- 1National Renewable Energy Laboratory, Biosciences Center, Golden, CO, United States
- 2National Renewable Energy Laboratory, National Bioenergy Center, Golden, CO, United States
The National Renewable Energy Laboratory (NREL) developed low severity Deacetylation and Mechanical Refining (DMR) process under atmospheric pressure, generates highly fermentable, low toxicity sugar syrups and highly reactive lignin streams. The dilute alkali deacetylation step saponifies acetyl groups from the hemicellulosic fraction of biomass into a black liquor waste stream that also contains solubilized acetic, ferulic, p-coumaric acids, lignin-carbohydrate complexes (LCCs), oligosaccharides, and solubilized lignin. Valorization of the soluble components in the deacetylation black liquor waste stream requires characterization of the black liquor. Our analyses show that glucan, xylan, and lignin were three main components in black liquor and the oligosaccharides in the black liquor were mainly dimers and trimers with arabinofuranose branch groups mainly on the xylooligomers. GPC chromatograms showed that the black liquor also contained oligomeric lignin moieties with molecular weights ranging between 1,800 and ~10,000 Da. Enzymatic saccharification of the black liquor was conducted based on the compositional and structural information. Incorporation of commercial cellulase and hemicellulase enzyme preparations with various accessary enzymes showed up to 57% hydrolysis of the xylooligosaccharides in the black liquor to monomeric sugars, which could be used to improve biorefinery carbon utilization in biological fermentations to improve end product fuel or bioproduct volumes per metric ton of biomass processed. This is so far the first study about characterizing DMR black liquor and exploring breaking down the oligosaccharides in black liquor for potential use.
Introduction
The development of renewable liquid fuels from lignocellulosic biomass is considered by Environmental Protection Agency (EPA) as one of the most important strategies to cease the global climate change attributed to Green House Gas (GHG) emissions from fossil fuels combustion. Lignocellulosic biomass can be converted to ethanol or other drop-in hydrocarbon fuels through single bioconversion or hybrid bio/catalytic conversion technologies in downstream processing steps using both the sugars and lignin moieties solubilized and fractionated in pre-treatment and enzymatic hydrolysis (Davis et al., 2013). Among all the pre-treatment processes, the Deacetylation and Mechanical Refining (DMR) process developed by National Renewable Energy Laboratory (NREL, Golden, Colorado) is a unique atmospheric pre-treatment process that has demonstrated high sugar concentration (>270 g/L fermentable sugars) and low toxicity sugar syrup production from corn stover, which also led to an ethanol product titer of 86 g/L in followed fermentation test (Chen et al., 2012, 2014, 2016). Basically, in deacetylation step, dilute sodium hydroxide (NaOH) was used to catalyze the saponification of the branched acetyl groups from hemicellulose backbone as well as to trigger partial delignification that occurs under boiling point and low alkali concentration (<0.2 M). Mechanical refining further improves the digestibility of deacetylated biomass by increasing the cellulose accessibility with cutting and fibrillation (Jones et al., 2013, 2014; Kim S. M. et al., 2016; Park et al., 2016). The DMR pre-treated biomass has shown good digestibility and superb fermentability as reported previously (Zhang et al., 2014; Chen et al., 2016; de Assis Castro et al., 2017).
In addition to removing acetyl groups on xylan, deacetylation also solubilizes a portion of carbohydrates from corn stover (xylan 2~10%, glucan ~1%) (Chen et al., 2012). Compared to severe alkaline pre-treatment conditions (Wyman et al., 2005; Huang et al., 2015; Kim J. S. et al., 2016), deacetylation in DMR process is too mild to cause complete degradation of carbohydrates therefore the solubilized carbohydrates in black liquor are likely in oligomeric form. As deacetylation targets on acetyl groups on hemicellulose, most of the dissolved carbohydrates are xylooligosaccharides. So far, there is barely any study on recycling these oligosaccharides in black liquor from DMR process. The dissolved hemicelluloses in black liquor pose several challenges regarding to the economic and environmental issues of biorefinery industries, as follows:
1) The loss of the hemicellulose from deacetylation lowers overall sugar and final product yields, leading to higher production cost (Davis et al., 2013).
2) The hemicellulose in the black liquor significantly increases the viscosity of the concentrated black liquor after evaporation, causing issues in handling the waste liquor (Chen et al., 2018).
3) The heating value of the hemicellulose is only 13.6 MJ/kg, which is only half of that of lignin (Sjostrom, 1993). The potential utilization of lignin for value-added products removes the major heating source from the black liquor, making the residual liquor incombustible (Chen et al., 2019).
In this context, for good use of black liquor, utilization of hemicelluloses has to be considered. In nature, xylooligomers are polysaccharides that are difficult to be used directly as carbon source (Bankeeree et al., 2018), so it is better if we can first break down it into monomeric sugars thus they can be used as carbon source for microbial growth. However, as hemicelluloses from biomass are highly substituted and possibly branched with side groups such as arabinose, galactose and linked to lignin moieties forming lignin carbohydrate complex (LCC) (Giummarella and Lawoko, 2017), it is very challenging for enzymes to hydrolyze. It is well-known that two types of hemicellulases are mainly responsible for degradation of xylan backbone (Collins et al., 2005): (1) endo-1,4-β-xylanase (EC 3.2.1.8), which randomly cleaves 1,4-β-D-xylosidic backbone linkages releasing xylo-oligosaccharides of variable lengths; (2) exo-1,4-β-xylosidase (EC 3.2.1.37), which successively removes D-xylose residues from the non-reducing ends of xylo-oligosaccharides and also cleaves xylobiose. Incorporating xylan backbone cleaving enzymes with accessory enzymes such as arabinofuranosidase has shown significant enhancement on the hydrolysis of the hemicelluloses from acid pre-treated/water extracted hemicelluloses (Sørensen et al., 2007; Hu et al., 2016). For the dissolved hemicellulose from deacetylation process, the xylan backbone is likely debranched due to the saponification, therefore a xylanase cocktail including accessary enzymes maybe necessary for xylan degradation. However, the structures of the hemicellulose in black liquor generated from DMR process still remain unknown due to the uniqueness of deacetylation conditions. To find a tailored xylanase cocktail specifically targeting at the structures of dissolved hemicellulose, characterization of black liquor especially dissolved oligosaccharides is very necessary for potential utilization in future.
