- 1School of Interdisciplinary and Transdisciplinary Studies, Indira Gandhi National Open University, New Delhi, India
- 2Department of Biochemistry, College of Medicine, Imam Abdulrahman Bin Faisal University, Dammam, Saudi Arabia
- 3Department of Botany, School of Life Sciences, Mahatma Gandhi Central University, Motihari, India
The crop residue burning in India particularly North-western India is responsible for air pollution episodes and public health concerns; greenhouse gases emissions and radiation imbalance; and declining soil organic matter and soil productivity. The objectives of this paper are to estimate the crop residue burning and emissions from crop residue burning, to recommend interventions in crop residue management and to propose a crop residue management-bioeconomy model incorporating strategies to sustainably manage the crop residues through interventions that enable waste valorization, food and nutritional security, farmers’ livelihood and sustainable agricultural production system. A national inventory on crop residue burning including the pollutant species was prepared using the IPCC methodology. The crop types included for the estimation are cereals, pulses, oilseeds, sugarcane, cotton, jute and Mesta. The total amount of crop residues generated and burned for the year 2017–18 was estimated at 516 million tonnes and 116 million tonnes respectively. It is estimated that 116.3 Tg of crop residues burning released about 176.1 Tg of CO2, 10 Tg of CO, 313.9 Gg of CH4, 8.14 Gg of N2O, 151.14 Gg of NH3, 813.8 Gg of NMVOC, 453.4 Gg of PM2.5, and 935.9 Gg of PM10. The emission estimates can be a proxy to prepare the national level inventory of air pollutant species from crop residue burning. The crop residue management (CRM) demands a transition from the traditional zone of CRM to bioeconomy zone of CRM, wherein the interventions aim at the sustainability of agroecosystem. The proposed bioeconomy model has a four-pronged strategy that includes smart agriculture practices, waste bioeconomy involving aspirational principles of bioeconomy, capacity building of stakeholders’ and proactive government policy. Sustainable agricultural bioeconomy provides ample opportunities to reduce crop residue burning, increase farmers’ livelihood and decarbonize the agricultural production. India’s efforts and policies can provide lessons for other agricultural regions having similar environmental constraints.
Introduction
Agricultural crop residue burning had become an annual feature in the densely populated agricultural regions of India (Shyamsundar et al., 2019), China (Chen et al., 2017) and Southeast Asia (Kim Oanh et al., 2018). Persistent demand to increase agricultural productivity have impelled the farmers to adopt practices, which quite often, cause ecological imbalance to agroecosystem. More so in North-western India, where crop residue burning is endemic. North-western India, known for 20th century Green Revolution (Erenstein, 2011), is popularly called as “breadbasket” of India as it accounts for two-thirds of food grains (Jethva et al., 2019; Chawala and Sandhu, 2020). Crop residue burning continues despite the implementation of “National policy for management of crop residues” (NPMCR, 2014), regulations by state governments and incentives that promote waste valorization and crop residue management. Assured income support from the government through “Minimum Support Price” for rice and subsidised power to access the irrigation system had driven the farmers to adopt the resource-intensive rice-wheat cropping system (Bhargava, 2018). Farmers were able to sow paddy crop well before the onset of monsoon, using the groundwater. Nevertheless, intensive rice cropping depleted groundwater resources (Famiglietti, 2014). The “Sub-Soil Water Act, 2009” was passed by the governments of Punjab and Haryana to arrest the further decline of groundwater. As per the Act, the sowing of the irrigated rice crop was delayed to sync the rice crop growth period with monsoon season (Sembhi et al., 2020). Nevertheless, it resulted in short turn-around time between the rice (Kharif season) and wheat (Rabi season) crop, which calls for use of combine harvesters (Lohan et al., 2018; NABARD, 2018; Ravindra et al., 2019) for mechanical harvesting of rice crop. Presently about 75% of the rice crop is harvested using mechanical harvesters (Vadrevu et al., 2011). The crop stubbles that are left on the cropland after mechanical harvesting pose striking challenges to the farmers on account of its height of the stubbles, nutritional composition and quality, cost involved in collection and transportation, and lack of appropriate on-site residue management technologies. Farmers consider open stubble burning as the easiest and most economic option of removal of crop stubbles (Chawala and Sandhu, 2020). The drivers to crop residue burning are an increase in the amount of crop residues due to increase in crop yield (Ravindra et al., 2019), labour scarcity (Lohan et al., 2018), the short time interval between the harvesting of monsoon (Kharif) crop and sowing of winter (Rabi) crop, absence of appropriate crop residue management technology (Shyamsundar et al., 2019), nutritionally poor rice crop residues (Singh and Sidhu, 2014), economic resource constraints, social influence (Lopes et al., 2020) and lack of awareness about the public health issues due to crop residue burning (Kim Oanh et al., 2018; Chawala and Sandhu, 2020). The intensity of open field crop residue burning is determined by the biophysical conditions, agricultural input-output and crop types (Fang et al., 2020). Crop residue burning releases air and short-lived climate pollutants, aerosols, particulate matter, soot particles (Jain et al., 2014; Jethva et al., 2019; Li et al., 2020; Chawala and Sandhu, 2020), benzene, isocyanic acid (Chandra and Sinha, 2016), polycyclic aromatic hydrocarbons (Tipayarom and Kim Oanh, 2020) into the atmosphere, perturbing the atmospheric chemistry and radiation balance, and driving the formation of Asian Brown Clouds (Ramanathan and Carmichael, 2008). Scientific curiosity in crop residue burning grew due to the emission of air pollutants, long-range transport of the air pollutants (Andreae and Merlet, 2001) and deteriorating air quality (Nair et al., 2020), loss of valuable soil nutrients (Jain et al., 2014; Jat et al., 2020), human health and well-being (Sarkar et al., 2018; Chakrabarti et al., 2019), greenhouse gas mitigation (Smith et al., 2007), urge to decarbonize agricultural production, waste valorization and circular bioeconomy (Venkatramanan et al., 2021). Figure 1 depicts the impacts of crop residue burning (CRB) on the atmospheric, soil and human environment. The impacts of CRB necessitates a nexus thinking (Bhuvaneshwari et al., 2019) and integrated approach (Ravindra et al., 2019) towards crop residue management (CRM). Crop residue management involves conservation agriculture, utilization of crop residues for biomass energy production, lignocellulosic crop residue composting, mushroom production and in-situ straw incorporation (Dey et al., 2020; Goswami et al., 2020; Meng et al., 2020; Singh et al., 2020).
Conservation agriculture enables resource and energy-efficient agriculture crop production (Jat, et al., 2020) using integrated management of agroecosystem (Reicosky, 2015) and conservation of biological resources. The conservation agriculture practice in the rice-wheat cropping system includes zero-till wheat cropping (Singh and Sindhu 2014). Maintenance of soil cover with residue and also sowing of wheat seeds in the presence of rice stubbles were possible only with Happy Seeder (Shyamsundar et al., 2019). Straw incorporation into the soil is a preferred intervention as it enables recycling of soil nutrients and improvement of soil ecosystem through the enrichment of soil biota (Chivenge et al., 2019). The nutrient concentration in the cereal straw is a function of crop variety, crop management practices and soil environment. The physical properties of soil that are improved due to in-situ incorporation of straw include infiltration rate, water holding capacity, bulk density, cation exchange capacity and soil structure (Lohan et al., 2018). The availability of soil organic carbon improves the microbial growth and mobilization of nutrients. In the rice-wheat cropping system of North-western India, application of rice residues was found to be beneficial on wheat yields (Gupta et al., 2007).
Biomass energy production is an appropriate intervention in crop residue management (Jiang et al., 2012; Hiloidhari et al., 2014) due to 1) significant amount of crop residue generation per annum, 2) crop residue generation is projected to increase due to increasing food demand, and adoption of high yielding varieties and farm mechanization, 3) spatio-temporal variation in the crop residue generation can be harnessed to decentralise the energy production and achieve energy security, 4) installed capacity of 9800 MW (500 biomass power plants and bagasse cogeneration) of projects across the country reiterates the renewable energy trajectory and pledge to mitigate the GHG from the energy sector, 5) bioenergy sector is closely linked with employment generation and well-being of the marginalised sections of the farming community. The biopower can be obtained from the crop residue biomass either through burning, bacterial action or liquid fuel. Farmers preferences were observed for crop residue-based power plants (Kaur, 2020).
