- 1Department of Physics, Suresh Gyan Vihar University, Jaipur, India
- 2Department of Inorganic Chemistry, Faculty of Science, P. J. Safarik University, Kosice, Slovak Republic
- 3Center of Excellence for Energy and Environmental Studies, Deenbandhu Chhotu Ram University of Science and Technology, Murthal, India
- 4Department of Chemistry, Deenbandhu Chhotu Ram University of Science and Technology, Murthal, India
- 5Department of Physics, School of Engineering and Technology, Central University of Haryana, Mahendergarh, India
The nanoporous metal-organic framework (MOF), MOF-76(Nd) [neodymium (III) benzene-1,3,5-tricarboxylate], has been synthesized, characterized, and tested for hydrogen storage and humidity sensing applications. These synthesized MOFs were characterized using scanning and transmission electron microscopy techniques. Thermal analysis revealed that, after the dehydration process, the compound showed high thermal stability up to 500°C. Hydrogen adsorption/desorption measurements of MOF-76(Nd) were performed at 77K and 20 bar and the material was further used for the humidity measurement at room temperature.
Introduction
To overcome hydrogen storage challenges several nanomaterials like zeolites, activated carbon, and carbon nanotubes have been widely studied by various research groups. Nowadays metal-organic frameworks (MOFs) have fascinated an excessive devotion of scientific community towards hydrogen storage applications (Broom et al., 2016; International Energy Agency, 2015; Toyota, 2018). Fast kinetics with complete reversibility can be achieved via physisorption technique; therefore this would be a safe and efficient system for hydrogen storage. Hydrogen storage with ambient conditions of temperature and pressure is a crucial task to empower this technology (Zacharia et al., 2010; Purewal et al., 2012; Ming et al., 2014).
MOFs are auspicious resources for hydrogen storage because of their high surface area, porosity, and flexible and tunable porous structure in comparison to the conventional materials like zeolites (Dailly and Poirier, 2011; Ardelean et al., 2013; Balderas-Xicohtencatl et al., 2018). Theoretically unbounded organic linkers with various metal nodes can easily be tuned in a specific manner to make the desired composition of MOFs for required target. High porosity, high surface area, and low density of MOFs are their key properties for gas adsorption, gas sensing, and gas storage applications (Almáši et al., 2018a; Almáši et al., 2018b). Hydrogen, carbon dioxide, and methane can easily be captured by different type of MOFs due to their ordered porous composition. Pore volume is an important factor to calculate the upper limit of physical gas storage (Almáši et al., 2014; Blanita et al., 2014; Mlsna et al., 2015; Heitzer et al., 2016).
Humidity sensing is another important application of MOFs. Humidity sensors are potentially applicable in agriculture, medicine, and industrial developments. For environment and equipment maintenance humidity control with high accuracy is required. Humidity sensing also plays an important role in food package, drug storage, and research labs (Li et al., 2013; Pal et al., 2016; Boudaden et al., 2018). Many commercial humidity sensors are available in market to detect unpredictable materials with different ppm level concentrations but humidity sensing lower than 100 ppm level is still under development (Chen and Lu, 2005; Hossein-Babaei and Shabani, 2014; Islam et al., 2015). There are several synthesis methods for MOFs having large range of chemical and physical features with wide prospect of engineering these highly ordered compositions of MOFs make them appropriate candidate for humidity sensing applications (Meek et al., 2011; Kreno et al., 2012; Bahreyni and Khoshaman, 2012; Liu et al., 2013). Adsorption or desorption of gas molecules produces modification in properties of the samples which can be calculated as amounts of analytes (Campbell and Dinca, 2017).
Even a lot of research work is not reported using MOFs for humidity sensing applications (Stavila et al., 2014). Manufacturing of humidity sensors having relative humidity less than 1% is generally expensive. It is a challenging task to use MOFs as a humidity sensing material because of its skeletal composition that may be breakdown in heavy moisture. Some of the MOFs are reported, for humidity sensing but showing less because of limited surface areas (Qiu et al., 2011; Yao and Zhu, 2014; Fischer et al., 2015)
In this research work, MOF-76(Nd) synthesized by using solvothermal method has been characterized by different techniques and further used for hydrogen storage and humidity sensing applications.
