- Department of Electronic Engineering, University of Nigeria, Nsukka (UNN), Nsukka, Nigeria
The objective of this work is to develop a power management system that will control the power flow of an integrated renewable energy system with the focus on solar energy and wind energy and dual energy storage systems (batteries are used as the primary energy storage system for short to moderate storage term, whereas hydrogen fuel cell is used as a backup and long-term energy storage). These storage systems are needed to provide high reliability and control systems are necessary for the stable and optimal operation of the whole system. An Intelligent Power Management System (IPMS) is developed to handle various changes in power supply and power demand by managing erratic power and provide suitable control algorithm for the whole system. In order to test and validate the proposed IPMS model, simulations were conducted under various power supply and power demand using power system modeled in HOMER environment. The performed simulations confirm the ability of the IPMS to satisfy the load at all times using solar and wind power (which are unsteady renewables), through the support of batteries and hydrogen fuel cell without a reduction in the power quality or load supply.
Introduction
The application of renewable energy sources for electricity generation and utilization has seen a huge potential, and a number of studies have been conducted by many researchers on various aspects of renewable energy development and utilization (Anees, 2012; Sen and Bhattacharyya, 2014; Abiola-Ogedengbe et al., 2015; Reboredo, 2015; Wesseh and Lin, 2015; Alharthi et al., 2018; Jiang et al., 2018; Mehrpooya et al., 2018; Shoeb and Shafiullah, 2018). A comprehensive literature review has also been made by many researchers on the impacts of renewable energy based power supply (Connolly et al., 2010; Hepbasli and Alsuhaibani, 2011; Parida et al., 2011; Bajpai and Dash, 2012; Bhattacharyya, 2012; Micheli et al., 2012; Chauhan and Saini, 2014; Sathish and Shivarama, 2015; Yasmeena and Das, 2015; Khoury et al., 2016; Olatomiwa et al., 2016; Owusu and Asumadu-Sarkodie, 2016; Jamal et al., 2017). Communities have moved into alternative energy studies, and many researches have been done on this area which include stand-alone renewable energy with storage system such as PV/battery (Ashhab et al., 2013) and integrated renewable energy system with storage system such as PV/wind/fuel cell (Ahmed et al., 2008; Ceran and Sroka, 2015), PV/wind/battery (Mohammadnezami et al., 2015; Al Badwawi et al., 2015; Das et al., 2016; Aziz et al., 2018) and PV/wind/battery/fuel cell (Dursun and Kilic, 2012). Many studies also have been done on hybrid system with battery bank and fuel-cell such as PV/Diesel/Battery (Madziga et al., 2018), PV/Diesel/Fuel Cell (Vivas et al., 2018), and PV/Wind/grid/battery (Alharthi et al., 2018). The major limitation of all these energy systems mentioned is the control requirement for optimal efficiency. In order to maintain the energy sustainability of different energy sources, many Power Management Systems (PMS) are proposed in literature (Behzadi and Niasati, 2015; Mhusa et al., 2015; Onur and Ismail, 2015; Albert Martin et al., 2015; Ranjit, 2016; Ceran et al., 2017; Sassi et al., 2017; Moghaddam et al., 2019). In Ceran et al. (2017), the authors developed computer simulation model to optimize integrated power generation system which utilizes photovoltaic modules (PV), wind turbines (WT), PEM fuel cells (FC), electrolyzer (EL) and hydrogen tank (HT) as the energy storage. The analysis of the integrated PV/WT/FC system was carried out for three different residential loads, local solar radiation and local wind speed, based on the real measurement values. The analysis shows the possibility of interbreeding renewable energy sources and the results confirm the effectiveness of the proposed approach, which could be assumed as a very useful tool in the design and analysis of an integrated power generation system. Ranjit (2016) proposed a complete hardware system development, implementation and construction of real-time direct current (DC) integrated renewable energy system (HRES) for solar-wind-battery energy source hybridized with grid network support for optimal system controllability and operation. The real-time DC HRES’s experimental results demonstrated the system’s ability to perform supervision, coordination, management and control of all the available energy sources with least dependency on the power source supply from the grid network. The obtained results demonstrated the optimal technical solution for hardware development, implementation, integration and construction. The proposed PMS in (Albert Martin et al., 2015) incorporates fuzzy logic control for a microgrid system which was fed with the PV-Wind integrated power generation system. The designed PMS was implemented and it achieves the optimization, and control of the distributed energy generation and battery management. Mhusa et al. (2015) proposed a control strategy for power management in solar/wind integrated power system based on Artificial intelligence techniques. Simulation results indicate that the proposed control system provides uninterrupted power, effective utilization of sources, minimizing usage of battery and can be concluded that the proposed power management system can satisfactory manage energy supply in a PV-Wind integrated power system. Moghaddam et al. (2019) designed an integrated PV/wind/battery system for improving the load supply reliability considering the Net Present Cost (NPC) as the objective function to minimize. They considered the deficit power-hourly interruption probability of the load demand, i.e. the reliability index and proposed for an improved crow search algorithm (ICSA). The proposed ICSA is used to solve the optimization problem for Zanjan city, Iran (minimizing the system power generation cost and improving the reliability of the load). Onur and Ismail (2015) proposed an intelligent power management system (IPMS) with fuzzy logic decision maker for maintaining the energy sustainability in renewable energy systems (RES) consisting of wind and photovoltaic (PV) solar panels. The proposed fuzzy reasoning based IPMS determines the amount of power to be supplied from each or both sources and tracks the maximum power operating point of the wind energy system. Sassi et al. (2017) developed a Power Management Supervisor (PMS) of a Hybrid Renewable Energy System (HRES) consisting of a Photovoltaic (PV), a wind turbine and a batteries bank, and can operate in grid connected or standalone mode for a smart-home. The developed multi-objective PMS satisfy the loads energy demand by determining the different subsystems’ states according to conditions use and utility grid availability; ensure the energy flux management and optimize the batteries utilization. Behzadi and Niasati (2015) proposed a PV/battery/FC based integrated system. Three energy management strategies were used in the research work. Firstly, the amount of excessive energy is measured, if the amount is positive then the excess amount is flowed into the battery storages till its state of charge is full. On the contrary, the fuel-cell will be connected to the load and will remain till the hydrogen’s pressure is above the critical value, otherwise load is connected to the battery storage. The second strategy the hydrogen pressure level is checked regardless the minimum battery storage state of charge. The last strategy measures the minimum power of produced by each component, once this information is gathered then the amount of power flow to each storage device is decided. In this paper, a Power Management System (PMS) is studied for an integrated PV/Wind/Battery/Fuel Cell System.
