of their intermittency and space disparity, a deeper integration of variable renewable energy sources (VRES) requires storage and transport in order to supply the energy demand at the right time and in the right place (Hall and Bain, 2008; Evans et al., 2012; Brouwer et al., 2016; Gallo et al., 2016; Rosa, 2017). Where typical container of batteries are limited in terms of storage capacity (up to 10 MWh) and present significant costs and self-discharge losses, the energy conversion into fuels provides a more affordable solution for higher storage capacity (from 100 GWh) and longer storage time scales (months to year) (Rosa, 2017). Due to the economic inertia and their infrastructure legacy (Ahlgren, 2012), fuels remain the most appropriate solution for sectors requiring high energy density (e.g. heavy-duty transport, shipping, aviation or chemical industry) (Zeman and Keith, 2008; Pearson et al., 2012; Rosa, 2017; Goede, 2018; Trieb et al., 2018; Decker et al., 2019; Albrecht and Nguyen, 2020; Stančin et al., 2020). Contino et al. (2020) point out that the energy transition is an interdisciplinary effort and not solely about the power sector. The latter represents only a fifth of the global energy consumption (International Energy Agency, 2020a). Also, Goede (2018) has shown in 2018 that CO2 emissions of the Netherlands are equally apportioned between the different types of end-use-demands (i.e. power, heat, mobility and non-energy). This highlights the necessity to consider every energy sector rather than focusing all efforts on the power system and even more, to shift toward a multi-vector interconnected energy system. In this cross-sectorial approach and with the perspective of increasing shares of VRES, fuels are promising energy carriers in order to maximise the overall system efficiency (Mathiesen et al., 2015; Stančin et al., 2020).
Given the growing diversity of pathways for transforming the renewable energy sources into fuels, clear classification and terminology are necessary (Bailera et al., 2017). As predicted by Ridjan et al. (Ridjan et al., 2016), there is now a need to support the right fuel technology development, via the use of a more comprehensive and quantitative terminology (e.g. specifying the share of biomass in the energy balance of the production of a biofuel). The objective of this terminology is to avoid the confusion between these fuels and thereby reduce misunderstanding in political or academic discussions. To a further extent, such an harmonisation may help “to facilitate comparison between national fuel market and enhance trading” (Food and Agriculture Organization of the United Nations, 2004). It also aims at promoting and increasing the use of biomass as energy carriers and electricity transformed into fuel. On a general public perspective, this terminology aims at simplifying and clarifying the addressed terms and allows to have a more critical mind. In Section 2, we present the terminology currently used in the literature. On top of this review of the scientific literature, this work also includes review of terminology defined by the International Organization for Standardization (ISO) and other official institutions (e.g. the Food and Agriculture Organization of the United Nations (FAO) or the International Energy Agency (IEA)) and presents the motivation for a new one, more quantitative and measurable. The latter is introduced in Section 3. Finally, Section 4 gives the room for a discussion and the actual recommendations.
2 Terminology Used in the Literature
Ridjan et al. (Ridjan et al., 2016) performed a first literature review between 2006 and 2014, looking in Scopus for the three keywords: (i) “synthetic fuels”, (ii) “electrofuels” and (iii)“alternative fuels.” Based on this extensive review work, they proposed the following definitions: (i) Synthetic fuels were defined as x-To-Liquid (xTL) processed fuels, within the scope of “Fischer-Tropsch fuels that are produced by gasification of either coal, natural gas or biomass.” Comparatively, similar definitions are given in (EIA, 2006) and (International Energy Agency, 2007), except that the latter excludes biomass from the potential feedstocks. (ii) Electrofuels were considered as a storage capacity of electricity into chemical bonds through so called coal-, biomass- and emission(CO2)-to-electrofuel (xTE) processes. (iii) In accordance with (European Commission, 2013), they presented alternative fuels as any fuel used as a substitution for fossil oil sources in the energy supply with no specific restrictions regarding the feedstock.
