- 1Institute of Engineering, National Autonomous University of Mexico, Mexico City, Mexico
- 2Department of Environmental Science, Aarhus University, Roskilde, Denmark
This paper aims to determine the environmental performance of the current landfill gas management system (based on flaring) in Mexico City (Bordo Poniente) in comparison to the collection of biogas generated from solid waste through one of the most innovative technology implemented in Denmark (Aikan Technology), which produces biogas and digestate from waste. Two alternative scenarios are proposed in which the Aikan Technology is implemented in Bordo Poniente to replace the existing system (reference scenario) that involves landfilling inorganic waste and composting organic waste in Bordo Poniente with no biogas recovery. The biogas recovered through Aikan Technology is exploited to provide heat and electricity (Scenario 1) or biofuel for the transportation sector (Scenario 2). A comparative life cycle assessment (LCA) was performed to evaluate the environmental performances associated with all scenarios by evaluating the impact categories Climate Change, Fossil Depletion, and Cumulative Energy Demand. According to the waste capacity of Bordo Poniente, the implementation of the Aikan Technology would result in a total annual reduction of 0.14 and 0.08 Mt of CO2 eq for Scenario 1 and Scenario 2, respectively. Scenario 1 was also the best performing scenario with respect to fossil depletion (−52 kg oil eq ton−1 of organic waste) and cumulative energy demand (−3,557 MJ ton−1 of organic waste). The implementation of Aikan Technology in Mexico City represents an important challenge and requires further research into its economic and political feasibility. When it comes to tackling global environmental problems, international agreements ascribe high importance to environmental technology transfer. This study represents a preliminary step in terms of environmental assessment and thus provides important information for policymakers attempting to achieve more sustainable management of solid waste in Mexico.
Introduction
Mexico City is the most populated City in Mexico with a population in 2018 of about 21,580,000 inhabitants who represent about 16% of the total population of the country (WPR, 2018). Mexico City is experiencing a progressive increase in generation of solid waste (SW), primarily resulting from an increasing rate of industrial development, as well as the distribution, trade, and widespread consumption of various products for human use. Consequently, there is an increased demand for urban infrastructure, energy, and water, and efficient waste management systems are required. The City it is divided into 16 boroughs that collectively generate 12,893 tons of SW per year with a per capita daily SW generation of 1.46 kg (SEDEMA., 2014). The per capita generation of SW in Mexico City is higher than the national average (0.98 kg) and about 49.5% of this waste corresponds to organic waste (Duran Moreno et al., 2013).
Personnel with a low educational level operate the waste management system in Mexico City (Buenrostro and Bocco, 2003; Zurita et al., 2018). Generally, the SW is mixed and requires sorting prior to further treatment, thus increasing the total costs of the SW management system (Santibáñez et al., 2013). Costs of SW management arise from generation, storage, sweeping, and collection by vehicles with a loading capacity between 3,000 and 5,000 kg (Duran Moreno et al., 2013). The SW is then transported to sanitary landfills and composting plants. However, not all of the waste is properly managed. According to SEDEMA. (2014), 63% of waste is sorted at two sorting plants prior to transport to five sanitary landfills outside of Mexico City and eight composting plants. The remaining 37% of the mixed waste is deposited in uncontrolled landfill sites.
Bordo Poniente is the only sanitary landfill within Mexico City and it also hosts a composting plant. In December of 2011 the landfill portion of Bordo Poniente was closed, and therefore, beginning in 2012 it only received organic waste for composting. In 2014, the composting plant in Bordo Poniente received about 98% of the total organic waste produced in Mexico City (SEDEMA., 2014). Until 2011, the methane generated from the landfill in Bordo Poniente was not being collected for energy, but was rather flared, that is, it was transformed to carbon dioxide and released into the atmosphere. Although post-treatment is still ongoing and the biogas that it is remaining in the landfill might still be exploited for providing energy (IPCC, 2006), a substantial amount of potential energy is currently lost.