Herein in this work, in order to finally utilize the valuable compounds in black liquor, a comprehensive compositional and spectral analyses for characterizing components in the black liquor was performed. The types of oligosaccharides were characterized and the functional branch groups on xylooligosaccharides were identified. In addition, enzymatic breakdown of oligosaccharides in black liquor was also conducted based on the structural information that we collected. All these results will help us to know about the structures of the components in DMR black liquor, to better understand the potential value of black liquor, and also help us to evaluate the possibility of utilizing the oligosaccharides in black liquor via enzymatic deconstruction. All presented results here could serve as a baseline for future assessment of recycling and reusability of black liquor from DMR process.
Materials and Methods
Black Liquor
Deacetylation of corn stover was performed in a 90-L paddle reactor. The deacetylation and black liquor preparation was as described in previous work (Chen et al., 2012, 2018). Briefly, dry corn stover chips were immersed in NaOH solution heated up to 80 or 92°C and held for 2 h. After deacetylation, the liquor was drained through the 8-inch screen located in the bottom port of the paddle mixer. In this study, three black liquor samples were prepared with different NaOH loadings.
1. DMR-BL-50: 50 g NaOH per kg of corn stover at 80°C (NaOH: ~4 g/L).
2. DMR-BL-70: 70 g NaOH per kg of corn stover at 92°C (NaOH: ~5.8 g/L).
3. DMR-BL-100: 100 g NaOH per kg of corn stover at 92°C (NaOH: ~8.3 g/L).
Composition Analysis for Black Liquor
The compositions of black liquor samples were measured in duplicate using the standard NREL methods for determining biomass carbohydrates, lignin, and organic acids (Sluiter et al., 2008a,b). The standard errors for these analyses were <1.5 wt%.
Gel Permeation Chromatography (GPC) Analysis
The black liquor samples were neutralized to pH 7.0 by adding HCl solution (5 M) and then freeze-dried for GPC analysis. To measure the molecular weight distribution, the freeze-dried sample was derivatized first. Fifty milligram of sample was acetylated in a mixture of pyridine (0.5 mL) and acetic anhydride (0.5 mL) at 40°C for 24 h with stirring. The reaction was terminated by addition of methanol (0.2 mL). After the acetylation solvents were evaporated under a stream of nitrogen gas, the sample was further dried in a vacuum oven at 40°C overnight and then suspended in tetrahydrofuran (THF, Baker HPLC grade). The solution was filtered through 0.45 μm nylon membrane syringe filters to separate THF soluble part before GPC analysis. GPC analysis was conducted using an Agilent HPLC with 3 GPC columns (Polymer Laboratories, 300 x 7.5 mm) packed with polystyrene-divinyl benzene copolymer gel (10 μm beads) having nominal pore diameters of 104, 103, and 50 Å. THF was used as the eluent at a flow rate of 1.0 mL/min. A diode array detector was employed measuring absorbance at 260 nm (band width 80 nm). Retention time was converted into molecular weight (Mw and Mn) by applying a calibration curve established using 16 different polystyrene standards (1 × 106 to 580 Da) plus toluene (92 Da). Cirrus GPC Software (Agilent) was used to perform the data analysis.
NMR Spectroscopy
Nuclear magnetic resonance (NMR) spectra were acquired for black liquor sample. The above neutralized and freeze-dried black liquor sample (50 mg) was suspended into 0.5 ml of d6- dimethylsulfoxide/d5-pyridine (4:1, v/v) in a NMR tube. The mixture was sonicated for 5 h until gel became homogeneous (Mansfield et al., 2012). Proton (1H) NMR spectrum was acquired at 25°C on a Bruker AVANCE 400 MHz spectrometer equipped with a 5 mm BBO probe with 1,024 points and a sweep width of 15 ppm. Tetramethylsilane was used as a reference. Heteronuclear Single Quantum Coherence (HSQC) nuclear magnetic resonance (NMR) spectra of black liquor samples were also acquired at 40°C on the same Bruker 400 MHz spectrometer using a BBO probe with Z gradient. Spectra were acquired with 1,024 points and a sweep width of 15 ppm in the F2 (1H) dimension and 512 points and 220 ppm of sweep width in the F1 (13C) dimension. DMSO peak was used as an internal reference (δH 2.5, δC 39.51 ppm). Peak assignment was performed according to literatures (Kim et al., 2008; Mansfield et al., 2012; da Costa Sousa et al., 2016).