More recently, Stančin et al. (2020) has shown that scientific research about “alternative fuels” has experienced a significant impulse since the 2000s. Therefore, in the continuity of (Ridjan et al., 2016), we performed a similar literature review in order to update the terminology used by the scientific community. Hence, the current work extends the previous review to cover publications from 2015 to 2020. The same methodology was followed, through a manual identification, and the same search terms were looked for in Scopus: the first search screened the terms of interest (i.e. synthetic fuels or electrofuels) complemented by narrowing-down terms (e.g. “alternative fuels,” “hydrocarbon,” “biomass,” or “ammonia”). This initial search resulted in 251 documents. Then, after screening the abstract (and, if necessary, the articles themselves), 75 relevant documents were selected as they used the searched terminology and gave an actual definition as well as identified the production process. The non-relevant documents usually contained information that fall out of the scope of the current work, e.g. information on specific catalysts. Out of the relevant documents, the main outcomes concern the relative majority of “synthetic fuels,” the emergence of “electrofuels” and the scattered diversity of other terms.
Where terms like “synthetic gas,” “electrofuels” or “synthetic natural gas” account for 19%, 13% and 8% of the additional relevant literature, respectively, “synthetic fuels” is used in 35% of the cases to cover fuels produced from a wide variety of primary resources (renewable or not) and through various conversion processes. To pick only a few examples, its usage ranged from liquid hydrocarbons produced from synthesis gas through the Fischer–Tropsch process (Haarlemmer et al., 2014; Trieb et al., 2018) to ammonia from the Haber-Bosch process (Bargiacchi et al., 2019) or biomass gasification or pyrolysis (Rao, 2015; Monaco et al., 2018). As a consequence of an increasing share of VRES in the energy system, “electrofuels” are gaining more popularity: where this term was used in 4% of the relevant literature between 2006 and 2014 (Ridjan et al., 2016), it is now present in 13% of the additional relevant literature reviewed for this work. These fuels are considered as being produced mainly based on the electricity provided by VRES (Brynolf et al., 2018). Besides water electrolysis, this electricity also supplies processes like CO2 hydrogenation (Pearson et al., 2012; Brynolf et al., 2018; Decker et al., 2019) or co-electrolysis of CO2 and H2O (Larsson et al., 2015; Hänggi et al., 2019). Aside the two aforementioned keywords, the literature makes use of a scattered diversity of terms that aim at precising the features of the considered fuel: for instance, “solar fuel,” “power gas” or “green synthetic fuel.” However, out of context, words like “green fuel,” “advanced fuel” or “solar fuel” may be misunderstood because of their multiple interpretations (Ridjan et al., 2016).
Consequently, in the context of the energy transition where new use of primary resources and conversion technologies are booming, we encompass in this work all the resources and conversion processes one might consider in the production of fuels. The objective of this taxonomy is to fit with the long-term perspective of this transition (e.g. carbon-neutrality, defossilisation) while being adapted for the current context. We do not only focus on carbon-based fuels nor on specific end-use sectors (e.g. transport), contrary to what is mostly seen in the literature. We propose a comprehensive and harmonised taxonomy, without having the ambition to apply strict classification methodologies (e.g. questionnaires, text-mining of corpora of the domain) (National Center for Biotechnology Information, 2021).
3 Classification and Definition of the Fuels
Given the difficulty to quantify and circumscribe the sustainability of a fuel, we decided here to suggest classification and definition of the fuels regardless of this feature. As described below, we opted for a focus on the energy balance of a fuel with regard to its chemical building blocks and the origin of the energy to supply the conversion and production process, without having the ambition to quantify the sustainability of the different fuels.