Mexico is one of the leading providers of energy, primarily from fossil fuels (Ruiz et al., 2008; G20, 2017), whereas the production and utilization of renewable energy sources are still not entirely developed (Mexico Institute, 2018). The consumption of hydrocarbon fuels in Mexico has been increasing over time and in 2014 it resulted about 88.7% of the gross primary energy supply (Alemán et al., 2014). Therefore, an energy transition from fossil fuel to renewable energy is urgent (Cancino et al., 2016). In this context, transitioning the waste management system to more sustainably harvest and provide bioenergy will allow Mexico to reduce the environmental impacts associated with the waste and energy sector (Tsydenova et al., 2018).
More appropriate waste treatment and disposal technologies are in use, especially in developed countries (Starr et al., 2015; Mereki et al., 2016; Bundhoo, 2018). In particular, some promising and innovative technologies that produce biogas from waste are currently implemented in Denmark (Lybæk and Kyær, 2017; Thomsen et al., 2018). For instance, Aikan Technology is one of the most innovative technologies implemented in Denmark, producing biogas and digestate from waste. Biogas is exploited for electricity and heat production, and the digestate for high quality organic fertilizer products returned to agricultural soils (Denmark Country Report, 2017). International agreements ascribe a key role to environmental technology transfer, particularly when it is applied to global environmental problems (Chuffart, 2013; Coelho et al., 2018). However, technology transfer cannot succeed without a pre-assessment in terms of environmental, economic, technological, and socio-cultural performances.
In this paper we perform a life cycle assessment (LCA) to compare the environmental performances of the existing, and two alternative waste management systems in Mexico City. Our analysis evaluates the potential environmental advantages of implementing Aikan Technology that recovers biogas used for electricity, heat, and biofuel production in Mexico. The current system is taken as a reference scenario (business as usual, REF). Two alternative scenarios are proposed in which the Aikan Technology is implemented in Bordo Poniente to replace the existing composting technology, and is combined with transitioning the current post-treatment of the closed landfill gas by flaring, to collection that provides Mexico City with combined heat and electricity. In the Scenario 1 (S1) analysis, landfill gas collection and the Aikan Technology are used to produce compost and biogas that provide electricity and heat. In the Scenario 2 (S2) analysis, biogas recovered from the landfill and from the Aikan Technology is exploited to generate biofuel for the transport sector. The impact categories evaluated in this study are: Climate Change (CC), Fossil Depletion (FD), and Cumulative Energy Demand (CED).
Materials and Methods
Description of the System
In the REF system, biogas is flared and compost is produced. In S1, biogas recovery is exploited to provide heat and electricity. In S2, biogas is exploited to produce biofuel for the transport sector. For alternative scenarios, the Aikan Technology is implemented to replace the Bordo Poniente composting plant. Moreover, the biogas that it is remaining in Bordo Poniente's landfill (closed in 2011) is collected and exploited for heat and electricity, providing a substitution for the coal-based energy supply in Mexico. The boundaries of the system considered in this study are presented in Figure 1.