Research and Development of Power Management System for HRES
Innovations in science and technology have helped energy sectors to expand its Research and Development (R&D). Research in its widest sense aims at extending knowledge in a particular field, having regard to the general, long-term value of such knowledge for a known need in industry. An innovative integrated energy system combining solar photovoltaic panels, wind turbine, battery storage and fuel cell requires an intelligent controller to manage the power flow and sustain the system. Principle of synergy states that merging different resources such as Solar PV-Wind-Battery-Fuel Cell to form an integrated system, will lead to higher work efficiency. However, in order for all these subsystems to work efficient and satisfies load demand taking into consideration the varying nature of renewable energy sources and changes in demand, the power management system is the solution for such integrated system. Therefore, a power management system with the certain algorithm (control strategies) should be established in order to manage the power flow between those subsystems. A power management control strategy for an integrated PV/wind/battery/fuel-cell standalone system is discussed by Dursun and Kilic (2012). The study is conducted and the operation of three established energy management strategies for an integrated PV/wind/battery/fuel-cell standalone system reviewed. PV and wind renewable energy sources were designated as primary power source supply, whereas fuel-cell component is installed as a backup energy source. The energy management strategy adopted in the research focuses on the energy produced by the PV and wind renewable energy sources and state of charge of the battery storage. Three strategies were applied to manage the power from the PV and wind renewable energy sources. They are explained by the authors as in the following:
• Strategy 1—When the battery state of charge is between the positive limits, and the renewable energy sources (PV and wind) produce excessive energy, then the battery storage discharges and the excessive energy is directed to power the electrolyser. On the contrary, when the battery storage is under its minimum state of charge and no excess energy is available from renewable energy sources (PV and wind) then the fuel-cell supplies the energy to the load and at the same time charges the battery storage.
• Strategy 2—When the battery state of charge is between a positive limit and additional energy is unavailable from the renewable energy sources (PV and wind), then the fuel-cell will not operate. During this condition, the battery storage will be discharged. However, if the battery storage state of charge is below the limit then fuel-cell will charge the battery storage.
• Strategy 3—When the battery state of charge is between a positive limit, and the renewable energy sources (PV and wind) produce surplus energy, then the electrolyser will operate and battery storage will be charged. On the contrary, when the battery storage state of charge is below the limits or excess energy from the renewable energy sources (PV and wind) are unavailable, then fuel-cell component will operate and battery storage will be discharged.
In this paper, the management strategy that describes the proposed intelligent power management algorithm of the integrated PV/wind/battery/fuel cell system which will be use to manage and strategies the energy delivery efficiently is thus: when the power generated from renewable energy system exceeds the power required for the load demand, the surplus power will be used to charge the batteries until it reaches its maximum level and the extra power will be reserved in the form of compressed hydrogen via conversion through the electrolyzer. On the contrary, when the power required for the load demand exceeds the power generated from renewable energy system, the battery will be used to ensure the load demand is met until decreased to its minimum level. However, if still, there is a deficit power, the fuel-cell will be used to compensate the deficit load demand and at the same time charges the battery storage. The aim of this study is to provide an algorithm for an integrated PV/wind/battery/fuel-cell system that will be used to develop its power management system model with the decision strategy (for greater flexibility and efficiency) and modes of control for the system operation.
Control Algorithm for Integrated PV/Wind/Battery/Fuel Cell System
Control strategies have been acknowledged as an efficient way to enhance process profitability. Therefore, reduction in total cost of ownership is the major benefit of combining control modeling and simulation into the energy development process (Ani, 2015). Control strategy is a critical requirement in the development of a power management system for Solar PV-Wind-Battery-Fuel Cell Integrated System. This research focuses on the development of an intelligent power management system for Solar PV-Wind-Battery-Fuel Cell Integrated System.
In integrated systems with batteries and without fuel cell, the dispatch strategy is straightforward: the battery charges only if the renewable energy generated is in excess after meeting the load demand, and the battery discharges if the load demand exceeds the renewable energy (Hsu and Kang, 2014; Ani, 2015). Nevertheless, the control strategies of an integrated system can become complicated if the system includes a fuel cell and batteries (Bernal-Agustín and Dufo-López, 2009). In this situation, it is important to decide how the batteries are charged and what component (batteries or fuel cell) have priority to supply energy when the load demand exceeds the energy generated from renewable energy sources.
Integrated System Operation
If the values of irradiation on an angled planes, speed of the wind and the consumption design is given, the system operation can be simulated using an hourly time step. In accordance with the system energy balance and on the storage continuity equation, the simulation method used here is related to that used by others (Sidrach de Cardona and Mora Lopez, 1992; Kaye, 1994; Ani, 2015). Taking into consideration the battery charger output power Pcharger(t), the PV output power Pp(t), the Wind output power Pw(t) and the load power PL(t) on the simulation step ∆t, the battery energy benefit during a charge time ∆t1 is given by (∆t1< ∆t) (Ani, 2015):
The battery energy loss during a discharge time ∆t2 is given by (∆t2 < ∆t) (Ani, 2014a):
The state of charge of the battery is defined during the simulation time-step ∆t (Ani, 2015) by:
If
Furthermore, if during the discharge period when the fuel cell is stopped,
As an input of a simulation time-step ∆t (taken as 1 h), a number of variables such as PV output power, Wind output power, load power, battery state of charge, and back-up fuel cell state (ON or OFF) were determined. A battery energy balance indicates the operating strategy of the PV/Wind/Battery/Fuel Cell integrated system: charge (energy balance positive) or discharge (energy balance negative). Presuming that the State of Charge (SOC) falls below SDM, the fuel cell is started; and given that the SOC exceeds SAR, the fuel cell is stopped. Thus, the charge and discharge times (Eqs 4, 5) should be calculated on the simulation time-step so as to compute the various energy flows in the system (Eqs 1, 2). Parameters for fuel cell operation and battery operation such as minimum SOC and maximum SOC are usually set on fix value. In this work, these parameters are programmable (changeable).
In a storage system involving fuel cell, the charging mode is in control of charging the batteries and/or of generating hydrogen fuel from the excess power (Althubaiti et al., 2017). This excess power is assigned to the battery and when the battery is fully charged, the remaining power goes to the water electrolysis. The discharging mode discharges the batteries and/or generates power from a hydrogen fuel cell to assist the load.