The lignocellulosic crop residues have huge potential to be used as feedstock for biofuel production (Prasad et al., 2020; Venkatramanan et al., 2021). The performance of microorganisms involved in the biofuel production from crop residues can be augmented by adopting metabolic engineering and synthetic biology approaches (Shah and Venkatramanan, 2019). However, the use of crop residues for biofuel production decreases soil organic carbon and increases CO2 emissions (Lal and Pimentel, 2007; Liska et al., 2014). The quantity of crop residues that can be exported to off-farm use depends on the soil erodibility, soil fertility status, climatic conditions and cultivation practices. Crop residues particularly paddy straw was hitherto not preferred for biogas production because of its lignocellulose content, low digestibility and low biogas yield. Nevertheless, to improve the biomethanation of paddy straw, Ngan et al. (2019) recommended 1) pre-treatment of paddy straw, which include “physical methods (particle size reduction), chemical methods (acid and alkali additions), and biological methods (fungi)”, 2) co-digestion of paddy straw with animal manure (Ngan et al., 2019). The pre-treatment of paddy straw enables break-down of tight linkages between cellulose, hemicellulose and lignin, and increases the biodegradability of the paddy straw. Cherubini and Ulgiati (2010) opined that a biorefinery approach to use crop residues can aid in sustainable utilization of lignocellulosic crop residues and also climate change mitigation. Biochar production from the pyrolysis of crop residues has potential application in the agricultural production system. It renders significant ecosystem services that include nutrient retention and cycling of soil nutrients, improvement in soil quality and soil health, adsorbent, soil ameliorant (Singh et al., 2015), carbon sequestration and climate change mitigation (Singh and Sidhu, 2014; Windeatt et al., 2014; Singh et al., 2015). Application of crop residue biochar can recycle the valuable major and micronutrients as well as silicon (Li et al., 2019). However, the biochar production and field-scale biochar application are challenged due to costly production process (Joseph et al., 2013). Zhou et al. (2018) developed an in-situ technique “burn and soil cover”, which uses crop residues and can be adopted at the farm level. Nevertheless, the export of crop residues from the farm to off-site for bioenergy generation must be examined critically. It is reported that the excessive removal of crop residues may be harmful to both the crop yields and soil environment (Lal, 2005; Jiang et al., 2012; Hiloidhari et al., 2014).
The objectives of this paper are to estimate the crop residue burning, to estimate emissions from crop residue burning, to recommend interventions in crop residue management and to propose a crop residue management-Bioeconomy model towards the management of crop residues.
Materials and Methods
Crop statistics of India were obtained from the ‘Agricultural Statistics at a glance 2018’ and ‘Economic Survey 2019–2020’ (Government of India, 2018; Economic Survey, 2020). The crop production data are compiled by the Directorate of Economics and Statistics, Ministry of Agriculture and Farmers Welfare, Government of India. The Ministry of Agriculture and Farmers Welfare has adopted a robust procedure to derive a relatively precise estimation of crop production data.
Selection of Crops
The crops included for the estimation of crop residue burning are rice, wheat, coarse cereals, pulses, oilseeds, sugarcane, cotton, jute and Mesta. The coarse cereal crops include maize, sorghum, ragi, bajra, small millets and barley. Data on pulse crops include red gram, black gram, green gram, gram, lentil and other pulses. The oilseed crops include groundnut, rapeseed and mustard, sesamum, linseed, castor seed, niger seed, safflower, sunflower and soybean. The crops chosen for this study were based on India-specific studies (Jain et al., 2014; NPMCR, 2014; Ravindra et al., 2019; Shyamsundar et al., 2019).
Estimation of Crop Residue Burning
Crop residue burning was calculated based on total crop production using the following equation (IPCC, 1996; Streets et al., 2003).
where, R = Total mass of crop residues burned in the field; P = Crop production; N = Production-to-residue ratio (crop-specific); D = Dry matter fraction; B = Fraction of dry matter residues burned in the field; and F = Burn efficiency ratio (crop-specific).
The crop residue burning estimates were calculated from 1950–51 to 2017–18 to understand the dynamics of crop residue generation. The crop production data were obtained from the ‘Agricultural Statistics at a glance 2018’ and ‘Economic Survey 2019–2020’. The crop-specific ‘residue to crop ratio’ and ‘dry matter fraction’ were obtained from the works of Jain et al. (2014), Streets et al. (2003) and Ravindra et al. (2019). The fraction of dry matter residues burned in the field was taken as 0.25, as in the developing countries, about 25% of total agriculture residues are burned in-situ (IPCC, 1996).
The value for crop-specific burn efficiency was taken as 0.9 (default value) from the revised 1996 IPCC guidelines for national GHG inventories. The coefficients used in the estimation of crop residue burning are shown in Table 1.
Estimation of Total Carbon and Total Nitrogen Released From CRB
The total carbon released (Gg) from CRB is calculated by multiplying the total biomass burned by the crop-specific carbon fraction (IPCC 1996). The total nitrogen released (Gg) from CRB is calculated by multiplying the total carbon released from crop residue burning by the Nitrogen-Carbon ratio (IPCC 1996).
Estimation of Pollutant Emissions From CRB
The atmospheric emissions of significant pollutant species were calculated by multiplying the mass of total dry matter burned on-site by an emission factor using the following equation (Streets et al., 2003):
where E = Total emissions from crop residue burning; M = Mass of total dry matter burned in-situ; and F = Emission factor.
The emission factor for any pollutant species is expressed as grams of released pollutant per kilogram of dry matter of crop residues burned in the field. The emission factors for the pollutant species [CO2, CO, CH4, BC, OC, N2O, Nitrogen Oxides (NO + NO2), NH3, SO2, Non-methane Volatile Organic Compounds, PM2.5 and PM10] were provided by Streets et al. (2003), IPCC (2006) and Kanabkaew and Oanh (2011). In the absence of emission factors for specific crop types, the emission factors for combined crops (general crop residues) were used (Kanabkaew and Oanh, 2011).
Results
Crop Residue Generation
The total amount of crop residues (dry matter) generated was increased from 79 million tonnes (1950–51) to 516 million tonnes (2017–18) (Table 2). The substantial increase in crop residue generation can be attributed to the increase in the net sown area and cropping intensity. The net sown area increased from 118.75 to 140 mha between 1950–51 and 1970–71. In the subsequent period, there is no significant change in the net sown area. It cannot be overemphasized that cropping intensity increased from 111.07% to 139.56% between 1950-51 and 2010–11. The increase in cropping intensity and total cropped area coupled with technological advancements were responsible for the increase in total food grain production from 50 million tonnes (1950–51) to 285 million tonnes (2017–18) and also the crop residue generation. In the present study, we estimated that the cereals generate about 334 million tonnes of crop residues (2017–18), followed by sugarcane (133 million tonnes) and fibre crops (17.7 million tonnes) (Table 2). Within the cereal crops’ category, while the rice crop contributes about 145.5 million tonnes, wheat crop contributes 149 million tonnes.
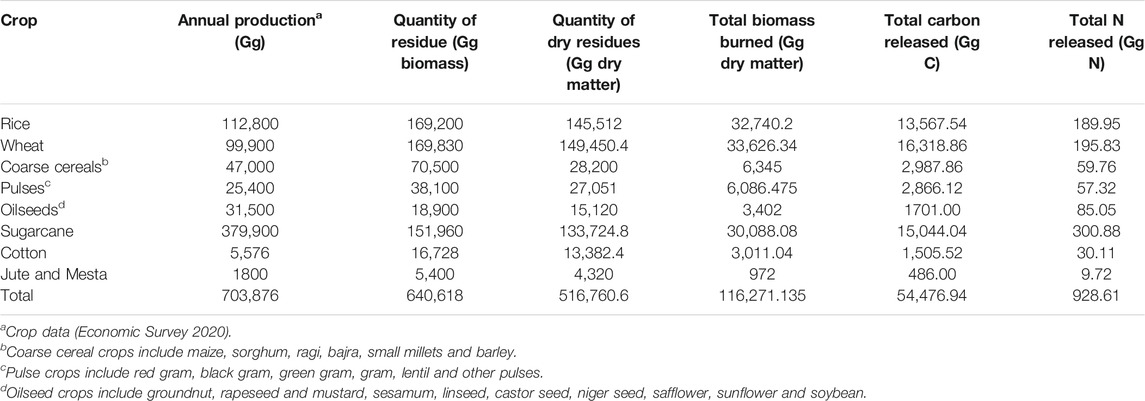
TABLE 2. Crop-wise production, crop residue generated, total carbon and nitrogen released in India (2017–18).