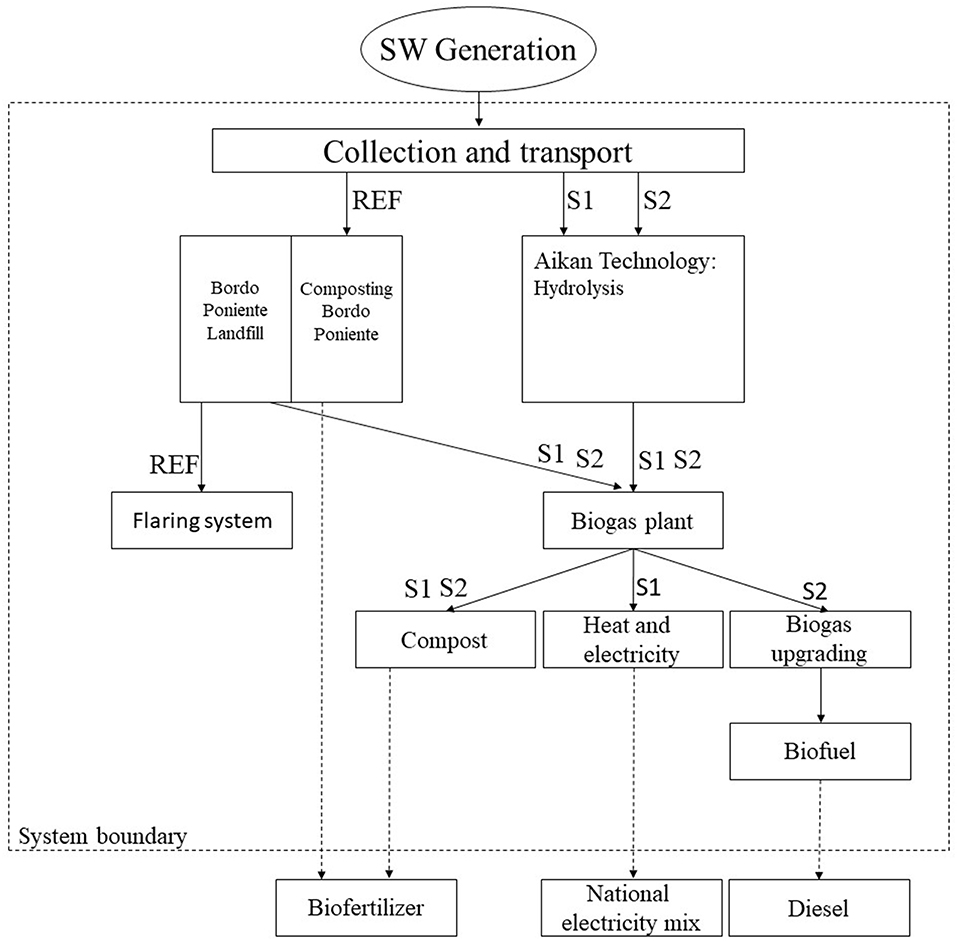
Figure 1. System boundaries. REF represents the reference scenario with compost production without biogas recovery; S1 represents biogas recovery and composting through Aikan Technology for electricity and heat providing; and S2 represents biogas recovery and composting through Aikan Technology for biofuel production for the transport sector. While in S1 the electricity produced is transferred to the national grid, and thus avoids the production and use of non-renewable energy, in S2 the production of biofuel replaces (in part) the use of diesel and thus reduce the utilization of fossil fuel in the transport sector.
Reference scenario refers to Bordo Poniente landfill, which is located 18 km northeast of Mexico City Center. Bordo Poniente receives an average of 12,500 tons of waste per day (Mejia-Dugand et al., 2013). Diesel-driven garbage trucks, with a loading capacity of 3,000–5,000 kg fresh weight, collect and transport the waste to the Bordo Poniente plant (Duran Moreno et al., 2013). Bordo Poniente landfill has been closed in 2011, but post-treatment is ongoing and involves flaring of methane emissions that could otherwise be exploited as an energy source (IPCC, 2006). Beginning in 2012, the Bordo Poniente plant only receives organic waste for composting (i.e., food and yard waste). The yearly disposal quantities of SW for the last six decades, and the SW composition, at the Bordo Poniente landfill can be found in Tables S1, S2 (see Supplementary Information).
The composting plant at Bordo Poniente has been in operation since 1988. It is the largest composting plant serving Mexico City with an annual capacity of about 912,500 tons of organic waste and an area of 30 hectares (Duran Moreno et al., 2013). The composting plant runs as an aerobic process with static piles and it processes the organic waste from households and gardens. The monthly quantities of organic waste that entered the composting plant and the quantities of compost produced in 2014 are presented in Table S3 (see Supplementary Information).
Key data including organic waste transportation from the transfer stations to the composting plant at Bordo Poniente, anaerobic digestion at the composting plant of Bordo Poniente, electricity and heat generation (S1), biofuel production for the transport sector (S2), energy utilization for biogas combustion, and upgrading are provided in Table S4 (see Supplementary Information).
Aikan Technology
The Aikan Technology consists of three phases: (i) hydrolysis, where solid organic matter is made soluble by microbial enzymatic degradation, (ii) methane production, where bacteria transform volatile organic matter into methane, and (iii) composting, where organic matter is sanitized and transformed into compost. The Aikan Technology starts with the separation of organic from the inorganic matter (e.g., plastic bags, metals) by means of a sorter. The organic waste is then mixed with wood pellets and yard waste, which are available in quantity at the landfill and improve the texture.
