- Department of Mechanical and Aerospace Engineering, Politecnico di Torino, Turin, Italy
According to the European Commission, sea waves have a great potential as renewable energy source. Despite wave energy technology is a field in continuous development, it is not yet competitive with the other renewables, due to the small quantities of devices sold, most of them being prototypal solutions at level. So far, various Wave Energy Converter concepts have been developed and some of them tested in full scale. The most recurrent test environment is the North Atlantic Ocean, which possesses high energy potential. The Mediterranean Sea on the other hand is less energetic, but also possesses less dangerous extreme conditions. It represents a favorable starting point to develop technologies that later will be scaled up to more powerful sites. This article illustrates the wave energy potential of the Mediterranean and analyses the wave energy converters engineered according to sea states characteristic of the Mediterranean Sea. Focus is brought to the Inertial Sea Wave Energy Converter (ISWEC) technology, which is one of the few Mediterranean concept to have reached Technology Readiness Level (TRL) 7. The article will document the deployment and the following open sea test campaign of a full-scale prototype off the shore of Pantelleria Island, Italy.
Introduction
The sea has always exerted its inevitable fascination on human beings. Waves that break on cliffs or on a beach allow to grasp the power available in this continuous surface movement. This continuous power, so much distributed in the World and relatively easy to access, makes wave energy an interesting phenomenon, so to be counted among the most promising renewable energies (European Commission, 2012). It is precisely from the comparison with other forms of energy that the exploitation of this form of energy can be responded to and motivated. The different power densities of some of the most well-known renewables can be explained with the wave-forming process: waves are mainly generated by the interaction between the wind and the sea surface; constant mechanical action of the wind, acting as a tangential effort, leads to the formation of waves (World Meteorological Organization, 1998). The wind itself is a derivative of solar energy.
The process of these physical phenomena brings with it an increase in the energy density, seen as the available resource power per meter. About the geographical distribution of the resource, we can take two different approaches:
- On one hand onshore devices such as wind, solar, and others give the possibility of having the source close to the place of use, to the network, so distributed and easily accessible to operators of maintenance.
- On the other hand, wave energy from the sea gives the possibility to avoid land use, which is widely discussed from public opinion. Installations could be in fact in areas close to the coast unused or of low tourist value, or at a distance enough to eliminate the visual impact for man.
Another factor of comparison concerns predictability: the wave resource is characterized by a high degree of predictive reliability with respect to solar and wind resources. On this purpose, several theoretical aspects involved in the estimation of wave energy statistics have been developed to represent the source in terms of mean wave power and return value of typical and extreme events in various coastal areas (Arena et al., 2015). This makes easier the integration of renewable energy in the continental or insular power grid and it consists in a reliable generation node in smart grids.
One last consideration lies in the availability of the resource: wave energy can be harmoniously integrated with solar and wind systems. Indeed, a very rough sea state usually is not contemporary with a day of intense sunshine and frequently comes as a result of an intensive wind phase. This allows to create an integrated energy mix, distributed over time.
It is worth to consider that nowadays technological achievements and energy potential in the Mediterranean Sea of some of the other well-known blue energy sources, in order to understand the advantageous characteristics of the wave energy in comparison to them:
- Tidal range and current: present a low power density in Mediterranean Sea as discussed by Soukissian et al. (2017) with some exception for Straits of Dardanelles, of Gibraltar and Strait of Messina.
- Salinity gradients: although some specific sites in Mediterranean Sea are suitable there is no technology currently developed to exploit this energy source (Alvarez-Silva et al., 2016).
- Thermal difference: a thermal difference of 20°C is needed to guarantee a good efficiency of the device. In Mediterranean Sea the temperature difference between sea surface and 1,000 m depth is below than 12°C (Soukissian et al., 2017).
The ENEA wave analysis forecast reported in Figure 1 shows that the Mediterranean wave potential, is regard to Italy, is mainly concentrated on the Italian West coasts, in particular Sardegna, then the Channel of Sicily. Indeed, the Mediterranean basin is almost surrounded by mountain ranges except for France where the wind can freely blow from west to east under the Coriolis effect.