The power that goes to the energy storage system is given by (Althubaiti, 2018):
Where
Description of System Components
According to the described algorithm, the integrated power system is consist of photovoltaic cell, wind turbine, battery and fuel cell back-up. Concept of integrated power system operation is to supply energy from photovoltaic cell and wind turbine for load demand and battery charging (Panajotovic, 2010) (if it is possible). If renewable energy sources (sun and wind) is not sufficient for load demand, difference of energy is provide from battery, or from fuel cell back-up if battery is discharged. The components that made up the integrated system are described as:
Photovoltaic Systems
The solar PV technology uses the electrical properties of certain materials to change solar energy into utilizable electricity. They are made up of photovoltaic cells, usually a substance with electrical conductivity that generates current when sunlight strikes them. Multiple cells can be build into modules that can be wired in an array of any size (Poullikkas, 2010).
Wind Turbines
A wind turbine is a machine that changes the kinetic energy of the wind into mechanical energy via the induced rotation of aerofoil-shaped rotors (Samuel, 2014), and then converts the mechanical energy into electricity for consumption. The modern wind turbine is a highly developed machinery with aerodynamically designed rotor and efficient power generation, transmission and regulation components (Paul, 2004).
Battery
A battery is a storage device that stores direct current (DC) electrical energy in electrochemical configuration for later use. The capacity of energy that will be stored or delivered from the battery is controlled by the battery controller or the inverter (Qonain et al., 2018). The inverter changes the DC electrical energy to alternative current (AC) electrical energy, which is the energy required to operate AC appliances. Although battery is used for storing when excessive energy is available and discharging when energy is required, it can also be used to formulate a strategy called “state of charge setpoint strategy.” This setpoint strategy is adopted in this current study and were used to control the start-up and stop of the fuel cell; where setpoints for the minimum state of charge (SOC_min) is 40% (when the fuel cell starts up) and the maximum state of charge (SOC_max) is 80% (when the fuel cell stops).
Fuel Cell
A fuel cell (FC) is a device that converts the chemical energy of a fuel (usually hydrogen derived from natural gas or biogas) and an oxidant (air or oxygen) into electricity without combustion (Charles, 2010). Excess energy is reserved in the form of compressed hydrogen via conversion through the electrolyzer (EL) (Chedid, 2007; Yogesh and Sendil, 2020). The FC is used to produce electricity if the load exceeds the electricity production from the integrated renewable system. It can also function as an emergency generator (Fuel Cell Today, 2020) on condition that the integrated renewable system fails. Fuel cells are driven by electrochemistry, not combustion. If the integrated renewable system generates more energy than needed by the load, then the excess energy is diverted towards an electrolyzer. The electrolyzer-produced hydrogen is stored in a tank for later use in the fuel cell stack. When there is a deficit of energy in the battery, hydrogen is used in the fuel cell and demand is satisfied (Ghosh et al., 2003).
Principle of Integrated Renewable Power System Operation
The integrated renewable system model to be described is the fundamental of the simulation. Aside from precise optimization, the quality and accuracy of the model and its application in the algorithm, greatly determines the usefulness of the simulation results. Figure 1 shows the integrated renewable system structure. Barley Dennis and Winn Byron (1996), has set guiding principles for control strategies. They advanced the control strategies model of Barley et al., initiating new parameters that have become of great significance in the control strategies of the Hybrid Optimization of Multiple Energy Resources (HOMER) software tool (Barley et al., 1995). The authors presented four control strategies, which are: Frugal dispatch strategy, Operation strategy, Load following strategy, and State of Charge (SOC) Set-point strategy (Ani, 2015). Out of these, the SOC_Setpoint strategy is adopted for this study. In SOC_Setpoint strategy, the fuel cell is ON, ready to supply to the load and charge the batteries until the SOC_Setpoint is reached. We therefore propose an optimization method of the control strategies based on Barley Dennis and Winn Byron (1996) of a (PV/Wind/Battery/Fuel Cell) integrated system with AC load, using Setpoints for the start-up and stop of the fuel cell and for the charging of batteries. The fuel cell starts up when the renewable energies and the battery cannot meet the load or if the battery state of charge has fallen below a specified value. The fuel cell stops when there is abundant power available from the renewable energies and the battery to supply the load demand or if the battery has attained an acceptable state of charge.
An Algorithm for Integrated PV/Wind/Battery/Fuel Cell System Sizing Simulation
The algorithm for the integrated system of renewable and fuel cell energy used in the simulation is presented in Figure 2. Inputs of the algorithm are the technical data of all the components of the energy system. These data consist of the climatic variables, the load demand and the constraints on the operation of the energy system (Ani, 2013).
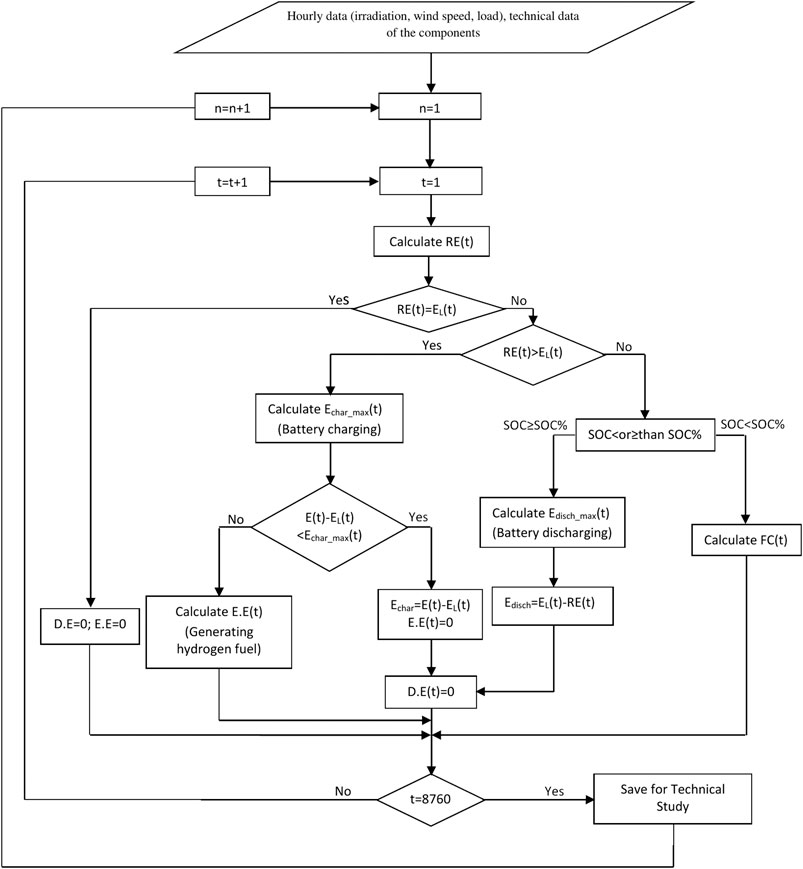
FIGURE 2. Algorithm for integrated PV/wind/battery/fuel cell system (Ahmed et al., 2010; Ani, 2015).