In both S1 and S2 the compost is used in the agricultural sector as a soil amendment that substitutes for some production and use of mineral fertilizer. In S1 the biogas is exploited to provide electricity and heat (Figure 1). The electricity produced is transferred to the national grid, and thus avoids the production and use of coal-based combined heat and power. The S1 scenario assumes the heat produced is discarded due to a lack of demand in Mexico City. In S2 the biogas is upgraded with the Aikan Technology and exploited to produce biofuel for use in the transportation sector (Figure 1). Globally, biogas is primarily used to provide heat and electricity (Petersson and Wellinger, 2009), though the use of biogas as a vehicle fuel is increasingly common in European countries and the United States (Börjesson and Mattiasson, 2008; Cong et al., 2017). In particular, as a biofuel we consider the production of compressed natural gas (CNG), which is used in heavy-duty vehicles (i.e., buses and trucks) as diesel substitute. We only included the operational phase; the construction phase is not included since the lifetime of the machinery is considered at least 30 years and therefore considered insignificant relative to an assessment of the biowaste management systems in 1 year.
Landfill
The methane generation from landfill is estimated by using the IPCC waste modeling according to IPCC (2006) (see Supplementary Information for more details about the estimation). The methane generation estimated from the landfill in REF, S1, and S2 is the same (about 255 Gg CH4 in 2011). However, in the REF, 97% of the generated methane is flared with no recovery and 3% is lost to the atmosphere (Borjesson and Berglund, 2006). In S1, 99% of the methane is collected for heat and electricity production and 1% of methane is lost to the atmosphere (Borjesson and Berglund, 2006). In S2, 98% of the methane is upgraded and used in the transportation sector and 2% is lost (Persson et al., 2006).
The CH4 emission from the Bordo Poniente landfill was estimated using a First Order Decay (FOD) model (IPCC, 2006). In this study, methane emissions were estimated for a period of 70 years (1960–2030). Methane emissions released from the landfill during the period 2011–2030 were estimated by taking into account the continued degradation of organic waste disposed before 2011. The estimation of disposed waste quantity in Bordo Poniente during the period 1960–2011 was developed using the per capita generation of waste by the Mexico City population. Data for population in Mexico City during the period 1960–2014 was provided by Borjesson and Berglund (2006). Although data for population was available for only some years (1960, 1970, 1980, 1990, 2006, 2009, and 2014), the population data in the remaining years were estimated by performing a linear interpolation per decade. For further information about the approach used for estimating methane emissions from the landfill see Supplementary Information (Chapter 1-IPCC waste modeling).
Composting
CH4 and N2O emissions are considered a part of the composting process. For estimating direct emissions from composting the amount of organic waste (i.e., food and yard waste) is required (IPCC, 2006). Data on the total amount of organic waste composted in 2014 was provided by the Environment Department of Mexico (SEDEMA., 2014) and is provided in Table S3 (see Supplementary Information). Default emissions factors (Tier 1) were used that correspond to 4 g CH4 kg−1 and 0.3 g N2O kg−1 of waste composted (IPCC, 2006), and were applied to calculate the emissions of the REF. In S1, the current composting process was replaced with the Aikan Technology for producing biogas and biofertilizer. In S2, biogas is upgraded and exploited to produce biofuel for use in the transport sector. Since no data was available for the NPK content of the Mexican compost, it was assumed that the NPK content of Mexican food, garden, and park waste was equal to the Danish values. The NPK content of the Danish compost produced with the Aikan Technology contains 15 kg nitrogen per ton, 5 kg phosphorous per ton, and 8 kg K per ton of dry weight (Kristensen, 2014). To estimate the fertilizer value we adopted substitution ratios of 95% and 63%, respectively according to Thomsen et al. (2017).
In S1 and S2, we considered a biogas yield of 80 Nm3 ton−1 of organic waste (wet weight), of which about 70% is methane (Kristensen, 2014). One ton of organic waste (wet weight) can be converted into about 290 kWh of electricity, 1,400 MJ heat, and 350 kg of organic fertilizer (Kristensen, 2014).