Crop Residue Burning
Figure 2 shows the increase in crop residue burning from 1950–51 to 2017–18. The crop residue burning in terms of total biomass burned increased from 18 million tonnes to 116 million tonnes between 1950–51 and 2017–18. The average decadal growth rate of crop residue burning between 1950–51 and 2010–11 was 32%. Granular analysis of crop residue burning data reveals that the increase in the crop residue burning between 1980–81 and 1990–91 was 45%. The trend in crop residue burning since 1990–91 and contribution of crops (type) to total biomass burned shows that the residues from the crops-rice, wheat and sugarcane account for about 80% of crop residue burning (Figure 3).
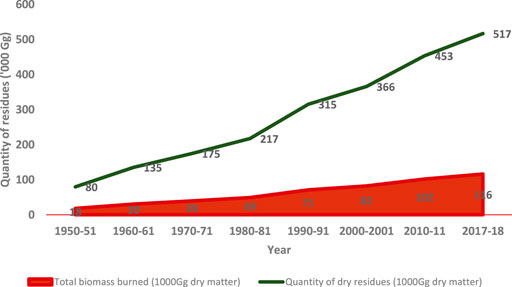
FIGURE 2. Crop residues generation and crop residue burning in India between 1950–51 and 2017–18. Source: Crop statistics of India were obtained from the ‘Agricultural Statistics at a glance 2018’ and ‘Economic Survey.019–-2020’ (Government of India, 2018; Economic Survey, 2020).
Emissions From Crop Residue Burning
The crop residue burning releases pollutant species including the non-CO2 emissions such as CH4, NOx and N2O. It is estimated that while the total amount of crop residues burned increased from 17.9 million tonnes (1950–51) to 116.3 million tonnes (2017–18), the total carbon released increased from 8,295 Gg·C to 54,476.9 Gg (Table 2). On the other hand, during the same period, the total nitrogen released increased from 151 Gg·N to 928.61 Gg·N (Table 2). In the present study, it is estimated that for the year 2017–18, about 116.3 Tg of CRB released about 176.1 Tg of CO2, 313.9 Gg of CH4 and 8.14 Gg of N2O (Table 3). The carbon dioxide emissions from crop residue burning are not reported as part of GHG inventory from the crop residue burning since the ‘carbon released from the burning process is assumed to be reabsorbed by the vegetation during the next growing season’ (IPCC, 2006). Rice, wheat and sugarcane crop residues account for a significant amount of methane and nitrous oxide emissions. The total amount of GHG emissions from crop residue burning (CO2 equivalent), which include only CH4 and N2O emissions, as per the IPCC (2006) guidelines, increased from 3,835.2 Gg·CO2 equivalent/year to 9,114.8 Gg CO2 equivalent/year (Figure 4).
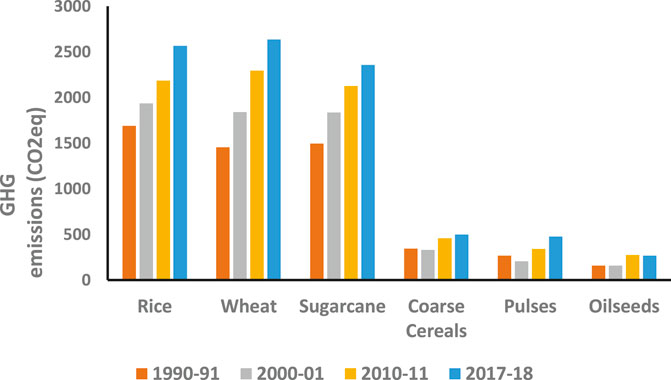
FIGURE 4. Total amount of GHG emissions from crop residue burning (CO2 equivalent). Note: GHG emissions from crop residue burning include only CH4 and N2O emissions, as per the IPCC (2006) guidelines.
The particulates PM2.5 and PM10 released from crop residue burning during 2017–18 were 453 and 935.8 Gg (Table 3), while for the year 1980–81, it was 190.7 and 393.8 Gg respectively. The particulate emissions increased by 137% between 1980–81 and 2017–18. Trace gases emissions from crop residue burning estimated in the present study include 151.1 Gg NH3, 46.5 Gg SO2 and 813.8 Gg NMVOC.
Discussion
The phenomenal growth in Indian agriculture in terms of total foodgrains production between 1950–51and 2017–18 (Economic Survey, 2020) was due to the development of high yielding varieties and hybrids, increase in cropping intensity, increase in irrigated area, lab to land programmes, farm mechanization and technology transfer. Nevertheless, agricultural growth has caused enormous pressure on environmental health. Prominent among them is the crop residue burning. In the present study, the total amount of crop residue generated was estimated to be 516 mt (2017–18) which is nearly five times more than the amount of crop residues generated in 1950–51. Estimates by Jain et al. (2014) and Ravindra et al. (2019) were 620 million tonnes and 487 million tonnes respectively. The differences in the estimates are due to the coefficients used in the estimation, and crop types were chosen for analysis. The substantial increase in crop residue generation is attributed to the increase in cropping intensity (Government of India, 2018; Economic Survey, 2020) and farm mechanisation (Lohan et al., 2018; NABARD, 2018). NABARD (2018) reported that “the average farm power available for the cultivated area of India has been increased from 0.25 kW/ha in 1951–52 to 2.02 kW/ha in 2016–17”. The sale of tractors also increased from 80,164 (1986–87) to 580,000 (2016–17). The use of farm power in agricultural operations range from 40 to 45%. In crops like wheat and rice, nearly 60–70% of harvesting and threshing operations are mechanized. Both rice and wheat account for nearly 60% of crop residue generation.
The crop residue burning episodes increased gradually since 1950–51. Nevertheless, the conspicuous increase in crop residue burning between 1980–81 and 1990–91 is attributed to an increase in cropping intensity during the same period. The observations also reveal that the farmers to increase agricultural productivity, endeavoured to cultivate the land more than once in a year. The need for agricultural intensification in the backdrop of rising labour scarcity for manual harvesting (Lohan et al., 2018) and constant demand for farm mechanization paved way for energy-intensive farm practices that include but not limited to the use of combine harvesters. The percentage share of agricultural workers in the total workers in India has been reduced from 69.7% to 54.6% from 1951 to 2011 (Government of India, 2018). The wage rates for the farmworkers range from Rupees 214 to Rupees 312 per day based on the skill of the workers (Government of India, 2018). The wage rates increase steeply during the harvesting season (Lohan et al., 2018). Mechanical harvesting using combine harvesters was extensively adopted in the rice-growing areas to hasten the harvesting process. The number of combine harvesters increased from 5,000 to 13,800 between 1990–91 and 2012–13 (Lohan et al., 2018). Vadrevu et al. (2011) reported that about 75% of rice harvesting was done by mechanical harvesting rather than manual harvesting. Use of combine harvesters generates an enormous amount of ‘root-bound and loose crop residues’ which are difficult to manage (Ravindra et al., 2019). It is reported that due to very short time interval (10–20 days), labour scarcity, unavailability of farm implements to plough back the stubbles into the soil or spread the stubbles as mulch, the farmers’ resort to on-site burning of crop residues (Shyamsundar et al., 2019; Kaur, 2020) (Figure 5). Absence of appropriate crop residue management technology and lack of awareness about the downside of crop residue burning also drive the farmers to stubble burning (Chawala and Sandhu, 2020). Further, paddy straw due to poor digestibility and low nutritive value are less preferred as ruminant feed. Lohan et al. (2018) reported that the lack of storage facilities and market opportunities also drive the farmers to burn crop residues. It is also believed by the farmers’ that on-site burning of crop residues control problematic weeds, pests and disease-causing organisms. CRB is much sought after by the farmers’ as it aids in quick disposal of the crop residues paving way for the sowing of the subsequent crop. The comparative estimates of crop residue burning in India are shown in Table 4. Crop residue burning strongly differs from other biomass burning on account of its causes, the timing of the fire event and location as well. Crop residue burning activity is influenced by the agricultural practices that include crop cycle, crop type, harvesting season, the potential use of residues, agricultural mechanization, the feasibility of on-farm residue collection and transportation and profitability of alternate options.
