- 1School of Engineering and Technology, Central Queensland University, Rockhampton, QLD, Australia
- 2Faculty of Engineering and Information Technology, University of Technology, Sydney, Sydney, NSW, Australia
- 3Mechanical Engineering Department, Prince Mohammad Bin Fahd University, Al Khobar, Saudi Arabia
- 4School of Engineering and Technology, Central Queensland University, Melbourne, VIC, Australia
- 5Chittagong University of Engineering and Technology (CUET), Chittagong, Bangladesh
- 6Department of Mechanical Engineering, Politeknik Negeri Medan, Medan, Indonesia
Biodiesels are renewable fuel that may be produced from various feedstock using different techniques. It is endorsed in some countries of the world as a viable substitute to diesel fuel. While biodiesel possesses numerous benefits, the cold flow properties (CFP) of biodiesel in comparison with petro-diesel are significantly less satisfactory. This is due to the presence of saturated and unsaturated fatty acid esters. The poor CFP of biodiesel subsequently affects performance in cold weather and damages the engine fuel system, as well as chokes the fuel filter, fuel inlet lines, and injector nozzle. Previously, attempts were made to minimize the damaging impact of bad cold flow through the reduction of pour point, cloud point, and the cold filter plugging point of biodiesel. This study is focused on the biodiesel CFP-related mechanisms and highlights the factors that initialize and pace the crystallization process. This review indicates that the CFP of biodiesel fuel can be improved by utilizing different techniques. Winterisation of some biodiesel has been shown to improve CFP significantly. Additives such as polymethyl acrylate improved CFP by 3-9 ° C. However, it is recommended that improvement methods in terms of fuel properties and efficiency should be carefully studied and tested before being implemented in industrial applications as this might impact biodiesel yield, cetane number, etc.
Introduction
Biofuels are biomass-derived renewable fuels that have great potential as an alternative to fossil fuels. They are inherently biodegradable, less toxic, oxygenated, and contain negligible amounts of sulfur (Bhuiya et al., 2020; Muhammad et al., 2021; Samani et al., 2021). These properties are the key factors that demonstrate favorable combustion and emission performances as transport grade fuel (Ashraful et al., 2014; Hagos et al., 2016). The main constituents of biodiesel fuels are saturated and unsaturated fatty acid alkyl esters and fatty acid methyl esters (FAME), but the composition and types of fatty acid esters vary based on the sources of feedstocks for biodiesel production.
Feedstocks for biofuel undergo a transesterification reaction to produce biodiesel along with a by-product, glycerine (Figure 1) (Mofijur et al., 2013; Shahabuddin et al., 2013; Mofijur et al., 2014; Mofijur et al., 2016; Rizwanul Fattah et al., 2020). Biodiesel fuels effectively contribute to reducing emissions like carbon dioxide (CO2), carbon monoxide (CO), particulate matters (PM), and total hydrocarbons (THC) when used in diesel engines at acceptable diesel-biodiesel blend ratios (Abdollahi et al., 2020; Mahlia et al., 2020). Although biodiesel typically reduces a variety of emissions, the general trend of combustion of this fuel shows an increase in the emission of oxides of nitrogen (NOx) at all loads of engine operation (Szybist et al., 2007; Lapuerta et al., 2008; Palash et al., 2013; Sahu et al., 2013; Lanjekar and Deshmukh, 2016). The feedstocks for biodiesel production may be sourced from edible oil sources, non-edible oil sources (Mofijur et al., 2013; Mofijur et al., 2020a), waste oils and fats, and advanced microalgae (Mofijur et al., 2019; Fattah et al., 2020).
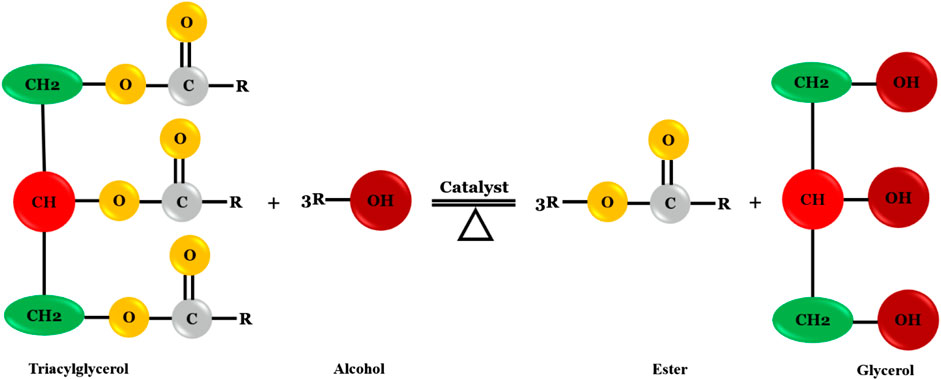
FIGURE 1. Typical transesterification reaction to produce biodiesel (Mofijur et al., 2020b).
Exposure to cold weather causes another dilemma for biodiesel fuels, known as the cold flow or low-temperature flow problem (Zuleta et al., 2012; Islam et al., 2016). Generally, both diesel and biodiesel fuels experience such issues during the winter season and in colder regions of the world; however, the condition is worse with biodiesel. Cloud point (CP) and pour point (PP) are the two primary properties for fuel to determine their cold flow specification (Boros et al., 2009). If blends of diesel and biodiesel fuels are exposed to cold temperatures for a significant period (e.g., parking outside for a few hours at night), the higher CP of the biodiesel could lead to gelation of the biofuel earlier than that of the regular diesel. There are insufficient field-based investigations and detailed reporting about this cold weather effect on biodiesel, based on an assortment of feedstocks from various regions (Dunn, 2009; Monirul et al., 2015; Tinprabath et al., 2016).
Since the sources of feedstocks for biodiesel production varies based on regional availabilities, the compositions of the fatty acid alkyl esters of these fuels also varies due to the disparity of feedstock. Therefore, it is essential to observe all the variations of the physicochemical properties of these fuels due to exposure to specified environmental conditions. Though generally overlooked by biodiesel producers, the CP is an equilibrium attribute of the fuel which can be expressed as linear functions of both the cold filter plugging point (CFPP) and the low-temperature filterability (LTFT) (Dunn and Bagby, 1995; Boros et al., 2009). If the CP of biodiesel is neglected in an environment having a temperature lower than the CP, the number of crystals produced in the fuel will increase with the reduction of temperature. Thus, this will affect fuel viscosity, pouring ability, filtration capacity, and volatility, etc. As a result, fuel starvation could be engendered in the combustion chambers. The engine’s ability for start-up, as well as the drivability, could also be affected due to fuel starvation (Kim et al., 2012; Dwivedi and Sharma, 2014).
