- 1Department of Physics, University of the West Indies, Mona Campus, Kingston, Jamaica
- 2Biden School of Public Policy and Administration, University of Delaware, Newark, DE, United States
- 3Department of Engineering, University of Oxford, Oxford, United Kingdom
The energy security of each Caribbean Community (CARICOM) member state is a key issue specifically addressed based on the energy demands of each nation. St. Vincent and the Grenadines (SVG) has the potential to strengthen its energy sector through the exploitation of immense untapped natural geothermal resources. Currently, SVG is planning to integrate base load power through a 10 Megawatt-electric (MWe) geothermal power plant (GPP1). The paper aims to highlight a detailed resource assessment profile of the renewables across SVG and the projected benefits of the proposed 10 MWe geothermal power potential, such as the positive economic development (displacing 149,000 bbls of crude oil), and the transition to a more climate-sensitive nation (displacing an estimated 0.172 million tCO2e/year). In addition, a volumetric method (Monte Carlo simulations) has been applied to reveal that the geothermal reservoir can sustain a minimum of 31 MWe, 34 MWe and 92 MWe over the lifespan of 25–30 years, for well 1 (SVG01), well 2 (SVG02) and well 3 (SVG03) respectively. Given the findings of the assessment and simulations, several policy approaches are identified as potential means of enhancing geothermal resource development and leveraging the resource for the islands’ sustainable energy demands. These include incentivization for public-private partnerships, information certainty, regulatory processes, and strengthened institutions.
Introduction
A shared interest in emerging global economies and nations is that of energy security. The precedence of the Paris Agreement in 2015 has also struck a clear chord with global leaders for the continued commitment to achieve sustainable development pathways. Sovereign Caribbean countries were at the forefront of the ratification of the Paris Agreement and under the guidance of the United Nations Sustainable Development Goals, have sort to advance the renewable energy (RE) agenda to build climate resilience in tandem with needed development strategies. Many CARICOM member states are still heavily dependent on the importation of fossil fuels, some more than others to satisfy their respective energy demands. Energy consumption and energy production are two key parameters contributing to the overall energy landscape and eventual economic benefits or losses, and within the Caribbean, the cost of the domestic retail electricity rate is averaged at US $0.35/kWh (Energy Chamber, 2017). Historically there have been economic winners and losers when factoring the contribution of energy costs into national development agendas, but some countries such as Trinidad and Tobago, an oil-rich nation of fewer than 1.5 million people, has built stability on the exploitation of oil, leading to it to be ranked third for per capita carbon dioxide (CO2) emissions (The World Bank, 2021). Other islands such as St. Vincent and the Grenadines (SVG) did not have the economic buffers of native oil supplies and thus fuel imports continue to see the country face an electricity rate of US $0.26/kWh (Gay et al., 2018) with little change in recent years.
The region is facing many threats from a changing climate regime and the 2017 hurricane season has shown many Caribbean island governments the impacts of extreme events, particularly on energy resilience and security, further bolstering efforts for a cleaner energy transition (Klotzbach et al., 2018; Popke and Harrison, 2018). The recent Intergovernmental Panel on Climate Change (IPCC) 1.5°C Special Report, with special contributions by many Caribbean and Central American authors, calls for more aggressive emissions targets to mitigate higher risks in regions such as the Caribbean (Taylor et al., 2018). Islands such as Trinidad and Tobago are seen as having a nascent energy policy strategy and transition to renewables, whereas other vulnerable countries such as Dominica are taking steps to become climate-smart nations. Small island developing states (SIDS) such as SVG are not only largely vulnerable due to their isolated grids and location but by the centralized and homogeneous nature of energy production. Diversifying interconnections and supply to the main grid will buffer the severity of climate impacts and decentralize power distribution.
It is important to stress that Caribbean islands are vulnerable to the capricious global energy markets and fluctuations in the price of fossil fuel-based products when setting the regional energy context, and as such, there is a strengthened and continued transition away from conventional fuels toward renewable forms of energy. One such effort contributing to this transition has yielded a regional energy policy in 2013 (Ochs et al., 2015). Energy policies greatly complement the drive toward higher penetration rates of renewables for nations, particularly in developing regions such as the Caribbean (Timilsina and Shah, 2016). Understanding the RE resources of Caribbean islands has also been built into growing energy policies and countries are taking the necessary exploration and feasibility assessments to see the continued independence from fossil fuels. SVG for instance currently possesses immense untapped power beneath the islands where geothermal energy resources can be used for electricity generation.
The draft report of the St. Vincent Geothermal Project (SVGP) phase I Exploratory Drilling Environmental and Social Impact Assessment (ESIA) by the Environmental Resources Management (ERM, 2016), has positioned SVG along the pathway toward the integration of a 10 MWe geothermal power plant to the island’s electric grid. The proposed geothermal project is located on the main island of St. Vincent and the SVGP, has two phases, phase I (exploration) and phase II (production).
This paper aims to provide a three-front approach at a detailed RE analysis (beneficial aspects toward SVG’s environment, economy, and electrical grid), power potential analysis (Monte Carlo simulations) and policy interventions. Therefore, the paper examines the RE profile of SVG and highlights significant environmental findings such as the CO2 reductions in the atmosphere, the economic savings which are presented through future scenarios of avoidable cash flows/spending, and the benefits to the electrical grid are demonstrated through baseload power incorporation. Furthermore, the paper provides a volumetric analysis utilizing the Monte Carlo method which is performed for all three wells of the SVGP to generate 90% confidence intervals of maximum potential power output per well. Finally, the research has explored the current policy environment, through an assessment of the Eastern Caribbean context, policy and regulatory practices in other geothermal-endowed developing countries will inform the interventions necessary for SVG geothermal integration. This study provides novel insights to enhance the understanding of the SVGP through environmental, economic and electrical grid benefits, power potential analysis and significant policy interventions.
Renewable Energy Landscape
Transitioning Towards Geothermal Energy
St. Vincent Electricity Services Limited (VINLEC) is a state-owned utility that has an installed generation capacity of 58.3 MW (MW) with roughly 56% and 3% utilization of hydropower and solar power, which accounts for 5.6 MW and 0.69 MW respectively, with the remaining power generated via diesel generators (Ochs et al., 2015). Currently, geothermal power plays no role in providing power to SVG, however, if the potential 10 MWe geothermal power plant becomes operational it can significantly change the economic and utility aspects. The nation of SVG has two ambitious yet capable RE targets that have been established through the implementation of the National Energy Action Plan (NEAP). NEAP exemplified an action plan to merge and transition policies into actionable steps. Hence these two RE targets for electric output by NEAP are 30% RE by 2015 and 60% RE by 2020.
The introductory section discussed the effects of climate change across the Caribbean islands, with one such effect being more intense spells of drought. This certainly will affect the hydropower sector available capacity (which can be reduced), which already is impacted by the dry season conditions across SVG. Therefore, the introduction of a RE resource that isn’t affected by climatic conditions will prove advantageous. Furthermore, geothermal power plants exemplify high capacity factors, as high as 90%, which allows the plant to run non-stop throughout the year, hence becoming a more consistent supply of baseload energy as opposed to other renewables. Geothermal energy (naturally high enthalpy hydrothermal systems) is a technically beneficial avenue across the Caribbean (young geological volcanic arch of islands) that is collectively comprised of 890 MW (Ochs et al., 2015) of resource potential, and can generate 24/7 low-carbon baseload power. Furthermore, to obtain a solidified grasp of the extent of geothermal power potential (GPP2) across the Eastern Caribbean islands a breakdown of GPP2 to other forms of major renewables per nation is highlighted in Figure 1. Geothermal energy can have applications either deep within the Earth’s crust or through temperatures near the surface. Hence depending on the temperature of the system, it can then be used for a geothermal heat pump, power plant or direct usage. Direct use purposes of geothermal energy can be used in agriculture mainly crop drying, greenhouse, aquaculture pond heating and animal husbandry, heat pumps for heating and cooling system, bathing and swimming, therapeutic use and district heating (Lund, 2018). Most direct-use temperature ranges between 5°C and 150°C in the low to medium temperatures for geothermal fluid, where geothermal fluids are extracted from reservoir drilling equipment.