MALDI-Time of Flight (ToF) Mass Spectroscopy
Twenty microliter of black liquor sample was injected and analyzed on an Acquity Ultra Performance Liquid Chromatography (UPLC) system (Waters Co., Milford, MA) equipped with a TQD Mass Spectrometer (MS) and Evaporative Light Scattering Detector (ELSD). Waters Masslynx 4.1 software version was used to collect and investigate the analytes of interest. A Shodex Sugar SZ5532 (Zinc) column (Showa Denko K.K., Japan, 6 × 150 mm, 6 μm particle size) was used in the chromatography system and the column temperature was maintained at 60°C. The buffers used to separate the analytes were 0.1% formic acid in water (A) and 0.1% formic acid in acetonitrile (B), and the flow rate was 0.9 mL min−1 A gradient program was used for the separation: 20% A and 80% B at time t = 0; 17% A and 83% B at t = 9 min; 30% A and 70% B at t = 25 min; 40% A and 60% B at t = 40 min; 20% A and 80% B at t = 45 min. For ELSD parameters, drift tube temperature was set at 76°C and gas pressure was at 28 psi. For TQD MS system, the cone voltage was set at 30 V, capillary voltage at 3,000 V, desolvation temperature at 300°C and a source temperature at 110°C. The MS was calibrated and tuned with Sodium Iodide and the black liquor samples were scanned from 50 to 2,000 amu with a 1 s scan time.
Enzymatic Digestion of Oligosaccharides in Black Liquor
Black liquor was subjected to enzymatic hydrolysis with a commercial Novozymes Cellic® cellulase Ctec2, hemicellulase Htec2 or Htec2 supplemented with accessory enzymes. Arabinofuranosidase (ABF) was purchased from Megazyme. Acetyl xylan esterase (AX), alpha-glucuronidase (α-GLU) and ferulic acid esterase (FAE) were heterologously expressed and purified in our lab. For Ctec2, 10 mg/g glucan (7.7 FPU/g glucan) loading was used in the hydrolysis; For Htec2, 10 mg/g xylan loading was used; for additional de-branching accessary enzymes including arabinofuranosidase (ABF), acetyl xylan esterase (AX), alpha-glucuronidase (α-GLU) and ferulic acid esterase (FAE), 5, 5, 1 and 1 mg/g xylooligomers were loaded respectively. The reaction volume was 3 mL. The hydrolysis was conducted under 50°C in 0.05 M citrate buffer solution (pH 4.8) for 48 h and then the reaction solution was applied to HPLC for analysis. The monomeric sugars (glucose, xylose, arabinose, and galactose) were analyzed using a High Pressure Liquid Chromatography (HPLC) system (Agilent 1100, Agilent Technologies, Palo Alto, CA) on an Aminex HPX87H Ion Exclusion column (300 × 7.8 mm, 8 μm particle size, Catalog No. 125-0140). The eluent was 0.01N H2SO4 at a flow rate of 0.6 mL/min. The peaks were identified and quantified with a refractive index detector (RID).
As an alternative way to check the ferulic acid ester branch on xylooligomers, the ferulic acid released after adding ferulic acid esterase was analyzed by HPLC on column Phenomenex® Luna® 5 μm C18(2) (100 Å 250 × 4.6 mm). The operation temperature was set to 30°C. The eluent was a gradient at a flowrate of 1.00 mL/min. Ferulic acid was detected and quantified with a diode array detector at 325 nm wavelength.
Results and Discussion
Composition of Black Liquors
Black liquor in this study was generated from deacetylation process of corn stover under mild alkaline condition. Some carbohydrates and lignin compounds were released into the black liquor. The compositions of the black liquors are shown in Table 1. Monomeric sugars glucose, xylose, arabinose and galactose were not detected in the black liquors. All sugars in the black liquor were in oligomeric form. This is very different from typical black liquors generated from kraft process which usually dissolves a lot of monosaccharides (Niemelä et al., 2007). In DMR black liquor, xylo-oligomers were higher in concentration than gluco-oligomers because deacetylation targeted at removing acetyl branch groups on xylan back bone, which made xylan easier to be degraded. A fair amount of lignin was also released from deacetylation process but it was much less than the lignin dissolved in kraft black liquor (Niemelä et al., 2007; Alekhina et al., 2015), indicating the deacetylation condition was pretty mild. As the deacetylation severity increased with the increase of NaOH loading and temperature, more lignin and oligomers including xylan, arabinan, and galactan were released into black liquors, as shown in Table 1 below.
Characterization of Lignin in Black Liquors by GPC and NMR Spectroscopy
As shown in the composition results above, the components in the black liquor were mainly carbohydrates, lignin and acetic acid. The black liquor samples of DMR BL-50, 70, and 100 were neutralized to pH 7.0 respectively by adding HCl solution (5 M) and then freeze-dried. The freeze dry solids were derivatized for GPC analysis, as described in Method part. GPC chromatograms of the three black liquor samples were shown in Figure 1. Each sample had mainly three portions with molecular weight (Mw) in the range of 100–420 Da, 420–1800 Da, and >1,800 Da, respectively. BL-50 has much lower intensity than BL-70 and BL-100 in all the ranges of Mw, which indicates lower concentration of lignin, i.e., less amount of lignin was solubilized in DMR-BL-50 liquor compared to DMR-BL-70 and BL-100. By calculation, the weight average molecular weights (Mw) for DMR-BL-50, BL-70, and BL-100 were 1,900, 1,700 and 2,100 Da respectively, as shown in Table 2. It was found that the sample DMR-BL-70 had the lowest weight average molecular weight 1,700 Da. The explanation for this is that BL-70 had more lignin compounds with low Mw (<420 Da) than BL-50, as indicated by higher intensity in low Mw area. Similarly, BL-100 had highest Mw of 2,100 Da which could be due to higher intensity corresponding for high Mw portion (>1,800 Da), compared to the other two samples. Higher response/intensity with the increase of NaOH loading indicates that more lignin monomers/dimers as well as lignin oligomers/polymers were released during deacetylation step.