It has been reported that a higher amount of saturated fatty acid esters in biodiesel fuels can lead to higher CFPP values in the fuel (Kerschbaum et al., 2008). Biodiesel fuels are unsuitable for use in colder regions because they have a higher measure of saturated fatty acid methyl esters. To be utilized in low-temperature conditions, the biodiesel fuels should have both lower CFPP values and less saturated fatty acid content (Dwivedi and Sharma, 2013). In recent years, researchers around the world have begun adopting several remedial actions. Their studies on cold flow properties and their remedial actions have been published in various journals, yet, not many researchers have reviewed these published findings.
This mini-review aims to make a critical analysis of the cold flow properties of biodiesel and their mechanisms, along with potential remedial techniques to improve cold flow properties. This review is relevant to ongoing studies focused on developing and processing cost-effective biodiesel production. This detailed knowledge of cold flow properties is of great benefit to those working in this important field.
Cold Flow Properties of Biodiesel
The use of biodiesel is affected by poor cold flow properties, namely CP, PP, and CFPP (Dwivedi and Sharma, 2014a). The poor cold flow characteristics of vegetable oils or their alkyl esters lead to injector coking, severe engine deposits, filter gumming, piston ring sticking, and thickening of lubrication oil from long-term use in diesel engines (Dwivedi and Sharma, 2013). Biodiesel should cotain relatively low concentrations of both long-chain saturated fatty acid methyl esters (FAME) and poly-unsaturated FAME to perform well in a low-temperature environment and to maintain stability at acceptable standards (Veríssimo and Gomes, 2011).
Mechanisms of the Cold Flow of Biodiesel
Crystallization occurs in two primary interrelated steps: nucleation and crystal growth (Biodiesel, 2015; Galimzyanov et al., 2019). In the first stage, when the temperature of biodiesel is lower than MP, the liquid molecules will produce a strong intermolecular force, thus generating enough thermodynamic force in the liquid. The molecules of the liquid then gather to form solid embryos called crystal lattices or crystallites. Crystal growth is the formation of lattice after nucleation, and large crystals are formed on the existing lattice (Figure 2). This growth continues until an interlocking network is formed, leading to fuel flow interruption and fuel starvation. This solidification or crystallization in the fuel occurs promptly, resulting in an agglomeration and clogging of both the fuel line and the filters. It causes major problems in engine operation and fuel distribution. The unsaturated fatty acid contents of the biodiesel fuels possess a lower melting point in comparison to the saturated compounds. As a result, the unsaturated compounds can dissolve the saturated compounds and result in gelation at a temperature that is higher than their melting points (Lopes et al., 2008; Ramos et al., 2009; Echim et al., 2012). Accordingly, biodiesel fuels that have more saturated fatty acid compounds (especially, the long-chain saturated esters) display higher CP and PP.
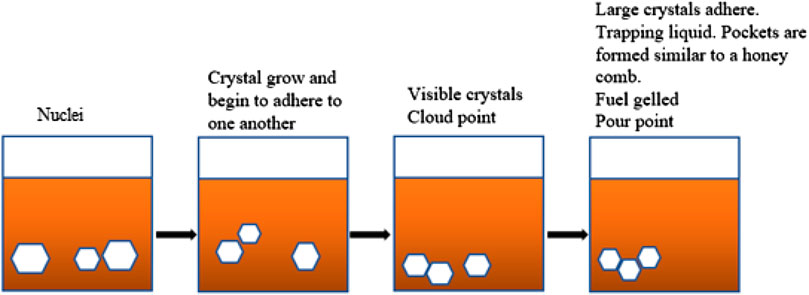
FIGURE 2. Mechanisms of the cold flow of biodiesel (Verma et al., 2016).
Approaches to Improve the Cold Flow Properties of Biodiesel
The cold flow of properties of biodiesel can be improved in a variety of ways. Winterization (Dunn et al., 1996), mixing additives (pour point depressant) (Chiu et al., 2004; Kumar and Singh, 2020), blending with diesel fuels (Magalhães et al., 2019), using branched-chain esters (Lee et al., 1995), blending with vegetable oils of lower crystallization temperature (Foglia et al., 1997), etc. are the more commonly used processes to improve the lower temperature flow properties of the fuel.
Winterization
It is often observed in the winter season (or temperatures lower than CP), that biodiesel fuel experiences crystallization. This results in plugged fuel filters and injectors due to higher CP and PP. Winterization of biofuel involves selective long-chain saturated components crystallization using controlled cooling conditions and removal of the crystallized fractions (Kumar and Singh, 2020). This increases the unsaturated FAME contents of biodiesel and reduces both CP and PP (Dunn et al., 1996; Fuel, 2003; Kerschbaum et al., 2008; Dwivedi and Sharma, 2014b). However, the removal of saturated FAME contents by this process decreases the CN of the fuel, as well as the loss of the total yield of biodiesel, by a significant quantity (Edith et al., 2012). Furthermore, this process may also make fuel susceptible to oxidative damage (Kumar and Singh, 2020). The loss may be minimized when the winterization process is executed after adding cold-flow property improving agents (e.g. hexane, isopropanol, etc.) (Knothe, 2005).
Vijayan et al. (Vijayan et al., 2018) carried out winterization of biodiesel at low temperatures (0°C–20°C) and found that sunflower biodiesel exhibited no crystal formation. Kumar and Sharma (Kumar and Sharma, 2018) used a 3-step winterization process and reported a reduction of CP by 5.5°C and a reduction of PP by 8.2°C. However, the authors also reported a reduction of 5.4%–15% biodiesel yield due to winterization.
Nainwal et al. (Nainwal et al., 2015) conducted winterization tests of Jatropha methyl ester and waste cooking oil methyl ester at a temperature 2°C lower than the respective CP. They observed a reduction of 4.1 wt% and 2.3 wt% of saturated FAMEs, respectively. The CP and PP were reduced by 11.8% and 8.3% for JME, 20.6%, and 22.6% for WCOME, respectively. C18:0 FAME content has a more adverse effect on the low-temperature characteristics of biodiesel than that of C16:0 due to a higher melting point (Moser et al., 2007). A partial winterization process to only remove the C18:0 or higher saturated FAMEs could reduce the quantity of total yield loss from biodiesel but achieve acceptable CFPP values. The use of a cross-flow micro heat exchanger to produce pure crystals of the saturated FAME in the winterization method may be effective in lowering the CFPP of the biodiesel (González Gómez et al., 2002; Kerschbaum et al., 2008). Other analyses in this method showed the reduction of CFPP of WCOME by 11K (Kerschbaum et al., 2008).