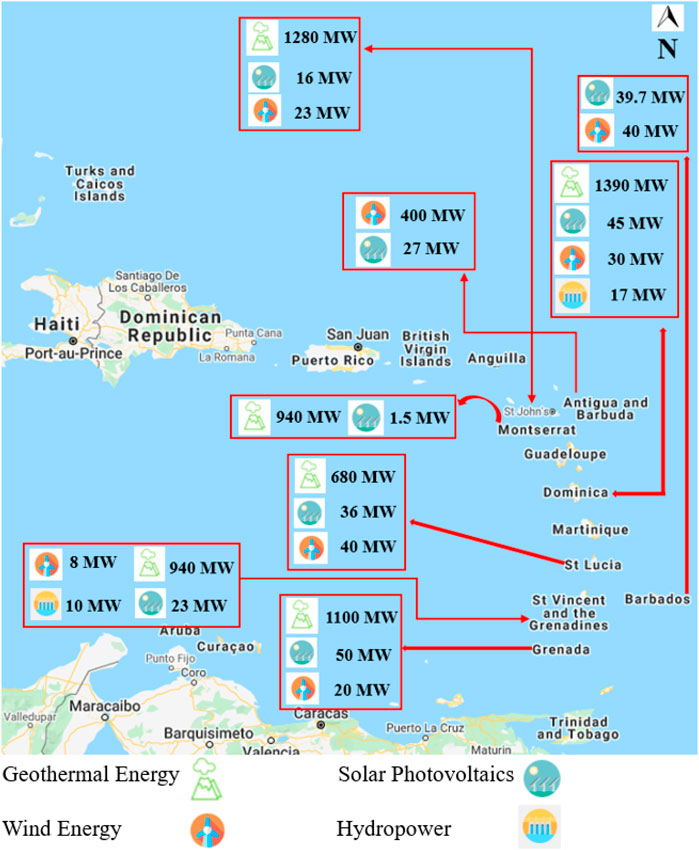
FIGURE 1. Renewable energy potential comparison across the Eastern Caribbean nations (Source: Ochs, et al., 2015).
St. Vincent Geothermal Landscape
Geothermal energy is a renewable form of energy and can be considered as heat mining. With proper energy efficiency practices of the reservoir, this form of energy becomes a sustainable usually over a 25–30 years timescale. Through the identification of subsurface reservoirs employing geochemical and geophysical methods consisting of hot fluid, within a permeable rock matrix (fractured network) the geothermal fluid can then be extracted. The Caribbean possesses active volcanism as a result of its proximity to active geological plates. These nations are on the crustal plate moving eastward along the North and South American plates whilst simultaneously being subducted by the westward-moving Atlantic Plate (Huttrer, 2010). Thus, like many other Eastern Caribbean (EC) islands, SVG is ideally situated along the Caribbean’s archipelago formed from a relatively young volcanic system, which physically impacts the island’s geology and landscape. Impure limestone and coral are the main exposed sedimentary rock types as found through the ERM (2016). The northern part of the main island, St. Vincent where the project site is located, mainly consists of pyroclastic deposits of the pre-Soufrière volcanic center. Within this mountainous region, a maximum slope of 8–16° exists across the project area terrain. Therefore, the site selection of the geothermal project is certainly impacted based on the geology of the island and its positioning relevant to the island’s volcano. It is determined that phase I of the geothermal project, entails two geothermal well pads (W1 and W3), each consisting of three wells. Exploratory drilling on the well pads will reach depths of 1 km–3 km for the extraction of geothermal fluid.
The conceptual model in Figure 2, provides a deeper understanding of the approximate depth, surface manifestations (fumaroles, hot springs), and isotherms (lines of constant temperature). Thus, the volcanic area is ideally situated for the presence of temperatures up to 230°C within the geothermal reservoir (ERM, 2016). St. Vincent like many other Caribbean islands exhibits conventional-type geothermal systems also known as hydrothermal systems. The three main parameters of a heat source, fluid flow at depth and a fractured network (permeable system) are all accounted for within this type of geothermal system.
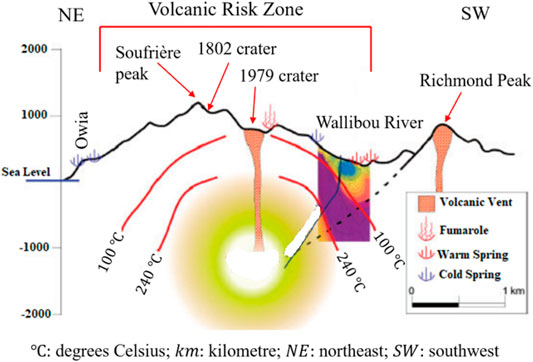
FIGURE 2. Reconstructed conceptual model of St. Vincent geothermal reservoir. This Figure has been reconstructed with permission granted from Reykjavik Geothermal Limited (Source: Reykjavik Geothermal, 2013).
Assessing the Renewable Energy Targets
Wind, solar and hydropower are among the major RE interests currently for SVG, however, this section of the paper assesses the possibility of integrating geothermal energy into the overall energy mix to reach the desired energy targets. For discussion, the 2027 RE target numbers are utilized as it is forecasted that in 2027 SVG will have an installed capacity of 108 MW of power, from which 32.4, 20.52, 6.48 and 4.32 MW of power are derived from wind, solar, hydropower and biomass respectively. Collectively, this accounts for 63.72 MW or roughly 59% RE integration. Hence, the implementation of the 10 MW geothermal power plant can have an enhanced outcome for the RE targets. In the reconstruction of Table 1, the conversion factors are reanalyzed to obtain the number of barrels of oil (bbl) from the calculated MWh/year (Society of Petroleum Engineering, 2020). CARICOM member states mainly employ the use of low-speed diesel engines to generate electricity, as such this results in emissions factors of about 680 gCO2e/kWh (Gay et al., 2018). Relevant capacity factors for the renewables were obtained from the United States Energy Information Agency (United States EIA, 2019).
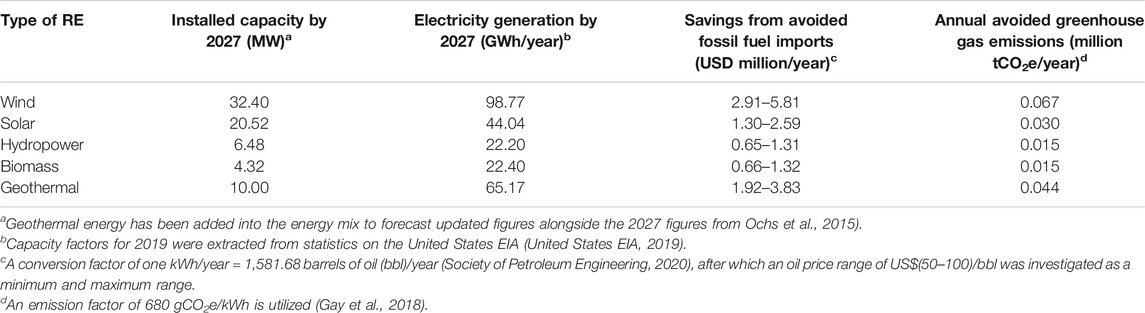
TABLE 1. Reconstructed data (estimated) for major forms of renewables for 2027 identifying energy installation, generation, avoided fuel imports and greenhouse gas emissions.
SVG has made RE progress from 2015 to 2018 particularly through solar photovoltaics. Statistics in 2018 indicate the total installed RE stands at 8.82 MW, this represents an increase of about 2.40 MW and 0.13 MW for solar photovoltaics and hydropower respectively from 2015 to 2018 according to the Caribbean Center for Renewable Energy and Energy Efficiency (CCREEE, 2018). The integration of geothermal energy through distributed generation into the VINLEC network presents a major opportunity to increase energy security, whilst improving the balance of trade by reducing oil importation. It should be noted that the proposed transmission line alignment plan will encounter challenges. From the proposed geothermal site two transmission lines will be fed into the nation’s electrical grid network, one along the Windward Highway and the other through an inland route. The inland route has the potential to impact sensitive species habitats and as such a transmission line feasibility study will be required to recommend an alternative route (ERM, 2016).
The paybacks through higher penetration rates of renewables and geothermal energy, in particular, can be translated to the following:
- The positive economic development associated with the avoided costs implying future savings as a result of the reduction and dependence on fossil fuel-based importations for electricity generation.
- The environmental and climatic mitigations through the reduction of the number of barrels of oil imported per year resulting in a favorable decline in the number of tonnes of CO2 emission avoided.
- VINLEC will have enhanced reliability of power having geothermal energy integration into the national grid owing to base load stabilities.
Projected Economic Benefits
Geothermal energy potential in SVG is projected at around 890 MW (Ochs, et al., 2015) of power, however, through the introduction of a 10 MWe power plant, this can yield significant future benefits to the island. Furthermore, this projected installed capacity can be absorbed into the energy mix providing invaluable base-load power. At the 2027 projections, wind energy capacity is more than double that of geothermal energy. However, geothermal still leads in all probabilistic scenarios (Figure 3) and more importantly will provide the greatest reduction in the estimated millions of imported bbls. Through geothermal energy, major economic alleviations can be attained, where a 2027 projection, inclusive of the 10 MWe geothermal power plant, will displace 149,000 bbls (Table 1). A detailed breakdown of the estimated number of bbls displaced per renewable is illustrated through label callouts in Figure 3. Collectively, all projected cases of renewables account for an estimated 205,000 bbls, with geothermal having 36.5% (the largest) of any projected renewable. Three cases are used to further display the variation of the price per bbl at US$50.00, US$75.00 and US$100.00 accounting for roughly, US$3.74 million, US$5.61 and US$7.48 million/year respectively through a 10 MWe geothermal injection. Future studies on this aspect can be linked to Key Performance Indicators (KPIs) such as Net Present Value (NPV) and Rate of Return (RoR), where a full economic evaluation of the project can be assessed for other potential wells across the island.