1H-NMR spectra of three black liquor samples are shown in Figure 2. 1H-NMR spectra of BL-70 and BL-100 were similar, but that of BL-50 was different from the other two samples. BL-50 had larger number of peaks of -CH-, -CH2-, and -CH3 than BL-70 and 100. There were large peaks from carbohydrates and small peaks from lignin side-chain at 3.0–4.5 ppm in BL-50. BL-70 and BL-100 had more sharp peaks as well as broad peaks in both lignin side-chain range (3.0–4.5 ppm) and aromatic range (6.0–7.5 ppm) than BL-50. This indicates that BL-70 and BL-100 contained more lignin monomers and dimers than BL-50, suggesting that more lignin was solubilized in black liquor with higher NaOH treatment.
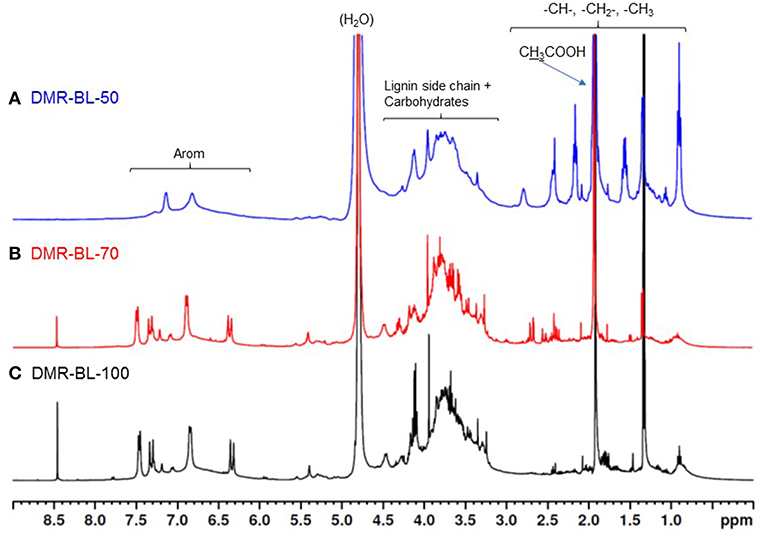
Figure 2. 1H-NMR spectra of three black liquor samples. (A) DMR BL-50, (B) DMR BL-70, and (C) DMR BL-100.
To understand chemical structural changes in lignin in each black liquor samples, 2D NMR analysis was also performed and these spectra are shown in Figure 3. In the range of “lignin side-chain and carbohydrates,” BL-70 and BL-100 had more peaks than BL-50. Anomeric peaks in carbohydrates (“C1 in sugar”) at δc/δH 4.0–5.5/95–110 ppm in BL-50 was smaller than those in BL-70 and BL-100. This result agreed with the composition data of black liquor samples in Table 1, indicating that more hemicelluloses were solubilized with higher NaOH loading treatment. Surprisingly, all samples had little or no peaks in the β-O-4 unit as well as resinol and phenylcoumaran units while GPC data showed that each black liquor sample contained oligomeric lignin moieties (Mw = 1,800–10,000 Da). This implies that these solubilized lignin oligomers were highly condensed and have many C-C bond linkages. This is probably because that (1) unexpected condensation reaction was occurred during deacetylation step between lignin monomers each other or between lignin monomers and carbohydrates, (2) corn stover lignin originally has some highly condensed structural units and these units are connected each other through ether/ester linkages. During deacetylation, these ether/ester linkages were cleaved and the highly-condensed portion were released in the black liquor.
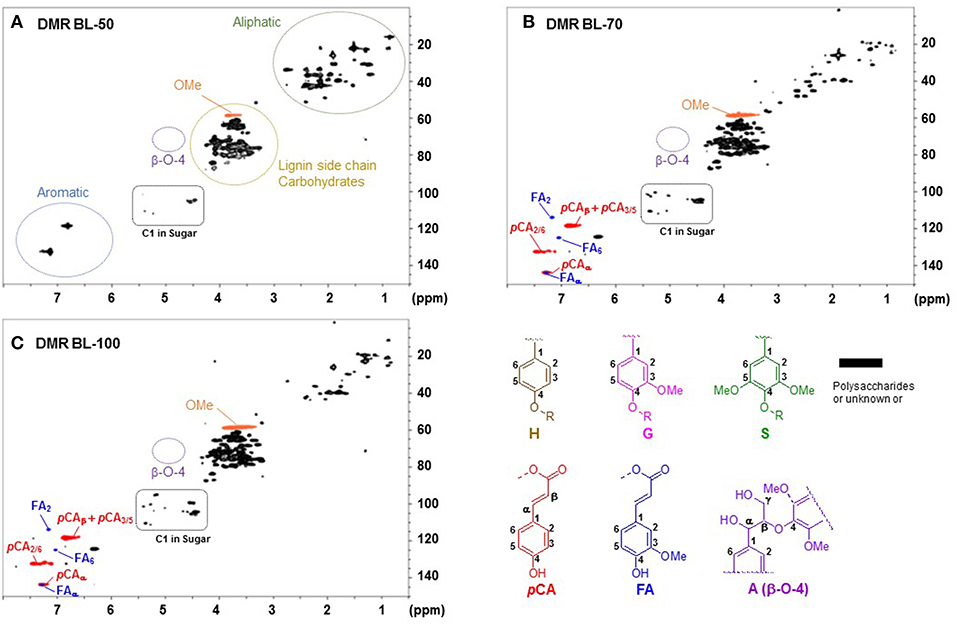
Figure 3. HSQC NMR spectra of three black liquor samples. (A) DMR BL-50, (B) DMR BL-70, and (C) DMR BL-100. H, p-hydroxyphenyl; G, guaiacyl; S, syringyl; A, β-aryl ether (β-O-4); pCA, p-coumarate; FA, ferulate.
In the region of aromatic peaks at δc/δH 6.0–8.0/110–150 ppm, BL-50 had two large unknown aromatic peaks which disappeared in BL-70 and BL-100. BL-70 and BL-100 had more peaks in the region including ferulate and p-coumarate units, suggesting that more ester bonds in the ferulate and p-coumarate units were cleaved with higher NaOH loading.