Doğan and Temur (Doğan and Temur, 2013) performed winterization of beef tallow biodiesel at 16.3°C, in which about 60 wt% of saturated FAME content was reduced, resulting in a reduction of CP and PP by 4°C and 7°C, respectively. Due to long-chain saturated FAME content (C20:0, C22:0, and C24:0) in peanut biodiesel (CP 20°C, PP 15°C, and CFPP 17°C), it shows a cold flow property with that of palm oil methyl ester (POME) despite having an almost equal amount of saturated FAME similar to soybean methyl ester (SBO) (Pérez et al., 2010).
Using Additives
Mixing suitable additives to improve the low-temperature characteristics of biodiesel is one of the more effective and efficient methodologies. Such additives are known as cold flow improvers (CFI). This process is convenient with significantly less investment (Chiu et al., 2004; Wang et al., 2014). CFI additives modify the agglomerative nature of the crystals in low temperatures, as well as transform their shapes from typical plate to needle-like. These smaller crystals do not face viscous resistance while flowing through the fuel filter and injector (Echim et al., 2012). The crystals are also incapable of forming gelation characteristics that disturb the pouring behavior of the fuel. Generally, the pour point depressant (PPD) type CFI additives are most effective for biodiesel (Chastek, 2011).
To observe the cold flow characteristics of WCOME, Wang et al. (Wang et al., 2014) considered four categories of frequently used PPD type CFI additives, namely: polymethyl acrylate (PMA), ethylene-vinyl acetate copolymer (EVAC), poly-α-olefin (PAO), and polymaleic anhydride (HPMA). Analyses showed that the addition of these CFIs of 0.04 wt% of the WCOME can effectively improve cold flow characteristics by reducing the CP, CFPP, and PP of the fuel. They reported that the effectiveness order of the additives is PMA>EVAC>PAO>HPMA, with the best contribution from PMA. The addition of CFI additives also influences the amelioration of both OSI and kinematic viscosity in favor of biodiesel operability. Leggieri et al. (Leggieri et al., 2018) used dimethyl azelate and triacetin as additives to improve FAME cold flow properties. However, they were only able to improve the CP slightly by 2–3°C. Dimethyl azelate alters the crystal formation to reduce the CP. Monirul et al. (Monirul et al., 2017) used poly(methyl acrylate) (PMA) to improve the cold flow properties of biodiesel-diesel blends. The authors reported that the addition of PMA improved PP, CP, and CFPP by 9, 3, and 8°C respectively. Senra et al. (Senra et al., 2019) studied the impact of glycerol-derived additives on biodiesel cold flow properties. The authors reported that the additives work as dilution, stop crystallization, and improve properties.
It has been shown that the addition of 1% v/v of PLMA to canola biodiesel would result in an optimal effective dose for improvement of the cold flow characteristics (Bozell et al., 2000). Although this fuel already possesses very low CP, PP, and LTFT, this can help to prepare binary or ternary blends with fuels that have higher cold flow parameters, for example, POME. The addition of up to 20% ethyl levulinate (EL), produced from esterification of levulinic acid (4-oxopentanoic acid) and ethanol to cottonseed oil methyl ester (CSOME) and poultry fat methyl ester (PFME) can reduce the CFPP from 5°C to 2°C and 4°C to 1°C, respectively. The ternary mixture of commercial CFI additives DEP, polyglycerol ester (PGE), laboratory-made CFI (PA) at ratios of 3:1:1 as well as 2:2:1 with a total dose content of 1% v/v could reduce the CFPP of POME (CFPP 16°C) by 7°C–i.e., reduced CFPP was found to be 9°C (Lv et al., 2013). This is one instance of the synergistic effect of additives blending to improve the cold flow property of fuel. Cold flow improver additives can also be prepared from the esterified derivatives of oleates and linoleates, or ring-opening reaction products due to epoxidation of these esters to adequately reduce the CFPP of biodiesel (De Torres et al., 2011). Kumar and Sharma (Kumar and Sharma, 2018) blended waste cooking biodiesel with ethanol at 2, 5, 10, 15, and 20% blend ratio. As ethanol contains lower PP and CP, blending with ethanol significantly reduced them for waste cooking biodiesel. The CP was reduced from 14.1 to 6.8°C and PP was reduced to 3.2 from 10.3°C.
Ozonized vegetable oils (0.5–1.5 wt%) could be considered as partially effective CFI additives for biodiesel fuels such as SFME, SBOME, RME, and POME (Soriano et al., 2006). The authors stated that ozonized vegetable oil (oz-VO) derived from the same source can be more effective in reducing the PP of biodiesel fuels–e.g., SFME, SBOME, and RME among others, with less effect on CP reduction. The higher amount of saturated FAME content in POME makes the oz-VO of palm oil ineffective to reduce the PP of POME, but the reduction of CP was observed from 18°C to 12°C. The authors explained through microscopic analysis that, the addition of oz-VO on the respective biodiesel forestalls the accumulation of crystals during temperature reduction by forming smaller regular-shaped crystals. As a result, the flow property is improved for biodiesel fuels.
Blending With Conventional Diesel Fuel
When biodiesel is blended with conventional diesel fuel, the overall quantity of FAME is reduced. Various types of biodiesels were blended effectively with diesel fuel such as palm oil, Jatropha curcas, Ceiba pentandra, Reutealis trisperma, Calophyllum inophyllum, etc (Ong et al., 2014; Dharma et al., 2016; Silitonga et al., 2019; Silitonga et al., 2020). As a result, the total effect of the biodiesel fuel property is changed from that of B100. Kim et al. (Kim et al., 2012) investigated the cold temperature (−16°C and −20°C) effect of various blends (B5, B10, and B20) of biodiesel fuels derived from soybean oil, waste cooking oil, rapeseed oil, cottonseed oil, palm oil, and Jatropha oil in both passenger car and light-duty truck engines. Based on the ‘start ability test’ and ‘drivability test’ for these samples, the researchers found that the B5 blends could pass the test for all of the test conditions. The other blends experienced some problems in these temperature ranges. Bencheikh et al. (Bencheikh et al., 2019) studied the effect of blending diesel with waste cooking biodiesel on cold flow properties. The authors reported that blending 80% diesel with 20% waste cooking biodiesel reduces CP, PP, and CFPP by 17, 21, and 21°C, respectively.