Experimental Details
Chemicals
All chemicals used in the synthesis of MOF-76(Nd) were purchased from Sigma-Aldrich (Nd(NO3)3·6H2O (99.9%), benzene-1,3,5-tricarboxylic acid (H3BTC, 95%), N,N´-dimethylformamide (DMF, 99.8%), and ethanol (EtOH, 99%)) with highest existing purity and used without further purification.
Synthesis of Metal-Organic Framework-76(Nd)
50 mg of Nd(NO3)3·6H2O (0.1141 mmol) and 20 mg of H3BTC (0.0952 mmol) were dissolved in the mixture of solvents EtOH/DMF/H2O in a volume ratio 3/3/2.5 (cm3). The reaction mixture was kept in glass autoclave and heated to 80°C with a heating rate of 2°C min−1 for 24 h. After this time the reaction mixture was cooled to ambient temperature with a cooling rate of 2°C min−1. Violet needle-shaped crystals of MOF-76(Nd) were filtered off, slightly washed with ethanol, and dried in the stream of air (yield: 42 mg, 96% based on H3BTC). Elemental analysis for Nd1C9H15O12 ([Nd(BTC)(H2O)]·5H2O; 459.45 g mol−1): clcd. C 25.53%, H 3.29%, found: C 25.68%, H 3.34%. Infrared spectroscopy (IR): ν(OH) – 3405 (m, br), ν(CH)ar – 3105, 3088 (w), ν(CC)ar – 1603 (s), νas(COO−) – 1540 (s), νas(COO−) – 1425 (s), δ(COO−) – 752 (s) cm−1 (w – weak, m – medium, s – strong, br – broad, ar – aromatic, s – symmetric, as – antisymmetric).
Characterization
CHNOS Elemental Analyzer Vario MICRO from Elementar Analysensysteme GmbH was used for elemental studies. Shape and size of these samples are observed using transmission electron microscope (HRTEM) (Technai G20-stwin) which was operated at voltage 200 kV and having resolution 2.32 (in angstrom). As-synthesized MOF-76(Nd) powder was dissolved in acetone using ultrasonicator and coated on the copper grid to observe TEM image. Surface morphology of MOF-76(Nd) is observed by field emission scanning electron microscopy (FESEM- JEOL JSM-6700F) which was operated at 15 kV accelerating voltage with a resolution of 1.0 nm. To determine the dehydration process and thermal stability of the sample thermogravimetric analyzer (Hitachi DSC7020) is used up to 600°C under air atmosphere and the sample weight was 1.4 mg. The infrared spectra of the sample were recorded using an Avatar FT-IR 6700 spectrometer in the ATR configuration and 4000–400 cm−1 wavenumbers’ range. This measurement was performed at room temperature.
Hydrogen Storage Measurements
High-pressure hydrogen adsorption/desorption isotherm was observed using a Sievert’s type setup (Setaram-HyEnergy PCTPro-2000). This is attached with a micro-doser (MD-HyEnergy) which helps in the measurements of small amounts of sample. To achieve liquid nitrogen (77 K temperature) the sample was dipped in a thermal bath. The amount of MOF-76(Nd) used for measurements was approximately 150 mg. The sample was activated for 12h at 240°C.
Humidity Sensing Measurements
Humidity sensing characteristics of MOF-76(Nd) have been measured on ceramic substrate having Ag-Pd interdigital electrode. Saturated salts solution of LiCl, MgCl2·6H2O, Mg (NO3)2·6H2O, NaCl, KCl, and K2SO4 have been used at ambient conditions with relative humidity value of 11%, 33%, 53%, 75%, 84%, and 98% respectively.
Results and Discussion
MOF-76(Ln) represents a family of nanoporous isostructural lanthanide-based MOFs with general composition [Ln(BTC)(H2O)]·G (Ln-lanthanide, BTC-benzene-1,3,5-tricarboxylate, G-guest). These compounds are characterized by high thermal stability, porosity, and three-dimensional transformable frameworks. In the present study, the neodymium form of MOF-76(Nd) with the chemical composition [Nd(BTC)(H2O)]·5H2O was prepared and tested as an adsorbent for hydrogen and humidity sensor. Compound MOF-76(Nd) crystallized in the tetragonal crystallographic system and consists of Nd(III) ions bridged by carboxylic groups of BTC linker under formation of the 3D polymeric framework with 1D sinusoidally shaped channels of size about 7 × 7 Å propagating along c axis (see Figure 1). Previous studies (Gustafsson et al., 2010; Almáši et al., 2015; Almáši et al., 2016; Dong et al., 2019; Zeleňák et al., 2019) have shown that compounds [Ln(BTC)(H2O)].G undergo structural changes depending on the temperature. The framework starts to transform after heating to ∼150°C from tetragonal to monoclinic and upon heating to ∼300°C the crystal structure is transformed back to the tetragonal system.