Toward a More Climate-Sensitive Region
Given the Caribbean’s vulnerability to climate change through rising sea-levels, intensified hurricane activity and precarious changes in water resources and precipitation, many islands are increasingly divesting in imported fossil fuels. It has been well understood for many years that the Caribbean region needs to adapt more mitigative and adaptive responses to address a future climate under global warming (Taylor et al., 2012). There is an enhanced desire for greater integration of renewables through distributed generations that are key to the reduction of greenhouse gases (CO2 in particular) being released into the atmosphere. Potential geothermal generation through the projected 10 MWe power plant can displace an estimated 0.044 million tCO2e/year, which is second in leading the charge toward CO2 reduction of all other renewables for the 2027 projections, even when other renewables are projected to have a greater number of installed power capacity compared to geothermal as seen in Figure 4. Regardless, geothermal power plants lead the way through higher capacity factors compared to other renewables such as wind and solar (with predominantly larger projected installed capacities), and as such, possess larger electricity generation potential with the displacement of the greatest quantity of CO2 to the atmosphere. Future in-depth investigations can be performed to evaluate other aspects that have incorporated the inherent carbon footprint associated with the construction of the plant (transport of materials, construction, etc.).
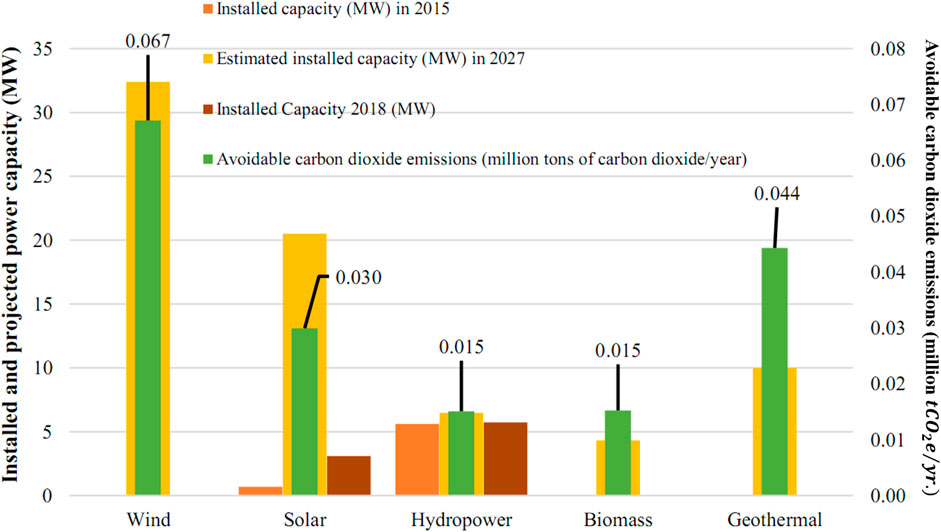
FIGURE 4. Projected 2027 scenarios of estimated power capacities and avoided carbon dioxide emission through existing renewables and the introduction of the 10 MWe geothermal power plant(Source: Gay et al., 2018; Ochs et al., 2015).
Geothermal Resource Assessment
The SVGP has been planned for development in two phases. Phase I–Exploration consists of the drilling and testing of two exploratory geothermal well pads and associated project facilities (e.g., water system, road improvements); phase II–Production, consists of the development of a geothermal power plant and transmission lines to bring electricity to the SVG power grid. The objective of phase I is to validate and quantify the Soufrière geothermal reservoir characteristics and suitability for production through exploratory drilling. After confirmation, SVGP would continue on to phase II. Phase II will also consist of the development of a geothermal power plant and transmission lines to bring geothermally derived electricity to the SVG power grid.
In 2019, SVG began its drilling program and results from the three wells have been summarized in Table 2 below.
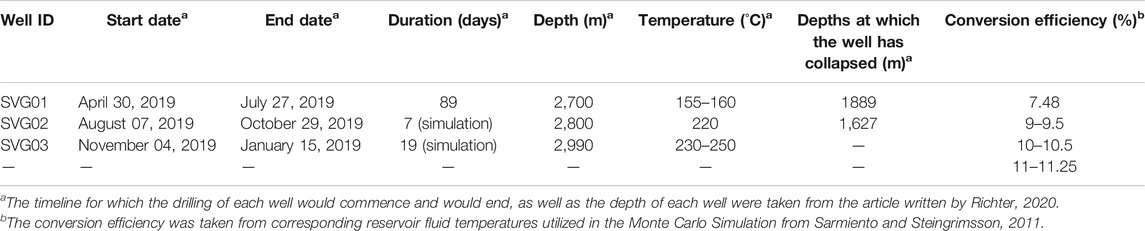
TABLE 2. Table identifying the updated data for the SVG01, SVG02 and SVG03 drilled to date, duration, fluid temperature, depths the wells have collapsed and the estimated conversion efficiency.
Characteristics of the Resource Area
The surface area was quantified using the geological and geophysical features of the island of St. Vincent. A fixed distribution was assigned and confirmed an area of
Figure 5 entails two geothermal well pads (W1 and W3), each consisting of three wells. The SVGP phase I exploratory drilling includes drilling deep wells (between 1 km and 3 km in depth) into the Earth’s crust which will then classify the thermal energy found in underground reservoirs of being either geothermal water or steam in composition. In addition, Richter (2019) stated that the preliminary well reached a depth of 2.8 km and collapsed in the last 1 km segment without permeability.
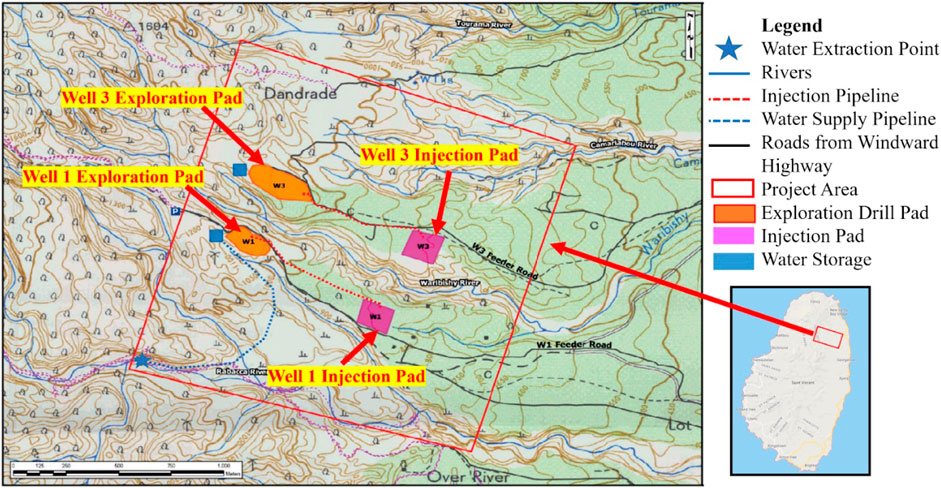
FIGURE 5. Reconstructed map of the geothermal resource and exploration site of phase one of St. Vincent geothermal plant. This Figure has been reconstructed with permission granted from Reykjavik Geothermal Limited (Source: ERM, 2016).
The geology of St. Vincent, situated in the Lesser Antilles, is characterized by highly volcanic rocks (igneous in nature) as a result of the Soufrière Volcano eruption in 1979. The northern area of St. Vincent is dominated by basaltic-andesites alongside xenoliths, where basaltic rocks are formed in the southern region of the island (ERM, 2016). The reservoir temperature value was revamped to a minimum value of 240°C to allow for the well to self-discharge (Sarmiento and Steingrimsson, 2011). The maximum value for the reservoir temperature was taken to be 310 °C which corresponds to the maximum expected value of the first well according to Blaha and the ERM (2016). The base temperature was modified to be 180 °C to account for the type of geothermal plant, whether it be binary, dry steam or flash steam plants. Hence, the Monte Carlo method can then be utilized to determine the potential and probable extent of power extraction of all three wells as seen in Table 2.
Volumetric Analysis–Monte Carlo Applications to the St. Vincent and the Grenadines Geothermal Resource Assessment
The volumetric approach is the primary simulation tool used in geothermal evaluations. It is considered the most realistic solution during the premature stages of geothermal production and exploration (Samiento and Bjornsson, 2007; De Freitas, 2016; Ofwona, 2008; Shah et al., 2018). Such calculations are performed to determine the generating capacity of potential power plants in various countries such as China and Dominica which have demonstrated that the approach is accurate to estimate the minimum engagement for geothermal fields with less than two or three exploratory wells (Samiento and Bjornsson, 2007). According to De Freitas (2016), there are many disadvantages in volumetric approaches in which it can overestimate and underestimate effects and doesn’t compensate for the normal reservoir recharge due to model limitations. Monte Carlo simulations (MCSs) are often used to overcome this ambiguity. Furthermore, they are used to ensure the reliability of a premature geothermal power plant, and to estimate the plant power potential that could be produced. A previous study highlighted these findings in the Olkaria I well (Kenya) where its plant reached its design life which leads to subsequent studies to determine whether the reservoir can still support further production, utilizing MCS’s to carry out estimations of the stored heat energy in the reservoir (Ofwona, 2008).