Characterization of Oligo-Saccharides in Black Liquor
It is well-known that glucan is composed of D-glucose monomers linked by glycosidic bonds. Compared to glucan, xylan is more complex in structure. Basically, xylan is a polysaccharide with a backbone composed of β-1,4 linkages between D-xylose residues. In addition, xylan backbone can be branched with D-glucuronic acid and D-glucuronic acid 4-O-methyl α-1,2 linked or D-arabinofuranose α-1,2 or α-1,3 linked. O-acetyl groups can also be found in the main chain of the xylan polymer. Some ferulic acid esters from lignin, which are attached to the O-5 of some arabinofuranose branches, can also be found in xylan.
As demonstrated by compositional data, two major sugar components in black liquor, i.e., glucose and xylose, existed in the form of oligomers. In order to get a qualitative view of the oligomomeric structures, we assessed these samples via MALDI TOF MS spectroscopy. The spectra of the three samples are similar. A representative spectrum of black liquor DMR BL-70 is presented in Figure 4. This MS spectrum gave a better understanding to the researchers of what the deacetylation brought to the biomass and specifically, to their oligosaccharide residues. As spectrum shows, all gluco- and xylo-oligomers in black liquor were identified as dimers and trimers, there were no oligomers with units more than three. This was quite different from Kraft black liquor which usually has much higher Mw (17–19 kDa) of xylan dissolved in the black liquor (Lisboa et al., 2005). In addition, no peaks assigned to acetyl or glucuronic acid branch groups were observed in spectrum indicating that the xylooligomers in black liquor didn't have these two functional groups. It also indicated that the acetyl groups on xylan had been removed by deacetylation process and released into the liquor as acetic acid. Other functional groups arabinofuranose or ferulic acid ester linkage might exist on the xylo-oligomers, but these have not been analyzed and added to the spectral library in our lab. Further investigation on these two branch groups will be done in the following enzymatic hydrolysis experiments by adding arabinofuranosidase and ferulic acid esterase. Characterization of these functional groups on xylooligomers will help find the enzyme cocktail formula to breakdown the oligosaccharides to their monomeric sugars. In the long run this could help increase the monomeric yields and help the utilization of black liquor.
Degradation of Oligomers in Black Liquor
The purpose of characterizing black liquor is finally realizing good utilization of the oligosaccharides and lignin in the liquor. For oligosaccharides in black liquor, due to limited utilizing capacity in nature, enzymatically breaking down oligomers into monomers should be explored. Since more xylo-oligomers were released into black liquor with the higher loading of NaOH in deacetylation process, breaking down xylooligomers is our priority. We expect that appropriate application of enzymes could be able to convert xylooligomers to xylose thus it could be utilized as carbon source in fermentation process for valuable products or chemically transformed to fuel intermediates.
Black liquor DMR BL-70 was subjected to enzymatic hydrolysis with a commercial hemicellulase Htec2 at 10 mg/g of enzyme loading. After 48 h of incubation at 50°C, it appeared that Htec2 was not as effective as we expected on conversion of xylo-oligomers in black liquor. As shown in Figure 5, 18% of xylooligomers were degraded while most was still remaining after digestion; TOF-MS spectra showed that the oligomers in black liquor were mainly short chain oligomers, therefore adding more exo-xylanase, i.e., β-xylosidase, might help break the xylooligomers since xylosidase can work on xylooligomers hydrolyzing the β-1,4 linkage from the non-reducing terminal releasing D-xylose residues. However, no significant improvement in hydrolysis was observed with β-xylosidase addition (5 mg/g xylooligomer loading, Figure 5), indicating other structural factors, for example, the branch groups might hinder the breakdown of xylan. It is noteworthy that, compared to xylooligomers, gluco-oligomers were much easier to be degraded into monomers, adding Ctec2 (10 mg/g loading) to the black liquor led to complete breakdown of the gluco-oligomers to glucose.
As the compositional data demonstrated, in addition to sugars, there was considerable amount of lignin in the black liquor. The existence of lignin might inhibit the enzymatic hydrolysis of xylooligomers. In this context, removing the lignin from the black liquor was conducted by acidifying the black liquor to pH 3.0 and precipitating the lignin out by centrifugation. By doing this, 85~90% of lignin was removed from the black liquor. The black liquor was then re-adjusted to pH 4.8 and hydrolyzed by Htec2 again. However, it turned out that lignin was not inhibitory to the enzymatic hydrolysis as shown in Figure 6, as no improvement in oligomer hydrolysis was found after lignin was precipitated out. These results led us to think that the recalcitrance of xylooligomers in black liquor was likely due to their structures, more specifically, the branch groups on the xylan. Further investigation was done targeting at these branch groups.
It is known that xylan hydrolysis requires a variety of enzymes including: endoxylanase, which randomly chop xylan backbone producing xylan oligomers and small moiety of free xylose; β-xylosidase, which hydrolyzes the β-1,4 linkage from the non-reducing terminal releasing D-xylose residues and some accessary enzymes including: α-arabinofuranosidase, which acts on the non-reducing terminal releasing arabinofuranoside residues; acetyl xylan esterase, which removes acetyl group from the xylan; α-glucuronidase, which hydrolyzes α-1,2 bonds between glucuronic acid and xylose; and ferulic acid esterase, which detaches the ferulic acid from arabinofuranose sugar. Earlier MS analysis demonstrated that no glucuronic acid or acetyl group remaining on xylooligomers after deacetylation. Considering the possible existence of arabinofuranose and ferulic acid ester linkage on the xylooligomers, we decided to add xylan-degrading accessary enzymes to the hydrolysis reaction in addition to Htec2 and xylosidase to check the xylooligomer degradation. The de-branching accessary enzymes were loaded as described in Methods.