Nainwal et al. (Nainwal et al., 2015) reported that blending high CP and PP based jatropha methyl ester (CP 20.2°C, PP 18°C) as well as waste cooking oil methyl ester (CP 14.5°C, PP 13.7°C) with conventional diesel (DF2, CP 6°C, PP 5°C) at various ratios results in a reduction of CP and PP of the blends. The CP and PP of B20 blends were found to be 14.9°C and 14°C for JME as well as 12°C and 11.5°C for WCOME, respectively. The researchers explained the effect as a cause of the reduction of total saturated FAME in the blend. The addition of JME and WCOME with kerosene (DF1, CP −10°C, PP −11°C) also reduced the CP and PP of the biodiesel blends significantly. The CP and PP of B20 blends were found as −1°C and −2.2°C for JME as well as −10.5°C and 12°C for WCOME, respectively (Nainwal et al., 2015).
Bhale et al. (Bhale et al., 2009) investigated the effect of ethanol and kerosene blends on improving cold flow properties of mahua methyl ester (MME, CP 18°C, PP 7°C) in place of additives. This involved a blend of MME with 20% ethanol, and 20% kerosene for which the CP and PP reduced to 8°C, 5°C, and −4°C, −8°C, respectively. The researchers reported pour point depression from 7°C to −5°C for the mix of 10% ethanol and 10% kerosene blend but did not publish the CP. The PP reduction (from 7°C to −5°C) of MME fuel was comparable to that of adding 2% Lubrizol.
Addition of Branched-Chain Fatty Acid Alkyl Esters
Branched-chain fatty acid methyl esters (BC-FAME) can be used as additives (e.g., methyl iso-oleate and methyl iso-stearate) as well as diluents to reduce the CP and PP of biodiesel (Knothe et al., 2000; Knothe, 2005; Dunn et al., 2015). The saturated BC-FAME (Me iso-C18:0) showed a better effect than that of unsaturated BC-FAME (Me iso-C18:1) to improve the cold flow property of the POME, CME, and SME when used as additives (up to 2%). A similar trend of effectiveness was also reported when these BC-FAMEs were used as diluents. Up to 50% mix of Me-iso-C18:0 as a diluent reduced the CP and PP of POME, CME, and SME up to acceptable ranges. Since there is no comparison of combustion and emission performances of these diluent fuel mixtures, further investigation will be required to assess this method’s economic impact. Other branched-chain esters, e.g. isopropyl as well as 2-butyl esters of fats and oils, typically have better low-temperature flow properties than their corresponding straight-chain isomers (Knothe et al., 2000).
Etherification of glycerol by-product from the biodiesel production process leads to the production of ethers of glycerol, which is then mixed with the pure biodiesel. As a result, the CP of the fuel reduces to lower than 0°C, approximately −5°C without the addition of any CP reducing reagents. The authors (Noureddini, 2001) also found that the di- and tri-ether glycerol had better performance in reducing the cold flow property of the biodiesel. An overall dose of 12% ethers mixed with 88% methyl soyate can achieve the above-mentioned CP.
Blending of Biodiesels
To solve the problem of poor biodiesel properties, the blending of biodiesels is a simple but effective method. Biodiesels of various FAME profiles are blended to achieve the acceptable CP, CFPP and PP for a particular regional application in the engines. The blending of different types of biodiesels can resolve the shortcoming of some biodiesels to be used in the low-temperature environment (Silitonga et al., 2013; Silitonga et al., 2018; Ong et al., 2019). For instance, palm: rapeseed: soybean blends of 20:60:20, 20:80:0, 40:20:40, 40:40:20, and 40:60:0 showed CFPP of −6°C, −5°C, −2°C, −3°C, and −3°C, respectively (Park et al., 2008). Among these blends, the ternary blend of 20:60:20 (palm: rapeseed: soybean) shows the best cold flow property by making good use of the unfavorable palm (CFPP 11°C) and soybean (CFPP −3°C) oils with rapeseed (CFPP −20°C).
As per the data provided (Park et al., 2008), the total unsaturated FAME content of palm, rapeseed, and soybean biodiesel fuels are 54.26%, 92.88%, and 83.16%, respectively; for which the CFPP value from the above correlations could be found as 9.58°C, −19.172°C, and −4.53°C. The correlations show the variation of −12.9%, −4.14%, and +51% of the reported CFPP values for palm, rapeseed, and soybean biodiesel, respectively. Blending methyl oleate (MO) with POME also reduces the CP and CFPP of the fuel but reduces the OS of the fuel (Altaie et al., 2015; Dunn, 1999). Altaie et al., (2015) observed that the 50:50 (POME: MO) blend can significantly improve the cold flow behavior of POME by reducing its CP from 18°C to 5.33°C and CFPP from 16°C to 1.33°C.
Sbihi et al., (2018) reported that cold flow properties depended upon the saturated and unsaturated FAMEs. Saturated FAMEs have a higher melting point that deteriorates the cold flow properties. In order to reduce the saturated FAMEs, the authors blended Citrillus colocynthis oil biodiesel and Camelus dromedaries fat biodiesel. They reported that the blend reduced the saturated FAMEs and as a result, there was a significant improvement of cold flow properties. Furthermore, the authors also reported an improvement in cetane numbers due to blending.
Ghanei, (2014) blended castor oil methyl ester with conventional diesel fuel, canola methyl ester, and waste frying oil methyl ester to investigate the low-temperature properties CP and PP. The reference value for the blending ratio was determined based on the kinematic viscosity (≤6 mm2/s) at 40°C as per the ASTM D-6751 standard. Based on the investigation, the maximum amount of castor oil methyl ester content in the diesel, canola methyl ester, and frying oil methyl ester are 48%, 35%, and 58%, respectively. The CP of these blends were found as −9.57°C, −6.50°C, and −5.71°C, respectively, and the PP values, are found as −22.20°C, −14.14°C and −13.35°C, respectively, for castor-diesel, castor-canola biodiesel, and castor-frying oil biodiesel blends. This blending technique seems effective, as the process of blending the biodiesel fuels not only improved the low-temperature flow characteristics of the fuel but also kept the viscosity within the standard limit. As a result, the process of improving one property would not have an adverse effect on another fuel property.