The Monte Carlo method can be utilized through three types of distribution such as uniform (rectangular), triangular and normal distributions. Normal and triangular distributions are categorized as parameters having limits and are defined whereas a rectangular distribution parameters, for simulation such as one of Monte Carlo, should be estimated or approximated. Fixed distribution parameters are constant parameters that are known or values that are well defined (Ofwona, 2008). The overall approach toward the Monte Carlo method has been succinctly illustrated in Figure 6. The Monte Carlo Simulation program is embedded in MS Excel. The Visual Basic Applications (VBA) code script (Figure 7) is a macro, which is a computer code, written in Excel, using the VBA Programming language. The supplementary VBA code script assists Figure 6 which demonstrates the methodology for the Monte Carlo Simulation. The power potential is assigned yi and the code iterates the random maximum and minimum values such as fluid density, fluid specific heat seen in step 1 for Figure 6. The parameters are evaluated, and the result is stored in yi where the simulation performed a total 20,000 sets of results. The results are displayed and analyzed using the cumulative frequency and probability distribution graphs as seen in Figures 8–10.
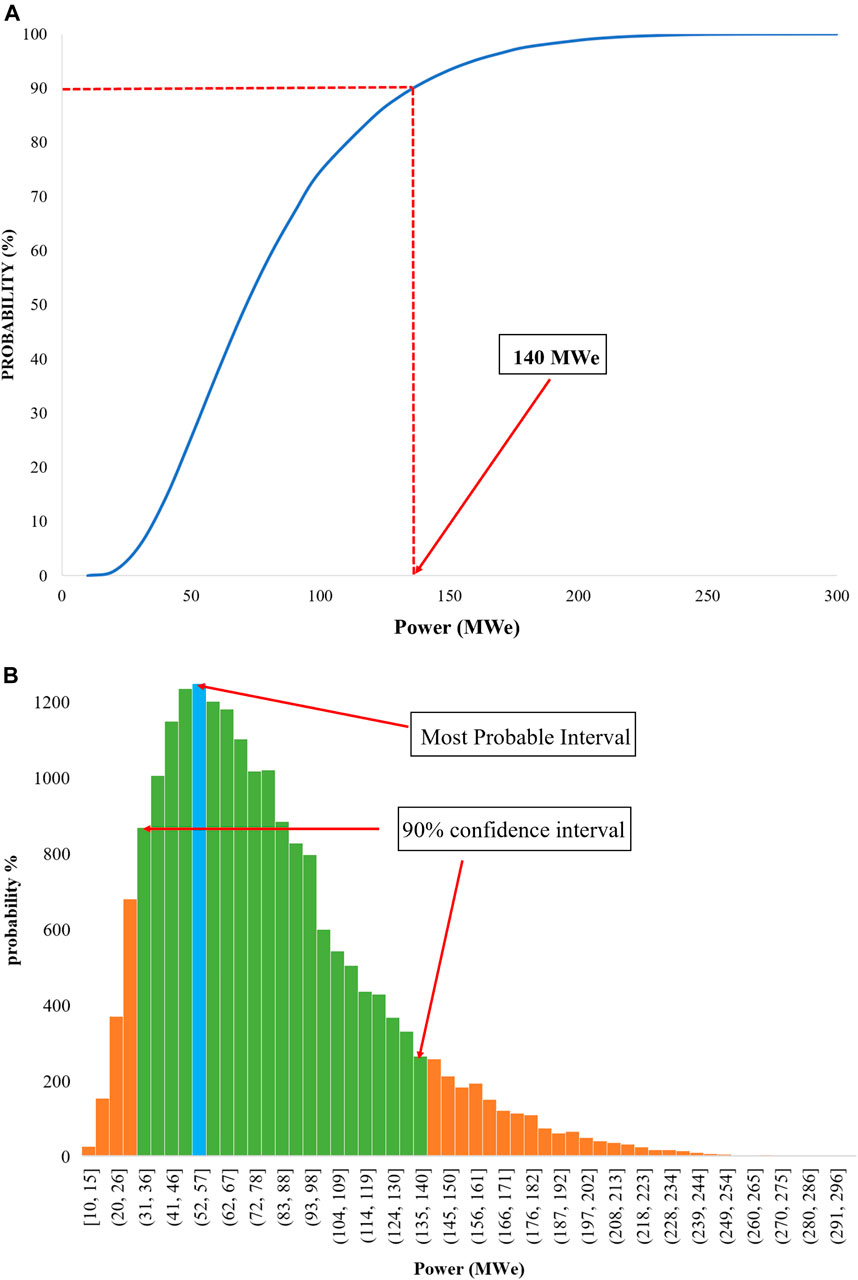
FIGURE 8. Monte Carlo probability distribution analyses for electrical power production (MWe) for SVG01, (A) volumetric cumulative and (B) frequency.
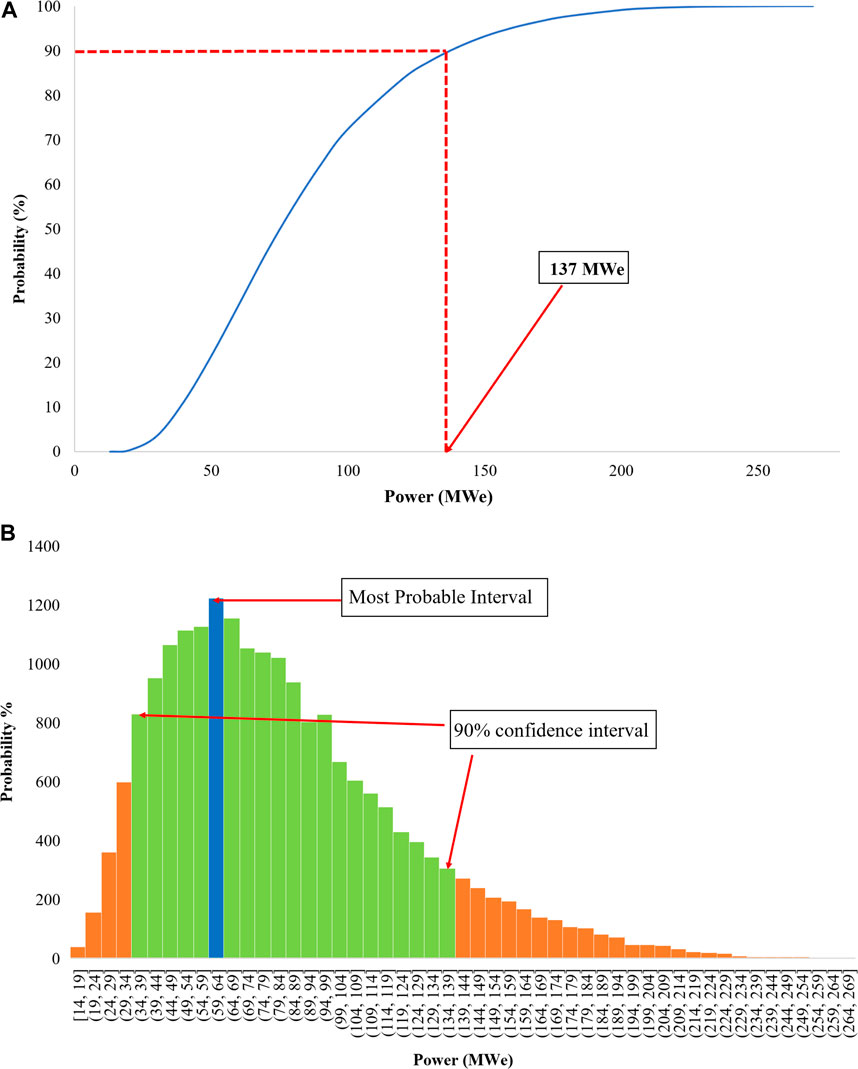
FIGURE 9. Monte Carlo probability distribution analyses for electrical power production (MWe) for SVG02, (A) volumetric cumulative and (B) frequency.