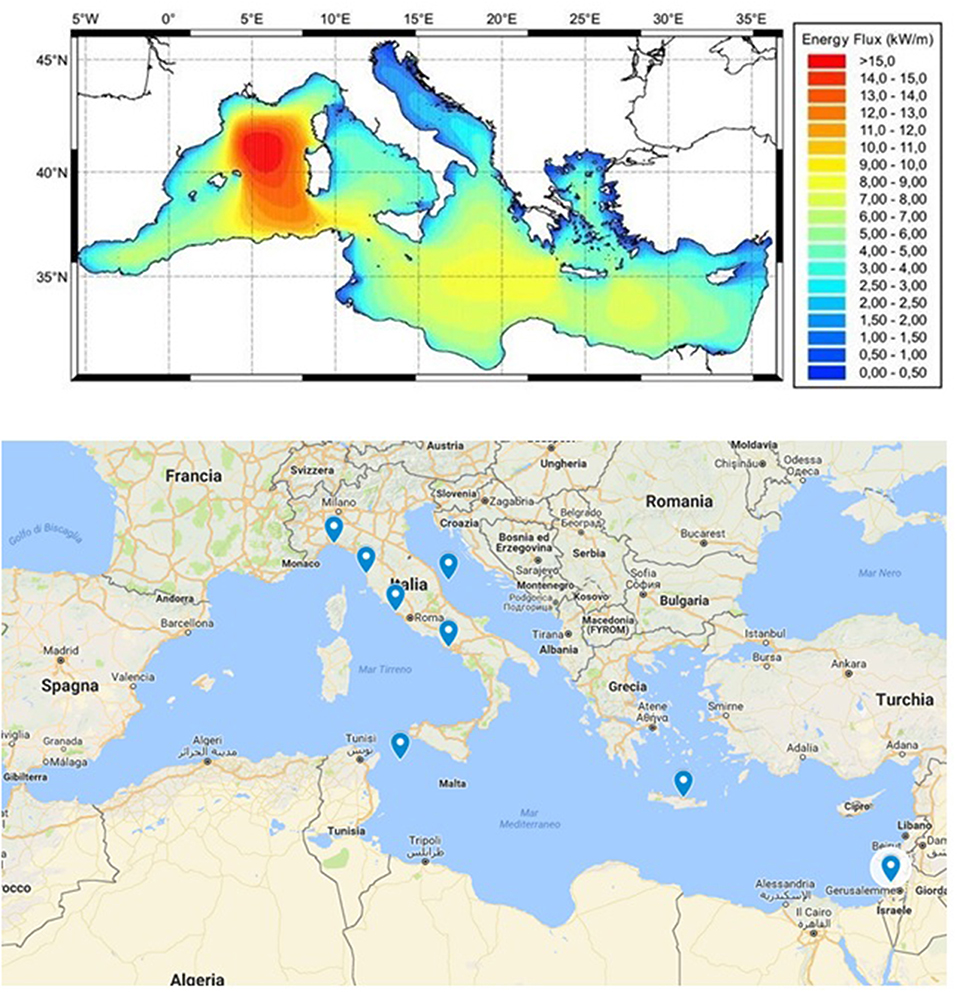
Figure 1. Mediterranean wave resource (Top) and Map of Mediterranean showing the places where the projects took place (Bottom). Map data ©2018 GeoBasis-DE/BKG (©2009), Google, Inst. Geogr. Nacional, Mapa GISrael, ORION-ME.
The Pantelleria Case
The territories most interested in energy security are obviously the most remote, and among them the most fragile from the landscape point of view are the islands. For this reason, our activity has focused on an island of the Mediterranean Sea: Pantelleria.
The island of Pantelleria is in the center of the western part of the Channel of Sicily about 110 km southwest of Sicily and 70 km east northeast of Tunisia. When, 10 years ago, the group of the Department of Mechanical and Aerospace Engineering of Polytechnic of Turin had to decide on a site for technology testing, Pantelleria seemed immediately an interesting candidate to host the device, mainly because of its availability: Pantelleria has a wave resurgence consisting in an annual average of about 7 kW/m, as can be seen in; secondly, the absence of an electricity connection between the island and the national electricity distribution.
The island should therefore independently provide to its energy production and currently such production is obtained mainly through fossil fuels and the consequent problems of pollution and fuel supply. Moreover, the cost of energy in Pantelleria and more generally in Italian minor islands, is three to five times higher than the cost on the mainland1, which makes small islands a favorable area for the implantation of renewables.
The Ministerial Decree concerning small islands fixes some clear targets for 2020 (Ministry of Economic Development, 2017) for instance Pantelleria, the biggest of the small islands of Sicily that is not connected to the grid, with 7,700 inhabitants and an annual electricity production of 44,170 MWh from diesel generators must achieve the following objectives within 2020:
- 2,720 kW of RES installed power;
- 3,130 m2 of solar thermal panels.
Furthermore, the Economic Development Ministry will fund with 10 million euro two innovative projects able to decrease by at least 20% the annual electricity production from fossil fuels.
To this end, studies have been carried out to represent the best energy scenario that contemplates the optimal RES mix based on the available resources. Given the need to reduce the impact on the territory as much as possible, the focus has been strongly on the energy of the sea waves. Since 2010, prior to any activity, a status monitoring was carried out in the most energy-intensive area of the island: a wave gauge was installed about 800 m from the port in the area north west of the island, exposed to the wind (and thus the waves) prevalent direction: the mistral (N).
In the proximity of the detection point it was then decided to install the power generation device (Figure 2).
From the data acquisition it was possible to identify the waves characteristics distribution on an annual basis. These data allow to define the power and therefore the energy available on the installation site. Following the acquisition of the above information, the team proceeded to the design phase of the machine to be installed.
In the following paragraphs, after the analysis of the state of the art, the design, and installation phase of the technology in test will be illustrated.
The Mediterranean Technologies
REWEC3: Resonant Wave Energy Converter
REWEC3 (Resonant Wave Energy Converter) is an oscillating water column (OWC) wave energy converter, which is equipped with a U-shaped duct used for connecting the water column to the open sea field as represented in Figure 3. This characteristic allows to increase significantly the hydrodynamic performance in comparison to traditional OWC plants. REWEC3 was developed by Mediterranean University of Reggio Calabria and a small prototype with scale 1:10 and length 16.2 m was installed at the Natural Ocean Engineering Laboratory NOEL of the Mediterranea University of Reggio Calabria (Malara et al., 2017; Moretti et al., 2019). The estimated average electrical energy produced during 1 year from a Rewec plant with total length of 1 km in central Mediterranean Sea is 6,000–9,000 MWh2. At present the first full-scale prototype is under construction in the Civitavecchia's harbor in the Tyrrhenian Sea (Arena et al., 2017).