Conclusion
Based on the information presented in this article, it can be clearly stated that both the oxidation stability and cold filter plugging point are the two key characteristics of biodiesel fuel. Both are dependent on the structure and quantity of FAMEs in the biodiesel but in a contrary manner. The cold flow properties have been observed as a considerable characteristic of fuel only in cold regions. Therefore, thermally susceptible fuels should be treated well by any of the effective methodologies presented here. In addition, the mixing of various methodologies could be significantly acceptable to optimize the result due to the necessity of using the most stable fuel in any environment. Winterisation of biodiesel resulted in the reduction of saturated fatty acid and thus improved cold flow properties. However, sometimes it may also influence biodiesel yield and the cetane numbers negatively. The polymethyl acrylate (PMA) additive improved the CFP by 3–9°C. Both the blending and mixing of CFI additives present potential candidates for improving the cold flow properties of any fuel or fuel blends. This review includes substantial knowledge that may aid in the selection of proper biodiesel for blending. In order to obtain both stable and improved low-temperature flow in biodiesel fuels, a combined methodology of blending for oxidation stability improvement and then the use of a CFI additive could be the most economical solutions.
Author Contributions
MH: Writing original draft, MR: Supervision, MK: Supervision, FD: Supervision, AA: Writing original draft, MM: Writing original draft, AS: Review and editing, MB: Review and editing.
Conflict of Interest
The authors declare that the research was conducted in the absence of any commercial or financial relationships that could be construed as a potential conflict of interest.
References
Abdollahi, M., Ghobadian, B., Najafi, G., Hoseini, S. S., Mofijur, M., and Mazlan, M. (2020). Impact of water – biodiesel – diesel nano-emulsion fuel on performance parameters and diesel engine emission. Fuel 280, 118576. doi:10.1016/j.fuel.2020.118576
Altaie, M. A., Janius, R. B., Rashid, U., Taufiq Yap, Y. H., Yunus, R., and Zakaria, R. (2015). Cold flow and fuel properties of methyl oleate and palm-oil methyl ester blends. Fuel 160, 238–244. doi:10.1016/j.fuel.2015.07.084
Ashraful, A. M., Masjuki, H. H., Kalam, M. A., Rizwanul Fattah, I. M., Imtenan, S., Shahir, S. A, et al. (2014). Production and comparison of fuel properties, engine performance, and emission characteristics of biodiesel from various non-edible vegetable oils: a review. Energy Convers. Manag. 80, 202–228. doi:10.1016/j.enconman.2014.01.037
Bencheikh, K., Atabani, A. E., Shobana, S., Mohammed, M. N., Uğuz, G., and Arpa, O. (2019). Fuels properties, characterizations and engine and emission performance analyses of ternary waste cooking oil biodiesel–diesel–propanol blends. Susta. Energy Technol. Asses. 35, 321–334. doi:10.1016/j.seta.2019.08.007
Bhale, P. V., Deshpande, N. V., and Thombre, S. B. (2009). Improving the low temperature properties of biodiesel fuel. Renew. Energy 34, 794–800. doi:10.1016/j.renene.2008.04.037
Bhuiya, M. M. K., Rasul, M., Khan, M., Ashwath, N., and Mofijur, M. (2020). Comparison of oil extraction between screw press and solvent (n-hexane) extraction technique from beauty leaf (Calophyllum inophyllum L.) feedstock. Ind. Crop. Prod. 144, 112024. doi:10.1016/j.indcrop.2019.112024
Boros, L., Batista, M. L. S., Vaz, R. V., Figueiredo, B. R., Fernandes, V. F. S., and Costa, M. C. (2009). Crystallization behavior of mixtures of fatty acid ethyl esters with ethyl stearate. Energy Fuel. 23, 4625–4629. doi:10.1021/ef900366y
Bozell, J. J., Moens, L., Elliott, D. C., Wang, Y., Neuenscwander, G. G., and Fitzpatrick, S. W. (2020). Production of levulinic acid and use as a platform chemical for derived products. Resour. Conserv. Recycl. 28, 227–239. doi:10.1016/S0921-3449(99)00047-6
Chastek, T. Q. (2011). Improving cold flow properties of canola-based biodiesel. Biomass Bioenergy 35, 600–607. doi:10.1016/j.biombioe.2010.10.024
Chiu, C-W., Schumacher, L. G., and Suppes, G. J. (2004). IImpact of cold flow improvers on soybean biodiesel blend. Biomass Bioenergy 27, 485–491. doi:10.1016/j.biombioe.2004.04.006
De Torres, M., Jiménez-Osés, G., Mayoral, J. A., and Pires, E. (2011). Fatty acid derivatives and their use as CFPP additives in biodiesel. Bioresour. Technol. 102, 2590–2594. doi:10.1016/j.biortech.2010.10.004
Dharma, S., Masjuki, H. H., Ong, H. C., Sebayang, A. H., Silitonga, A. S., Kusumo, F, et al. (2016). Optimization of biodiesel production process for mixed Jatropha curcas-Ceiba pentandra biodiesel using response surface methodology. Energy Convers. Manag. 115, 178–190. doi:10.1016/j.enconman.2016.02.034
Doğan, T. H., and Temur, H. (2013). Effect of fractional winterization of beef tallow biodiesel on the cold flow properties and viscosity. Fuel 108, 793–796. doi:10.1016/j.fuel.2013.02.028
Dunn, R. (1999). Thermal analysis of alternative diesel fuels from vegetable oils. J. Am. Oil Chem. Soc. 76, 109–115. doi:10.1007/s11746-999-0056-9
Dunn, R. O., and Bagby, M. O. (1995). Low-temperature properties of triglyceride-based diesel fuels: transesterified methyl esters and petroleum middle distillate/ester blends. J. Am. Oil Chem. Soc. 72, 895–904. doi:10.1007/BF02542067
Dunn, R. O. (2009). Effects of minor constituents on cold flow properties and performance of biodiesel. Prog. Energy Combust. Sci. 35, 481–489. doi:10.1016/j.pecs.2009.07.002
Dunn, R. O., Ngo, H. L., and Hass, M. J. (2015). Branched-chain fatty acid methyl esters as cold flow improvers for biodiesel. J. Am. Oil Chem. Soc. 92, 853–869. doi:10.1007/s11746-015-2643-2
Dunn, R. O., Shockley, M. W., and Bagby, M. O. (1996). Improving the low-temperature properties of alternative diesel fuels: vegetable oil-derived methyl esters. J. Am. Oil Chem. Soc. 73, 1719–1728. doi:10.1007/BF02517978
Dwivedi, G., and Sharma, M. P. (2013). Cold flow behavior of biodiesel- A review. Int. J. Renew. Energy Resour. 3, 827–836.