The crop residue burning releases pollutant species including CO2, CH4, N2O, particulates and trace gases. It is estimated that crop residue burning releases a significant amount of carbon and nitrogen into the atmosphere. Our estimates are in line with the reports of Jain et al. (2014) and Ravindra et al. (2019). Nitrogenous fertilizers and carbon-based inputs were used extensively in India since the 1960s along with high yielding rice and wheat varieties (Erenstein, 2011). Maheswarappa et al. (2011) reported that the carbon-based input to the Indian agriculture increased from 69.7 Tg·C equivalent in 1960–61 to 281.2 Tg·Ceq/yr by 2008–09 due to extensive use of external carbon-intensive inputs. In our study, it is estimated that the total carbon released from residue burning increased from 14 Tg·C to 47.9 Tg·C between 1960–61 and 2010–11. The quantity of carbon released from crop residue burning, though a function of crop type, is influenced greatly by the number of external inputs added and crop management practices. Further, the carbon sustainability index which is the function of input use decreased since 1960–61 due to the excessive use of agricultural inputs (Maheswarappa et al., 2011). In the present study, it is estimated that the particulate emissions from crop residue burning increased by 137% between 1980–81 and 2017–18. Jethva et al. (2019) reported that ‘highest number of fire count detection and record-high PM2.5 load was observed in the CRB season of 2016–17. While estimates by Jain et al. (2014) was 384.18 Gg (PM2.5), Ravindra et al. (2019) estimated about 823.36 Gg (PM2.5). The variations in the estimates are mainly due to the emission coefficients used for the estimation of the particulate matter. It must be emphasized that particulate emissions from crop residue burning have increased due to the widespread burning of crop residues in North-western India and consequent health impacts were observed in Delhi and surrounding regions during the post-monsoon season (Awasthi et al., 2011; Bhuvaneshwari et al., 2019; Shyamsundar et al., 2019). Guttikunda and Goel (2013) observed that the particulate emission load is responsible for 7,350–16,200 premature deaths and 6.0 million asthma attack/year in Delhi and National Capital Region. Further, the particulates and gaseous emissions are the main cause of haze and smog formation during the post-monsoon season (Zhang et al., 2016; Bhuvaneshwari et al., 2019; Shyamsundar et al., 2019).
Crop residue burning plays an important role in the atmospheric carbon balance as a source of greenhouse gases (GHGs). The present attempt to estimate crop residue generation, burning and emissions of significant pollutant species, though adopted robust methodology and guidelines are constrained by few limitations. The emissions from crop residue burning show geographical differences, due to “spatially differentiated activity” and “geographically different emission coefficients” (Charkovska et al., 2019). Uncertainties in crop residue burning emissions can be attributed to a lack of knowledge about crop statistics and actual value of pollutant emissions. Nevertheless, attempts to limit uncertainties are needed to develop a comprehensive national policy on crop residue management. The uncertainty of crop production data depends primarily on the reliability of the crop production estimates. We obtained the crop production data from the ‘Agricultural Statistics at a glance 2018’ and ‘Economic Survey 2019–2020’ (Government of India, 2018; Economic Survey, 2020). The crop production data compiled by the Directorate of Economics and Statistics, Ministry of Agriculture and Farmers Welfare, Government of India were used to estimate the crop residue generation. Previous studies conducted on crop residue burning by Jain et al. (2014), Lohan et al. (2018), Jethva et al. (2019) and Ravindra et al. (2019) were also based on the crop production data from the Ministry of Agriculture and Farmers Welfare, Government of India. It must be noted that the Ministry of Agriculture and Farmers Welfare has adopted a robust procedure to derive a relatively precise estimation of crop production data (Hiloidhari et al., 2014). The crops included for the estimation of crop residue burning are not exhaustive. However, the study included major crops based on India-specific studies (Jain et al., 2014; NPMCR, 2014; Ravindra et al., 2019; Shyamsundar et al., 2019). The crops included for our study are rice, wheat, coarse cereals, pulses, oilseeds, sugarcane, cotton, jute and Mesta. By adopting the revised 1996 IPCC guidelines for National GHG inventory, we have estimated the total crop residue generated for the year 2017–18 to be 516 million tonnes. Other studies have also estimated the total amount of crop residues generated between 500 million tonnes and 620 million tonnes/year (Jain et al., 2014; Ravindra et al., 2019). The variability in the estimates of crop residue generation arise due to the use of different crop types and crop combination and also different grain/straw ratios. The crop-specific ‘residue to crop ratio’ is a significant input to estimate crop residue burning. Care was taken to obtain the values for the ‘residue to crop ratio’ from the reputed and time-tested scientific study (Streets et al., 2003; Jain et al., 2014; Ravindra et al., 2019). The amount of crop residues burnt in-situ varies widely with crop type and region. For instance, the fraction of rice crop residues burned in-situ varies between 8 and 80% across the states of India. Similar is the case with the wheat crop (10–23%), sugarcane (25%) and other crops (10%). To overcome the limitation of spatio-temporal variations, the IPCC default value of 0.25 was taken as the fraction of dry matter residues burned in the field (IPCC, 1996). Spatial-temporal variation of the crop residue burning demands the use of satellite observations, monitoring using an artificial intelligence-enabled unmanned aerial vehicle, combined with farmers’ survey to reduce the uncertainty involved in the national inventory of GHG emissions from crop residue burning. Nevertheless, the satellite observation of crop residue burning activity is constrained due to “small, short-lasting agricultural fires”, “limited overpasses” and hazy meteorological conditions. The satellite observations can be supplemented with the household survey to improve the estimation of crop residue burning (Liu et al., 2020). For the estimation of total carbon and total nitrogen released from agriculture, many models have been developed (Dimitrov and Wang, 2019). Nevertheless, the IPCC approach to estimate total carbon and total nitrogen released from CRB (IPCC, 1996) is transparent and comparable among countries and regions. So, we adopted the IPCC methodology to estimate the total carbon and total nitrogen emissions. Future research must focus on creating spatially explicit GHG emissions inventories, which will be highly helpful to identify the GHG sources and sinks, calculate the carbon footprint and create agricultural policy that reduce emissions (Shen et al., 2019).
Recommended Interventions in Crop Residue Management
The CRB has been increasing spatio-temporally and it is projected to increase further under business as usual scenario (Ravindra et al., 2019), due to rising food demand, agricultural mechanization, the short turnaround time between paddy and wheat crop (Shyamsundar et al., 2019) and resource constraints faced by the marginal and small farmers. Under these circumstances, the alternative options to crop residue burning should possess virtues of profitability, sustainability, technical feasibility and adoption potential. Present scenario of crop residue burning calls for an integrated agricultural bioeconomy approach. The agricultural bioeconomy approach towards crop residue management entails options which are sustainable, up-scalable, crop-and region-specific, socially inclusive, environmentally sound and technically robust. The agricultural bioeconomy approach further harnesses the synergies that are existing among the alternative options, aims at mitigating climate change and contributes to the achievement of sustainable development goals. The inclusion of bioeconomy perspectives in the management of crop residues is driven by factors such as:
(a) Human well-being, health and sustainable living;
(b) Sustainable agricultural production system and urge to decarbonize the agricultural economy;
(c) Emerging opportunities through waste valorization and production of value-added products;
(d) Alternative options to crop residue burning which has the potential to strengthen gender equality and provide sustainable livelihood opportunities;
(e) Circularity in agriculture production system exemplified through reuse and recycling of crop residues; and
(f) Climate change mitigation.
Agricultural bioeconomy, in effect, plays a significant role in the transition and transformation of input-intensive agriculture to multifunctional agriculture (Jordan et al., 2007), wherein the crop wastes are recycled, re-used to the benefit of the agroecosystem. The CRM demands a transition from the traditional zone of CRM to bioeconomy zone of CRM, wherein the interventions aim at the sustainability of agroecosystem (Figure 6).
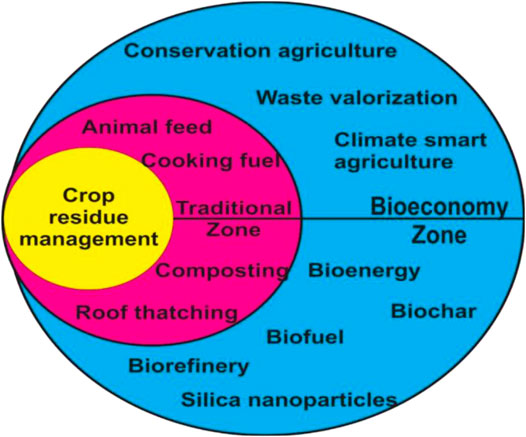
FIGURE 6. Crop Residue Management: A paradigm shift from the traditional approach to Agricultural Bioeconomy and circular economy.