As Figure 7 shows, adding accessary enzymes to Htec2 did improve the xylooligomer conversion. By adding totally four accessary enzymes i.e., arabinofuranosidase (ABF), acetyl xylan esterase (AX), alpha-glucuronidase (α- GLU) and ferulic acid esterase (FAE), the xylooligomer degradation increased from 18 to 57%. If only ABF and FAE added, targeting at arabinofuranose and ferulic acid ester linkage, respectively, 51% of xylooligomers were broken into monomers, which was much higher than the hydrolysis by Htec2 only (18%). This indicates that the functional branch groups are largely accounted for the recalcitrance of xylooligomers to enzymatic attack in black liquor. Further checking the hydrolyzed products by HPLC, revealed that lot of arabinose were released after adding ABF while no ferulic acid were released after adding FAE. This suggests that arabinofuranose but not ferulic acid ester branch groups existed on the xylooligomers; and it was mainly the breakage of arabinofuranose branch group that contributed to further hydrolysis of xylooligomers. Thus, combined with the MS spectra analysis above, we conclude that the xylan released into the black liquor after deacetylation process were mainly short chain xylooligomers with arabinofuranose branch group, and adding accessary enzyme ABF could greatly enhance the breakdown of the xylooligomers in the black liquor.
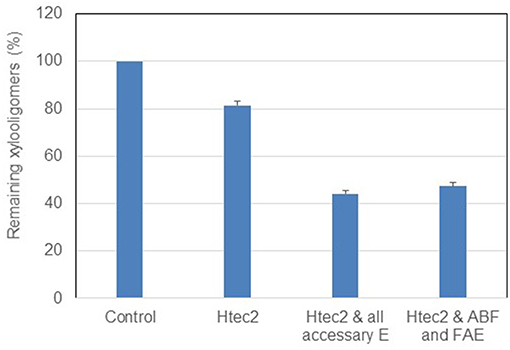
Figure 7. Enzymatic degradation of xylooligomers in black liquor with supplement of accessary enzymes.
However, it is noteworthy that after enzymatic digestion, about 40% of xylooligomers still remained undigested in the black liquor. Increasing the enzyme loading of Htec2 and accessary enzymes didn't help further improve the xylooligomer degradation. This indicates that those xylo-oligomers were quite recalcitrant to enzymatic hydrolysis or some compounds in black liquor inhibited the enzymatic digestion. The above experiment showed that the lignin in the black liquor didn't have much negative effect on the enzymatic digestion. It is more likely that the remaining xylooligomers are very recalcitrant, maybe due to the short chain structure of the xylooilgomers which made it difficult for the enzyme to bind on the xylooligomers and cleave the xylosidic linkage. Same phenomenon was also observed in our research experience in trying to breakdown the xylooligomers in dilute acid pre-treated slurry generated in our lab (data not shown), that 30~40% of xylooligomers still remained undigested no matter increasing dosage of enzyme or extending oligomer hold time were applied. To ferment the biomass-derived hydrolysate for biofuel/biochemical production, completely breaking down the oligosaccharides is very necessary because few microorganisms have the capacity to digest the oligosaccharides. Further investigation unveiling the recalcitrance of these xylooligomers has to be done, including studies on kinetics of xylooligomers/enzymes interaction. In order to realize real practice of enzymatic deconstruction of xylooligomers in black liquor, cost-effective production of accessary enzymes should also be considered. Meanwhile, alternative way to enzymatic hydrolysis followed by biological fermentation of black liquor should be explored. Direct thermochemical conversion and upgrading of polysaccharides in black liquor could be promising, as for thermochemical route to upgrade the biomass-derived sugars, breaking polysaccharides into monomers maybe not necessary (Cai et al., 2014).
Conclusion
In order to utilize the organic compounds in the black liquor generated from deacetylation of corn stover, characterization of black liquor was first performed in this study. Compositional analysis showed that glucan, xylan, and lignin were three main components in black liquor. MALDI TOF-MS spectra revealed that the polysaccharides in the black liquor were basically trimers and dimers. No acetyl or glucuronic acid groups were detected on xylooligomers while arabinofuranose branch groups were proved existent on the xylooligomers in black liquor. Commercial cellulase enzymes Ctec2 could easily break the glucooligomers in black liquor into glucose while xylooligomers were more recalcitrant to hemicellulases. Adding accessary enzymes to hemicellulase Htec 2 could significantly increase the degradation of xylooligomers from 18 to 57%, indicating branch groups largely accounted for the recalcitrance of xylooligomers All these compositional, structural information plus the preliminary enzymatic saccharification results in this study will help us understand the potential value of black liquor and help us evaluate the possibility of utilizing the oligosaccharides in black liquor via enzymatic deconstruction. Overall, this work provides a baseline for potential utilization of solubilized components in the black liquor from DMR process waste stream.
Data Availability
All datasets generated for this study are included in the manuscript.
Author Contributions
WW, XC, and MT conceived and designed the experiments. WW and XC conducted enzymatic degradation of oligomers and wet chemistry analysis. RK performed NMR and GPC analyses. WW drafted the manuscript. XC, RK, and MT contributed to the manuscript preparation. The xylanase enzymes used in this study AX, α-GLU, and FAE were provided by courtesy of Dr. Steve Decker from Enzymology group in NREL. All authors read and approved the final manuscript.