Dwivedi, G., and Sharma, M. P. (2014a). Impact of cold flow properties of biodiesel on engine performance. Renew. Sustain. Energy Rev. 31, 650–656. doi:10.1016/j.rser.2013.12.035
Dwivedi, G., and Sharma, M. P. (2014b). Prospects of biodiesel from pongamia in India. Renew. Sustain. Energy Rev. 32, 114–122. doi:10.1016/j.rser.2014.01.009
Echim, C., Maes, J., and Greyt, W. D. (2012). Improvement of cold filter plugging point of biodiesel from alternative feedstocks. Fuel 93, 642–648. doi:10.1016/j.fuel.2011.11.036
Edith, O., Janius, R. B., and Yunus, R. (2012). Factors affecting the cold flow behaviour of biodiesel and methods for improvement – a review. Pertanika J. Sci. Technol. 20, 1–14.
Fattah, I. M. R., Noraini, M. Y., Mofijur, M., Silitonga, A. S., Badruddin, I. A., and Khan, T. M. Y. (2020). Lipid extraction maximization and enzymatic synthesis of biodiesel from microalgae. Appl. Sci. 10, 6103. doi:10.3390/app10176103
Foglia, T. A., Nelson, L. A., Dunn, R. O., and Marmer, W. N. (1997). Low-temperature properties of alkyl esters of tallow and grease. J. American Oil Chem. Soc. 74, 951–955.
Fuel (2003). Standard (Biodiesel) determination the biodiesel fuel quality standard. Australia: Department of the Environment.
Galimzyanov, B. N., Ladyanov, V. I., and Mokshin, A. V. (2019). Remarkable nuances of crystallization: from ordinary crystal nucleation to rival mechanisms of crystallite coalescence. J. Crystal Growth 526, 125214. doi:10.1016/j.jcrysgro.2019.125214
Ghanei, R. (2014). Improving cold-flow properties of biodiesel through blending with nonedible castor oil methyl ester. Environ. Prog. Sustain. Energy 34, 897–902. doi:10.1002/ep.12049
González Gómez, M. E., Howard-Hildige, R., Leahy, J. J., and Rice, B. (2002). Winterisation of waste cooking oil methyl ester to improve cold temperature fuel properties. Fuel 81, 33–39. doi:10.1016/S0016-2361(01)00117-X
Hagos, F. Y., Ali, O. M., Mamat, R., and Abdullah, A. A. (2016). Effect of emulsification and blending on the oxygenation and substitution of diesel fuel for compression ignition engine. Renew. Sustain. Energy Rev. 75, 1281–1294. doi:10.1016/j.rser.2016.11.113
Islam, M. M., Hassan, M. H., Kalam, M. A., Zulkifli, N. W., Habibullah, M., and Hossain, M. M. (2016). Improvement of cold flow properties of Cocos nucifera and Calophyllum inophyllum biodiesel blends using polymethyl acrylate additive. J. Clean. Prod. 137, 322–329. doi:10.1016/j.jclepro.2016.07.080
Kerschbaum, S., Rinke, G., and Schubert, K. (2008). Winterization of biodiesel by micro process engineering. Fuel 87, 2590–2597. doi:10.1016/j.fuel.2008.01.023
Kim, J. K., Yim, E. S., Jeon, C. H., Jung, C. S., and Han, B. H. (2012). Cold performance of various biodiesel fuel blends at low temperature. Int. J. Automot. Technol. 13, 293–300. doi:10.1007/s12239-012-0027-2
Knothe, G. (2005). Dependence of biodiesel fuel properties on the structure of fatty acid alkyl esters. Fuel Process. Technol. 86, 1059–1070. doi:10.1016/j.fuproc.2004.11.002
Knothe, G., Dunn, R., Shockley, M., and Bagby, M. (2000). Synthesis and characterization of some long-chain diesters with branched or bulky moieties. J. Am. Oil Chem. Soc. 77, 865–871. doi:10.1007/s11746-000-0138-x
Kumar, D., and Singh, B. J. F. (2020). Effect of winterization and plant phenolic-additives on the cold-flow properties and oxidative stability of Karanja biodiesel. Fuel 262, 116631. doi:10.1016/j.fuel.2019.116631
Kumar, M., and Sharma, M. P. (2018). Investigating and improving the cold flow properties of waste cooking biodiesel using winterization and blending. Mater. Today: Proceedings. 5, 23051–23056. doi:10.1016/j.matpr.2018.11.034
Lanjekar, R. D., and Deshmukh, D. (2016). A review of the effect of the composition of biodiesel on NOx emission, oxidative stability and cold flow properties. Renew. Sustain. Energy Rev. 54, 1401–1411. doi:10.1016/j.rser.2015.10.034
Lapuerta, M., Armas, O., and Rodríguez-Fernández, J. (2008). Effect of biodiesel fuels on diesel engine emissions. Prog. Energy Combust. Sci. 34, 198–223. doi:10.1016/j.ejpe.2019.03.001
Lee, I., Johnson, L. A., and Hammond, E. G. (1995). Use of branched-chain esters to reduce the crystallization temperature of biodiesel. J. American Oil Chem. Soc. 72, 1155–1160.