For each combination n, the total power, RE(t) (Renewable Energies: PV and Wind) and FC(t) (Fuel Cell Energy), generated by the PV cells and wind turbine, and Fuel Cell at hour t is calculated using (Lal Kumar et al., 2011):
Where
During system operation of the integrated PV/Wind/Battery/Fuel Cell system, different situations may appear:
The load demand (EL(t)) can be less than the total energy generated by the PV cells and wind turbine. In this case, the excess energy is stored in the batteries (Echar) after calculation, as a precursory, the maximum amount of energy that can be charged (Echar_max(t)) in the battery bank. The surplus of energy (E.E(t)), if there exist, goes to the water electrolysis which then generates hydrogen fuel.
The total energy generated by the PV cells and wind turbine can be less than the demand of the load. In this situation, the load must be covered by the energy stored in batteries (Edisch) after calculating, as a preliminary, the maximum amount of energy that can be discharged (Edisch_max(t)) from the battery bank (Ahmed et al., 2010). The shortage of energy (D.E(t)) is zero.
The load demand can be equal to the total energy generated by the PV cells and wind turbine, then the battery capacity remains constant.
The demand of the load can be greater than both the total energy generated by renewable energy (PV and Wind) and the energy stored in the batteries (Edisch). In this situation, the load should be covered by the fuel cell.
Finally, a technical study will be done.
Intelligent Power Management System (IPMS)
An operational control strategy is made up of certain preset control settings that are set when installing the system. Such settings relate to the setpoint of when to switch ON the fuel cell or not, based on certain values describing the system state, such as the battery state of charge and the demand placed on the system. For the purpose of this study, we developed an Intelligent Power Management System (IPMS) for an Integrated PV/Wind/Battery/Fuel Cell System. This power management system collects the energy generation data from different components within the integrated renewable system and identifies the load to be powered. The time-independent IPMS setting for Solar PV-Wind-Battery-Fuel Cell Integrated Renewable System is shown in Figure 3, and its modes of control for the system operation is shown in Figure 4. The intelligent power management system uses a sliding control for the system operation of the integrated renewable system; seeing the PV energy generation as the primary source of energy, wind energy generation as the secondary source, the battery as the supplement and the fuel cell as the back-up source of energy. Charging mode is activated when there is surplus power from the renewable energy. The decision of distributing the surplus power is achieved and implemented by the IPMS. This surplus power is assigned by the IPMS to the battery controller and when the battery is fully charged, the remaining power goes to the water electrolysis through the hydrogen controller. The discharging mode is activated when power is required to satisfy the load. In this case, the decision of distributing the required power is done by the IPMS. The required power is requested from the battery through the battery controller that decides whether the battery can support the load or not. If the battery cannot support the load, then the fuel cell will be assigned to supply the load and charge the battery through the hydrogen controller. The fuel cell is set to supply only 5 kW whenever it is called to supply. The system moves between different modes dependant on the power needed by the load and the power able to be supplied by each of the sources. Figure 4 indicates the flow between the different modes. At first, the power supplied by the PV panels and the wind turbine is computed for each hour over the year and stored in matrices, so that power availability in each hour can be retrieved easily (Ani, 2014b).
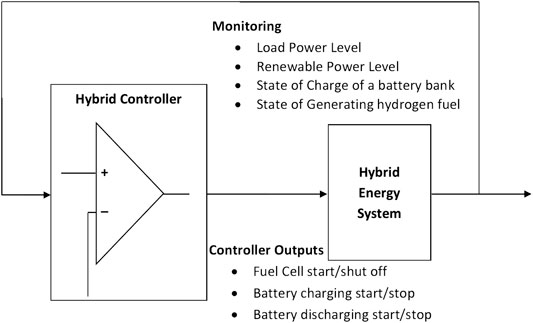
FIGURE 3. Block Diagram of intelligent power management system for solar PV-wind-battery-fuel cell integrated system (Pipattanasomporn, 2004; Ani, 2015).
Decision Strategy and Modes of Control for the System Operation
Mode 1
When the system is in mode 1, it uses entirely the energy generated by the PV panels to satisfy the load demand. As the wind turbines are connected to the system, despite that, it is not used to satisfy the load in this mode. Rather, the energy generated by the wind turbine in addition to any surplus energy from the PV panels will be used to charge the batteries and/or for generating hydrogen fuel.
Mode 2
The system enters mode 2 when the power generated by the PV panel is insufficient to satisfy the load. In this mode, if the energy available from the PV panels and the wind turbine combined is in surplus of what is needed by the load, then the sufficient power available from the PV panel is used to satisfy the load and the power from the wind turbine is supplied using sliding control to match the power needed by the load. The surplus energy from the wind turbine can be used to charge the batteries and/or for generating hydrogen fuel, as in mode 1.
Decision Mode
There is however a possibility, a time that the amount of power required by the load is not able to be supplied by mode 2, then the program determines what element (batteries or fuel cell) have priority to supply energy.
The program determines what element has priority to supply energy depend on (Ani, 2014a):
• If the state of charge of the battery is greater than the minimum amount (40%) and thus the battery is able to supply power to the load, in that case the battery will be used.
• If the combined power of the PV panels and wind turbine is not sufficient to supply the load and the battery is at its minimum state of charge and so cannot be used to satisfy the shortage of power required, in that case the fuel cell will be used to meet the load and charge the battery (The fuel cell is set to supply only 5 kW whenever it is called to supply the load and charge the battery).
From the results of the control simulation (shown in Tables 1–3), we were able to see the performance of the system - the power supplied by each of the energy sources, and the power required by the load.
Simulation
In order to test the developed control model, a typical household (relatively large single family dwelling) in Nigeria was chosen and the electrical requirements analysed. The peak demand for the chosen household is 4.667 kW, while the intermediate load is between 3.299 kW and 4.467 kW as shown in Figure 5. This particular household is located in Abaji (Abuja, FCT). Its specific geographical location is 9° 00ʹ N latitude and 7° 00ʹ E longitude with annual average solar daily radiation of 5.45 kWh/m2/d and annual average wind of 2.4 m/s, which were obtained from the National Aeronautics and Space Administration (NASA) Surface Meteorology and Solar Energy web site (National Aeronautics and Space Administration (NASA), 2010). The integrated renewable system consists of 10 kW PV solar power, 10 kW wind power, 5 kWh battery bank, 10 kW Water Electrolysis, 10 kW fuel cells, and 30 kg hydrogen tank. Simulation was conducted on the control model with the real data of solar, wind and house demand powers and see how the IPMS manage the power flow between various subsystems, power supply charging and power demand discharging. For more feasible results and comprehensive analysis of the proposed technique, these data (solar power, wind power and load demand) were applied to a power system modeled in HOMER environment.