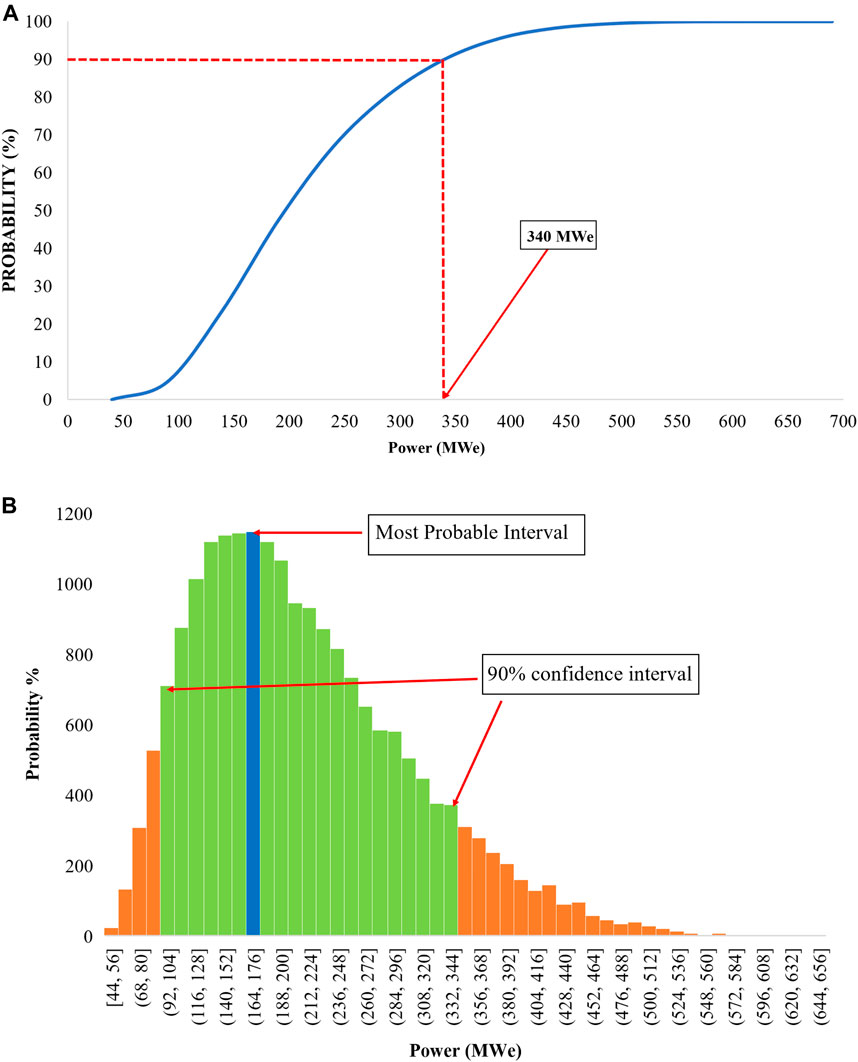
FIGURE 10. Monte Carlo probability distribution analyses for electrical power production (MWe) for SVG03, (A) volumetric cumulative and (B) frequency.
Geothermal resource evaluation is an estimation of the amount of thermal energy consumed below the surface of the Earth that can be collected from the geothermal reservoir and used efficiently for a long period (Shah et al., 2018). The volumetric approach is focused on the calculation of total heat energy retained in rocks and fluids contained in pores. The equations used for measuring thermal energy for a reservoir occupied by liquids (Ofwona, 2008; Sarmiento and Steingrimsson, 2011) is as follows:
where
where the above parameters are as follow:
The determination of the power plant sizing that can be supported by the resource is a crucial aspect of the overall project. This can be determined through the use of the following equation:
where
Resource Area
The surface area was quantified using the geological, geophysical features of the country. A fixed distribution was assigned and confirmed area of 4
The St. Vincent Geothermal Project would be developed in two phases. Phase I–Exploration (the Project), consists of the drilling and testing of two exploratory geothermal well pads and associated project facilities (e.g., water system, road improvements); phase II–Production, consists of the development of a geothermal power plant and transmission lines to bring electricity to the SVG power grid. The objective of phase I is to confirm La Soufriere Volcano geothermal reservoir characteristics and suitability for production through exploratory drilling. After confirmation, SVGCL would continue onto phase II. Phase II–Production, consists of the development of a geothermal power plant and transmission lines to bring geo-thermally derived electricity to the SVG power grid.
Reservoir Thickness
Reservoir thickness was assigned a triangular distribution. It is determined that phase I of the geothermal project, entails two geothermal well pads (W1 and W3), each consisting of three wells.
The St. Vincent Geothermal Project phase I exploratory drilling Project includes drilling deep wells (between 1,000 and 3,000 m deep) in the Earth’s crust describe the thermal energy found in underground reservoirs of geothermal water or steam. In addition, Richter (2019) stated that the preliminary well reached a depth of 2,800 collapsed in the last 1,000 m segment without permeability (Richter, 2019).
Recovery Factor
Recovery factor is the ratio of extracted thermal energy to the whole geothermal energy contained in given volumes of rock and water (Muffler, 1979). Usually, 25% recovery factor corresponds to the 10% for Andesite rocks porosity. A triangular distribution ranging from 0.1 to 0.25.
Porosity
Porosity is the volume of void spaces. So, based on the rock density the porosity can be found. A fixed distribution was assigned to porosity for all three wells. The porosity is 10% which corresponds to the andesite rock density (De Freitas, 2016).
Reservoir Fluid Temperature
The reservoir temperature value was revamped to 240°C. The reservoir temperature should be this minimum value to allow for the well to self-discharge (Sarmiento and Steingrimsson, 2011). The maximum value for the reservoir temperature was taken to be 310°C which corresponds to the maximum expected value of the first well according to Blaha and the Environmental Resources Management (ERM, 2016).
The potential power output is a function of numerous parameters of the geothermal reservoir and the powerplant . As such, Table 3 highlights all parameters used throughout this process and the best estimates for the input parameters for the MCS, highlighted in Table 3, were selected from various data sources. The values were compared for each source and a fixed value (with confidence limits) were assigned as suitable estimates. Utilizing the best estimates of parameters from Table 3, the MCS was then undertaken and the results are shown in Figures 8–10.
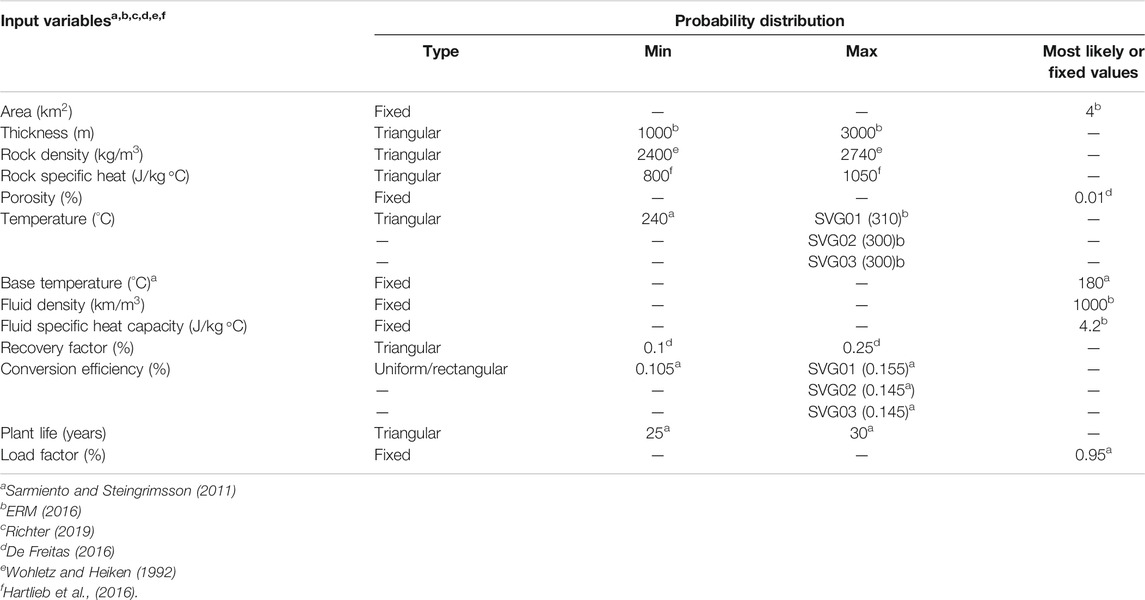
TABLE 3. Best estimates of input parameters for all three wells (SVG01, SVG02, SVG03) for the volumetric assumption utilized for the Excel-based Monte Carlo simulation.
The input parameters used in the SVGP W1 (SVG01), were used to carry out the MCS. The accuracy of the simulation increases with the number of runs performed, and therefore, a total number of 20,000 runs were undertaken. The MCSs yielded two distinctive graphs, that of cumulative probability curves as a function of the potential power output and frequency probability distribution function. Each well the results indicate that there is a 90% probability that the output of the geothermal plant installation will generate up to 140, 137 and 340 MWe for wells SVG01, SVG02 and SVG03 respectively over the expected lifetime of 25–30 years. Table 4 further summarizes additional key findings from the MCSs.
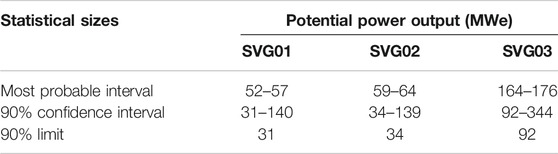
TABLE 4. The Monte Carlo generation capacity estimates for SVG01, SVG02, and SVG03 for the generation period of 25–30 years.