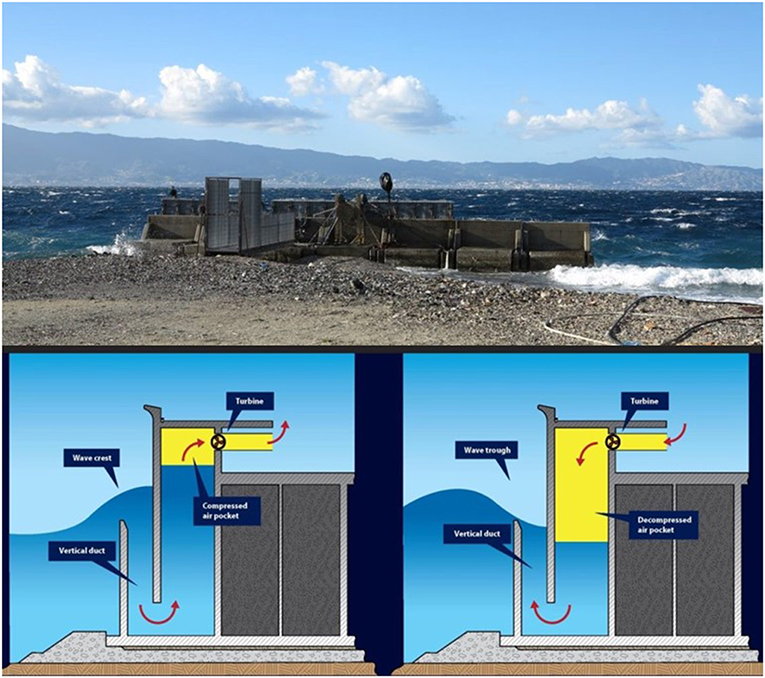
Figure 3. 10 REWEC3 scale device (Top—from www.noel.unirc.it) and schematic representation of REWEC3 (Bottom).
Poly-OWC: Polymeric Oscillating Water Column
Poly-OWC (Polymeric Oscillating Water Column) introduced the new category of Polymeric Wave Energy Converters, characterized by the usage of Dielectric Elastomer (DETs) as shown in Figure 4. The working principle of Poly-OWC is the variable capacitance electrostatic generation principle, where the voltage of the charges lying on DET is increased by the deformation of the membrane. Poly-OWC was developed by Scuola Superiore Sant'Anna of Pisa; at the present the Technology Readiness Level (TRL) is 3/4 and a first small scale prototype has been built (Moretti et al., 2018; Chen et al., 2019).
University of Florence—OWC
University of Florence and AM3 Spin Off Srl are developing an OWC wave energy converter WEC. The hull is designed as a motion attenuator device for large floating platforms with the additional benefit of providing an efficient renewable energy source as show in Figure 5 (Left). The technological readiness of the device is TRL4 and the technological concept has been validated through CFD modeling and laboratory experiments. CFD simulations are provided in Figure 5 (Right) (Cappietti et al., 2019).
Generma wec
SME Generma Srl has developed another wave energy converter based on the attenuator concept. The technology consists of unit elements connected by hinges in a floating modular structure. The relative rotation of modules under wave motions moves hydraulic pistons that compress fluid in a closed circuit. As a result, energy conversion is achieved by a modified Pelton turbine and an asynchronous generator. Initially, a small-scale device 5 kW was tested in laboratory and in 2016 a near-scale prototype 80 m long and 1.9 m wide with nominal power 150 kW has been realized in order to make field tests in Adriatic Sea, as shown in Figure 6 (Generma.com, 2016).
Sea Spoon
Seaspoon is a wave energy converter developed by the University of Genova, in collaboration with RINA Consulting with TRL 6. The Seaspoon device captures the wave energy by mean of a horizontal axis rotor orthogonal respect to the incoming wave direction. The “spoon” device, a plate stiffly coupled to the rotor, boosts the conversion efficiency of the rotor immersed in the particle flux, the small scale prototype and the schematic representation are show in Figure 7. The Seaspoon full scale device was installed in the open sea in front of Genova city in 2015 with nominal capacity 1kW and 2 m wave length after having been tested in University campus, instead the small scale device has 10 W rated power and 0.6 m wave front length (Di Fresco and Traverso, 2013).
Gel System
Gel system is a wave energy converter developed by SEAPOWER scrl and it is designed to function near the coast or in shallow waters. The device consists of a floating body linked to a fixed frame that is left free to oscillate around a horizontal axis under the action of waves. Inside the hull there is a permanent magnet electric generator which is integrated in the PTO (Power Take Off) system that results the transformation of linear motion induced by the waves into rotary motion of the generator rotor. A 1:5 scale and a full-scale prototype with a PTO realized by Umbra Group S.p.A. as shown in Figure 8 (top) was tested in the wave tank located at the University of Naples “Federico II.” The technological readiness of the device (TRL) is 5 and it is ready for testing in real sea conditions (SEAPOWERSRCL, 2017; Coiro et al., 2018, 2019).