An essential distinction has to be pointed out between renewable and non-renewable fuels. In accordance with (EU-Commission, 2003), renewable fuels are fuels produced from renewable energy sources. Renewable energy sources are non-fossil sources that are naturally replenished on a human timescale (wind, solar, geothermal, wave, tidal, hydro-power, biomass) (Ellabban et al., 2014). A fuel can be defined as “renewable” only when it is based on renewable sources. To be “sustainable,” a “renewable fuel” should not increase the concentration of CO2 in the atmosphere (Dincer and Bicer, 2020), among other things (European Commission, 2019b). On the contrary, non-renewable fuels are those that are not based on renewable sources. In practice, these cluster fuels produced from fossil sources (e.g. coal, natural gas, oil). Finally, the nuclear-based fuels are considered separately. Even though nuclear power plants do not emit CO2 to produce electricity or heat, uranium is not a renewable resource.
Given these definitions and looking at the primary resources, the distinction is clear between renewable and non-renewable sources. However, the production and end-use fuels are harder to group as they can use both renewable and non-renewable resources. In other words, the same end-use fuel can be produced from different resources by different processes, which explains the ambiguity in the definition of these fuels. As illustrated on Figure 1, hydrogen is a good example as it can be produced from fossil or renewable resources and by different processes: for instance, (i) steam reforming of fossil natural gas (75% of the current global production of hydrogen today (International Energy Agency, 2019)); (ii) partial oxidation of heavy hydrocarbons; (iii) coal gasification; (iv) electrolysis with electricity generated from fossil fuels; (v) electrolysis with electricity generated from renewable sources; (vi) thermochemical conversion of ligno-cellulosic biomass; (vii) steam reforming of biogas or even (viii) thermochemical water splitting using heat from concentrated solar power or nuclear wastes (Acar and Dincer, 2014).
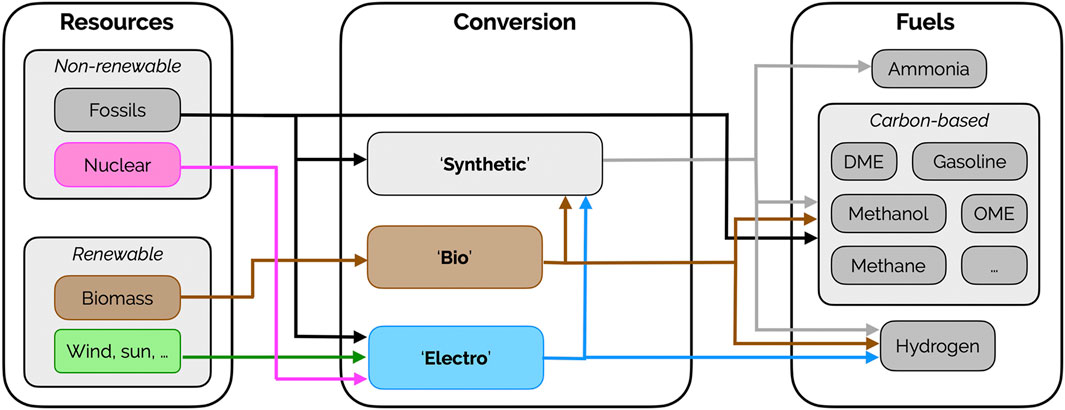
FIGURE 1. Overview of the main pathways to produce fuels. The boxes in the “conversion” category illustrates the terminology to use in the different cases: first, a fuel can be defined as bio or electro if the absolute majority of the energy balance of its production consists of biomass or electricity, respectively. Then, a fuel can be defined as synthetic if it has been obtained through a synthesis process from another fuel, regardless of the feedstock. The box “Fuels” gives some examples to illustrate the terminology used and applied to very common fuels.