Results and Discussions
The designed software results were carried out followed with HOMER data to prove the accuracy of the analysis. Comparatively, results obtained from designed software module and that gotten from HOMER setup shows a close agreement between them. The results of the demand met by the integrated renewable energy system (PV/Wind/Battery/Fuel Cell) for three consecutive days are shown in Tables 1–3 and Figures 6–11. The renewable energy generated as seen in Tables 1–3 were calculated (addition of PV and Wind outputs) from the renewable energy resources (see the Supplementary Appendix).
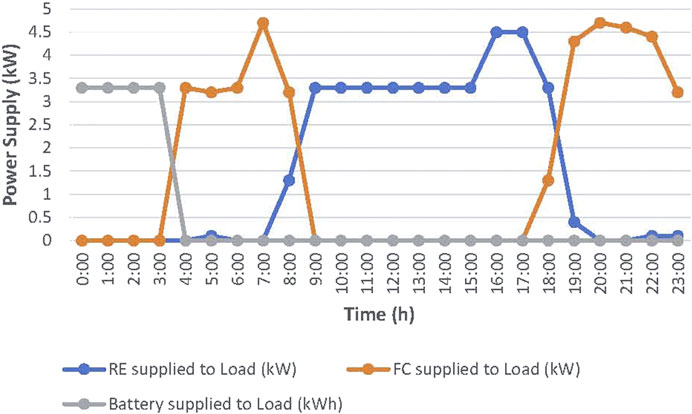
FIGURE 6. Power Supplied by the Renewable Energy, Fuel Cell, and Battery to satisfy the Load Demand in Day 1
Discussions
Tables 1–3 and Figures 6–11 above show how the demand is met by the renewable energy system (PV and wind), and also how the sources were allocated according to the load demand and availability. It was observed that the variations are both in the demand and the availability of sources. The battery or the fuel cell compensates the deficit depending on the decision mode.
The simulation results above show how the proposed IPMS assigned the battery to supply the load (as in Figure 6: 0:00 h–3:00 h; Figure 10: 20:00 h–23:00 h) due to unavailability of the renewable energy sources. The renewable energy is prioritized to supply the load, and this can be seen from the Figures where the renewable energy system is at the least level (Figure 6: 5:00 h; 22:00 h, and 23:00 h; Figure 8: 1:00 h–3:00 h; 19:00 h–20:00 h; Figure 10: 6:00 h–7:00 h), and the IPMS assigned the fuel cell to assist the renewable energy to satisfy the load. The fuel cell is used by the IPMS to assist the increasing load demand supply (4.467 and 4.667 kW) as the renewable energy sources are unavailable (as in Figure 6: 7:00 h, and 20:00 h; Figure 8: 7:00 h, 21:00 h, and 22:00 h). The storage system operate as an assistive to the renewable energy (RE) as the RE has shortage of power (Figure 10: 18:00 h–19:00 h). In this case, the renewable energy system and battery storage system were assigned to power the load.
The simulation results also show how the IPMS utilizes the battery bank effectively. It was observed that whenever surplus power is available from the renewable sources, the IPMS switches the batteries into charging mode (as in Figure 7: 10:00 h–17:00 h; Figure 9: 11:00 h–14:00 h; Figure 11: 9:00 h–17:00 h) while the energy from the fuel cell (as in Figure 7: 4:00 h–23:00 h; Figure 9: 0:00 h–23:00 h; Figure 11: 0:00 h–9:00 h) charges the battery till its state of charge is full. For instance in Table 3 above, at 9:00 h when the battery state of charge is 80.30%, the IPMS turns off the fuel cell and allocates renewable energy (PV and wind) to supply the load demand as well as charging the battery. This is the confirmation of what was mentioned in the Figure 4, that the IPMS turns off the fuel cell when the load demand can be met together by the PV, wind, and battery bank.
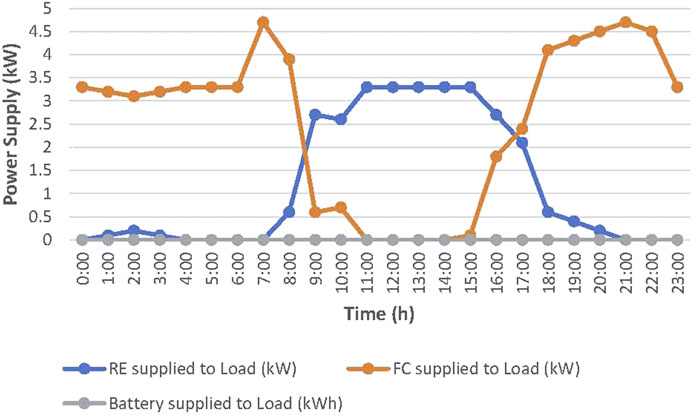
FIGURE 8. Power Supplied by the Renewable Energy, Fuel Cell, and Battery to satisfy the Load Demand in Day 2
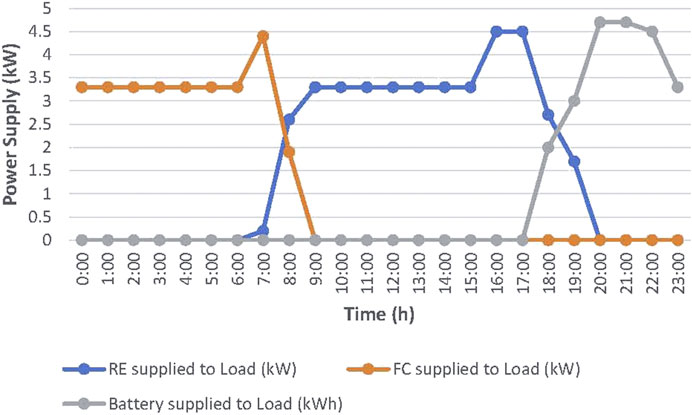
FIGURE 10. Power Supplied by the Renewable Energy, Fuel Cell, and Battery to satisfy the Load Demand in Day 3
In summary, the proposed IPMS is developed to ensure the continuity of power source supply to the load and controls the operations of the renewable energy sources (PV and Wind) and energy storage systems (Batteries and Fuel Cell). The renewable energy is prioritized to supply the load, and the excess energy (if any) is flowed into the battery storages. The storage system operate as an assistive to the renewable energy (RE) when the RE has shortage of power. The Fuel cell is used to assist the increasing load demand supply and concurrently charge the battery storage when the renewable energy sources are unavailable and the battery storage have reached its minimum state of charge. Fuel cell is also used to assist the renewable energy to supply the load when the renewable energy system and battery state of charge is at the least level. The results proofs that the energy management system for an integrated PV/Wind/battery/fuel cell system is competence to supervise, manage and control the integrated renewable energy system.