The nation of SVG has undertaken the approach to integrate a 10 MWe geothermal power plant. Based on the findings of the Monte Carlo simulations the reservoir can sustain a minimum of 31, 34, and 92 MWe over the lifespan of 25–30 years, for SVG01, SVG02, and SVG03 respectively. It should be noted that the 90% limit specifies that the reservoir can be sustained at a minimum power capacity when extended between 25 and 30 years, which is generally the generation time for geothermal plants. The power potential of the wells or outcomes can be reduced since the volumetric method does not take into consideration the possibility that natural causes may result in the recharge or possible depletion of the reservoir. Therefore, the SVGP approach toward a 10 MWe geothermal power plant is 3.1 times less than the initial 90% confidence interval of the MCSs, however, this can be viewed as a conservative and more financially stable investment.
During the premature stages of a geothermal power plant, the favorable method used to investigate the resource assessment is the volumetric method. The volumetric method is defined by the calculation of thermal energy, which is stored in Earth especially in rocks and fluids where an area is removed based on specific reservoir characteristics. Knowledge of such key findings (potential power output) leads to a better understanding of the system sizing (resource) and its economic viability.
Policy Interventions for Renewable Energy
A future SVG that is transformed through the utilization of a diverse RE portfolio depends on the policy foundations set in place now. Incentive-oriented policies are capable of attracting RE investors thereby increasing the penetration rates of RE. Furthermore, the economic benefits can be bestowed upon this nation by aggressively implementing and enforcing the required policies and regulatory framework for greater RE integrating and energy efficiency standards. Some of such policy instruments in place in SVG are net metering/billing, tax reduction/exemption to promote energy efficiency, and restrictions on incandescent bulbs (Shah, 2018).
Future plans to grant generation of electricity from renewable sources by independent power producers and developing a net-metering scheme for small-scale RE generators are progressive steps. Through net-metering, independent power producers will be reimbursed with monetary value rather than a reduced future electricity rate that net-billing proposes. SVG National Energy Action Plan also includes energy efficiency practices across the island especially regarding generation and consumption. This support is targeted toward fiscal incentives, energy audits and efficiency standards.
Another major policy adaptation should allow the energy markets of SVG to open up further to incorporate the flexible and cost-effective provision of stored energy. A recent study by Chen et al. (2020) argues that the increased injection of Battery Energy Storage Systems (BESSs) within the energy landscape of the Caribbean, such as Jamaica in their study, will create further opportunity of RE development, grid stabilization, and the overall reduction of energy prices under optimal scenarios of implementation. Battery technologies, in its various forms of chemistries and thermodynamics, have sharply dropped in recent years and will continue to see further competitiveness (though price bottoms exist that make certain technologies less competitive) as global markets respond to the increased supply of stored energy (Hsieh et al., 2019). Examples of battery storage implementations across the Caribbean such as in Jamaica, Barbados and the Bahamas (Ashtine et al., 2018) have shown that increased storage will allow islands such as SVG to further explore RE sources of energy as it transitions to a low-carbon economy.
One major part of the SVG energy policy that requires strengthening is on the financing side. As government investment policy is developed, there must be strict requirements for transparency, accountability and fiscal compliance. As of the date, the exploratory drilling for geothermal energy in the La Soufrière region of the country has been funded by a contingent grant which will need to be repaid only if the project is a success. This was made public by the SVG government, in the midst of reports that the permeability of the first drilled well fell below expectations. The loan is valued at US$15.9 million with a 3.5% interest rate. The drilling contract for the geothermal project in SVG costs $21 million. Drilling on the third well (SVG03) is currently underway and the way in which the financing is set up, the facility mitigates the risk so there is no exposure to the government or stress on the government to pay back capital if the project fails. This contingent grant is a grant through the International Development Bank (IDB) which comes through the Caribbean Development Bank (Caribbean Development Bank, 2016) and this is the money that was used to do the first phase of drilling.
The Policy Environment for Geothermal Development in St. Vincent and the Grenadines
Since EC economies are relatively small markets with low population numbers, overall energy demand is also relatively low. Notwithstanding this, intended and projected industry growth in areas such as ports, logistics and tourism are likely to increase energy demands moving forward. Geothermal energy projects are normally capital–intensive and bear high costs for surface exploration and exploratory drilling of potential sites in the early stages of development and other significant costs in the importation of the technology, insurance, and freight as EC countries are non–manufacturers of these technologies and in subsequent construction activities. There is also the situation of a lack of local/regional technical expertize required to develop geothermal energy projects and to regulate them. Owing to the relatively small energy demand, the size of geothermal projects has to be scaled down which adds to more complex issues along the geothermal development path.
The RE industry in SVG is mostly driven by the public sector but for the scale of investment necessary for geothermal projects, the investment will need to come from the private sector. Private investors can bring financial resources as well as vast technical expertize to drive those projects but getting the attention of private developers, in this case, is a challenge (Shah and Niles, 2016). There is a high level of uncertainty involved as exploration and drilling that can lead to nowhere and constitute a risk to project developers, investment has to be made in training the necessary workforce and the low level of energy demand and small plant size can lead to longer payback period over the total capital invested. In many EC countries, a poor institutional, legal and regulatory framework is an additional barrier as well as the poor negotiation capabilities in signing wide-reaching contracts with utilities and potential investors (OECS, 2018). Regulatory functions are distributed among different ministries and governmental agencies and laws governing the exploration of the geothermal resource are lacking. In a situation where a public-private partnership (PPP) is required, there are still questions of whom to grant the ownership of the resource and the responsibility for its monitoring for the sustainable usage of the geothermal reservoir (Gischler et al., 2017).
The aforementioned, describes challenges that have not gone unnoticed to the EC countries and intergovernmental organizations like IRENA (International Renewable Energy Agency). In 2018, experts from Caribbean countries and multilateral organizations gathered in St. Lucia to look for some solutions to these problems in a roundtable discussion which was a bold step forward in the development of geothermal energy in the region (OECS, 2018). The discussion brought a better understanding of the problem and what the solutions to these problems could be. These solutions have to be integrated into a particular policy framework that can facilitate the implementation of the most feasible solution and improve on energy development. This overview dictates a policy framework to address the few ‘gaps’ in the development of geothermal energy in the EC and to ensure the sustainable supply of the resource.
Quality Information
Geothermal resource analyses of data and information do not guarantee exact predictions. Even after huge progress in assessing geothermal potential, it is difficult to predict well pressure and temperature, the exact depth of the reservoir or steam output from it, which are key variables to ensure adequate management and longevity of the resource (Malafeh and Sharp, 2015; ESMAP, 2012). Faced with this uncertainty, the least that policymakers can do is to make geothermal resource information available to potential developers. Information like seismic data, groundwater location and any information obtained from surface exploration technologies about well temperature, pressure or permeability should be public records for project developers to access as well as for technical experts to build a conceptual model of the geothermal system that allows a better understanding of the reservoir characteristics (ESMAP, 2012). The collection of information is not an activity that is completed when drilling starts on a potential site or investment secured. It should be a continuous process for effective resource management. Utilizing geothermal involves the extraction of heat energy from the reservoir and overexploitation can lead to the production of electricity or usable heat from the plant to be drastically reduced (Axelsson, 2003). Overexploitation can occur over poor monitoring of key variables from exploratory phases to drilling to complete operation which can lead to inaccurate prediction of long–term production.
Management of the Resource
Another cautionary note for newcomers of the geothermal industry like the EC countries is that more investors or project developers do not necessarily mean greater long–term development and synergistic benefits from the resource. For a geothermal system, there is a certain level of maximum energy below which constant production should be maintained to ensure the reservoir lasts for hundreds of years (termed as “sustainable production”). Production greater than the maximum exploitable limit leads to excessive production which will lead to exhaustion of the reservoir (Axelsson et al., 2005). Excessive production can occur when licenses are given to many project developers leading to resource usage without common management or control (Axelsson et al., 2005). Such a situation has already occurred in California with ‘The Geysers’. Twenty plants were built producing more than 2,000 MW (now over 7,000 MW) from the field and there was a drastic pressure drop from 1985 to 1995. From 1987 to 1999, production at The Geysers decreased by 59%, and reinjection has to be performed to stabilize the reservoir to only half its historical peak (Barker, 2000; ESMAP, 2012). The bottom–line as has been highlighted in an ESMAP report, is that geothermal developers and decision-makers have to adopt a more judicious approach in developing geothermal and ‘the limits of sustainability’ has to be respected.