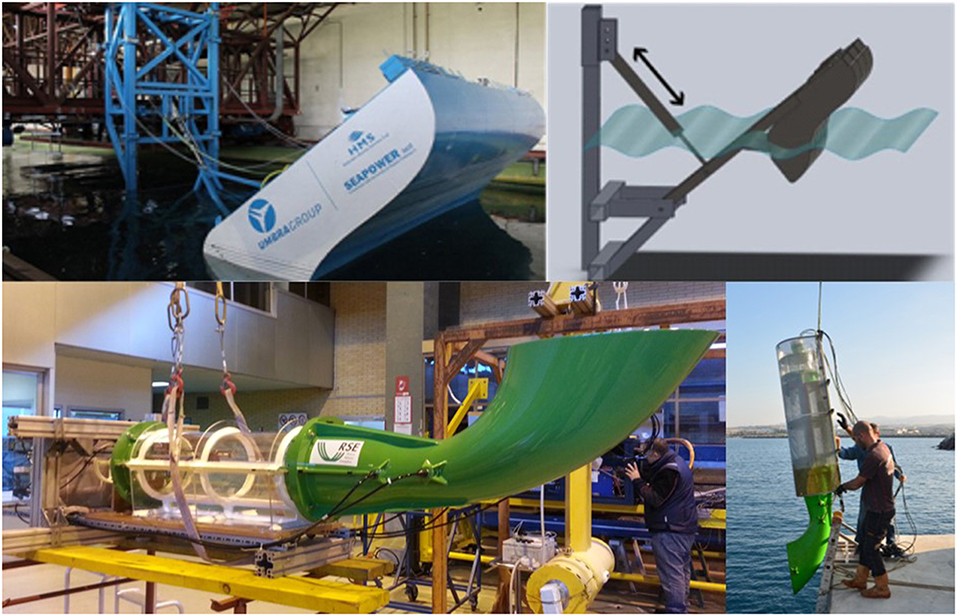
Figure 8. (Top) Full scale GEL prototype (Left) and schematic representation of GEL (Right). (Bottom) Full scale WAVESAX prototype (Left) and its deployment in Civitavecchia (Right).
Wavesax
WAVESAX is an innovative wave energy converter developed by RSE S.p.A. It belongs in the OWC category and the TRL of the technology is 5/6. The device is integrated in coastal structures (harbors, ports, etc.) and it consists of a vertical pipe in which water moves upward and downward, following the wave motion a prototype and an application are reported in Figure 8 (bottom). A small scale (1:20) was tested in HMRC (Hydraulic Marine Research Center) in Ireland and another one with scale 1:5 was tested in at the ECN Hydrodynamic and Ocean Engineering Tank, France (Peviani, 2015).
Obrec
OBREC (Overtopping BReakwater for Energy Conversion) is a wave energy converter developed by the Università degli studi di Campania “Luigi Van-vitelli.” The device is embedded into a breakwater and the working principle is based on the wave overtopping process. A small scale prototype (1:30) was tested at Aalborg University in Denmark during two experimental campaigns in 2012 and 2014. A full scale of 6-m length was installed in the port of Naples in 2015 along the San Vincenzo breakwater, full scale OBREC and his schematic representation are reported in Figure 9, where the sea depth is about 25 m and the available power is estimated to be 2.5 kW/m. Currently the TRL is 5 (Contestabile et al., 2016).
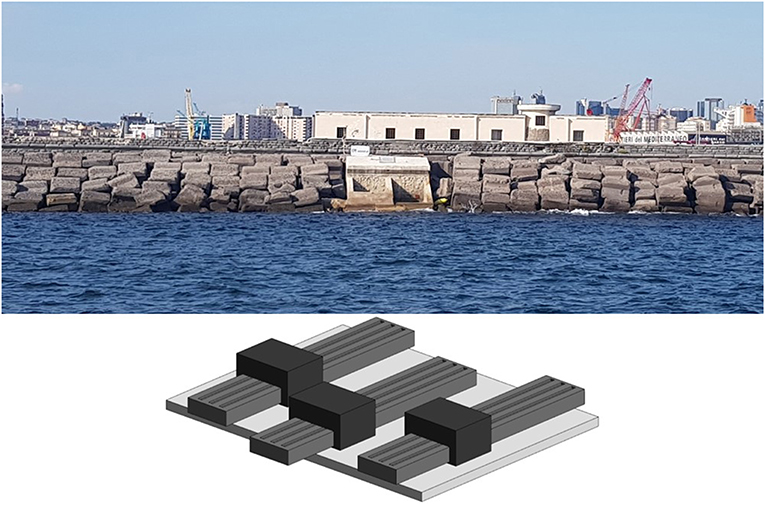
Figure 9. Full scale OBREC in the port of Naples (Top). Schematic representation of a 40 South Energy H24 wave energy converter plant (Bottom).
40South Energy H24
40South Energy H24 is a wave energy converter assembled in two parts: a 12-m long guide and a mobile component left free to slide on it. The plant is constituted of multiple modules mounted 12 m deep and fixed to the seabed. The device is activated by the force of the waves impacting the mobile component and causing its relative motion with respect to the guide. The H24 WEC was developed by 40SOUTH ENERGY Srl. They've also built an energetic park in Marina di Pisa in 2015, where a plant of 50 kW, constituted of 4 H24 devices, has been deployed and connected to the grid for an experimental campaign (40South Energy, 2012).
Sinnpower Wec
SINNPOWER provides wave energy converter modules which are floating heaving point absorbers. Single devices can be installed and function in ports, offshore structures, fish farms, etc. However, a WEC array is also available which is constituted by many single modules. Every module generates about 3 kW (SINN Power GmbH Wave Energy, 2017). In December 2015, SINN Power started operating the first wave energy converter module on the Greek island Crete and specifically on the port wall of Heraklion, a graphic representation of the whole system is shown in Figure 10. By 2019, the company plans to install three more WECs at the port of Crete (Knappik, 2018).