Contribution to the Field Statement
The DMR process brought up by NREL proves to be a highly successful pre-treatment process. Meanwhile, the deacetylation step in DMR process to remove acetyl groups generates the black liquor mainly containing acetic acids, oligosaccharides and lignin. Although the black liquor has potential value to be utilized, the composition of black liquor and the structures of the components are not clear which poses challenges to the potential utilization in biorefinery. In this context, the presented study was designed to characterize the black liquor collecting compositional and structural information of black liquor via various analyses including GPC, NMR, and TOF-MS spectroscopy. The work especially focused on the dissolved hemicelluloses which accounted for most of the carbohydrates in black liquor. This is so far the first study about characterizing black liquor and exploring breaking down the oligosaccharides in black liquor for potential use. The compositional and structural information provided in this presentation, together with the enzymatic saccharification results of the xylooligosaccharides provide a baseline for potential utilization of black liquor from deacetylation process. Frontiers in Energy Research readers will view this work as an important contribution to the field.
Conflict of Interest Statement
The authors declare that the research was conducted in the absence of any commercial or financial relationships that could be construed as a potential conflict of interest.
Acknowledgments
This work was authored by Alliance for Sustainable Energy, LLC, the Manager and Operator of the National Renewable Energy Laboratory for the U.S. Department of Energy (DOE) under Contract No. DE-AC36-08GO28308. Funding provided by U.S. Department of Energy Office of Energy Efficiency and Renewable Energy Bioenergy Technologies Office. The views expressed in the article do not necessarily represent the views of the DOE or the U.S. Government. The U.S. Government retains and the publisher, by accepting the article for publication, acknowledges that the U.S. Government retains a non-exclusive, paid-up, irrevocable, worldwide license to publish or reproduce the published form of this work, or allow others to do so, for U.S. Government purposes.
References
Alekhina, M., Ershova, O., Ebert, A., Heikkinen, S., and Sixta, H. (2015). Softwood kraft lignin for value-added applications: Fractionation and structural characterization. Ind. Crops Prod. 66, 220–228. doi: 10.1016/j.indcrop.2014.12.021
Bankeeree, W., Akada, R., Lotrakul, P., Punnapayak, H., and Prasongsuk, S. (2018). Enzymatic hydrolysis of black liquor xylan by a novel xylose-tolerant, thermostable β-xylosidase from a tropical strain of Aureobasidium pullulans CBS 135684. Appl. Biochem. Biotechnol. 184, 919–934. doi: 10.1007/s12010-017-2598-x
Cai, C. M., Nagane, N., Kumar, R., and Wyman, C. E. (2014). Coupling metal halides with a co-solvent to produce furfural and 5-HMF at high yields directly from lignocellulosic biomass as an integrated biofuels strategy. Green Chem. 16, 3819–3829. doi: 10.1039/C4GC00747F
Chen, X., Katahira, R., Ge, Z., Lu, L., Hou, D., Peterson, D. J., et al. (2019). Microbial electrochemical treatment of biorefinery black liquor and resource recovery. Green Chem. 21, 1258–1266. doi: 10.1039/C8GC02909A
Chen, X., Kuhn, E., Jennings, E. W., Nelson, R., Tao, L., Zhang, M., et al. (2016). DMR (deacetylation and mechanical refining) processing of corn stover achieves high monomeric sugar concentrations (230 g L– 1) during enzymatic hydrolysis and high ethanol concentrations (> 10% v/v) during fermentation without hydrolysate purification or concentration. Energy Environ. Sci. 9, 1237–1245. doi: 10.1039/C5EE03718B
Chen, X., Kuhn, E., Nagle, N., Nelson, R., Tao, L., Crawford, N., et al. (2018). Recycling of dilute deacetylation black liquor to enable efficient recovery and reuse of spent chemicals and biomass pretreatment waste. Front. Energy Res. 6:51. doi: 10.3389/fenrg.2018.00051
Chen, X., Shekiro, J., Franden, M. A., Wang, W., Zhang, M., Kuhn, E., et al. (2012). The impacts of deacetylation prior to dilute acid pretreatment on the bioethanol process. Biotechnol. Biofuels 5:8. doi: 10.1186/1754-6834-5-8
Chen, X., Shekiro, J., Pschorn, T., Sabourin, M., Tao, L., Elander, R., et al. (2014). A highly efficient dilute alkali deacetylation and mechanical (disc) refining process for the conversion of renewable biomass to lower cost sugars. Biotechnol. Biofuels 7:98. doi: 10.1186/1754-6834-7-98
Collins, T., Gerday, C., and Feller, G. (2005). Xylanases, xylanase families and extremophilic xylanases. FEMS Microbiol. Rev. 29, 3–23. doi: 10.1016/j.femsre.2004.06.005
da Costa Sousa, L., Jin, M., Chundawat, S. P., Bokade, V., Tang, X., Azarpira, A., et al. (2016). Next-generation ammonia pretreatment enhances cellulosic biofuel production. Energy Environ. Sci. 9, 1215–1223. doi: 10.1039/C5EE03051J
Davis, R., Biddy, M., Tan, E., Tao, L., and Jones, S. (2013). Biological Conversion of Sugars to Hydrocarbons Technology Pathway. Golden, CO: NREL/TP-5100-58054. doi: 10.2172/1076636