Leggieri, P. A., Senra, M., and Soh, L. (2018). Cloud point and crystallization in fatty acid ethyl ester biodiesel mixtures with and without additives. Fuel 222, 243–249. doi:10.1016/j.fuel.2018.02.100
Lopes, J. C. A., Boros, L., Krähenbühl, M. A., Meirelles, A. J. A., Daridon, J. L., and Pauly, J. (2008). Prediction of cloud points of biodiesel. Energy Fuel. 22, 747–752. doi:10.1021/ef700436d
Lv, P., Cheng, Y., Yang, L., Yuan, Z., Li, H., and Luo, W. (2013). Improving the low temperature flow properties of palm oil biodiesel: addition of cold flow improver. Fuel Process. Technol. 110, 61–64. doi:10.1016/j.fuproc.2012.12.014
Magalhães, A. M., Pereira, E., Meirelles, A. J., Sampaio, K. A., and Maximo, G. J. J. F. (2019). Proposing blends for improving the cold flow properties of ethylic biodiesel. Fuel 253, 50–59. doi:10.1016/j.fuel.2019.04.129
Mahlia, T. M. I., Syazmi, Z. A. H. S., Mofijur, M., Abas, A. E. P., Bilad, M. R., Ong, H. C, et al. (2020). Patent landscape review on biodiesel production: technology updates. Renew. Sustain. Energy Rev. 118, 109526. doi:10.1016/j.rser.2019.109526
Mofijur, M., Atabani, A. E., Masjuki, H. H., Kalam, M. A., and Masum, B. M. (2013). A study on the effects of promising edible and non-edible biodiesel feedstocks on engine performance and emissions production: a comparative evaluation. Renew. Sustain. Energy Rev. 23, 391–404. doi:10.1016/j.rser.2013.03.009
Mofijur, M., Masjuki, H. H., Kalam, M. A., and Atabani, A. E. (2013). Evaluation of biodiesel blending, engine performance and emissions characteristics of Jatropha curcas methyl ester: Malaysian perspective. Energy 55, 879–887. doi:10.1016/j.energy.2013.02.059
Mofijur, M., Masjuki, H. H., Kalam, M. A., Atabani, A. E., Fattah, I. M. R., and Mobarak, H. M. (2014). Comparative evaluation of performance and emission characteristics of Moringa oleifera and Palm oil based biodiesel in a diesel engine. Ind. Crop. Prod. 53, 78–84. doi:10.1016/j.indcrop.2013.12.011
Mofijur, M., Rasul, M. G., Hassan, N. M. S., and Nabi, M. N. (2019). Recent development in the production of third generation biodiesel from microalgae. Energy Procedia 156, 53–58. doi:10.1016/j.egypro.2018.11.088
Mofijur, M., Rasul, M. G., Hyde, J., Azad, A. K., Mamat, R., and Bhuiya, M. M. K. (2016). Role of biofuel and their binary (diesel–biodiesel) and ternary (ethanol–biodiesel–diesel) blends on internal combustion engines emission reduction. Renew. Sustain. Energy Rev. 53, 265–278. doi:10.1016/j.rser.2015.08.046
Mofijur, M., Kusumo, F., Fattah, I. M. R., Mahmudul, H. M., Rasul, M. G., Shamsuddin, A. H., et al. (2020a). Resource recovery from waste coffee grounds using ultrasonic-assisted technology for Bioenergy production. Energies 13, 1770. doi:10.3390/en13071770
Mofijur, M., Siddiki, S. Y. A., Shuvho, M. B. A., Djavanroodi, F., Fattah, I. M. R., and Ong, H. C. (2020b). Effect of nanocatalysts on the transesterification reaction of first, second and third generation biodiesel sources- A mini-review. Chemosphere 128642. doi:10.1016/j.chemosphere.2020.128642
Monirul, I., Kalam, M., Masjuki, H., Zulkifli, N., Shahir, S., Mosarof, M, et al. (2017). Influence of poly (methyl acrylate) additive on cold flow properties of coconut biodiesel blends and exhaust gas emissions. Renewable energy 101, 702–712. doi:10.1016/j.renene.2016.09.020
Monirul, I. M., Masjuki, H. H., Kalam, M. A., Zulkifli, N. W. M., Rashedul, H. K., and Rashed, M. M. (2015). A comprehensive review on biodiesel cold flow properties and oxidation stability along with their improvement processes. RSC Adv. 5, 86631–86655. doi:10.1039/c5ra09555g
Moser, B. R., Haas, M. J., Winkler, J. K., Jackson, M. A., Erhan, S. Z., and List, G. R. (2007). Evaluation of partially hydrogenated methyl esters of soybean oil as biodiesel. Eur. J. Lipid Sci. Technol. 109, 17–24. doi:10.1002/ejlt.200600215
Muhammad, G., Alam, M. A., Mofijur, M., Jahirul, M. I., Lv, Y., and Xiong, W. (2021). Modern developmental aspects in the field of economical harvesting and biodiesel production from microalgae biomass. Renew. Sustain. Energy Rev. 135, 110209. doi:10.1016/j.rser.2020.110209
Nainwal, S., Sharma, N., Sharma, A. S., Jain, S., and Jain, S. (2015). Cold flow properties improvement of Jatropha curcas biodiesel and waste cooking oil biodiesel using winterization and blending. Energy. 89, 702–707. doi:10.1016/j.energy.2015.05.147
Noureddini, H. (2001). “System and process for producing biodiesel fuel with reduced viscosity and a cloud point below thirty-two (32) degrees fahrenheit.”in Papers in biomaterials, chemical and biomolecular engineering research and publications. United States: The Board of Regents of the University of Nebraska, 1–35.