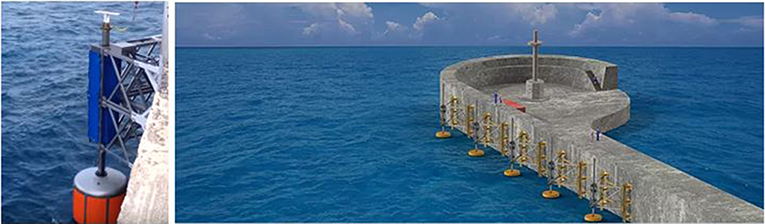
Figure 10. WEC module (Left) and schematic representation of port of Heraklion, Greece (Right) © SINN Power.
Eco Wave Power Convertor
EWP Convertor (Eco Wave Power Convertor) absorbs energy from wave power using uniquely shaped floaters which follow the elevation of the water when a wave passes. The devices are attached by robust arms to any man-made structure such as break waters, jetties, piers, poles, and floating and fixed platforms, as shown in Figure 11. The company has installed a 10 kW research and development power station in Jaffa port in July 20153 (Techtime.news, 2015).
Pendulum Wave Energy Converter (PeWEC)
PeWEC (Pendulum Wave Energy Converter) is an offshore, floating, pendulum-based Wave Energy Converter developed by W4E, Polytechnic of Turin and ENEA, with TRL5. It is mainly composed of a floating hull moored on the seabed and a pendulum connected to the shaft of an electrical generator which is integral with the hull structure. Likely in the case of ISWEC device, pendulum, electrical generator and all other equipment necessary for the device functioning are enclosed in the hull. At present a full-scale devise is under construction, how you can notice in Figure 12 (Pozzi, 2018).
ISWEC (Inertial Sea Wave Energy Converter)
It is a device based on the technology developed by the Polytechnic of Turin and implemented by the company Wave for Energy Srl (Bracco et al., 2011). It consists in floater anchored to the seabed with a loose mooring, allowing the pitching movement and orientation to follow the main direction of the front wave. Outside it looks like a completely closed hull, with the only electric cable that, through a joint, passes through the hull and connects with a static cable positioned on the seabed and reaching as far as the beach, in a transformer cabin to the island network4 (Bracco et al., 2011). Unlike the other devices, PEWEC and ISWEC equipment are enclosed inside the hull avoiding the contact with the sea water that results in an increase of the durability of the device. Also, the device is modular so for instance the gyroscopic unit can be changed in order to vary the nominal power of the converter.
The heart of the machine is the gyroscopic system: two flywheels of 10 tons placed in rotation that generate the inertial reaction torques that arise from the interaction between their speed and the pitching motion of the hull on two internal precession shafts, on which the permanent magnet electric generators are keyed5.
The possibility to vary the angular velocity of the flywheel allows the device to adapt and maximize performance for different sea states, increasing the flexibility of the converter. Some features of the machine deployed in Pantelleria are presented in Figure 13 (Left).
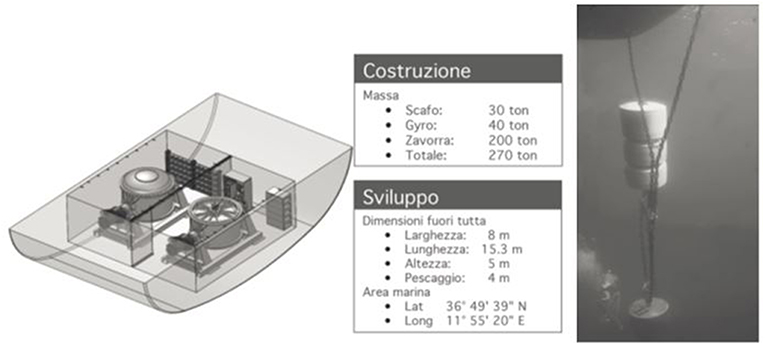
Figure 13. ISWEC hull and principal components. Dimensions and features of the device (Left) and picture of mooring (Right).
During the device operation the mechanical power contained in the movement of the waves is transferred to the hull, thus causing the motion of the latter and the pitching motion is the most evaluable response. The angular velocity of the hull, combined with the angular momentum of the flywheel placed in rotation, causes a gyroscopic moment that causes the transfer of mechanical power between the hull and the inner shaft of precession, on which the electric PTO is mounted.
As mentioned previously, the flywheel speed is adjusted accordingly to the working sea conditions in order to maximize energy absorption. The angular velocity in working conditions and the regulation law of the absorption PTO is updated hourly, using the forecasts provided by ENEA: every day the sea state forecasts are transmitted and received automatically from the device for the next 3 days with hourly resolution.
The Mooring System
As mentioned above, the energy production system is bound to the sea bottom with loose mooring to guarantee that the dynamics of the hull are not compromised; at the same time the moorings constitute the constraints of moving the machine, as well as the main safety features.
At the time of launch, in the absence of electrical connection with the distribution network, the hull was left free to orientate itself to the direction of the wave, rotating around the constraint on the bottom: this condition occurs using a single mooring line that engages the bow of the device obtaining a situation very similar to the commonly defined “wheel boat.” At present the electric cable connecting to the network is being installed and at the same time two stern moorings are being installed in such a way that the system is aligned with the prevailing one with a variability of about ±20°.
This mooring configuration does not significantly compromise the device's productivity as the wave characteristics of the sleeping installation site are characterized by an extremely declared directionality.