To produce biofuels from the biogas in S2, further purification is required. The most widely used technologies for biogas upgrading are pressure swing adsorption, water scrubbing, organic physical scrubbing, and chemical scrubbing (Petersson and Wellinger, 2009). All upgrading technologies are subject to methane losses that average to 3% (methane content between 96 and 99%) and an electricity consumption average of 0.25 kWh Nm−3 of biogas (Petersson and Wellinger, 2009). These values were used for the calculations of methane emissions in S2.
Life Cycle Assessment
An LCA study was performed in accordance with the international standards ISO 14040-44 (ISO, 2006a,b).
A functional unit of 1 ton (dry weight) of organic waste feedstock was used to quantify all inputs and outputs of the treatment processes in order to compare the resource use efficiency and environmental performance of the REF and alternative scenarios.
The LCA was performed in SimaPro 8.0.4 software (Pré Consultants, Amersfoort, Netherlands) and the integrated inventory was done in Ecoinvent v3.1 (Weidema et al., 2013), and it was evaluated using the impact assessment method ReCiPe (Goedkoop et al., 2013). Concerning the electricity, the specific Mexican medium voltage (average valid for Mexico e.g., “MX”) provided by Ecoinvent was used. Concerning the collection and transport of waste, diesel-driven garbage trucks collect, and transport the waste to the Bordo Poniente plant. As process, the municipal waste collection service by 21 metric ton lorry (average valid for all the countries e.g., “GLO”) was used. We estimated the distance of the transport as average distance between all the districts in Mexico City and Bordo Poniente. All the distances and the average distance estimated (16 km) are showed in Table S5 (see Supplementary Information). Regarding the process relative to the anaerobic digestion plant average global data from Ecoinvent as well as for Nitrogen, Phosphate, and Potassium fertilizers. Concerning the diesel in the S2 scenario the activity data provided from Ecoinvent was relative to the European average (“RER”).
Since waste treatment impacts are mainly climate change-related and environmental quality issues, we focused on the following impact categories:
• Climate change (CC), expressed in kg CO2eq,
• Fossil depletion (FD), expressed in kg oil eq,
• Cumulative energy demand (CED), expressed in MJ.
Results
The methane emissions from the deposited waste in the Bordo Poniente landfill during the period 1960–2030 increased from 1960 to the closing date in 2011. From 2011 to 2030, methane emissions decrease as the remaining organic degradable waste in the landfill continues to degrade (Figure 2).
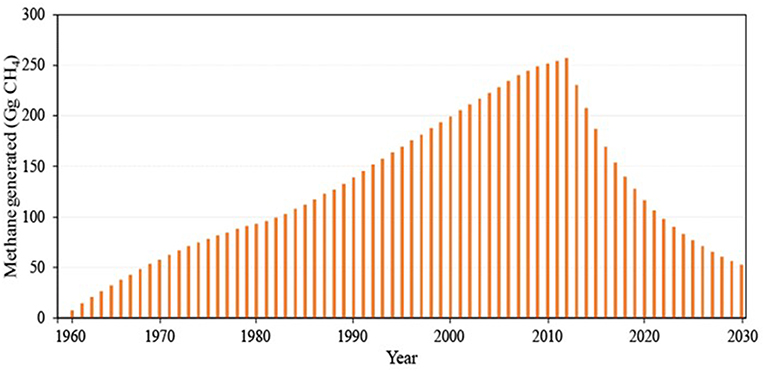
Figure 2. Methane emissions from deposited waste in BP landfill. The trend of methane emissions from deposited waste in Bordo Poniente landfill during the period 1960–2030 figure shows an increasing trend from 1960 to 2011 and a decreasing trend for the remaining years. It should be noted that Bordo Poniente landfill has been closed in 2011. From 2012, Bordo Poniente plant only receives organic waste for composting.
Figures 3–5 report the LCA results calculated for the climate change, fossil depletion, and cumulative energy demand, respectively. Positive performance values represent negative environmental impacts (a potential environmental burden or damage). Negative values represent avoided impacts, or positive impacts (a potential environmental benefit). In case of cumulative energy demand, a positive value corresponds to energy consumption whereas negative value is avoided energy consumption. The results of each impact category are examined via contribution analysis in the following sections.