Conclusion
An Intelligent Power Management System (IPMS) was developed to ensure the continuity of power source supply to the load and controls the operations of the renewable energy sources (PV and Wind) and energy storage systems (Batteries and Fuel Cell). The proposed strategy solves the problem related to power needs by satisfactorily supplying the load demand while the power flow is adequately controlled. Surplus power is directed to the storage system and the IPMS provides the stability of the complete system. Simultaneously, it effectively controls the charging and discharging processes of the battery to extend its performance and longevity. Furthermore, at any moment there is need to satisfy the load demand and the renewable energy system and battery state of charge is at the least level (or when the renewable energy sources are unavailable and the battery storage have reached its minimum state of charge), the IPMS smoothly supply the demand (load) from the fuel cell to avoid system failures. Each one of the categorized modes (Mode 1, Mode 2, and Decision Mode) were tested under multiple operating scenarios, and the obtained results confirmed its capability to control integrated (PV/Wind/Battery/Fuel Cell) system.
Data Availability Statement
The original contributions presented in the study are included in the article/Supplementary Material, further inquiries can be directed to the corresponding author.
Author Contributions
The author confirms being the sole contributor of this work and has approved it for publication.
Conflict of Interest
The author declares that the research was conducted in the absence of any commercial or financial relationships that could be construed as a potential conflict of interest.
Acknowledgments
The author of this paper is very grateful to Grace Ifunanya Anayochukwu for her encouragement, support, caring, and pre-editing the article prior to its submission and publication. The author is also grateful to the Editor and Reviewers for their useful contributions.
Supplementary Material
The Supplementary Material for this article can be found online at: https://www.frontiersin.org/articles/10.3389/fenrg.2021.613958/full#supplementary-material.
References
Abiola-Ogedengbe, A., Hangan, H., and Siddiqui, K., (2015). Experimental investigation of wind effects on a standalone photovoltaic (PV) module. Renew. Energ. 78, 657–665. doi:10.1016/j.renene.2015.01.037
Ahmed, N. A., Miyatake, M., and Al-Othman, A., (2008). Power fluctuations suppression of stand-alone hybrid generation combining solar photovoltaic/wind turbine and fuel cell systems. Energy Conver. Manag. 49, 2711–2719. doi:10.1016/j.enconman.2008.04.005
Ahmed, S., Othman, H., and Anis, S. (2010). Optimal sizing of a hybrid system of renewable energy for a reliable load supply without interruption. Eur. J. Scientific Res. 45 (4), 620–629.
Al Badwawi, R., Abusara, M., and Mallick, T. (2015). A review of hybrid solar PV and wind energy system, Smart Sci. 3 (3), 127–138. doi:10.1080/23080477.2015.11665647
Albert Martin, R. A., Mathew, R. G., and Rajeswari, N. (2015). Implementation of energy management system to PV-wind hybrid power generation system for DC microgrid applications. Int. Res. J. Eng. Technol. (Irjet) 2 (08), 204–210.
Alharthi, Y., Siddiki, M., and Chaudhry, G. (2018). Resource assessment and techno-economic analysis of a grid-connected solar PV-wind hybrid system for different locations in Saudi Arabia. Sustainability 10, 3690. doi:10.3390/su10103690
Althubaiti, M., Bernard, M., and Musilek, P. (2017). "Fuzzy logic controller for hybrid renewable energy system with multiple types of storage," in 2017 IEEE 30th Canadian conference on electrical and computer Engineering (CCECE), 30 April–3 May 2017, Windsor, ON (Piscataway: IEEE), 1–6. doi:10.1109/CCECE.2017.7946738
Althubaiti, M. (2018). Fuzzy logic controller for hybrid renewable energy system with multiple types of storage. MS Dissertation. Edmonton, (AB): Department of Electrical and Computer Engineering, University of Alberta
Anees, A. S. (2012). “Grid integration of renewable energy sources: challenges, issues and possible solutions.” in 2012 IEEE 5th India International Conference on Power Electronics (IICPE), 6–8 Dec. 2012, Delhi, India, (Piscataway: IEEE), p. 1–6. doi:10.1109/IICPE.2012.6450514
Ani, V. A. (2013). Optimal energy system for single household in Nigeria. Int. J. Energ. Optimization Eng. (Ijeoe) Publ. IGI Glob. 2, 3. doi:10.4018/ijeoe.2013070102
Ani, V. A. (2014a). Simulation and optimization of photovoltaic/diesel hybrid system for off-grid banking industry. Int. J. Energ. Optimization Eng. (Ijeoe) Publ. IGI Glob. doi:10.4018/ijeoe.2014040102
Ani, V. A., (2014b). Optimal operational strategy for PV/Wind-Diesel hybrid power generation system with energy storage. Int. J. Energ. Optimization Eng. (Ijeoe) Publ. IGI Glob. 3. doi:10.4018/ijeoe.2014010107
Ani, V. A. (2015). “Energy optimization at GSM base station sites located in rural areas.” Ph.D. Dissertation. Nsukka (UNN): Department of Electronic Engineering, University of Nigeria.
Ashhab, M. S. S., Kaylanib, H., and Abdallah, A. (2013). PV solar system feasibility study. Energ. Convers. Management 65, 777–782. doi:10.1016/j.enconman.2012.02.030
Aziz, A. S., Tajuddin, M. F. N., and Adzman, M. R. (2018). Feasibility analysis of PV/wind/battery hybrid power generation: a case study. Int. J. Renew. Energ. Res. 8, 661–671.
Bajpai, P., and Dash, V. (2012). Hybrid renewable energy systems for power generation in stand-alone applications: a review. Renew. Sustain. Energ. Rev. 16 (5), 2926–2939. doi:10.1016/j.rser.2012.02.009
Barley Dennis, C., and Winn Byron, C. (1996). Optimal dispatch strategy in remote hybrid power systems. Solar Energy 58 (4), 165–179.
Barley, C. D., Winn, C. B., Flowers, L., and Green, H. J. (1995). Optimal control of remote hybrid power systems”. Part 1: Simplified model. Golden, CO, United States: National Renewable Energy Lab.