Therefore, besides the aforementioned renewable/non-renewable distinction, we propose to categorise fuels in three groups whose definitions are broad enough to capture the diversity of the fuels while maintaining a simplified representation: (i) Biofuels as the absolute majority of the energy balance of their production consists of biomass; (ii) Electrofuels, similarly to biofuels, but with electricity instead of biomass. A priori, it is complicated to give an exact number to quantify this “majority” of the energy balance but we propose a solution in Section 4 to remove this ambiguity. (iii) Synthetic fuels when the end-use fuel results from the upgrade of another fuel to improve physical and/or chemical characteristics (e.g. to increase the volumetric energy density). Figure 1 illustrates this taxonomy by representing the physical, not lexical, link between different kinds of concepts (e.g. resource, conversion and fuel).
Biofuels are those based on biomass as their chemical building blocks and energy supply for their own conversion process (Figure 1). Biomass is mainly harvested in a solid form and has a lower energy density than fossil hydrocarbons. Thus, the “bio” processes aim at concentrating the energy and converting it into a fuel more convenient to use (e.g. pellet from woody wastes). Similarly to the Office of Energy Efficiency and Renewable Energy (Office of Energy Efficiency and Renewable Energy, 2021), in the literature, biofuels mainly target liquid or gaseous transport fuels (e.g. biodiesel and bioethanol) and imply a sustainable production (Pöttering and Necas, 2009; European Commission, 2019c). The definition given by official institutions (e.g. the FAO or the European Commission) is aligned with the ISO 16559:2014: “biofuel is a solid, liquid or gaseous fuel produced directly or indirectly from biomass” (Food and Agriculture Organization of the United Nations, 2004; International Organization for Standardization, 2014; European Commission, 2019a). To add a quantitative and measurable aspect in the terminology, we propose the following definition: biofuels are fuels produced from biomass as the major component of their energy balance. Even if our terminology lacks the subtlety brought by terms like “woodfuels,” “agrofuels,” or “municipal by-products,” that allow to highlight the source of biomass at stake, provided by the FAO (Food and Agriculture Organization of the United Nations, 2004), this terminology aims at harmonising and desambiguating the meaning of the general term “biofuel.”
Electrofuels are, according to O’Connell et al. (2020), fuels whose energy content comes from renewable electricity. On top of that, Goldmann et al. (2018) even add that they should be carbon-neutral and serve as a storage capacity of electricity. To be more comprehensive, we define electrofuels as fuels produced from electricity, as the resource used to produce this electricity may vary. This electricity is transformed into hydrogen through electrolysis. This hydrogen can be upgraded in more complex fuels when combined with other molecules (e.g. carbon mono/dioxide or nitrogen). Electrofuels group all the fuels where the absolute majority of the energy contained into the fuel comes from electricity. In this sense, it is important to point out that “electrofuels” do not especially imply “renewable fuels” (Figure 1). For instance, the electricity needed to produce H2 through an electrolyser can be generated from renewable or non-renewable sources (Bhandari et al., 2014), even if the most sustainable way to produce electrofuels remains through the electricity produced in excess from VRES.
Synthetic fuels are fuels which have been obtained through a synthesis process from another fuel. It encompasses upgrading processes, such as methane produced through the Sabatier process or longer hydrocarbons based on syngas through the Fischer-Tropsch process. We extend the definition of the European Commission and Energy Information Agency who consider synthetic fuels as “any liquid fuel obtained from coal, natural gas or biomass” (EIA, 2006; European Commission, 2018). By extending this definition and focusing on the synthesis operation, similarly to Speight (2020), we are able to include emerging fuels like ammonia from the Haber-Bosch process. Contrary to biofuels and electrofuels, the resources used for producing synthetic fuels are not strictly identified. For instance, electrolysis-produced H2 can be upgraded with synthesis gas from the gasification of biomass to form methanol (Mignard and Pritchard, 2008). Another example could be the production of ammonia via the synthesis of H2 from methane-reforming. Consequently, defining a fuel only as “synthetic” gives a reduced insight about the fuel, especially about its “renewability.” Therefore, we recommend to avoid using “synthetic fuel.” In the former example, one would then rather define the produced methanol as a “bio-electrofuel” given that its main sources are electricity and biomass.