Ong, H. C., Masjuki, H. H., Mahlia, T. M. I., Silitonga, A. S., Chong, W. T., and Yusaf, T. (2014). Engine performance and emissions using Jatropha curcas, Ceiba pentandra and Calophyllum inophyllum biodiesel in a CI diesel engine. Energy 69, 427–445. doi:10.1016/j.energy.2014.03.035
Ong, H. C., Milano, J., Silitonga, A. S., Hassan, M. H., Shamsuddin, A. H., and Wang, C. T. (2019). Biodiesel production from Calophyllum inophyllum-Ceiba pentandra oil mixture: optimization and characterization. J. Clean. Prod. 219, 183–198. doi:10.1016/j.jclepro.2019.02.048
Pérez, A., Casas, A., Fernández, C. M., Ramos, M. J., and Rodríguez, L. (2010). Winterization of peanut biodiesel to improve the cold flow properties. Bioresour. Technol. 101, 7375–7381. doi:10.1016/j.biortech.2010.04.063
Palash, S. M., Kalam, M. A., Masjuki, H. H., Masum, B. M., Rizwanul Fattah, I. M., and Mofijur, M. (2013). Impacts of biodiesel combustion on NOx emissions and their reduction approaches. Renew. Sustain. Energy Rev. 23, 473–490. doi:10.1016/j.rser.2013.03.003
Park, J. Y., Kim, D. K., Lee, J. P., Park, S. C., Kim, Y. J., and Lee, J. S. (2008). Blending effects of biodiesels on oxidation stability and low temperature flow properties. Bioresour. Technol. 99, 1196–1203. doi:10.1016/j.biortech.2007.02.017
Ramos, M. J., Fernández, C. M., Casas, A., Rodríguez, L., and Pérez, A. (2009). Influence of fatty acid composition of raw materials on biodiesel properties. Bioresour. Technol. 100, 261–268. doi:10.1016/j.biortech.2008.06.039
Rizwanul Fattah, I. M., Ong, H. C., Mahlia, T. M. I., Mofijur, M., Silitonga, A. S., Rahman, S. M. A, et al. (2020). State of the art of catalysts for biodiesel production. Frontiers in Energy Research 8, 101. doi:10.3389/fenrg.2020.00101
Sahu, J. N., Mahalik, K. K., Nam, H. K., Ling, T. Y., Woon, T. S., and bin Abdul Rahman, M. S. (2013). Feasibility study for catalytic cracking of waste plastic to produce fuel oil with reference to Malaysia and simulation using ASPEN plus. Environ. Prog. Sustain. Energy 33, 298–307. doi:10.1002/ep.11748
Samani, B. H., Behruzian, M., Najafi, G., Fayyazi, E., Ghobadian, B., and Behruzian, A. (2021). The rotor-stator type hydrodynamic cavitation reactor approach for enhanced biodiesel fuel production. Fuel 283, 118821. doi:10.1016/j.fuel.2020.118821
Sbihi, H. M., Nehdi, I. A., Mokbli, S., Romdhani-Younes, M., and Al-Resayes, S. I. (2018). Study of oxidative stability and cold flow properties of Citrillus colocynthis oil and Camelus dromedaries fat biodiesel blends. Indust. Crops Product. 122, 133–141. doi:10.1016/j.indcrop.2018.05.071
Senra, M., McCartney, S. N., and Soh, L. (2019). The effect of bio-derived additives on fatty acid methyl esters for improved biodiesel cold flow properties. Fuel 242, 719–727. doi:10.1016/j.fuel.2019.01.086
Shahabuddin, M., Liaquat, A. M., Masjuki, H. H., Kalam, M. A., and Mofijur, M. (2013). Ignition delay, combustion and emission characteristics of diesel engine fueled with biodiesel. Renew. Sustain. Energy Rev. 21, 623–632. doi:10.1016/j.rser.2013.01.019
Silitonga, A., Shamsuddin, A., Mahlia, T., Milano, J., Kusumo, F., and Siswantoro, J. (2020). Biodiesel synthesis from Ceiba pentandra oil by microwave irradiation-assisted transesterification: ELM modeling and optimization. Renew. Energy 146, 1278–1291. doi:10.1016/j.renene.2019.07.065
Silitonga, A. S., Mahlia, T. M. I., Kusumo, F., Dharma, S., Sebayang, A. H., Sembiring, R. W, et al. (2019). Intensification of Reutealis trisperma biodiesel production using infrared radiation: simulation, optimisation and validation. Renew. Energy 133, 520–527. doi:10.1016/j.renene.2018.10.023
Silitonga, A. S., Masjuki, H. H., Mahlia, T. M. I., Ong, H. C., Chong, W. T., and Boosroh, M. H. (2013). Overview properties of biodiesel diesel blends from 1 edible and non-edible feedstock. Renew. Sustain. Energy Rev. 22, 346–360. doi:10.1016/j.rser.2013.01.055
Silitonga, A. S., Masjuki, H. H., Ong, H. C., Sebayang, A. H., Dharma, S., and Kusumo, F. (2018). Evaluation of the engine performance and exhaust emissions of biodiesel-bioethanol-diesel blends using kernel-based extreme learning machine. Energy 159, 1075–1087. doi:10.1016/j.energy.2018.06.202
Soriano, N. U., Migo, V. P., and Matsumura, M. (2006). Ozonized vegetable oil as pour point depressant for neat biodiesel. Fuel 85, 25–31. doi:10.1016/j.fuel.2005.06.006
Szybist, J. P., Song, J., Alam, M., and Boehman, A. L. (2007). Biodiesel combustion, emissions and emission control. Fuel Process. Technol. 88, 679–691. doi:10.1016/j.fuproc.2006.12.008
Tinprabath, P., Hespel, C., Chanchaona, S., and Foucher, F. (2016). Impact of cold conditions on diesel injection processes of biodiesel blends. Renew. Energy 96, 270–280. doi:10.1016/j.renene.2016.04.062
Veríssimo, M. I. S., and Gomes, M. T. (2011). Assessment on the use of biodiesel in cold weather: pour point determination using a piezoelectric quartz crystal. Fuel 90, 2315–2320. doi:10.1016/j.fuel.2011.02.034
Verma, P., Sharma, M. P., and Dwivedi, G. (2016). Evaluation and enhancement of cold flow properties of palm oil and its biodiesel. Energy Reports 2:8–13. doi:10.1016/j.egyr.2015.12.001
Vijayan, S. K., Naveena Victor, M., Sudharsanam, A., Chinnaraj, V. K., and Nagarajan, V. (2018). Winterization studies of different vegetable oil biodiesel. Bioresource Tech. Rep. 1, 50–55. doi:10.1016/j.biteb.2018.02.005
Wang, J., Cao, L., and Han, S. (2014). Effect of polymeric cold flow improvers on flow properties of biodiesel from waste cooking oil. Fuel 117, 876–881. doi:10.1016/j.fuel.2013.10.006
Keywords: renewable energy, feedstock, fuel characteristics, biodiesel, cold flow improver
Citation: Hazrat MA, Rasul MG, Mofijur M, Khan MMK, Djavanroodi F, Azad AK, Bhuiya MMK and Silitonga A (2020) A Mini Review on the Cold Flow Properties of Biodiesel and its Blends. Front. Energy Res. 8:598651. doi: 10.3389/fenrg.2020.598651
Received: 25 August 2020; Accepted: 28 October 2020;
Published: 18 December 2020.
Edited by:
Eriola Betiku, Obafemi Awolowo University, NigeriaReviewed by:
Michael Senra, Lafayette College, United StatesAugustine Ayeni, Covenant University, Nigeria
Copyright © 2020 Hazrat, Rasul, Mofijur, Djavanroodi, Khan, Azad, Bhuiya and Silitonga. This is an open-access article distributed under the terms of the Creative Commons Attribution License (CC BY). The use, distribution or reproduction in other forums is permitted, provided the original author(s) and the copyright owner(s) are credited and that the original publication in this journal is cited, in accordance with accepted academic practice. No use, distribution or reproduction is permitted which does not comply with these terms.
*Correspondence: M. A. Hazrat, aC5hbGlAY3F1LmVkdS5hdQ==; YWxpaGF6cmF0MjBAZ21haWwuY29t