Behzadi, M. S., and Niasati, M. (2015). Comparative performance analysis of a hybrid PV/FC/battery stand-alone system using different power management strategies and sizing Approaches. Int. J. Hydrogen Energ. 40 (1), 538–548. doi:10.1016/j.ijhydene.2014.10.097
Bernal-Agustín, J. L., and Dufo-López, R. (2009). Simulation and optimization of stand-alone hybrid renewable energy systems. Renew. Sustainable Energ. Rev. 13 (8), 2111–2118. doi:10.1016/j.rser.2009.01.010
Bhattacharyya, S. C. (2012). Energy for Sustainable Development: a critical review and analysis. Energy Sustai. Dev. 16 (3), 260–271. doi:10.1016/j.esd.2012.05.002
Ceran, B., and Sroka, K. (2015). Performance analysis of a hybrid generation system of wind turbines, photovoltaic modules, and a fuel cell. Acta. Energetica. 2 (23), 36–42. doi:10.12736/issn.2300-3022.2015204
Ceran, B., Hassan, Q., Jaszczur, M., and Srokal, K. (2017). An analysis of hybrid power generation systems for a residential load. Energy and Fuels; E3S Web of Conferences 14, 0102. doi:10.1051/e3sconf/20171401020
Charles, K. (2010). Fuel cell technology: a clean, reliable source of Stationary power clean energy states alliance (CESA). Available at: www.cleanenergystates.org/JointProjects/hydrogen.html (Accessed October 3, 2020)
Chauhan, A., and Saini, R. P. (2014). A review on integrated renewable energy system based power generation for stand-alone applications: configurations, storage options, sizing methodologies and control. Renew. Sustain. Energ. Rev. 38, 99–120. doi:10.1016/j.rser.2014.05.079
Chedid, R., Chaaban, F. B., and Shihab, R., (2007). "A Simplified electric circuit model for the analysis of hybrid wind-fuel cell systems." 2007 IEEE Power Engineering Society General Meeting, 24–28 June, Tampa, FL, (Piscataway: IEEE)
Connolly, D., Lund, H., Mathiesen, B. V., and Leahy, M. (2010). A review of computer tools for analysing the integration of renewable energy into various energy systems. Appl. Energ. 87, 1059–1082. doi:10.1016/j.apenergy.2009.09.026
Das, H. S., Dey, A., Tan, C. W., and Yatim, A. H. M. (2016). Feasibility analysis of standalone PV/Wind/Battery hybrid energy system for rural Bangladesh. Int. J. Renew. Energ. Res. (Ijrer) 6 (2), 402–412.
Dursun, E., and Kilic, O. (2012). Comparative evaluation of different power management strategies of a stand-alone PV/wind/PEMFC hybrid power system,” Int. J. Electr. Power Energ. Syst. 34 (1), 81–89. doi:10.1016/j.ijepes.2011.08.025
Fuel Cell Today (2020). Opening doors to fuel cell commercialization, the Technology, Education kit 2. Available at: www.fuelcelltoday.com (Accessed September 09, 2020).
Ghosh, P. C., Emonts, B., and Stolten, D. (2003). Comparison of hydrogen storage with diesel-generator system in a PV–WEC hybrid system. Solar Energy 75 (3), 187–198. doi:10.1016/j.solener.2003.08.004
Hepbasli, A., and Alsuhaibani, Z. (2011). A key review on present status and future directions of solar energy studies and applications in Saudi Arabia. Renew. Sustain. Energ. Rev. 15, (9), 5021–5050. doi:10.1016/j.rser.2011.07.052
Hsu, D., and Kang, L. (2014). Dispatch analysis of off-grid diesel generator-battery power systems. Int. J. Emerging Electric Power Syst. 15 (2), 161–170. doi:10.1515/ijeeps-2013-0134
Jamal, T., Urmee, T., Calais, M., Shafiullah, G. M., and Carter, C. (2017). Technical challenges of PV deployment into remote Australian electricity networks: a review. Renew. Sustain. Energ. Rev. 77, 1309–1325. doi:10.1016/j.rser.2017.02.080
Jiang, F., Xie, H., and Ellen, O. (2018). Hybrid energy system with optimized storage for improvement of sustainability in a small town. Sustainability 10, 2034. doi:10.3390/su10062034
Kaye, J. (1994). “Optimising the value of photovoltaic energy in electricity supply systems with storage.” in 12th EC Photovoltaic Solar Energy Conference, Amsterdam, 431–434.
Khoury, J., Mbayed, R., Salloum, G., Monmasson, E., and Guerrero, J., (2016). "Review on the integration of photovoltaic renewable energy in developing countries—special attention to the Lebanese case", Renew. Sustain. Energ. Rev., vol. 57, pp. 562–575.
Lal Kumar, D., Bhusan Dash, B., and Akella, A. K. (2011). Optimization of PV/Wind/Micro-Hydro/Diesel hybrid power system in HOMER for the study area. Int. J. Electr. Eng. Inform. 3 (3), 307–325. doi:10.15676/ijeei.2011.3.3.4
Madziga, M., Rahil, A., and Mansoor, R. (2018). Comparison between three off-grid hybrid systems (solar photovoltaic, diesel generator and battery storage system) for electrification for Gwakwani village, South Africa. Environments 5, 57. doi:10.3390/environments5050057
Mehrpooya, M., Mohammadi, M., and Ahmadi, E. (2018). Techno-economic-environmental study of hybrid power supply system: a case study in Iran. Sustain. Energ. Technol. Assess. 25, 1–10. doi:10.1016/j.seta.2017.10.007
Mhusa, N. J., Nyakoe, G. N., and Mgaya, E. V. (2015). Power management in photovoltaic-wind hybrid system based on Artificial intelligence. J. Multidisciplinary Eng. Sci. Technol. (Jmest) 2 (1), 140–148.
Micheli, L., Sarmah, N., Luo, X., Reddy, K. S., and Mallick, T. K. (2012). Opportunities and challenges in micro- and nanotechnologies for concentrating photovoltaic cooling. A review” Renew. Sustainable Energ. Rev. 20, 595–610. doi:10.1016/j.rser.2012.11.051
Moghaddam, S., Bigdeli, M., Moradlou, M., and Siano, P. (2019). Designing of stand-alone hybrid PV/wind/battery system using improved crow search algorithm considering reliability index. Int. J. Energ. Environ. Eng. 10, 429–449. doi:10.1007/s40095-019-00319-y
Mohammadnezami, M. H., Ehyaei, M. A., Rosen, M. A., and Ahmadi, M. H. (2015). Meeting the electrical energy needs of a residential building with a wind-photovoltaic hybrid system. Sustainability 7, 2554–2569. doi:10.3390/su7032554
Muselli, M., Notton, G., Poggi, P., and Louche, A. (2000). PV-hybrid power systems sizing incorporating battery storage: an analysis via simulation calculations. Renewable Energy 20 (1), 1–7. doi:10.1016/S0960-1481(99)00094-4
National Aeronautics and Space Administration (NASA) (2010). Atmospheric science data center Available at: http://eosweb.larc.nasa.gov/sse/2010. (Accessed September 9, 2020).