Finally, besides these recommended terms in the context of the energy transition, others are mainly misleading: “alternative” or “non-conventional” fuels. These terms are defined in opposition to another kind of fuels, mostly fossil. However, alternatives of today will be conventions of tomorrow. This means that depending on the “conventional” of the context, these terms will represent different fuels. Because of this implied ambiguity, we recommend not to use these words as their interpretation is case and time sensitive.
4 Discussion
In the urging necessity for an energy transition and the persisting need for fuels, technologies to convert primary resources into end-use fuels are rapidly multiplying. In this context, we suggest comprehensive terminology and classification to characterise the fuels. Given the limited insight provided by the widely-used term “synthetic fuels,” we recommend to use more specific terms like “biofuels”, “electrofuels,” or even hybridised terms (e.g. “bio-electrofuels”). These allow to highlight the contribution of biomass and electricity in the energy balance of the fuel.
Giving an exact share of biomass (or electricity) above which a fuel can be defined as biofuel (or electrofuel) would open an endless debate. Therefore, to emphasise the effort to step away from fossil fuels, we suggest to specify the contribution of biomass (or electricity) within the energy balance of the production of the fuel looking at both the feedstock and the energy to supply the conversion or production process. This would give, for instance, “bio(25) methanol” if 25% of the energy balance to produce the methanol comes from biomass (e.g. biomass gasification). Another example could be “electro(40) ammonia” if 40% of the energy balance comes from electricity (e.g. water electrolysis, air-captured nitrogen and power supply of the Haber-Bosch process). As electricity can be produced from a wide variety of sources (Figure 1), we recommend to give insights on the composition of this electricity (e.g. from wind, solar or the grid mix).
To fully embrace these new terminology and classification, industries and academics will have to switch of perspective as studies on energy balance of biofuels or electrofuels too often consider the energy distribution over the different production steps or the feedstock only rather than over all the resources at stake (Slade and Bauen, 2013; O’Connell et al., 2019).
Author Contributions
The literature review and the majority of the paper writing have been done by XR. Editing the figure and part of paper writing have been done by GL. HJ and FC supervised the work.
Funding
Fonds de transition énergétique—SPF Economie—Belgique.
Conflict of Interest
The authors declare that the research was conducted in the absence of any commercial or financial relationships that could be construed as a potential conflict of interest.
Acknowledgments
The authors would finally like to acknowledge and thank the insightful feedbacks of Charles Lhuillier from Vrije Universiteit Brussel and Arnaud Rouanet, Martin Colla and Davide Tonelli from UCLouvain.
References
Acar, C., and Dincer, I. (2014). Comparative Assessment of Hydrogen Production Methods from Renewable and Non-renewable Sources. Int. J. Hydrogen Energ. 39, 1–12. doi:10.1016/j.ijhydene.2013.10.060
Ahlgren, W. L. (2012). The Dual-Fuel Strategy: An Energy Transition Plan. Proc. IEEE 100, 3001–3052. doi:10.1109/JPROC.2012.2192469
Albrecht, F. G., and Nguyen, T.-V. (2020). Prospects of Electrofuels to Defossilize Transportation in Denmark - A Techno-Economic and Ecological Analysis. Energy 192, 116511. doi:10.1016/j.energy.2019.116511
Alperson-Afil, N., and Goren-Inbar, N. (2010). The Acheulian Site of Gesher Benot Ya’aqov Volume II: Ancient Flames and Controlled Use of Fire. Berlin: Springer Science & Business Media.