Changes in the Regulatory and Legal Framework
For geothermal development to strive, there needs to be an adequate legal and regulatory framework. The central ‘legal’ debate for geothermal energy is around the ownership of the resource. It is clear from the above discussion that the resource has to be managed sustainably but a central question remains undiscussed-who to manage the resource and how? In many cases, the right of ownership of the geothermal resource lies with the government. In the case of New Zealand, the country went from a single owner system where the government-owned the resource to a system of management between private and public stakeholders (Malafeh and Sharp, 2015). It is difficult to say which system is adequate for EC countries due to the nascent area of research in geothermal energy policy. However, though state-owned, there are various ways the private sector can be involved which have been done in many countries and proven successful. In Iceland, the ownership of the resource is attached to the ownership of the land but drilling to tap geothermal energy is not allowed unless the developer has a license issued by the state (Ketilsson et al., 2010). The state can lease land (with the proven geothermal resource) for over 60 + years to interested private developers. Some countries like Indonesia and Turkey have implemented specific geothermal law over resource governance whereas others are incorporating provisions for geothermal in existing mining laws (Aksoy, 2014; Pambudi, 2018). At this stage, among the EC countries, Nevis and SVG are debating to pass regulations assigning ownership and rights to explore geothermal resource and, with these regulatory changes, a positive development to tap in the resource is being made (Gischler et al., 2017). Regulatory Incentives such as feed-in-tariff policies reduce investor risks through guaranteed price for a guaranteed period of time, often coupled with guaranteed grid access; yet are flexible and policymakers can adjust payment levels to accurately reflect technology costs.
Institutions
A regulator is recommended for the development of geothermal energy in the EC regions. Such recommendation was also made in a report from the Inter-American Development Bank (IDB) if geothermal activities are to be promoted (Gischler et al., 2017). In the EC, various activities in geothermal energy development involve adhering to regulations from different government agencies and ministries and often their actions are not coordinated leading to unnecessary delays in licensing and governmental approvals which can be a disincentive to a project developer. In this case, a few countries experiences show that the establishment of a dedicated national organization capable of managing large–scale projects can be useful. In Mexico, for example, with the establishment of Comisión de Energía Geotérmica (which was then taken over by the national utility), geothermal development grew from 37 MW to 950 MW placing Mexico third in geothermal power production, after the United States and The Philippines (ESMAP, 2012). In Indonesia, the state-owned Pertamina Geothermal Energy started operation by overseeing a 272 MW of geothermal capacity and by now the installed capacity of geothermal has already reached 1340 MW (ESMAP, 2012; Nasruddin et al., 2016). In the Philippines, the establishment of the Philippines National Oil Company Energy Development Corporation (PNOC EDC) took over drilling and exploration functions and eventually added some 700 MW of geothermal power to their grid (ESMAP, 2012). In the case of the EC, such an institutional structure is welcome and can promote growth in this sector. In a 2018 roundtable discussion on geothermal energy development in the Caribbean, setting up a ‘knowledge hub’ to facilitate knowledge sharing and expertize was pointed out which we believe is the right direction EC countries can take if implemented successfully (OECS, 2018).
Lastly, the island’s geothermal resources with central-generation capacity potential are largely located on public lands that are at the intersection of multiple agency jurisdictions. Agency coordination on environmental impact assessments is needed and should be coordinated between the ministries and units in charge of land use, energy, and the environment through regulatory permitting that includes public consultations.
Financing
There are several economic instruments provided to incentivize project developers to invest in the geothermal energy sector. Some popular ones are tax credits, subsidies, feed-in tariff schemes, investment grants or soft loans among others. A particular one is a PPP. There are major risks in the construction of a geothermal plant related to the resource, finance, regulatory, institutional capacity among others. A PPP will be effective as it will allow public stakeholders to concentrate on risky upstream stages with the public sector focusing on the capital investment and with drilling and construction operations. PPPs can help to increase the availability of funds for geothermal projects and allows partners to channel more private, public and multilateral resources together (Gischler et al., 2017). EC governments that lacked the skills and expertize will eventually benefit from a vast technical experience of the private sector in this case and overcome another one of their barriers. Developing countries like the Philippines and Mexico have established PPP to launch geothermal projects which have been very successful as of date. The establishment of a PPP between the government and developers also depends on how risk-averse the private sector is. More risk-averse project developers will not take responsibility for the exploration because of the uncertainty. These activities can be covered by the government leaving the investment and management responsibilities to the private sector. Long term or multiyear financing is needed for upfront cost defrayment to reduce risk and provide support until power production begins. These are not an exhaustive list of policy elements. However, policymakers can consider these policy items as the building block of geothermal energy policy in the SVG.
Conclusion
In this study we examined a resource assessment approach to the potential power outputs of the geothermal energy landscape associated with SVG. Plans for the integration of geothermal energy into the SVG energy mix and the national electricity grid has begun through the proposed 10 MWe power plant. Through SVG’s pending geothermal integration and expansion, our research has highlighted a three-front approach at a detailed RE analysis firstly at highlighting the positive economic development in the near-future (displacing an equivalent of 149,000 bbls of oil), and the progress as SVG transitions to a more climate-sensitive nation (displacing an estimated 0.172 million tCO2e/year). Secondly, a power potential analysis was performed using the volumetric method (through Monte Carlo simulations) which revealed the reservoir can sustain a minimum of 31, 34, and 92 MWe over the lifespan of 25–30 years for the SVG01, SVG02, and SVG03 wells respectively. There is evidence to support the viability of future expansions/developments of geothermal power plants in SVG, affording the nation an increase in valuable baseload power and greatly reducing its dependency on conventional fuels.
While we have been able to present a technical, resource and economic driven basis for future geothermal development in St. Vincent and the Grenadines, the national policy environment that could support and propel such development, is itself not wholly formed or clearly directed. In our assessment of the policy and regulatory environment, several suggestions can be made. These include clarifications around public-private partnerships and institutional arrangements which are being strengthened and may come to fruition in the near-future. Further policy priorities of urgency include regulatory processes such as those for operation licenses, strengthening finance mechanisms to open more investment in the market, and greater technical and information generation capacity to reduce risk and uncertainty among industry actors. Therefore, for a small island developing states such as St. Vincent and the Grenadines, the road toward energy security is within their grasp through the integration of renewable and geothermal energy in particular. Due to its significant base load energy generation to the electrical grid, geothermal energy can provide stable and reliable power.
Author Contributions
RKK: Lead on Section 2 – Overall synthesis of the paper across the Abstract, Sections 1 – Introduction, Section 2 - RE landscape (lead on this section), Section 3 - geothermal resource assessment, and Section 5 – Conclusion. Development/reconstruction of Figures in Sections 2 and 3. MA: Overall synthesis of the paper with more specific contributions to the Introduction and policy sections. Aided in the organization and finalizing of the paper. KS: Lead on section 4 – Policy.Infusion of policy aspects across paper including the Introduction and Conclusion. Overall synthesis and organization of the paper. SL: Contributed mainly to Section 3 - Geothermal Resource Assessment. Performed the data collection, generation of simulations and analyses using the Monte Carlo method.
Disclaimer
Frontiers Media SA remains neutral with regard to jurisdictional claims in published maps and institutional affiliations.
Conflict of Interest
The authors declare that the research was conducted in the absence of any commercial or financial relationships that could be construed as a potential conflict of interest.
Nomenclature
bbl barrels of oil
BESS Battery energy storage systems
CARICOM Caribbean community
CCREEE Caribbean center for renewable energy and energy efficiency
CO2 Carbon dioxide
EC Eastern caribbean
ERM Environmental resource management
ESMAP Energy sector management assistance program
GPP1 Geothermal power plant
IPCC Intergovernmental panel on climate change
IRENA International renewable energy agency
KPI Key performance indicators
MCS Monte carlo simulations
MW Mega-watts
MWe Mega-watts electric
NEAP National energy action plan
NPV Net present value
OECS Organization of eastern caribbean states
PNOC EDC Philippines national oil company energy development corporation
PPP Public-private partnership
RE Renewable energy
RoR Rate of return
SIDS Small island developing states
SVG 01 St. vincent geothermal well 1
SVG 02 - St. vincent geothermal well 2
SVG 03– St. vincent geothermal well 3
SVGP– St. vincent geothermal project
VINLEC– St. vincent electricity services limited
W1– Well 1
W3– Well three
GPP2 geothermal power potential
References
Aksoy, N. (2014). Power generation from geothermal resources in Turkey. Renew. Energ. 68, 595–601. doi:10.1016/j.renene.2014.02.049
Ashtine, M., Koon Koon, R., Grant, D., and Maharaj, A. (2018). Advancing the caribbean energy landscape – a comprehensive review of the state of electric vehicles and storage systems. Trinidad and Tobago, Caribbean: The Caribbean Academy of Sciences.
Axelsson, G. (2003). Essence of geothermal energy management. Reykjavík, Iceland: The United Nations University – Geothermal Training Programme.
Axelsson, G., Stefánsson, V., Björnsson, G., and Lio, J. (2005). “Sustainable management of geothermal resources and utilization for 100–300 years,” in Proceedings World geothermal congress, Antalya, Turkey, April 24–April 25, 2005.
Caribbean Development Bank (2016). St. Vincent and the grenadines receives funding for geothermal energy development. Available at: https://www.caribank.org/newsroom/news-and-events/st-vincent-and-grenadines-receives-funding-geothermal-energy-development (Accessed November 01, 2018).