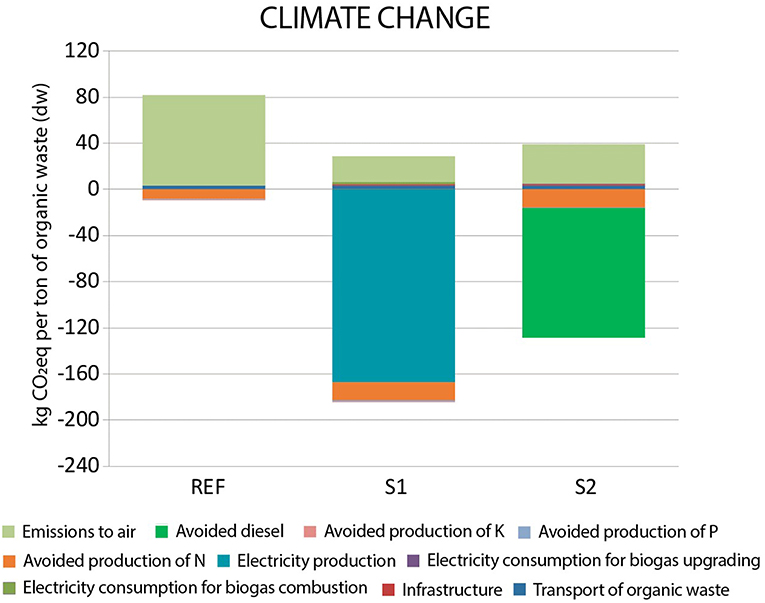
Figure 3. Comparison of the reference and alternatives scenarios for the category of climate change. Results are displayed as equivalent kg of CO2 per ton of dry weight organic waste.
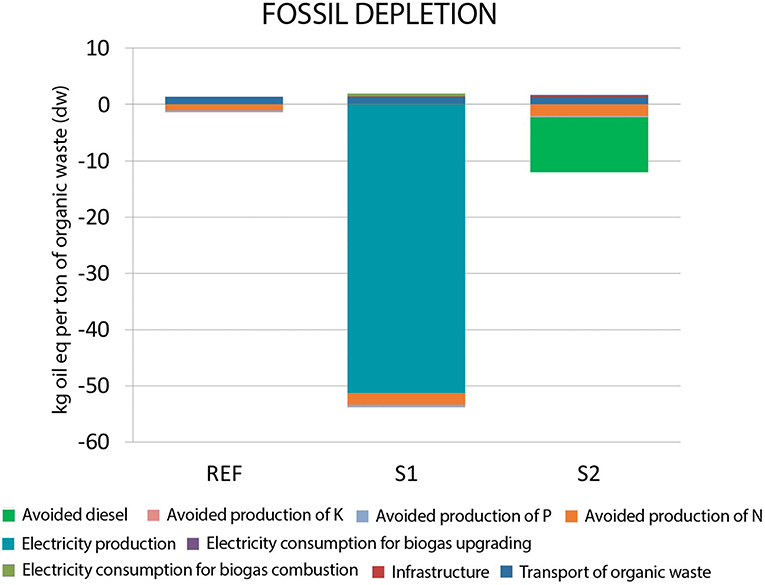
Figure 4. Comparison of the reference and alternatives scenarios for the category of fossil depletion. Results are displayed as equivalent kg of CO2 per ton of dry weight organic waste.