Bailera, M., Lisbona, P., Romeo, L. M., and Espatolero, S. (2017). Power to Gas Projects Review: Lab, Pilot and Demo Plants for Storing Renewable Energy and Co2. Renew. Sust. Energ. Rev. 69, 292–312. doi:10.1016/j.rser.2016.11.130
Bargiacchi, E., Antonelli, M., and Desideri, U. (2019). A Comparative Assessment of Power-To-Fuel Production Pathways. Energy 183, 1253–1265. doi:10.1016/j.energy.2019.06.149
Bhandari, R., Trudewind, C. A., and Zapp, P. (2014). Life Cycle Assessment of Hydrogen Production via Electrolysis - a Review. J. Clean. Prod. 85, 151–163. doi:10.1016/j.jclepro.2013.07.048
Brouwer, A. S., van den Broek, M., Zappa, W., Turkenburg, W. C., and Faaij, A. (2016). Least-cost Options for Integrating Intermittent Renewables in Low-Carbon Power Systems. Appl. Energ. 161, 48–74. doi:10.1016/j.apenergy.2015.09.090
Brynolf, S., Taljegard, M., Grahn, M., and Hansson, J. (2018). Electrofuels for the Transport Sector: A Review of Production Costs. Renew. Sust. Energ. Rev. 81, 1887–1905. doi:10.1016/j.rser.2017.05.288
Contino, F., Moret, S., Limpens, G., and Jeanmart, H. (2020). Whole-energy System Models: The Advisors for the Energy Transition, 81. Amsterdam: Elsevier, 100872. doi:10.1016/j.pecs.2020.100872
Decker, M., Schorn, F., Samsun, R. C., Peters, R., and Stolten, D. (2019). Off-grid Power-To-Fuel Systems for a Market Launch Scenario - A Techno-Economic Assessment. Appl. Energ. 250, 1099–1109. doi:10.1016/j.apenergy.2019.05.085
Dincer, I., and Bicer, Y. (2020). Enhanced Dimensions of Integrated Energy Systems for Environment and Sustainability. Berlin: Springer, 403, 440. doi:10.1016/b978-0-12-809943-8.00007-8
Ellabban, O., Abu-Rub, H., and Blaabjerg, F. (2014). Renewable Energy Resources: Current Status, Future Prospects and Their Enabling Technology. Renew. Sust. Energ. Rev. 39, 748–764. doi:10.1016/j.rser.2014.07.113
Eu-Commission (2003). Directive 2003/30/ec of the European Parliament and of the council of 8 May 2003 on the Promotion of the Use of Biofuels or Other Renewable Fuels for Transport. London: Official Journal of the European Union, 5.
European Commission (2019b). A Sustainable Europe by 2030. Available at: https://ec.europa.eu/commission/publications/reflection-paper-towards-sustainable-europe-2030_en#:∼:text=First\%20published\%20on,deeply\%20rooted\%20in\%20European\%20policies (Accessed January 20, 2021).
European Commission (2019c). Biofuels. Available at: https://ec.europa.eu/energy/topics/renewable-energy/biofuels/overview_fr (Accessed July 1, 2020).
European Commission (2019a). Glossary: Biofuels. Available at: https://ec.europa.eu/eurostat/statistics-explained/index.php?title=Glossary:Biofuels.
European Commission (2013). Proposal for a Directive of the European Parliament and of the council on the Deployment of Alternative Fuels Infrastructure. Berlin: Springer.
European Commission (2018). Synthetic Fuels. Available at: https://ec.europa.eu/knowledge4policy/glossary/synthetic-fuels_en (Accessed July 7, 2020).
Evans, A., Strezov, V., and Evans, T. J. (2012). Assessment of Utility Energy Storage Options for Increased Renewable Energy Penetration. Renew. Sust. Energ. Rev. 16, 4141–4147. doi:10.1016/j.rser.2012.03.048
Food and Agriculture Organization of the United Nations (2004). Unified Bioenergy Terminology—UBET. London: Tech. Rep., Food and Agriculture Organization of the United Nations.