CCREEE (2018). Energy report card - St. Vincent & the grenadines. Available at: https://ccreee.org/sites/default/files/documents/files/st.vincent.pdf (Accessed March 01, 2020).
Chen, A. A., Stephens, A. J., Koon Koon, R., Ashtine, M., and Mohammed-Koon Koon, K. (2020). Pathways to climate change mitigation and stable energy by 100% renewable for a small island: Jamaica as an example. Renew. Sust. Energ. Rev. 121, 109671.
De Freitas, M. A. (2016). Report No.: 12. Geothermal resource assessment of the Wotten Waven geothermal field – Dominica, West Indies.
Energy Chamber (2017). Understanding the electricity subsidy in T&T. Available at: https://energynow.tt/blog/understanding-the-electricity-subsidy-in-tt (Accessed February 02, 2018).
ERM (2016). St. Vincent geothermal project phase I exploratory drilling environmental and social impact assessment (ESIA) draft report.
ESMAP (2012). Technical Report 002/12. Geothermal energy: planning and financing power generation. Energy sector management assistance program.
Gay, D., Rogers, T., and Shirley, R. (2018). Small island developing states and their suitability for electric vehicles and vehicle-to-grid services. Utilities Policy 55, 69–78.
Gischler, C., Janson, N., Gonzalez, C., Cordoba, M. J., and Santana, S. (2017). Unlocking geothermal power. Washington, DC: Inter-America Development Bank.
Hartlieb, P., Toifl, M., Kuchar, F., Meisels, R., and Antretter, T. (2016). Thermo-physical properties of selected hard rocks and their relation to microwave-assisted comminution. Minerals Eng. 91, 34–41. Available at: https://www.sciencedirect.com/science/article/pii/S0892687515301278.
Hsieh, I. Y. L., Pan, M. S., Chiang, Y. M., and Green, W. H. (2019). Learning only buys you so much: practical limits on battery price reduction. Appl. Energ. 239, 218–224.
Huttrer, G. W. (2010). “Country update for eastern Caribbean island nations,” in Proceedings of the World Geothermal Congress. Bali, Indonesia, April 25–30, 2010.
Ketilsson, J., Olafsson, L., Steinsdottir, G., and Johanneson, G. A. (2010). Legal framework and national policy for geothermal development in Iceland. Proceedings World geothermal congress 25 1–6.
Klotzbach, P. J., Schreck, C. J., Collins, J. M., Bell, M. M., Blake, E. S., and Roache, D. (2018). The extremely active 2017 North Atlantic hurricane season. Monthly Weather Rev. 146 (10), 3425–3443.
Lund, J. W. (2018). Direct utilization of geothermal resources world wide role of royalties in sustainable geothermal energy development. Energy Policy 85, 235–242. Available at: https://www.researchgate.net/publication/324576296_Direct_Heat_Utilization_of_Geothermal_Resources_Worldwide.
Malafeh, S., and Sharp, B. (2015). Role of royalties in sustainable geothermal energy development. Energy Pol. 85, 235–242.
Muffler, L. P. J. Editor (1979). Assessment of geothermal resources of the United States – 1978. SArlington, VA: U.S. Geological Survey Circular 790.
Nasruddin, A. M. A., Daud, Y., Surachman, A., Sugiyono, A., Aditya, H. B., and Mahlia, T. M. I. (2016). Potential of geothermal energy for electricity generation in Indonesia: a review. Renew. Sust. Energ. Rev. 53, 722–740.
Ochs, A., M., Konold, K., Auth, E. M., and Killen, P. (2015). Caribbean sustainable energy roadmap and strategy (C-SERMS): baseline report & assessment. Washington, DC: World Watch Institute.
Ofwona, C. (2008). “Geothermal resource assessment- case example, Olkaria I,” in Proceedings of the Short Course III on exploration for geothermal resources, Kenya, October 24–November 17, 2008.
Pambudi, N. A. (2018). Geothermal power generation in Indonesia, a country within the ring of fire: current status, future development and policy. Renew. Sust. Energ. Rev. 81, 2893–2901.
Popke, J., and Harrison, C. (2018). Energy, resilience, and responsibility in post-Hurricane Maria Dominica: ethical and historical perspectives on ‘building back better’. J. Extreme Events 5 (04), 1840003.
Reykjavik Geothermal (2013). Report No. 13002-01. A geothermal desk study for Mt. Soufrière. St. Vincent.
Richter, A. (2019). Drilling of second well with positive results at St. Vincent geothermal project. Available at: http://www.thinkgeoenergy.com/drilling-of-second-well-with-positive-results-at-st-vincent-geothermal-project/ (Accessed December 13, 2019).
Richter, A. (2020). Geothermal project in St. Vincent and grenadines facing challenges with drilled wells. Available at: https://www.thinkgeoenergy.com/geothermal-project-in-st-vincent-grenadines-facing-challenges-with-drilled-wells/ (Accessed January 01, 2020).
Samiento, Z. F., and Bjornsson, G. (2007). Geothermal resource assessment - volumetric reserves estimation and numerical modeling, in Short Course on Geothermal Development in Central America - Resource Assessment and Environmental Management. San Tecla, El Salvador: UNU-GTP and La Geo.
Sarmiento, Z. F., and Steingrimsson, B. (2011). “Resource assessment I: introduction and volumetric assessment”. Short course on geothermal drilling, resource development and power plants. El Salvador: UNU-GTP and La Geo.
Shah, K. U., and Niles, K. (2016). Energy policy in the Caribbean green economy context and the Institutional Analysis and Design (IAD) framework as a proposed tool for its development. Energy Policy 98, 768–777.
Shah, K. U. (2018). Regulatory impact assessment for implementing energy efficient lighting standards in the small island developing state of Antigua & Barbuda. Energ. Strategy Rev. 22, 216–229.
Shah, M., Vaidya, D., and Sircar, A. (2018). Using Monte Carlo Simulation to estimate geothermal resources in Dholera geothermal field, Gujarat, India. in Multiscale and Multidisciplinary Modeling Experiments and Design, Berlin, Germany: Springer.
Society of Petroleum Engineering (2020). Unit conversion factors. Available at: https://www.spe.org/industry/unit-conversion-factors.php (Accessed March 10, 2020).
Taylor, M. A., Clarke, L. A., Centella, A., Bezanilla, A., Stephenson, T. S., Jones, J. J., et al. (2018). Future Caribbean climates in a world of rising temperatures: the 1.5 vs 2.0 dilemma. J. Clim. 31 (7), 2907–2926.
Taylor, M. A., Stephenson, T. S., Chen, A. A., and Stephenson, K. A. (2012). Climate change and the caribbean: review and response. Caribbean Studies 40 (2), 169–200. doi:10.2307/41917607
The World Bank (2021). CO2 emissions (metric tons per capita). Available at: https://data.worldbank.org/indicator/EN.ATM.CO2E.PC?end=2016&start=1960&view=chart (Accessed January 13, 2021).
Timilsina, G. R., and Shah, K. U. (2016). Filling the gaps: policy supports and interventions for scaling up renewable energy development in Small Island Developing States. Energy Policy 98, 653–662.
United States EIA (2019). Independent statistics & analysis - electric power monthly. Available at: https://www.eia.gov/electricity/monthly/epm_table_grapher.php?t=epmt_6_07_b (Accessed March 10, 2019).
Wohletz, K., and Heiken, G. (1992). Volcanology and geothermal energy. Available at: https://publishing.cdlib.org/ucpressebooks/view?docId=ft6v19p151&chunk.id=d0e17866&toc.id=&brand=ucpress (Assessed November 7, 2019).
Keywords: St. Vincent, geothermal energy, resource assessment, economic benefits, energy policy, climate sensitivity
Citation: Koon Koon R, Shah K, Ashtine M and Lewis S (2021) A Resource and Policy Driven Assessment of the Geothermal Energy Potential Across the Islands of St. Vincent and the Grenadines. Front. Energy Res. 9:546367. doi: 10.3389/fenrg.2021.546367
Received: 28 March 2020; Accepted: 02 February 2021;
Published: 22 April 2021.
Edited by:
Davide Astiaso Garcia, Sapienza University of Rome, ItalyReviewed by:
Behnam Mohammadi-ivatloo, University of Tabriz, IranAnıl Erdoğan, Dokuz Eylül University, Turkey
Murat Ünverdi, Adnan Menderes University, Turkey
Copyright © 2021 Koon Koon, Shah, Ashtine and Lewis. This is an open-access article distributed under the terms of the Creative Commons Attribution License (CC BY). The use, distribution or reproduction in other forums is permitted, provided the original author(s) and the copyright owner(s) are credited and that the original publication in this journal is cited, in accordance with accepted academic practice. No use, distribution or reproduction is permitted which does not comply with these terms.
*Correspondence: Kalim Shah, 1kalshah1@gmail.com