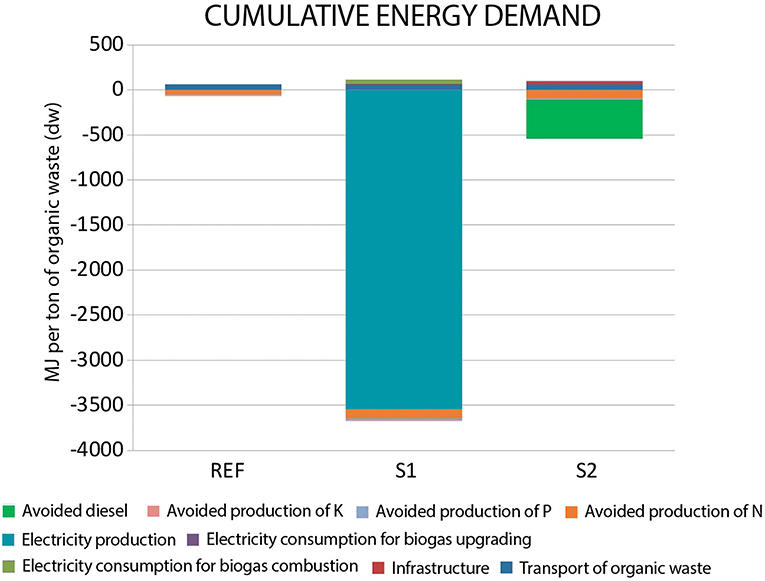
Figure 5. Comparison of the reference and alternatives scenarios for the category of cumulative energy demand. Results are displayed as equivalent kg of CO2 per ton of dry weight organic waste.
Climate Change
Biofuel production from alternative S1 was the best performing scenario, saving 155 kg CO2eq ton−1 dry weight organic waste, followed by alternative S2 saving 89 kg CO2eq ton−1 dry weight organic waste (Figure 3). Therefore, both alternative scenarios provide climate change impact reduction. The most relevant contribution in scenario S2 for avoiding impacts was the substitution of transportation fuel with biofuel, causing avoided emissions from diesel of 112 kg CO2eq ton−1 of organic waste (dry weight; Figure 3). In S1, electricity provided the largest contribution to the reduction of impact (−167 kg CO2eq ton−1 organic waste). Summarizing, in S1, a net negative CO2 emissions were due to the biogas-based electricity production, whereas in S2 the substitution of diesel-powered vehicles was the main driver of impact reduction.
For the REF scenario, the largest impact was represented by emissions into the atmosphere (77 kg CO2eq ton−1 of organic waste (Figure 3). However, production of N fertilizer drives a moderate decrease of net CO2 emissions (−8 kg CO2eq ton−1 of organic waste). Production of P and K mineral fertilizers represented insignificant reductions to net CO2 emissions.
Fossil Depletion
S1 was also the best performing scenario with regards to fossil depletion, scoring −52 kg oil eq ton−1 of organic waste (Figure 4). In particular, electricity production was the category determining the highest impact reduction with −51 kg oil eq ton−1 of organic waste (Figure 4). Other impact categories such as avoided production of N and P fertilizer also mitigated impact, however their contribution was less relevant (−2 and −0.3 kg oil eq ton−1 of organic waste, respectively).
A reduction of the impact was also found with the S2 scenario (−10 kg oil eq ton−1 of organic waste). In particular, the avoided emissions from diesel fuel were influential (Figure 4), causing −9.7 kg oil eq ton−1 of organic waste. Avoided production of N also contributed to the mitigation with −2 kg oil eq ton−1 of organic waste.
With the REF scenario, the impacts were roughly balanced (Figure 4). The kg of oil eq released from the transport of organic waste (1.31 kg oil eq ton−1 of organic waste) was near completely balanced by reductions caused by avoided production of N and P (−1.05 and −0.18 kg oil eq ton−1 of organic waste, respectively).
Cumulative Energy Demand
S1 was the best scenario in terms of cumulative energy demand reduction, with a decrease of 3,557 MJ ton−1 of organic waste, mainly due to electricity production (−3548 MJ ton−1 of organic waste; Figure 5). Avoided production of N and P contributed less to the decrease (−99 and −19 MJ ton−1 of organic waste, respectively). S2 also resulted in a reduction to cumulative energy demand with a decrease of 452 MJ ton−1 of organic waste (Figure 5). Avoided emissions from diesel (−436 MJ ton−1 of organic waste) and avoided production of N (−99 MJ ton−1 of organic waste) were the primary mitigating factors (Figure 5). Avoided production of P was a less relevant, causing a reduction of −8 MJ ton−1 of organic waste. For the REF scenario, the impact was roughly balanced (Figure 5). Cumulative energy used to transport organic waste (62 MJ ton−1 of organic waste) was reduced by avoided production of N and P (−50 and −11 MJ ton−1 of organic waste, respectively).