Figure 13 (Right) shows an image of the bow mooring in which the constituent elements are distinguished: catenary, jumper, and counterweight. The presence of these elements, appropriately sized, ensures the non-linearity of the force exerted by the mooring on the device in such a way as to preserve the mooring itself from “tears” which could cause damage.
ISWEC in Pantelleria
The launch of ISWEC on the island took place in August 2015. The installed system presented some partitions: there was no connection to the network (therefore the machine was in stand-alone operation) with elements of dissipation of the energy produced, and the system only saw one of the two gyroscopic units installed. The decision to proceed with subsequent steps of experimentation was born with the aim of proceeding to intermediate checks of the design choices been made. In Figure 14, a photograph during the transport on the job site and an image of the system while working are shown.
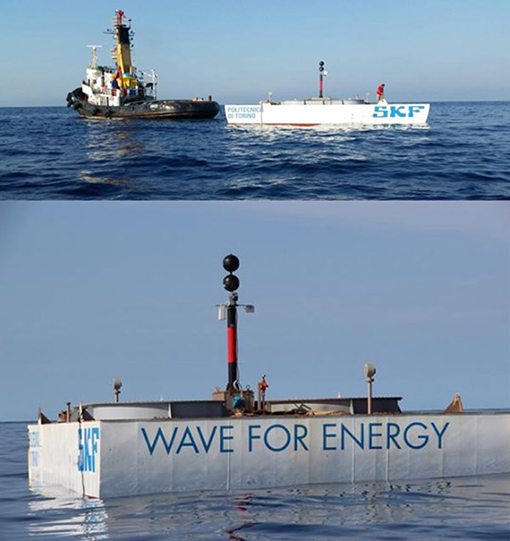
Figure 14. Transportation of ISWEC to the installation site in Pantelleria. August 2015 (Top) and ISWEC deployed at sea (Bottom).
In the first test phase of the system, the sea conditions encountered have become extremely challenging, with significant heights and peak periods characteristic of oceanic sites. This made it possible to test the tightness of the device and the mooring system.
In none of these phases the system has failed. At the same time, it was possible to verify part of the theoretical bases on which the design criteria were based with the validation of the predictive mathematical models of the behavior of the system, both from the dynamic point of view and from the performance point of view in terms of energy produced (Cagninei et al., 2015).
From an analysis of the trends the following considerations can be made:
The angular speed of the flywheel was 150 rpm, while the optimal speed for the 5 kW/m scenario is equal to 450 rpm. By observing the gross electrical power and the corresponding gross overall efficiency, a decrease in performance is obtained as the wave power increases: this phenomenon finds its explanation precisely in the choice, imposed by the experimenter, to keep the angular speed of the flywheel constant. The set speed allows the system good performance near a power of 3 kW/m. However, the angular velocity of the flywheel has not changed as the power of the wave has increased and the performance has therefore decreased.
The device operated with the following average characteristics:
- Average wave power density: 4.6 kW/m
- Gross electric power produced (Reference to the “Gross Pelect” size of the graph): 3.2 kW. At this average power corresponds a Relative Capture Width average (“Gross RCW”) equal to 8.9%
- Device Machine losses in these conditions are 1.95 kW
- Net electric power produced (Reference to the “Net Pelect” size of the graph) is equal to 1.25 kW. At this average power corresponds a Relative Capture Width medium (Reference to the “Net RCW” size of the graph) equal to 3.4%.
The behavior is considered acceptable since it consists in a “border” zone of the envelope of machine operation. The sea states encountered from the end of September to December 2015 are presented in Figure 15.
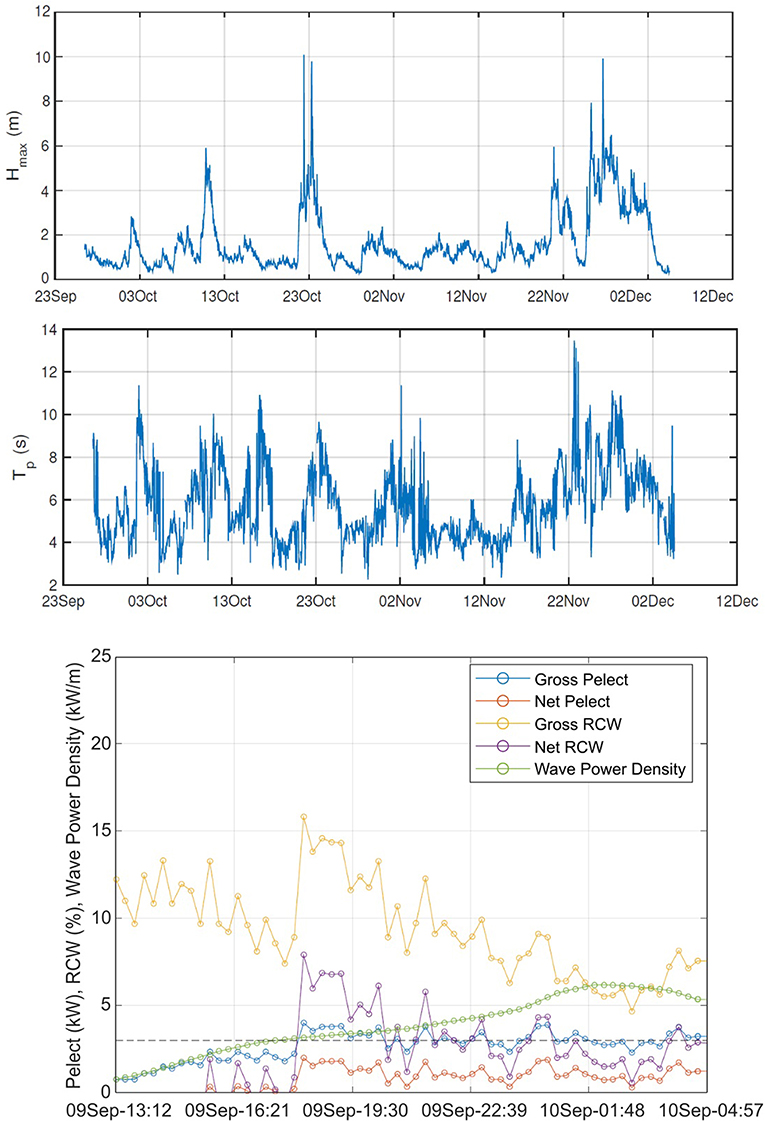
Figure 15. Historical data of sea states encountered between September and December 2015 (Top), experimental performance of ISWEC in Pantelleria (Bottom).