de Assis Castro, R. C., Fonseca, B. G., dos Santos, H. T. L., Ferreira, I. S., Mussatto, S. I., and Roberto, I. C. (2017). Alkaline deacetylation as a strategy to improve sugars recovery and ethanol production from rice straw hemicellulose and cellulose. Ind. Crops Prod. 106, 65–73. doi: 10.1016/j.indcrop.2016.08.053
Giummarella, N., and Lawoko, M. (2017). Structural Insights on Recalcitrance during Hydrothermal Hemicellulose Extraction from Wood. ACS Sust. Chem. Eng. 5, 5156–5165. doi: 10.1021/acssuschemeng.7b00511
Hu, J., Davies, J., Mok, Y. K., Gene, B., Lee, Q. F., Arato, C., et al. (2016). Enzymatic hydrolysis of industrial derived xylo-oligomers to monomeric sugars for potential chemical/biofuel production. ACS Sust. Chem. Eng. 4, 7130–7136. doi: 10.1021/acssuschemeng.6b02008
Huang, C., He, J., Wang, Y., Min, D., and Yong, Q. (2015). Associating cooking additives with sodium hydroxide to pretreat bamboo residues for improving the enzymatic saccharification and monosaccharides production. Bioresour. Technol. 193, 142–149. doi: 10.1016/j.biortech.2015.06.073
Jones, B. W., Venditti, R., Park, S., and Jameel, H. (2014). Comparison of lab, pilot, and industrial scale low consistency mechanical refining for improvements in enzymatic digestibility of pretreated hardwood. Bioresour. Technol. 167, 514–520. doi: 10.1016/j.biortech.2014.06.026
Jones, B. W., Venditti, R., Park, S., Jameel, H., and Koo, B. (2013). Enhancement in enzymatic hydrolysis by mechanical refining for pretreated hardwood lignocellulosics. Bioresour. Technol. 147, 353–360. doi: 10.1016/j.biortech.2013.08.030
Kim, H., Ralph, J., and Akiyama, T. (2008). Solution-state 2D NMR of ball-milled plant cell wall gels in DMSO-d 6. Bioenergy Res. 1, 56–66. doi: 10.1007/s12155-008-9004-z
Kim, J. S., Lee, Y., and Kim, T. H. (2016). A review on alkaline pretreatment technology for bioconversion of lignocellulosic biomass. Bioresour. Technol. 199, 42–48. doi: 10.1016/j.biortech.2015.08.085
Kim, S. M., Dien, B. S., and Singh, V. (2016). Promise of combined hydrothermal/chemical and mechanical refining for pretreatment of woody and herbaceous biomass. Biotechnol. Biofuels 9:97. doi: 10.1186/s13068-016-0643-6
Lisboa, S., Evtuguin, D., Neto, C. P., and Goodfellow, B. (2005). Isolation and structural characterization of polysaccharides dissolved in Eucalyptus globulus kraft black liquors. Carbohydr. Polym. 60, 77–85. doi: 10.1016/j.carbpol.2004.11.024
Mansfield, S. D., Kim, H., Lu, F., and Ralph, J. (2012). Whole plant cell wall characterization using solution-state 2D NMR. Nat. Protoc. 7, 1579–1589. doi: 10.1038/nprot.2012.064
Niemelä, K., Tamminen, T., and Ohra-aho, T. (2007). Black liquor components as potential raw materials. TAPPSA J. 25–28.
Park, J., Jones, B., Koo, B., Chen, X., Tucker, M., Yu, J.-H., et al. (2016). Use of mechanical refining to improve the production of low-cost sugars from lignocellulosic biomass. Bioresour. Technol. 199, 59–67. doi: 10.1016/j.biortech.2015.08.059
Sjostrom, E. (1993). Wood Chemistry Fundamental and Applications. 2nd Edn. San Diego, CA: ACADEMIC PRESS, inc.
Sluiter, A., Hames, B., Ruiz, R., Scarlata, C., Sluiter, J., and Templeton, D. (2008a). Determination of Ash in Biomass. Golden, CO: NREL Laboratory Analytical Procedure (LAP).
Sluiter, A., Hames, B., Ruiz, R., Scarlata, C., Sluiter, J., Templeton, D., et al. (2008b). Determination of Structural Carbohydrates and Lignin in Biomass. Golden, CO: NREL Laboratory Analytical Procedure (LAP).
Sørensen, H. R., Pedersen, S., Jørgensen, C. T., and Meyer, A. S. (2007). Enzymatic hydrolysis of wheat arabinoxylan by a recombinant “minimal” enzyme cocktail containing β-xylosidase and novel endo-1, 4-β-xylanase and α-L-arabinofuranosidase activities. Biotechnol. Prog. 23, 100–107. doi: 10.1021/bp0601701
Wyman, C. E., Dale, B. E., Elander, R. T., Holtzapple, M., Ladisch, M. R., and Lee, Y. (2005). Comparative sugar recovery data from laboratory scale application of leading pretreatment technologies to corn stover. Bioresour. Technol. 96, 2026–2032. doi: 10.1016/j.biortech.2005.01.018
Keywords: deacetylation, black liquor, characterization, xylooligosaccharides, enzymatic saccharification
Citation: Wang W, Chen X, Katahira R and Tucker M (2019) Characterization and Deconstruction of Oligosaccharides in Black Liquor From Deacetylation Process of Corn Stover. Front. Energy Res. 7:54. doi: 10.3389/fenrg.2019.00054
Received: 28 February 2019; Accepted: 23 May 2019;
Published: 07 June 2019.
Edited by:
Anli Geng, Ngee Ann Polytechnic, SingaporeReviewed by:
Diogo M.F. Santos, Association of Instituto Superior Técnico for Research and Development (IST-ID), PortugalYong Xu, Nanjing Forestry University, China
Copyright © 2019 Wang, Chen, Katahira and Tucker. This is an open-access article distributed under the terms of the Creative Commons Attribution License (CC BY). The use, distribution or reproduction in other forums is permitted, provided the original author(s) and the copyright owner(s) are credited and that the original publication in this journal is cited, in accordance with accepted academic practice. No use, distribution or reproduction is permitted which does not comply with these terms.
*Correspondence: Wei Wang, V2VpLldhbmdAbnJlbC5nb3Y=; Xiaowen Chen, WGlhb3dlbi5DaGVuQG5yZWwuZ292