Traditionally crop residues were used as a domestic fuel, animal feed, animal bedding material, packaging material, and roof thatching (Lohan et al., 2018). In the present scenario of the huge availability of crop residues, there is a need for finding alternative uses of crop residues. Interventions are needed in the backdrop of declining soil fertility and productivity; urge to achieve food and nutritional security and carbon sustainability, and to minimise health concerns and GHG emissions during the life cycle of crop production. In the multifunctional agricultural system, to ensure economic efficiency, the system must factor the principles of reuse and recycle of crop residues. In the following sections, we present an overview of the interventions in CRM that meet the environmental, social and economic objectives of agricultural bioeconomy. The crop residue management can be viewed through the lens of a circular economy to figure out the different pathways for sustainable management of crop residues (Figure 7). The circular economy approach enables maximisation of the services provided by the crop residues and at the same time minimise the negative impacts of the crop residue burning. In this regard, the interventions from the perspective of circular bioeconomy involve recirculation of material flows and adoption of restoration cycle (formation of new biomass). The interventions in crop residue management are conservation agriculture (Shyamsundar et al., 2019), in-situ straw incorporation (Chivenge et al., 2019), biomass energy production (Jiang et al., 2012; Hiloidhari et al., 2014; Ngan et al., 2019), biofuel generation (Liska et al., 2014; Prasad et al., 2020), crop residue-based biorefinery (Cherubini and Ulgiati, 2010), biochar production (Joseph et al., 2013; Singh and Sidhu, 2014; Windeatt et al., 2014; Singh et al., 2015; Zhou et al., 2018; Li et al., 2019), composting (Aynehband et al., 2017; Nghi et al., 2019) and mushroom production (Philippoussis, 2009; NPMCR, 2014; Lohan et al., 2018; Thuc et al., 2019).
Potential of CRM Initiatives to Achieve SDGs
CRM using the bioeconomy principles and guidelines provide a path to reduce the emissions of pollutant species; opportunities to small farmers to generate value-added products from crop residues, and improve soil health and quality through straw incorporation into the soil (Figure 8). The bioeconomy principles aim to 1) ensure food and nutritional security, 2) reduce dependency on non-renewable resources and augment the use of biomass for energy, 3) manage the natural resources sustainably, and 4) minimize greenhouse gases emissions and mitigate climate change. Crop residue burning releases climate pollutants (CO2, CH4 and N2O), ozone, aerosols (Black carbon, organic carbon), trace gases (NH3, SO2, Non-methane Volatile Organic Compounds) and particulates (PM2.5 and PM10) (Crutzen and Andreae, 1990; Andreae and Merlet, 2001; Koppmann et al., 2005; Mittal et al., 2009; Chang and Song, 2010; Sahai et al., 2011; Zhang et al., 2011). Avoiding crop residue burning per se mitigate the emission of air pollutants (Zhang et al., 2016), leading to public health benefits (Jethva et al., 2019; Shyamsundar et al., 2019), human well-being and climate change mitigation (Smith et al., 2007). Alternative options to crop residue burning (Shyamsundar et al., 2019) aid in achieving SDG 3 (Good health and well-being) and SDG 13 (Climate Action). As regards the GHG emissions, crop residue burning contributes about 0.027 GtCO2 eq. So, adoption of climate-smart agricultural practices (Venkatramanan and Shah, 2019) and conservation agriculture practices which entail the incorporation of crop stubbles into the soil in addition to sequestering carbon (SDG 13—Climate action), increase the soil organic carbon content through enhanced activity of soil organisms (SDG 15—Life on land). The crop residues with wide ‘Carbon/Nitrogen’ ratio are food resources to the soil micro-organisms and the straw incorporation into the soil increases the metabolic activity of and diversity of soil microorganisms (SDG 15). Crop residues used as industrial raw materials or feedstock for the generation of biofuel and bioenergy (SDG 7) provide economic and livelihood opportunities to farmers (SDG 1—Poverty reduction; SDG 2—Food and nutritional security). Crop residues driven cottage industries such as paddy straw mushroom production provide employment opportunities to the farmers (SDG 8), particularly the women (SDG 5), which greatly improve the household food and nutrition security (SDG 2). Ronzon and Sanjuan (2020) emphasized the importance of bioeconomy in achieving twelve out of 17 sustainable development goals.
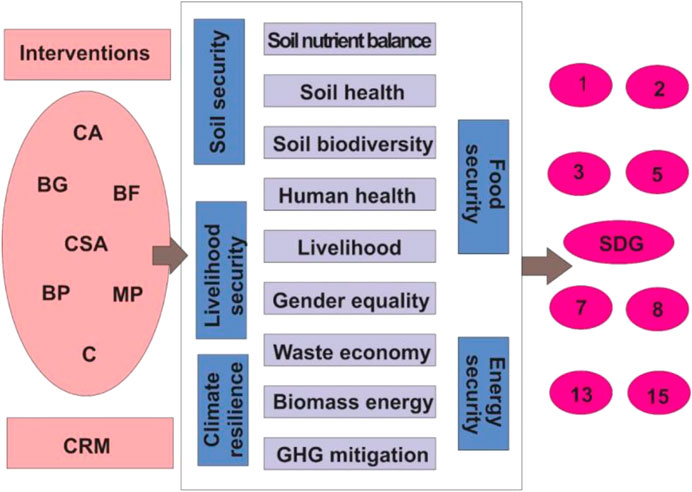
FIGURE 8. Mapping of benefits of bioeconomy driven interventions in crop residue management. Abbreviations: CA, Conservation Agriculture; CSA, Climate Smart Agriculture; C, Composting; BF, Biofuel; BP, Biochar Production; BG, Biogas; MP, Mushroom Production.
CRM-Bioeconomy Model
Figure 9 depicts a bioeconomy model towards the management of crop residues. The bioeconomy approach has the potential to provide ample opportunities to reduce CRB, increase farmers’ livelihood and decarbonise the agriculture production system. The proposed bioeconomy model has a four-pronged strategy which is depicted in Figure 9 as four quadrants. The focus of agricultural bioeconomy is on the “production, utilisation and conservation of biological resources”. In the sphere of agriculture production, the aim is to increase agricultural production through sustainable intensification (Garnett et al., 2013; Campbell et al., 2014); multi-functional agriculture (Jordan et al., 2007); energy plantations (Lal, 2005; Lal and Pimentel, 2007); climate-smart agriculture (Venkatramanan and Shah, 2019); and conservation agriculture. These strategies, in effect, aim to sustain crop production, use and re-use agricultural resources sustainably, mitigate the GHG emissions and adapt the agricultural production system to changing climate. Crop residues are the most important renewable resources on the earth. It essentially provides more opportunities than challenges, provided the interventions in CRM imbibe the circularity concept and meet the economic, ecological and social objectives of sustainable development. The smart agricultural practices sync with the broad contours of the aspirational principles and criteria for sustainable bioeconomy (FAO, 2019). CRM interventions besides the traditional use of crop residues include biorefineries, waste valorization, biomass conversion, power generation, bioethanol production and mushroom production. The bioeconomy perspective of these interventions can be gauged from the synergies and nexus between the interventions and the sustainable criteria mapped in the aspirational principles for sustainable bioeconomy. The co-benefits of the bioeconomy driven interventions include the food and nutrition security, sustainable intensification (principle 1), biodiversity conservation, climate change mitigation, soil quality improvement (principle 2), improvement in farmers’ income, the resilience of the farmers’ (principle 4), waste re-use, recycle and resource-use efficiency (principle 5) and adoption of both innovative and indigenous traditional knowledge in CRM (principle 7). Notwithstanding the positives of the bioeconomy approach in CRM, the success of the interventions lies with proactive government policy and capacity building, which have been aptly factored in the model. The ignorance and lack of awareness about CRB, public health issues, soil health must be removed through education, awareness campaigns, demonstration farms and farmers’ training. Further, government policies can reduce CRB through penal action, economic incentives and support. The options that are integrated into the proposed agricultural waste bioeconomy model for the agricultural landscape in India can provide lessons for other agricultural regions having similar environmental constraints and ecological settings.
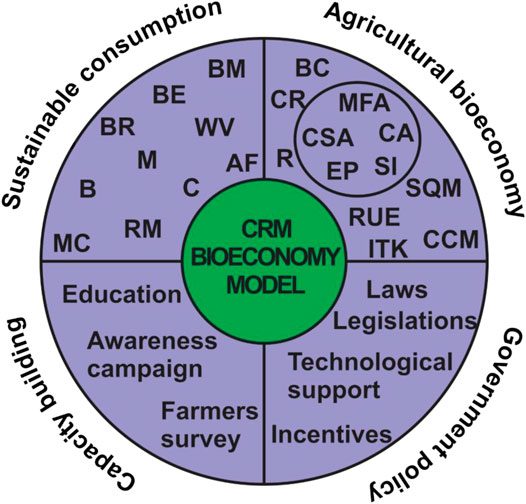
FIGURE 9. Crop Residue Management–A bioeconomy model. Agricultural bioeconomy quadrant: SI, Sustainable Intensification; CSA, Climate-Smart Agriculture; MFA, Multifunctional Agriculture; CA, Conservation Agriculture; EP, Energy Plantations; BC, Biodiversity Conservation; CR, Climate Resilience; CCM, Climate Change Mitigation; SQM, Soil Quality Management; R, Resilience of biomass producers; RUE, Resource Use Efficiency; ITK-Indigenous Traditional Knowledge. Sustainable consumption quadrant: BR, Biorefinery; BE, Biomass Energy; BM, Building Material; B, Biofuel; MC, Mushroom Cultivation; M, Mulch; WV, Waste Valorization; AF, Animal Feed; C, Compost; RM, Roofing Materials.