Gallo, A. B., Simões-Moreira, J. R., Costa, H. K. M., Santos, M. M., and Moutinho dos Santos, E. (2016). Energy Storage in the Energy Transition Context: A Technology Review. Renew. Sustain. Energy Rev. 65, 800–822. doi:10.1016/j.rser.2016.07.028
Goede, A. P. H. (2018). CO2 Neutral Fuels. EPJ Web Conf. 189, 00010. doi:10.1051/epjconf/201818900010
Goldmann, A., Sauter, W., Oettinger, M., Kluge, T., Schröder, U., Seume, J., et al. (2018). A Study on Electrofuels in Aviation. Energies 11, 392. doi:10.3390/en11020392
Haarlemmer, G., Boissonnet, G., Peduzzi, E., and Setier, P.-A. (2014). Investment and Production Costs of Synthetic Fuels - A Literature Survey. Energy 66, 667–676. doi:10.1016/j.energy.2014.01.093
Hall, P. J., and Bain, E. J. (2008). Energy-storage Technologies and Electricity Generation. Energy policy 36, 4352–4355. doi:10.1016/j.enpol.2008.09.037
Hänggi, S., Elbert, P., Bütler, T., Cabalzar, U., Teske, S., Bach, C., et al. (2019). A Review of Synthetic Fuels for Passenger Vehicles. Energ. Rep. 5, 555–569. doi:10.1016/j.egyr.2019.04.007
International Energy Agency (2020a). Renewables. https://www.iea.org/fuels-and-technologies/renewables (Accessed July 9, 2020).
International Energy Agency (2019). The Future of Hydrogen. https://www.iea.org/reports/the-future-of-hydrogen
International Energy Agency (2007). Tracking Industrial Energy Efficiency and CO2 Emissions. Organisation for Economic Co-operation and Development.
International Energy Agency (2020b). World Energy Outlook 2019. https://www.iea.org/reports/world-energy-outlook-2019
International Organization for Standardization (2014). ISO 16559:2014 - Solid Biofuels – Terminology, Definitions and Descriptions. Geneva, CH: Standard, International Organization for Standardization.
Larsson, M., Grönkvist, S., and Alvfors, P. (2015). Synthetic Fuels from Electricity for the Swedish Transport Sector: Comparison of Well to Wheel Energy Efficiencies and Costs. Energ. Proced. 75, 1875–1880. doi:10.1016/j.egypro.2015.07.169
Mathiesen, B. V., Lund, H., Connolly, D., Wenzel, H., Østergaard, P. A., Möller, B., et al. (2015). Smart Energy Systems for Coherent 100% Renewable Energy and Transport Solutions. Appl. Energ. 145, 139–154.
Mignard, D., and Pritchard, C. (2008). On the Use of Electrolytic Hydrogen from Variable Renewable Energies for the Enhanced Conversion of Biomass to Fuels. Chem. Eng. Res. Des. 86, 473–487. doi:10.1016/j.cherd.2007.12.008
Monaco, F., Lanzini, A., and Santarelli, M. (2018). Making Synthetic Fuels for the Road Transportation Sector via Solid Oxide Electrolysis and Catalytic Upgrade Using Recovered Carbon Dioxide and Residual Biomass. J. Clean. Prod. 170, 160–173. doi:10.1016/j.jclepro.2017.09.141
National Center for Biotechnology Information (2021). Fossil Fuels. https://www.ncbi.nlm.nih.gov/mesh/?term=fossil+fuel (Accessed April 27, 2021).
O’Connell, A., Kousoulidou, M., Lonza, L., and Weindorf, W. (2019). Considerations on GHG Emissions and Energy Balances of Promising Aviation Biofuel Pathways. Renew. Sust. Energ. Rev. 101, 504–515. doi:10.1016/j.rser.2018.11.033
O’Connell, A., Prussi, M., Padella, M., Konti, A., and Lonza, L. (2020). Advanced Alternative Fuels Technology Development Report 2020 Tech. Rep. JRC123166. Luxembourg: Publications Office of the European Union. doi:10.2760/412802
Office of Energy Efficiency and Renewable Energy (2021). Full Text Glossary. https://www.energy.gov/eere/bioenergy/full-text-glossary#biofuels (Accessed April 14, 2021).