About performance in terms of productivity, the results of the testing phase were in good agreement with those obtained previously in the simulation and design phase. However, the system has worked on the island (in this first phase without the connection to the grid due to the lack of the underwater cable) with the presence of only one gyroscopic and the tuning phase has taken place into the sea so the production, in this first experimental phase was limited.
At this moment the prototype is in the process of completing the upgrade procedure and currently has received the addition of the second gyro group and the installation of the electrical infrastructure (submarine cable and connection to the grid). Soon there will be a new launch, and the second test phase will therefore begin.
Conclusions
The energy from sea waves remains today a very unbalanced issue toward applied research, but this application, like others already present at the prototype level in other installation sites, show how technologies are evolving toward solutions aimed at ensuring, in addition to performance, even requirements such as the safety and durability of the components. Each design choice is strongly conditioned by the future productive transferability of the devices hence the energy cost is included among the parameters to be optimized.
The Mediterranean Sea, due to the reduced wave energy potential, can be used as a cradle for more powerful seas, like the North Sea, where the wave period is comparable, but the wave height is much higher. The device tested in the Mediterranean behaves as a scaled device on a real test site, able to reproduce the full-scale system with smaller financial effort.
For some specific applications, the identified technological solutions are already competitive compared to the estimated costs of producing energy from fossil fuels. Obviously, when the sales volumes become large, it will be possible to obtain a further reduction in the cost of energy in order to offer the conversion of another renewable energy source to be integrated into the energy mix which will hopefully be the energy production of the future.
Author Contributions
GM produced this review with the contribution of her reasearch team at Politecnico di Torino.
Conflict of Interest
The author declares that the research was conducted in the absence of any commercial or financial relationships that could be construed as a potential conflict of interest.
Footnotes
1. ^Italian Republic. Decree of the Ministry of Economic Development 14/02/2017: “Disposizioni per la progressiva copertura del fabbisogno delle isole minori non interconnesse attraverso energia da fonti rinnovabili”. OJ of the Italian Republic, 18/05/2017.
2. ^Wavenergy.it Srl. Available online at: https://www.wavenergy.it/about/ (accessed August 30, 2019).
3. ^Eco Wave Power Technology. Available at: http://www.ecowavepower.com (accessed June 17, 2019).
4. ^Wave for Energy Srl Company. Available online at: http://www.waveforenergy.com.
5. ^Wave for Energy Srl Youtube Channel. Available online at: https://www.youtube.com/channel/UC1imzSpUaWpRatfttVppt_Q.
References
40South Energy (2012). Brochure for R38 and R115 Wave Energy Converter. Available online at: https://www.enelgreenpower.com/it/media/press/d/2013/06/enel-green-power-e-40south-energy-insieme-per-lo-sviluppo-dellenergia-prodotta-dalle-onde-del-mare (accessed June 17, 2019).
Alvarez-Silva, O. A., Osorio, A. F., and Winter, C. (2016). Practical global salinity gradient energy potential. Renew. Sustain. Energy Rev. 60, 1387–1395. doi: 10.1016/j.rser.2016.03.021
Arena, F., Laface, V., Malara, G., Romolo, A., Viviano, A., Fiamma, V., et al. (2015). Wave climate analysis for the design of wave energy harvesters in the Mediterranean Sea. Renew. Energy 77, 125–141. doi: 10.1016/j.renene.2014.12.002
Arena, F., Romolo, A., Malara, G., Fiamma, V., and Laface, V. (2017). “The first worldwide application at full-scale of the U-OWC device in the port of Civitavecchia: initial energetic performances,” in Proceedings of the ASME 36th International Conference on Ocean, Offshore and Arctic Engineering, OMAE2017-62036 (Trondheim). doi: 10.1115/OMAE2017-62036
Bracco, G., Giorcelli, E., and Mattiazzo, G. (2011). ISWEC: A gyroscopic mechanism for wave power exploitation. Mech. Mach. Theory 46, 1411–1424. doi: 10.1016/j.mechmachtheory.2011.05.012
Cagninei, A., Raffero, M., Bracco, G., Giorcelli, E., Poggi, D., and Mattiazzo, G. (2015). Productivity analysis of the full scale inertial sea wave energy converter prototype: a test case in Pantelleria Island. J. Renew. Sustain. Energy 7:061703. doi: 10.1063/1.4936343
Cappietti, L., Simonetti, I., and Crema, I. (2019). “Laboratory experiments on the performance of an OWC-WEC:fixed condition versus floating platform-embodied condition,” in 13th International Conference of European Wave and Tidal Energy EWTEC,2019 (Naples).