Conclusions
Agricultural crop residues are the carbon-rich biomass that is left in the field after harvesting the economic part of the crop. The quantity of crop residues that are generated is increasing year after year on account of the agricultural extent, intensification of agriculture and farm mechanization. The total amount of crop residues generated for the year 2017–18 was 516 million tonnes. Agricultural crop residue burning has emerged as an important challenge in the agricultural production system on account of rising air pollution episodes, the release of short-lived climate pollutants and declining soil health. The total crop residue burned increased from 18 million tonnes to 116 million tonnes between 1950–51 and 2017–18. Spatial-temporal variation of the crop residue burning demands the use of satellite observations, monitoring using an artificial intelligence-enabled unmanned aerial vehicle, combined with farmers’ survey to reduce the uncertainty involved in the national inventory of GHG emissions from crop residue burning. The drivers to crop residue burning are quantum of crop residue generation; labour scarcity; nutritionally poor rice crop residues; the short time interval between the harvesting of monsoon (Kharif) crop and sowing of winter (Rabi) crop; absence of appropriate crop residue management technology; economic resource constraints and lack of awareness about the public health issues due to crop residue burning. Identification of drivers of crop residue burning provides necessary input for the development of location-specific and demand-based alternative options and preparing a socially inclusive national policy on crop residue management. The CRM demands a transition from the traditional zone of CRM to bioeconomy zone of CRM, wherein the interventions aim at the sustainability of agroecosystem. Sustainable agricultural bioeconomy provides ample opportunities to reduce crop residue burning, increase farmers’ livelihood and decarbonize the agricultural production. The options that are integrated into the proposed agricultural waste bioeconomy model for the agricultural landscape in India can provide lessons for other agricultural regions having similar environmental constraints and ecological settings.
Data Availability Statement
The raw data supporting the conclusion of this article will be made available by the authors, without undue reservation.
Author Contributions
All authors have made a substantial, direct, and intellectual contribution to the work and approved it for publication.
Conflict of Interest
The authors declare that the research was conducted in the absence of any commercial or financial relationships that could be construed as a potential conflict of interest.
Abbreviations
C, carbon; CO2e, carbon dioxide equivalent; GWP, global warming potential; GHGs, greenhouse gases; Gt, gigatonnes; MT, million tonnes; Tg, teragram; Gg, gigagram; CRB, crop residue burning; CRM, crop residue management; mha, million hectares.
References
An, L., Liu, Y., and Hao, L. (2016). Contributions of open crop straw burning emissions to PM 2.5 concentrations in China. Environ. Res. Lett. 11, 014014. doi:10.1088/1748-9326/11/1/014014
Andreae, M., and Merlet, P. (2001). Emission of trace gases and aerosols from biomass burning. Global Biogeochem. Cycles 15, 955–966. doi:10.1029/2000gb001382
Awasthi, A., Agarwal, R., Mittal, S., Singh, N., Singh, K., and Gupta, P. (2011). Study of size and mass distribution of particulate matter due to crop residue burning with seasonal variation in the rural area of Punjab, India. J. Environ. Monit. 13, 1073–1081. doi:10.1039/c1em10019j |
Aynehband, A., Gorooei, A., and Moezzi, A. (2017). Vermicompost: an eco-friendly technology for crop residue management in organic agriculture. Energy Procedia. 141, 667–671. doi:10.1016/j.egypro.2017.11.090
Bhargava, A. (2018). Climate variability, rice production and groundwater depletion in India. Environ. Res. Lett. 13. doi:10.1088/1748-9326/aaade9
Bhuvaneshwari, S., Hettiarachchi, H., and Meegoda, J. (2019). Crop residue burning in India: policy challenges and potential solutions. Int. J. Environ. Res. Publ. Health. 16, 832. doi:10.3390/ijerph16050832
Campbell, B., Thornton, P., Zougmoré, R., van Asten, P., and Lipper, L. (2014). Sustainable intensification: what is its role in climate-smart agriculture? Curr. Opin. Env. Sust. 8, 39–43. doi:10.1016/j.cosust.2014.07.002
Chakrabarti, S., Khan, M., Kishore, A., Roy, D., and Scott, S. (2019). Risk of acute respiratory infection from crop burning in India: estimating disease burden and economic welfare from satellite and national health survey data for 250 000 persons. Int. J. Epidemiol. 48, 1113–1124. doi:10.1093/ije/dyz022 |
Chandra, B. P., and Sinha, V. (2016). Contribution of post-harvest agricultural paddy residue fires in the N.W. Indo-Gangetic Plain to ambient carcinogenic benzenoids, toxic isocyanic acid and carbon monoxide. Environ. Int. 88, 187–197. doi:10.1016/j.envint.2015.12.025 |
Chang, D., and Song, Y. (2010). Estimates of biomass burning emissions in tropical Asia based on satellite-derived data. Atmos. Chem. Phys. 10, 2335–2351. doi:10.5194/acp-10-2335-2010
Charkovska, N., Horabik-Pyzel, J., Bun, R., Danylo, O., Nahorski, Z., Jonas, M., et al. (2019). High-resolution spatial distribution and associated uncertainties of greenhouse gas emissions from the agricultural sector. Mitig. Adapt. Strategies Glob. Change. 24, 881–905. doi:10.1007/s11027-017-9779-3
Chawala, P., and Sandhu, H. A. S. (2020). Stubble burn area estimation and its impact on ambient air quality of Patiala & Ludhiana district, Punjab, India. Heliyon. 6, e03095. doi:10.1016/j.heliyon.2019.e03095 |
Cherubini, F., and Ulgiati, S. (2010). Crop residues as raw materials for biorefinery systems—a LCA case study. Appl. Energy. 87, 47–57. doi:10.1016/j.apenergy.2009.08.024
Chen, J., Li, C., Ristovski, Z., Milic, A., Gu, Y., Islam, M. S., et al. (2017). A review of biomass burning: Emissions and impacts on air quality, health and climate in China. Sci. Total Environ. 579 1000–1034. doi:10.1016/j.scitotenv.2016.11.025
Chivenge, P., Rubianes, F., Van Chin, D., Van Thach, T., Khang, V., Romasanta, R., et al. (2019). “Rice straw incorporation influences nutrient cycling and soil organic matter,” in In sustainable rice straw management Editors M. Gummert, Nguyen Van Hung, P. Chivenge, and B. Douthwaite (Switzerland, Cham): Springer), 131–144. doi:10.1007/978-3-030-32373-8_8
Crutzen, P., and Andreae, M. (1990). Biomass burning in the tropics: impact on atmospheric chemistry and biogeochemical cycles. Science 250, 1669–1678. doi:10.1126/science.250.4988.1669 |
Dey, D., Gyeltshen, T., Aich, A., Naskar, M., and Roy, A. (2020). Climate adaptive crop-residue management for soil-function improvement; recommendations from field interventions at two agro-ecological zones in South Asia. Environ. Res. 183, 109164. doi:10.1016/j.envres.2020.109164 |
Dimitrov, D. D., and Wang, J. (2019). Geographic Inventory Framework for estimating spatial pattern of methane and nitrous oxide emissions from agriculture in Alberta, Canada. Environmental Development 32, 100461. doi:10.1016/j.envdev.2019.100461
Economic Survey (2020). Economic survey 2019–20. Statistical appendix. Available at: https://www.indiabudget.gov.in/economicsurvey/doc/Statistical-Appendix-in-English.pdf. (Accessed April 14, 2020).
Erenstein, O. (2011). Cropping systems and crop residue management in the Trans-Gangetic Plains: issues and challenges for conservation agriculture from village surveys. Agric. Syst. 104, 54–62. doi:10.1016/j.agsy.2010.09.005
Famiglietti, J. S. (2014). The global groundwater crisis. Nat. Clim. Change. 4, 945–948. doi:10.1038/nclimate2425
Fang, Y., Xu, K., Guo, X., and Hong, Y. (2020). Identifying determinants of straw open field burning in northeast China: toward greening agriculture base in newly industrializing countries. J. Rural Stud. 74, 111–123. doi:10.1016/j.jrurstud.2019.12.013
FAO (2019). Towards sustainable bioeconomy guidelines. Available at: http://www.fao.org/3/ca5145en/ca5145en.pdf (Accessed April 24, 2020).