The as-synthesized material was initially characterized by transmission electron microscopy (TEM) and obtained TEM micrographs are depicted in Figure 2. TEM images represent the distribution of as-synthesized MOF-76(Nd) material. TEM images show the contrast of as-synthesized MOF-76(Nd). It is clear from TEM images that synthesized material is having rod-like structure with an average length of about 20–40 µm and showing no agglomeration.
Figure 3 shows energy dispersion spectra of MOF-76(Nd) and in inset of this figure elemental composition of this MOF in weight % and atomic % is represented. In the synthesized material, Nd have been observed 99.12 in weight %. The atomic % of Nd has been found 92.59. In this way, presence of Nd is confirmed by EDS. The presence of iron and copper in the EDS spectrum comes from the holder used in the measurement. The presence and weight percentage of carbon and hydrogen in MOF-76(Nd) were also determined using elemental analysis. Observed and calculated values correlate well with the chemical composition of the material; see Synthesis of Metal-Organic Framework-76(Nd).
Distribution of MOF´s crystals and their structure are shown using SEM image. Figure 4 shows the rods of MOF-76(Nd) and it is also confirmed from FESEM that MOF-76(Nd) rods have length in the range of 20–40 μm. EDS map scanning has also been observed and showing elemental presence of Nd as 24.606 in weight% and 2.091 in atomic % as shown in inset of EDS spectra of Figure. 4.
Thermogravimetric (TG) analysis was used to study the dehydration process and the decomposition temperature of the material. Figure 5 shows the TG curve of MOF-76(Nd) measured in the air atmosphere and temperature up to 600°C. The sample is thermally stable after heating to 90°C; in the temperature range 90–150°C the dehydration process takes place. The release of water molecules was accompanied by an endothermic effect on the DTA curve with a minimum at 126°C. The total mass loss of 18 wt% in the first stage of thermal decomposition was observed and corresponds to the release of six water molecules (clcd. mass loss 23.5 wt%). Dehydrated form of MOF-76(Nd) is considerably stable in 150oC to 500oC temperature.
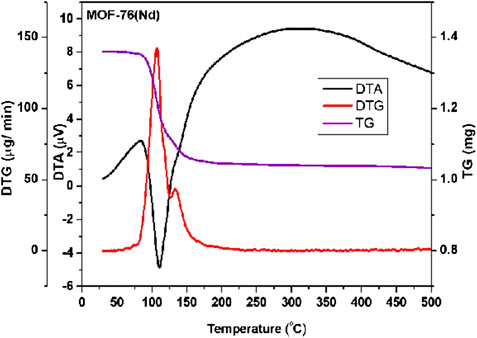
FIGURE 5. TG/DTG-DTA curves of MOF-76(Nd) measured in temperature range of 25–600°C and in an air atmosphere.
The infrared spectroscopy (IR) was further used to confirm the presence of BTC and water molecules in prepared material. The IR spectrum of MOF-76(Nd) is shown in Figure 6 and the wavenumbers of the principal IR absorption bands are listed in Synthesis of Metal-Organic Framework-76(Nd). The presence of water molecules in prepared sample is evident from a broad absorption band located at 3405 cm−1 corresponding to ν(OH) stretching vibration. The BTC ligand is characterized by weak absorption bands at 3105 cm−1 and 3088 cm−1 which could be assigned to aromatic ν(CH)ar vibrations. Further, benzene-1,3,5-tricarboxylate ion is characterized by strong absorption bands of carboxylate groups located at 1540 cm−1 for antisymmetric (νas(COO−)) and at 1425 cm−1 for symmetric (νs(COO−)) stretching vibrations.
Figure 7 shows hydrogen adsorption/desorption isotherms of MOF-76(Nd) measured at 77K and up to 20 bar. As can be seen, the activated material does not absorb hydrogen in the whole pressure range. We suppose that compound at low temperature undergoes also the structural change, similar to the previously observed changes with increasing temperature (Gustafsson et al., 2010; Almáši et al., 2015; Almáši et al., 2016; Zeleňák et al., 2019), leading to the formation of a nonporous modification. Another reason for explanation the low adsorption capacity of hydrogen may be the low activation temperature of the sample.