Our World in Data (2021). Global Energy Consumption Is Still Rising. https://ourworldindata.org/energy (Accessed January 28, 2021).
Pearson, B. R. J., Eisaman, M. D., Turner, J. W. G., Edwards, P. P., Jiang, Z., Kuznetsov, V. L., et al. (2012). Energy Storage via Carbon-Neutral Fuels Made from Co2, Water, and Renewable Energy 100. Berlin: Springer.
Pöttering, H., and Necas, P. (2009). Directive 2009/30/ec of the European Parliament and of the council of 23 April 2009 Amending Directive 98/70/ec as Regards the Specification of Petrol, Diesel and Gas-Oil Introducing a Mechanism to Monitor and Reduce Greenhouse Gas Emissions and Amending council Directive 1999/32/ec as Regards the Specification of Fuel Used by Inland Waterway Vessels and Repealing Directive 93/12/ec. Off. J. Eur. Union 140, 88–112. doi:10.1017/cbo9780511610851.034
Rao, A. (2015). Sustainable Energy Conversion for Electricity and Coproducts: Principles, Technologies, and Equipment. Hoboken: John Wiley & Sons.
Ridjan, I., Mathiesen, B. V., and Connolly, D. (2016). Terminology Used for Renewable Liquid and Gaseous Fuels Based on the Conversion of Electricity: a Review. J. Clean. Prod. 112, 3709–3720. doi:10.1016/j.jclepro.2015.05.117
Rosa, R. (2017). The Role of Synthetic Fuels for a Carbon Neutral Economy. C-Journal of Carbon Research 3, 11. doi:10.3390/c3020011
Slade, R., and Bauen, A. (2013). Micro-algae Cultivation for Biofuels: Cost, Energy Balance, Environmental Impacts and Future Prospects. Biomass and bioenergy 53, 29–38. doi:10.1016/j.biombioe.2012.12.019
Speight, J. G. (2020). Synthetic Fuels Handbook: Properties, Process, and Performance. London: McGraw-Hill Education.
Stančin, H., Mikulčić, H., Wang, X., and Duić, N. (2020). A Review on Alternative Fuels in Future Energy System. Renew. Sust. Energ. Rev. 128, 109927. doi:10.1016/j.rser.2020.109927
Trieb, F., Moser, M., and Kern, J. (2018). Liquid Solar Fuel - Liquid Hydrocarbons from Solar Energy and Biomass. Energy 153, 1–11. doi:10.1016/j.energy.2018.04.027
Keywords: taxonomy, renewable fuels, biofuels, electrofuels, synthetic fuels
Citation: Rixhon X, Limpens G, Jeanmart H and Contino F (2021) Taxonomy of the Fuels in a Whole-Energy System. Front. Energy Res. 9:660073 660073. doi: 10.3389/fenrg.2021.660073
Received: 28 January 2021; Accepted: 18 May 2021;
Published: 28 June 2021.
Edited by:
Biagio Fernando Giannetti, Paulista University, BrazilReviewed by:
Lina F. Soualmia, Université de Rouen, FranceFederica Vezzani, University of Padua, Italy
Giorgio Maria Di Nunzio, University of Padua, Italy
Copyright © 2021 Rixhon, Limpens, Jeanmart and Contino. This is an open-access article distributed under the terms of the Creative Commons Attribution License (CC BY). The use, distribution or reproduction in other forums is permitted, provided the original author(s) and the copyright owner(s) are credited and that the original publication in this journal is cited, in accordance with accepted academic practice. No use, distribution or reproduction is permitted which does not comply with these terms.
*Correspondence: Xavier Rixhon, xavier.rixhon@uclouvain.be