Garnett, T., Appleby, M., Balmford, A., Bateman, I., Benton, T., Bloomer, P. P., et al. (2013). Agriculture. Sustainable intensification in agriculture: premises and policies. Science 341, 33–34. doi:10.1126/science.1234485
Goswami, S. B., Mondal, R., and Mandi, S. K. (2020). Crop residue management options in rice–rice system: a review. Arch. Agron Soil Sci. 66, 1218–1234. doi:10.1080/03650340.2019.1661994
Government of India (2018). Agricultural statistics at a glance 2018 Delhi.: Department of Agriculture, Cooperation & Farmers Welfare, Directorate of Economics & Statistics, Government of India.
Gupta, R., Singh, Y., Ladha, J. K., Singh, B., Singh, J., Singh, G., et al. (2007). Yield and phosphorus transformations in a rice-wheat system with crop residue and phosphorus management. Soil Sci. Soc. Am. J. 71, 1500–1507. doi:10.2136/sssaj2006.0325
Guttikunda, S., and Goel, R. (2013). Health impacts of particulate pollution in a megacity—Delhi, India. Environ. Dev. 6, 8–20. doi:10.1016/j.envdev.2012.12.002
Hiloidhari, M., Das, D., and Baruah, D. (2014). Bioenergy potential from crop residue biomass in India. Renew. Sustain. Energy Rev. 32, 504–512. doi:10.1016/j.rser.2014.01.025
IPCC (1996). Greenhouse gas inventory workbook. Revised 1996 IPCC guidelines for national greenhouse gas inventories. Available at: https://www.ipcc-nggip.iges.or.jp/public/gl/invs5c.html (Accessed April 14, 2020).
IPCC (2006). IPCC guidelines for national greenhouse gas inventories. Available at: https://www.ipcc-nggip.iges.or.jp/support/Primer_2006GLs.pdf (Accessed April 14, 2020).
Jain, N., Bhatia, A., and Pathak, H. (2014). Emission of air pollutants from crop residue burning in India. Aerosol Air Qual. Res. 14, 422–430. doi:10.4209/aaqr.2013.01.0031
Jat, H. S., Jat, R. D., Nanwal, R. K., Lohan, S. K., Yadav, A. K., Poonia, T., et al. (2020). Energy use efficiency of crop residue management for sustainable energy and agriculture conservation in NW India. Renew. Energy. 155, 1372–1382. doi:10.1016/j.renene.2020.04.046
Jethva, H., Torres, O., Field, R., Lyapustin, A., Gautam, R., and Kayetha, V. (2019). Connecting crop productivity, residue fires, and air quality over northern India. Sci. Rep. 9, 16594. doi:10.1038/s41598-019-52799-x |
Jiang, D., Zhuang, D., Fu, J., Huang, Y., and Wen, K. (2012). Bioenergy potential from crop residues in China: availability and distribution. Renew. Sustain. Energy Rev. 16, 1377–1382. doi:10.1016/j.rser.2011.12.012
Jordan, N., Boody, G., Broussard, W., Glover, J., Keeney, D., McCown, B., et al. (2007). Sustainable development of the agricultural bio-economy. Science 316, 1570–1571. doi:10.1126/science.1141700 |
Kanabkaew, T., and Oanh, N. T. K. (2011). Development of spatial and temporal emission inventory for crop residue field burning. Environ. Model. Assess. 16, 453–464. doi:10.1007/s10666-010-9244-0
Kaur, S. (2020). Public preferences for setting up a biomass power plant to combat open-field burning of rice crop residues: a case study of district Sangrur, Punjab, India. Biomass Bioenergy 138, 105577. doi:10.1016/j.biombioe.2020.105577
Kim Oanh, N. T., Permadi, D. A., Hopke, P. K., Smith, K. R., Dong, N. P., and Dang, A. N. (2018). Annual emissions of air toxics emitted from crop residue open burning in Southeast Asia over the period of 2010–2015. Atmos. Environ. 187, 163–173. doi:10.1016/j.atmosenv.2018.05.061
Koppmann, R., von Czapiewski, K., and Reid, J. (2005). A review of biomass burning emissions. Part I: gaseous emissions of carbon monoxide, methane, volatile organic compounds, and nitrogen-containing compounds. Atmos. Chem. Phys. Discuss. 5, 10455–10516. doi:10.5194/acpd-5-10455-2005
Lal, R., and Pimentel, D. (2007). Biofuels from crop residues. Soil Tillage Res. 93, 237–238. doi:10.1016/j.still.2006.11.007
Lal, R. (2005). World crop residues production and implications of its use as a biofuel. Environ. Int. 31, 575–584. doi:10.1016/j.envint.2004.09.005 |
Li, R., Chen, W., Zhao, H., Wu, X., Zhang, M., Tong, D. Q., et al. (2020). Inventory of atmospheric pollutant emissions from burning of crop residues in China based on satellite-retrieved farmland data. Chin. Geogr. Sci. 30, 266–278. doi:10.1007/s11769-020-1110-7
Li, Z., Song, Z., Singh, B., and Wang, H. (2019). The impact of crop residue biochars on silicon and nutrient cycles in croplands. Sci. Total Environ. 659, 673–680. doi:10.1016/j.scitotenv.2018.12.381 |
Liska, A., Yang, H., Milner, M., Goddard, S., Blanco-Canqui, H., Pelton, M., et al. (2014). Biofuels from crop residue can reduce soil carbon and increase CO2 emissions. Nat. Clim. Change. 4, 398–401. doi:10.1038/nclimate2187
Liu, T., Mickley, L. J., Singh, S., Jain, M., DeFries, R. S., and Marlier, M. E. (2020). Crop residue burning practices across north India inferred from household survey data: Bridging gaps in satellite observations. Atmos. Envirot.: X, 100091. doi:10.1016/j.aeaoa.2020.100091
Lohan, S., Jat, H., Yadav, A., Sidhu, H., Jat, M., Choudhary, M., et al. (2018). Burning issues of paddy residue management in north-west states of India. Renew. Sustain. Energy Rev. 81, 693–706. doi:10.1016/j.rser.2017.08.057
Lopes, A. A., Viriyavipart, A., and Tasneem, D. (2020). The role of social influence in crop residue management: evidence from Northern India. Ecol. Econ. 169, 106563. doi:10.1016/j.ecolecon.2019.106563
Maheswarappa, H., Srinivasan, V., and Lal, R. (2011). Carbon footprint and sustainability of agricultural production systems in India. J. Crop Improv. 25, 303–322. doi:10.1080/15427528.2011.567382
Meng, L., Alengebawy, A., Ai, P., Jin, K., Chen, M., and Pan, Y. (2020). Techno-economic assessment of three modes of large-scale crop residue utilization projects in China. Energies 13. doi:10.3390/en13143729
Mittal, S., Singh, N., Agarwal, R., Awasthi, A., and Gupta, P. (2009). Ambient air quality during wheat and rice crop stubble burning episodes in Patiala. Atmos. Environ. 43, 238–244. doi:10.1016/j.atmosenv.2008.09.068
NABARD (2018). Sectoral paper on farm mechanization. Farm sector policy department. Mumbai, India: NABARD Head Office. Available at:https://www.nabard.org/auth/writereaddata/file/NSP%20Farm%20Mechanisation.pdf (Accessed April 14, 2020).
Nair, M., Bherwani, H., Kumar, S., Gulia, S., Goyal, S., and Kumar, R. (2020). Assessment of contribution of agricultural residue burning on air quality of Delhi using remote sensing and modelling tools. Atmos. Environ. 230, 117504. doi:10.1016/j.atmosenv.2020.117504
Ngan, N., Chan, F., Nam, T., Van Thao, H., Maguyon-Detras, M., Hung, D., et al. (2019). “Anaerobic digestion of rice straw for biogas production,” in In sustainable rice straw management. Editors M. Gummert, Nguyen Van Hung, P. Chivenge, and B. Douthwaite (Switzerland, Cham): Springer), 65–92. doi:10.1007/978-3-030-32373-8_5
Nghi, N., Romasanta, R., Van Hieu, N., Vinh, L., Du, N., Ngan, N., et al. (2019). “Rice straw-based composting,” in Sustainable rice straw management. Editors M. Gummert, Nguyen Van Hung, P. Chivenge, and B. Douthwaite (Switzerland, Europe: Springer), 33–41. doi:10.1007/978-3-030-32373-8_3
NPMCR (2014). National policy for management of crop residues. New Delhi, India: Government of India, Ministry of Agriculture, Department of Agriculture & Cooperation (Natural Resource Management Division), Krishi Bhawan. Available at:http://agricoop.nic.in/sites/default/files/NPMCR_1.pdf (Accessed April 24, 2020).