Figure 8 shows humidity sensing curve of MOF-76(Nd) in the 11–98 %RH range at room temperature. The %RH measurements were performed using resistance variance method. At low humidity conditions (11 %RH), sample exhibits very high impedance; however, a dramatic decrease in impedance was observed at high humidity conditions (98 %RH). Figure 8 shows the decrease order in a resistance of relative humidity that could be ranged in the following order: 9462 kΩ at 11 %RH, 6554 kΩ at 33%RH, 4043 kΩ at 53%RH, 2171 kΩ at 75%RH, 1089 kΩ at 84%RH, and 468 kΩ at 98%RH. The sample shows a linear response and can be considered for the evaluation of sensing characteristics.
Conclusion
This work represents a nanoporous MOF-76(Nd) for hydrogen storage and humidity sensing applications. Characterization results showed that synthesized MOF-76(Nd) are having rod-like structure with an average length of about 20–40 µm with no agglomeration. The elemental analysis confirmed the presence of BTC and water molecules and EDS map scanning are also indicating the elemental presence of Nd. The thermal stability of prepared MOF-76(Nd) material is after the dehydration process between 150oC and 500oC temperature. MOF-76(Nd) is producing less than 1 wt% of adsorption/desorption capacity at 20 bar and 77 K. This indicates that high-pressure hydrogen storage may be achieved, but it requires an effective activation process which leads to the significant hydrogen storage capacity. Therefore, it is concluded that MOF-76(Nd) needs essential improvements to be an efficient material which could be useful in on-board hydrogen systems. The sample showed a linear response with relative humidity and can be considered for the evaluation of humidity sensing characteristics.
Data Availability Statement
The raw data supporting the conclusions of this article will be made available by the authors, without undue reservation.
Author Contributions
AG helped in data analysis and prepared a manuscript draft. MA helped in synthesis of MOFs, characterization, and data analysis. DP helped in data analysis. EP helped in gas sensing measurements. JL helped in finalization of the manuscript. AS planned the entire experiments and hydrogen storage measurements.
Conflict of Interest
The authors declare that the research was conducted in the absence of any commercial or financial relationships that could be construed as a potential conflict of interest.
Acknowledgments
MA acknowledges the VEGA project No. 1/0745/17, VVGS UPJS project No. 2019-1033, and the Ministry of Education, Science, Research and Sport of the Slovak Republic and the Accreditation Commission for the financial support of the TRIANGEL team in the frame of the scheme “Top Research Teams in Slovakia”.
References
Almáši, M., Zeleňák, V., Opanasenko, M., and Čejka, J. (2014). A novel nickel metal-organic framework with fluorite-like structure: gas adsorption properties and catalytic activity in Knoevenagel condensation. Dalton Trans 43, 3730. doi:10.1039/c3dt52698d
Almáši, M., Zeleňák, V., Opanasenko, M., and Císařová, J. (2015). Ce(III) and Lu(III) metal-organic frameworks with Lewis acid metal sites: preparation, sorption properties and catalytic activity in Knoevenagel condensation. Cat. Today 243, 184. doi:10.1016/j.cattod.2014.07.028
Almáši, M., Zeleňák, V., Kuchár, J., Bourrelly, S., and Llewellyn, P. (2016). New members of MOF-76 family containing Ho(III) and Tm(III) ions: Characterization, stability and gas adsorption properties. Colloids Surf. A: Physicochem. Eng. Aspects 496, 114–124. doi:10.1016/j.colsurfa.2015.10.048