Chen, Y., Agostini, L., Moretti, G., Fontana, M., and Vertechy, R. (2019). Dielectric elastomer materials for large-strain actuation and energy harvesting: a comparison between styrenic rubber, natural rubber and acrylic elastomer. Smart Mater. Struct. 28. doi: 10.1088/1361-665X/ab3b32
Coiro, D., Troise, G., and Bizzarrini, N. (2018). Experiences in developing tidal current and wave energy devices for Mediterranean Sea. Front. Energy Res. 6:136. doi: 10.3389/fenrg.2018.00136
Coiro, D., Troise, G., Bizzarrini, N., and Castellini, L. (2019). “Numerical and experimental test on a large-scale model of a pivoting wave energy conversion system,” in European Wave and Tidal Energy Conference -EWTEC 2019 Proceedings (Napoli).
Contestabile, P., Ferrante, V., Di Lauro, E., and Vicinanza, D. (2016). “Prototype overtopping breakwater for wave energy conversion at port of Naples,” in Proceedings of the Twenty-Sixth International Ocean and Polar Engineering Conference (Rhodes: ISOPE).
Di Fresco, L., and Traverso, A. (2013). “THE SEASPOON, innovative wave energy converter,” in Proceedings of 2013 Ocean (San Diego, CA: IEEE).
European Commission (2012). Blue Growth Opportunities for Marine and Maritime Sustainable Growth, COM 494 Final. Available onine at: http://ec.europa.eu/maritimeaffairs/sites/maritimeaffairs/files/docs/publications/bluegrowth_en.pdf (accessed June 17, 2019).
Generma.com (2016). Wave Energy Converter-GENERMA. Available online at: https://www.generma.com/pages/wave-energy/wave-energy-converter (accessed June 17, 2019).
Knappik, C. (2018). Wave Energy Converter Installation in Heraklion. Available online at: https://www.sinnpower.com (accessed June 17, 2019).
Malara, G., Romolo, A., Fiamma, V., and Arena, F. (2017). On the modelling of water column oscillations in U-OWC energy harvesters. Renew. Energy 101, 964–972. doi: 10.1016/j.renene.2016.09.051
Ministry of Economic Development (2017). Fonti rinnovabili per isole non interconnesse. Available online at: http://www.sviluppoeconomico.gov.it/index.php/it/198-notizie-stampa/2036486-fonti-rinnovabili-nelle-isole-minori-non-interconnesse (accessed June 17, 2019).
Moretti, G., Malara, G., Scialò, A., Daniele, L., Romolo, A., Vertechy, R., et al. (2019). Modelling and field testing of a breakwater-integrated U-OWC wave energy converter with dielectric elastomer generator. Renew. Energy 146, 628–642. doi: 10.1016/j.renene.2019.06.077
Moretti, G., Rosati, P., Gastone, P., Righi, M., Forehand, D., Ingram, D., et al. (2018). Resonant wave energy harvester based on dielectric elastomer generator. Smart Mater. Struct. 27. doi: 10.1088/1361-665X/aaab1e
Peviani, M. (2015). “WAVESAX device: conceptual design and perspectives,” in Proceedings of 8th European Seminar OWEMES (Rome).
Pozzi, N. (2018). Numerical Modelling and Experimental Testing of a Pendulum Wave Energy Converter (PeWEC). Politecnico di Torino, Torino, Italy.
SEAPOWERSRCL (2017). Gel: Clean Energy From the Sea. Available online at: http://www.seapowerscrl.com/ocean-and-river-system/gel (accessed June 17, 2019).
Soukissian, T. H., Denaxa, D., Karathanasi, F., Prospathopoulos, A., Sarantakos, K., Iona, A., et al. (2017). Marine renewable energy in the Mediterranean Sea: statuts and perspectives. Energies 10:1512. doi: 10.3390/en10101512
Techtime.news (2015). Eco Wave Power Raises $2 Million for Sea Waves Energy Generator. Available online at: http://techtime.news/2015/08/25/eco-wave/ (accessed June 17, 2019).
Keywords: Wave Energy, Mediterranean Sea, Wave Energy Converters (WECs), State of the Art, ISWEC
Citation: Mattiazzo G (2019) State of the Art and Perspectives of Wave Energy in the Mediterranean Sea: Backstage of ISWEC. Front. Energy Res. 7:114. doi: 10.3389/fenrg.2019.00114
Received: 31 August 2018; Accepted: 04 October 2019;
Published: 29 October 2019.
Edited by:
Simone Bastianoni, University of Siena, ItalyReviewed by:
Pasquale Contestabile, Università Degli Studi Della Campania L. Vanvitelli, ItalyAngeliki Fotiadou, Aristotle University of Thessaloniki, Greece
Copyright © 2019 Mattiazzo. This is an open-access article distributed under the terms of the Creative Commons Attribution License (CC BY). The use, distribution or reproduction in other forums is permitted, provided the original author(s) and the copyright owner(s) are credited and that the original publication in this journal is cited, in accordance with accepted academic practice. No use, distribution or reproduction is permitted which does not comply with these terms.
*Correspondence: Giuliana Mattiazzo, Z2l1bGlhbmEubWF0dGlhenpvJiN4MDAwNDA7cG9saXRvLml0