Philippoussis, A. N. (2009). “Production of mushrooms using agro-industrial residues as substrates,” in In biotechnology for agro-industrial residues utilisation -utilisation of agro-residues. Editors P. S. Nigam, and A. Pandey (Netherlands, Dordrecht: Springer), 163–196. doi:10.1007/978-1-4020-9942-7_9
Prasad, S., Kumar, S., Sheetal, K., and Venkatramanan, V. (2020). “Global climate change and biofuels policy: Indian perspectives,” in Global climate change and environmental policy: agriculture perspectives. Editors V. Venkatramanan, and Shachi. Shah (Singapore, Asia: Springer Nature Singapore Pte Ltd.), 207–226. doi:10.1007/978-981-13-9570-3_6
Ramanathan, V., and Carmichael, G. (2008). Global and regional climate changes due to black carbon. Nat. Geosci. 1, 221–227. doi:10.1038/ngeo156
Ravindra, K., Singh, T., and Mor, S. (2019). Emissions of air pollutants from primary crop residue burning in India and their mitigation strategies for cleaner emissions. J. Clean. Prod. 208, 261–273. doi:10.1016/j.jclepro.2018.10.031
Reicosky, D. (2015). Conservation tillage is not conservation agriculture. J. Soil Water Conserv. 70, 103A–108A. doi:10.2489/jswc.70.5.103a
Ronzon, T., and Sanjuán, A. (2020). Friends or foes? A compatibility assessment of bioeconomy-related Sustainable Development Goals for European policy coherence. J. Clean. Prod. 254, 119832. doi:10.1016/j.jclepro.2019.119832 |
Sahai, S., Sharma, C., Singh, S., and Gupta, P. (2011). Assessment of trace gases, carbon and nitrogen emissions from field burning of agricultural residues in India. Nutrient Cycl. Agroecosyst. 89, 143–157. doi:10.1007/s10705-010-9384-2
Sarkar, S., Singh, R. P., and Chauhan, A. (2018). Increasing health threat to greater parts of India due to crop residue burning. The Lancet Planetary Health. 2, e327–e328. doi:10.1016/S2542-5196(18)30166-9 |
Sembhi, H., Wooster, M., Zhang, T., Sharma, S., Singh, N., Agarwal, S., et al. (2020). Post-monsoon air quality degradation across Northern India: assessing the impact of policy-related shifts in timing and amount of crop residue burnt. Environ. Res. Lett. 15. doi:10.1088/1748-9326/aba714
Shah, S., and Venkatramanan, V. (2019). in “Advances in microbial technology for upscaling sustainable biofuel production,” in new and future developments in microbial biotechnology and bioengineering. Editors V. K. Gupta, and A. Pandey (Netherlands, Europe: Elsevier), 69–76. doi:10.1016/b978-0-444-63504-4.00005-0
Shen, J., Melaku, N. D., Treu, R., and Wang, J. (2019). Inventories of methane and nitrous oxide emissions from animal and crop farms of 69 municipalities in Alberta, Canada. J. Clean. Prod. 234, 895–911. doi:10.1016/j.jclepro.2019.06.270
Shyamsundar, P., Springer, N. P., Tallis, H., Polasky, S., Jat, M. L., Sidhu, H. S., et al. (2019). Fields on fire: alternatives to crop residue burning in India. Science. 365, 536–538. doi:10.1126/science.aaw4085 |
Singh, R., Babu, J., Kumar, R., Srivastava, P., Singh, P., and Raghubanshi, A. (2015). Multifaceted application of crop residue biochar as a tool for sustainable agriculture: an ecological perspective. Ecol. Eng. 77, 324–347. doi:10.1016/j.ecoleng.2015.01.011
Singh, R., Yadav, D. B., Ravisankar, N., Yadav, A., and Singh, H. (2020). Crop residue management in rice–wheat cropping system for resource conservation and environmental protection in north-western India. Environ. Dev. Sustain. 22, 3871–3896. doi:10.1007/s10668-019-00370-z
Singh, Y., and Sidhu, H. (2014). Management of cereal crop residues for sustainable rice-wheat production system in the indo-gangetic plains of India. Proc. Indian Natl. Sci. Acad. 80, 95–114. doi:10.16943/ptinsa/2014/v80i1/55089
Smith, P., Martino, M., Cai, Z., Gwary, D., Janzen, H., Kumar, P., et al. (2007). in “Agriculture, in climate change 2007: mitigation of climate change. Contribution of working group III to the fourth assessment report of the intergovernmental panel on climate change. Editors Metz, B. (Cambridge, United Kingdom: Cambridge University Press), 497–540.
Streets, D., Yarber, K., Woo, J., and Carmichael, G. (2003). Biomass burning in Asia: annual and seasonal estimates and atmospheric emissions. Global Biogeochem. Cycles 17, 10–11. doi:10.1029/2003gb002040
Thuc, L., Corales, R., Sajor, J., Truc, N., Hien, P., Ramos, R., et al. (2019). “Rice-straw mushroom production,” in Sustainable rice straw management Editors M. Gummert, N. Van Hung, P. Chivenge, and B. Douthwaite (Cham, Switzerland: Springer), 93–109. doi:10.1007/978-3-030-32373-8_6
Tipayarom, A., and Kim Oanh, N. T. (2020). Influence of rice straw open burning on levels and profiles of semi-volatile organic compounds in ambient air. Chemosphere. 243, 125379. doi:10.1016/j.chemosphere.2019.125379 |
Vadrevu, K., Ellicott, E., Badarinath, K., and Vermote, E. (2011). MODIS derived fire characteristics and aerosol optical depth variations during the agricultural residue burning season, north India. Environ. Pollut. 159, 1560–1569. doi:10.1016/j.envpol.2011.03.001 |
Venkatramanan, V., and Shah, S. (2019). “Climate smart agriculture technologies for environmental management: the intersection of sustainability, resilience, wellbeing and development,” in Sustainable green technologies for environmental management Editors S. Shah, V. Venkatramanan, and R. Prasad (Singapore, Asia: Springer Nature Singapore Pte Ltd.), 29–51. doi:10.1007/978-981-13-2772-8_2
Venkatramanan, V., Shah, S., and Prasad, R. (2021). Sustainable bioeconomy: pathways to sustainable development goals. Singapore: Springer Nature. doi:10.1007/978-981-15-7321-7
Windeatt, J., Ross, A., Williams, P., Forster, P., Nahil, M., and Singh, S. (2014). Characteristics of biochars from crop residues: potential for carbon sequestration and soil amendment. J. Environ. Manag. 146, 189–197. doi:10.1016/j.jenvman.2014.08.003
Wyse, S., Graber, E., Chia, C., Munroe, P., Donne, S., Thomas, T., et al. (2013). Shifting paradigms: development of high-efficiency biochar fertilizers based on nano-structures and soluble components. Carbon Manag. 4, 323–343. doi:10.4155/cmt.13.23
Zhang, H., Hu, D., Chen, J., Ye, X., Wang, S., Hao, J., et al. (2011). Particle size distribution and polycyclic aromatic hydrocarbons emissions from agricultural crop residue burning. Environ. Sci. Technol. 45, 5477–5482. doi:10.1021/es1037904 |
Keywords: crop residue burning, greenhouse gas, bioeconomy, conservation agriculture, sustainable development goals, crop residue management, North-western India, rice-wheat cropping system
Citation: Venkatramanan V, Shah S, Rai AK and Prasad R (2021) Nexus Between Crop Residue Burning, Bioeconomy and Sustainable Development Goals Over North-Western India. Front. Energy Res. 8:614212. doi: 10.3389/fenrg.2020.614212
Received: 05 October 2020; Accepted: 15 December 2020;
Published: 26 January 2021.
Edited by:
Suyun Xu, University of Shanghai for Science and Technology, ChinaReviewed by:
Muhammad Farooq, University of Engineering and Technology, PakistanJunye Wang, Athabasca University, Canada
Copyright © 2021 Venkatramanan, Shah, Rai and Prasad. This is an open-access article distributed under the terms of the Creative Commons Attribution License (CC BY). The use, distribution or reproduction in other forums is permitted, provided the original author(s) and the copyright owner(s) are credited and that the original publication in this journal is cited, in accordance with accepted academic practice. No use, distribution or reproduction is permitted which does not comply with these terms.
*Correspondence: V. Venkatramanan, vvenkatramanan@rediffmail.com; Ram Prasad, ramprasad@mgcub.ac.in