Almáši, M., Zeleňák, V., Palotai, P., Beňová, E., and Zeleňáková, A. (2018b). Metal-organic framework MIL-101(Fe)-NH2 functionalized with different long-chain polyamines as drug delivery system. Inorg. Chem. Commun 93, 115–120. doi:10.1016/j.inoche.2018.05.007
Almáši, M., Zeleňák, V., Gyepes, R., Bourrelly, S., Opanasenko, M. V., Llewellyn, P. L., et al. (2018a). Microporous lead-organic framework for selective CO2 adsorption and heterogeneous catalysis. Inorg. Chem 57, 1774–1786. doi:10.1021/acs.inorgchem.7b02491
Ardelean, O., Blanita, G., Borodi, G., Lazar, M. D., Misan, I., Coldea, I., et al. (2013). Volumetric hydrogen adsorption capacity of densified MIL-101 monoliths. Int. J. Hydrog. Energy 38, 7046–7055. doi:10.1016/j.ijhydene.2013.03.161
Bahreyni, B., and Khoshaman, A. H. (2012). Application of metal organic framework crystals for sensing of volatile organic gases. Sens. Actuators B 162, 114–119. doi:10.1016/j.snb.2011.12.046
Balderas-Xicohtencatl, R., Schlichtenmayer, M., and Hirscher, M. (2018). Volumetric hydrogen storage capacity in metal-organic frameworks. Energy Technol 6, 578–582. doi:10.1002/ente.201700636
Blanita, G., Coldea, I., Misan, I., and Lupu, D. (2014). Hydrogen cryo adsorption by hexagonal prism monoliths of MIL-101. Int. J. Hydrog. Energy 39, 17040–17046. doi:10.1016/j.ijhydene.2014.08.038
Boudaden, J., Steinmaßl, M., Endres, H., Drost, A., Eisele, I., Kutter, C., et al. (2018). Polyimide-based capacitive humidity sensor. Sensors 18, 1516. doi:10.3390/s18051516
Broom, D. P., Webb, C. J., Hurst, K. E., Parilla, P. A., Gennett, T., Brown, C. M., et al. (2016). Outlook and challenges for hydrogen storage in nanoporous materials. Appl. Phys. A 122, 151. doi:10.1007/s00339-016-9651-4
Campbell, M. G., and Dinca, M. (2017). Metal-organic frameworks as active materials in electronic sensor devices. Sensors 17, 1108. doi:10.3390/s17051108
Chen, Z., and Lu, C. (2005). Humidity sensors: a review of materials and mechanisms. Sensor Lett 3, 274. doi:10.1166/sl.2005.045
Dailly, A., and Poirier, E. (2011). Evaluation of an industrial pilot scale densified MOF-177 adsorbent as an on-board hydrogen storage medium. Energy Environ. Sci 4, 3527–3534. doi:10.1039/C1EE01426A
Dong, J., Zhao, D., Lu, Y., and Sun, W. Y. (2019). Photoluminescent metal–organic frameworks and their application for sensing biomolecules. J. Mater. Chem. A 7, 22744. doi:10.1039/C9TA07022B
Fischer, J. K., Sippel, P., Denysenko, D., Lunkenheimer, P., Volkmer, D., and Loidl, A., (2015). Metal-organic frameworks as host materials of confined supercooled liquids. J. Chem. Phys 143, 154505. doi:10.1063/1.4933308
Gustafsson, M., Bartoszewicz, A., Martin-Matute, B., Sun, J., Grins, J., Zhao, T., et al. (2010). Family of highly stable lanthanide metal-organic frameworks: structural evolution and catalytic activity. Chem. Mater 22, 3316–3322. doi:10.1021/cm100503q
Heitzer, H. M., Marks, T. J., and Ratner, M. A. (2016). Computation of dielectric response in molecular solids for high capacitance organic dielectrics. Acc. Chem. Res 49, 1614. doi:10.1021/acs.accounts.6b00173
Hossein-Babaei, F., and Shabani, P. (2014). A gold/organic semiconductor diode for ppm-level humidity sensing. Sens. Actuators B 205, 143–150. doi:10.1016/j.snb.2014.08.061
International Energy Agency (2015). Technology roadmap: hydrogen and fuel cells Paris: OECD/IEAAvailable at: http://www.iea.org/publications/freepublications/publication/TechnologyRoadmapHydrogenandFuelCells.pdf. [Accessed October 2018].