Olatomiwa, L., Mekhilef, S., Ismail, M., and Moghavvemi, M. (2016). Energy management strategies in hybrid renewable energy systems: a review. Renew. Sustain. Energ. Rev. 62, 821–835. doi:10.1016/j.rser.2016.05.040
Onur, O. M., and Ismail, H. A. (2015). A new energy management technique for PV/Wind/Grid renewable energy system. Int. J. Photoenergy 2015, 356930. doi:10.1155/2015/356930
Owusu, P. A., and Asumadu-Sarkodie, S. (2016). A review of renewable energy sources, sustainability issues and climate change mitigation. Cogent. Eng. 3, 1167990. doi:10.1080/23311916.2016.1167990
Panajotovic, B. (2010). "Design and “inteligent” control of hybrid power system in telecommunication."in Melecon 2010 - 2010 15th IEEE Mediterranean Electrotechnical Conference, 26–28 April 2010, Valletta, Malta, (Piscataway: IEEE)
Parida, B., Iniyan, S., and Goic, R. (2011). A review of solar photovoltaic technologies. Renew. Sustainable Energ. Rev. 15, 1625–1636. doi:10.1016/j.rser.2010.11.032
Paul, G. (2004). Wind power: renewable energy for home, farm, and business. Hartford, Vermont, United States: Chelsea Green Publishing Company.
Pipattanasomporn, M. (2004). “A study of remote area internet access with embedded power generation.” PhD dissertation. Blacksburg, (VA): Virginia Polytechnic Institute and State University.
Poullikkas, A. (2010). Technology and market future prospects of photovoltaic systems. Int. J. Energ. Environ. 1 (4), 617–(634.)
Qonain, R., Rafi, M., Khan, I., and Sageer, S., (2018). Ecological and economical friendly analysis of a hybrid solar grid diesel connected power generation system. Int. J. Appl. Power Eng. (Ijape) 7 (1), 1–9. doi:10.11591/ijape.v7.il.pp1-9
Ranjit, S. S. S. (2016). “A design Scheme of energy management, control, optimisation system for hybrid solar-wind and battery energy storages system.” Ph.D. Dissertation. London, United Kingdom: Department of Electronic & Computer Engineering, College of Engineering, Design and Physical Sciences Brunel University London.
Reboredo, J. C. (2015). Renewable energy contribution to the energy supply: is there convergence across countries? Renew. Sustain. Energ. Rev. 45, 290–295. doi:10.1016/j.rser.2015.01.069
Samuel, P. (2014). “Wind turbine Selection: a case-study for búrfell, Iceland.” MS Dissertation. Reykjavík, (Iceland): Department of Sustainable Energy Engineering Iceland School of Energy, Reykjavík University
Sassi, A., Zaidi, N., Nasri, O., and Slama, J. B. H. (2017). “Energy management of PV/Wind/Battery hybrid energy system based on batteries utilization optimization.” in 2017 International Conference on Green Energy Conversion Systems (GECS), 23–25 March 2017, Hammamet, (IEEE).
Sathish, K. K., and Shivarama, K. K. (2015). A review on hybrid renewable energy systems. Renew. Sustainable Energ. Rev. 52, 907–916. doi:10.1016/j.rser.2015.07.187
Sen, R., and Bhattacharyya, S. C. (2014). Off-grid electricity generation with renewable energy technologies in India: an application of HOMER. Renew. Energ. 62, 388–398. doi:10.1016/j.renene.2013.07.028
Shoeb, M., and Shafiullah, G. (2018). Renewable energy integrated islanded microgrid for sustainable irrigation—a Bangladesh perspective. Energies 11, 1283. doi:10.3390/en11051283
Sidrach de Cardona, M., and Mora Lopez, L. I. (1992). “Optimizing of hybrid photovoltaic generator systems for installations of rural electrification.” 11th European Photovoltaic Solar Energy Conference, Montreux, 1287–(1290.)
Vivas, F., Heras, A. D. L., Segura, F., and Andújar, J. (2018). A review of energy management strategies for renewable hybrid energy systems with hydrogen backup. Renew. Sustain. Energ. Rev. 82, 126–155. doi:10.1016/j.rser.2017.09.014
Wesseh, P. K., and Lin, B. (2015). Renewable energy technologies as beacon of cleaner production: a real options valuation analysis for Liberia. J. Clean. Produc. 90, 300–310. doi:10.1016/j.jclepro.2014.11.062
Yasmeena, S., and Das, G. T. (2015). A review of technical issues for grid connected renewable energy sources. Int. J. Energ. Power Eng. 4 (5–1), 22–32. doi:10.11648/j.ijepe.s.2015040501.14
Yogesh, P., and Sendil, M. (2020). Photovoltaic fuel cell hybrid system implemented by Matlab/Simulink. Available at: https://www.scribd.com/document/102608759/Hybd-Cell-Wordform (Accessed October 3, 2020)
Keywords: power management system, hybrid system, optimization, control algorithm, fuel cell, renewable system, integrated system
Citation: Ani VA (2021) Development of an Intelligent Power Management System for Solar PV-Wind-Battery-Fuel-Cell Integrated System. Front. Energy Res. 9:613958. doi: 10.3389/fenrg.2021.613958
Received: 04 October 2020; Accepted: 19 January 2021;
Published: 18 March 2021.
Edited by:
George Xydis, Aarhus University, DenmarkReviewed by:
András Dán, Budapest University of Technology and Economics, HungaryKenneth Okedu, Caledonian College of Engineering, Oman
Bhupendra Gupta, Jabalpur Engineering College, India
Copyright © 2021 Ani. This is an open-access article distributed under the terms of the Creative Commons Attribution License (CC BY). The use, distribution or reproduction in other forums is permitted, provided the original author(s) and the copyright owner(s) are credited and that the original publication in this journal is cited, in accordance with accepted academic practice. No use, distribution or reproduction is permitted which does not comply with these terms.
*Correspondence: Vincent Anayochukwu Ani, YW5heW9jaHVrd3UudmluY2VudEBnbWFpbC5jb20=