Islam, T., Nimal, A. T., Mittal, U., and Sharma, M. U. (2015). A micro interdigitated thin film metal oxide capacitive sensor for measuring moisture in the range of 175-625 ppm. Sens. Actuators B, 221, 357–364. doi:10.1016/j.snb.2015.06.101
Kreno, L. E., Leong, K., Farha, O. K., Allendorf, M., Van Duyne, R. P., and Hupp, J. T. (2012). Metal-organic framework materials as chemical sensors. Chem. Rev 112, 1105. doi:10.1021/cr200324t
Li, X. J., Wang, L. L., Wang, H. Y., Wang, W. C., Li, K., and Wang, X. C. (2013). Capacitive humidity sensing properties of ZnO cauliflowers grown on silicon nanoporous pillar array. Sens. Actuators B, 177, 740–744. doi:10.1016/j.snb.2012.11.070
Liu, D., Zhang, W., Huang, H., Yang, Q., Xiao, Y., Ma, Q., et al. (2013). A new metal–organic framework with high stability based on zirconium for sensing small molecules. Microp. Mesop. Mater 171, 118–124. doi:10.1016/j.micromeso.2013.01.003
Meek, S. T., Greathouse, J. A., and Allendorf, M. D. (2011). Metal-organic frameworks: a rapidly growing class of versatile nanoporous materials. Adv Mater Weinheim 23, 249. doi:10.1002/adma.201002854
Ming, Y., Purewal, J., Liu, D., Sudik, A., Xu, C., Yang, J., et al. (2014). Thermophysical properties of MOF-5 powders. Microp. Mesop. Mater 185, 235–224. doi:10.1016/j.micromeso.2013.11.015
Mlsna, T., Dissanayake, S., Vanlangenberg, C., and Patel, S. V. (2015). Conducting absorbent composite for parallel plate chemicapacitive microsensors with improved selectivity. Sens. Actuators B 206, 548. doi:10.1016/j.snb.2014.09.069
Pal, M., Saha, D., and Ghara, D. K. (2016). Nanoporous ɣ-alumina based novel sensor to detect trace moisture in high temperature and high-pressure environment. Sens. Actuators B 222, 1043. doi:10.1016/j.snb.2015.09.068
Purewal, J. J., Liu, D., Yang, J., Sudik, A., Siegel, D. J., Maurer, S., et al. (2012). Increased volumetric hydrogen uptake of MOF-5 by powder densification. Int. J. Hydrog. Energy 37, 2723–2727. doi:10.1016/j.ijhydene.2011.03.002
Qiu, S., Liu, J., Sun, F., Zhang, F., Wang, Z., Zhang, R., et al. (2011). In situ growth of continuous thin metal-organic framework film for capacitive humidity sensing. J. Mater. Chem 21, 3775–3778. doi:10.1039/C0JM03123B
Stavila, V., Talin, A. A., and Allendorf, M. D. (2014). MOF-based electronic and opto-electronic devices. Chem. Soc. Rev 43, 5994. doi:10.1039/c4cs00096j
Yao, S., and Zhu, Y. (2014). Wearable multifunctional sensors using printed stretchable conductors made of silver nanowires. Nanoscale 6, 2345. doi:10.1039/c3nr05496a
Zacharia, R., Cossement, D., Lafi, L., and Chahine, R. (2010). Volumetric hydrogen sorption capacity of monoliths prepared by mechanical densification of MOF-177. J. Mater. Chem 20, 2145. doi:10.1039/b922991d
Keywords: metal-organic framework, synthesis, characterization, metal-organic framework-76(Nd), hydrogen storage, humidity sensing
Citation: Garg A, Almáši M, Rattan Paul D, Poonia E, Luthra JR and Sharma A (2021) Metal-Organic Framework MOF-76(Nd): Synthesis, Characterization, and Study of Hydrogen Storage and Humidity Sensing. Front. Energy Res. 8:604735. doi: 10.3389/fenrg.2020.604735
Received: 10 September 2020; Accepted: 06 November 2020;
Published: 18 January 2021.
Edited by:
Chhagan Lal, University of Rajasthan, IndiaReviewed by:
Ranjith Kottokkaran, Iowa State University, United StatesAndreas Rossos, University of Ioannina, Greece
Balram Tripathi, University of Puerto Rico, Puerto Rico
Surendar Karwasara, S. S. Jain Subodh P.G. College, India
Copyright © 2021 Garg, Almáši, Rattan Paul, Poonia, Luthra and Sharma. This is an open-access article distributed under the terms of the Creative Commons Attribution License (CC BY). The use, distribution or reproduction in other forums is permitted, provided the original author(s) and the copyright owner(s) are credited and that the original publication in this journal is cited, in accordance with accepted academic practice. No use, distribution or reproduction is permitted which does not comply with these terms.
*Correspondence: Anshu Sharma, YW5zaHVzaHNoYXJkYUBnbWFpbC5jb20=; Miroslav Almáši, bWlyb3NsYXYuYWxtYXNpQHVwanMuc2s=