Discussion
The overall variations in LCA results among the three scenarios were mainly due to the different options proposed for biogas exploitation. Scenario 1 performed best in all three categories, and while S2 also provided impact reduction, it was lower than S1. The reference scenario was the worst performing in all three categories analyzed. The analysis revealed that implementing Aikan Technology, as a replacement for the existing composting technology in Bordo Poniente, would result in impact reductions associated with climate change, fossil depletion, and cumulative energy demand. Furthermore, we found that when Aikan Technology was exploited to produce compost and biogas for electricity and heat production (S1), the impact reductions were greater than when it was exploited to generate biofuel for the transportation sector (S2). Therefore, the production of electricity and heat was more important than the production of biofuel in terms of environmental impact mitigation. With regards to cumulative energy demand, the reduction of impact in S1 was about seven times larger than the reduction in S2. The Aikan Technology allows for the production of energy from waste while retaining valuable resources (i.e., N, P, and K) in the compost that is produced after the anaerobic digestion. The compost produced is an environmentally beneficial side product that can be used as a fertilizer on agricultural soil. However, we found that the impact reduction resulting from avoided production of N, P, and K was less relevant relative to the substitution of fossil fuel energy provided by S1 and S2.
Mexico is responsible for 1.6% of global fossil fuel power production, placing it in the top 15 (14th) of emitter countries (Atlas, 2018). Applying Danish technology can improve the environmental performance of Mexico by improving waste management and providing a potential new energy source. For instance, we found that both S1 and S2 scenarios would save a relevant quantity of CO2e ton−1 of organic waste compared to REF (Figure 3). According to the waste capacity of Bordo Poniente, implementation of the Aikan Technology would result in a total annual reduction of about 0.14 and 0.08 Mt of CO2e for S1 and S2, respectively. Therefore, the alternative scenarios presented are potential paths that could decrease GHG emissions in Mexico and contribute to the mitigation of climate change.
One of the biggest limitations in the coming years will be the separate collection of organic and inorganic waste. Keeping organic wastes separate allows for their reuse and recycling as biowaste. It also allows reducing the amount of waste dispatched to illegal open-dump sites (Meng et al., 2018). Likewise, electricity prices, grid interconnection, fuel production, and high capital start-up cost may present financial barriers, thus necessitating the maintenance of valuable materials and products within a sustainable circular economy (European Parliament, 2017; Velenturf and Jopson, 2019). A guideline on separate waste collection was adopted with new waste regulations in July 2017 and is expected to increase the amount of reused or recycled waste and decrease the amount of illegal waste disposal (Tsydenova et al., 2018). Policy regulations and instruments, such as subsidies from the National Council of Science and Technology of Mexico that support participation in the Horizon 2020 program (European Commission, 2017), are needed to enable waste valorization through the implementation of environmental technologies. Actions, including agenda in policy development, formulation, adoption, and evaluation, are also required to achieve the goal of reducing carbon emissions and waste generation while providing energy in a profitable manner. The study presented here demonstrates that the Aikan Technology provides a viable option to improve the waste management system in Mexico City, because of its established status as an advanced and innovative technology and high efficiency.
The United Nations Framework Convention on Climate Change has recognized the importance of technology transfer for achieving environmental impact mitigations. Since the COP 13 in Bali, technology transfer has also become one of the four pillars of the post-2012 climate change regime. Indeed, transfer of environmental related technology and information from developed to developing countries is needed especially because in the latter, municipalities face budget insufficiencies, lack knowledge, lack equipment, and have deficient infrastructure (Abarca et al., 2013). Although the challenge is difficult, some technology transfers have already been successfully implemented. For instance, within a period of 15 years, the Municipality of Durango has improved the overall management of its municipal solid waste by investing in and acquiring new technologies and equipment, and by outsourcing services (Valencia-Vazquez et al., 2014). Another similar project involved Korea transferring waste-to-energy technology to Santiago, Dominican Republic. This project focused on the production of renewable energy with high-energy waste from the city's Rafey landfill to provide a power supply that would foster commercialization in nearby communities and urban areas. However, cooperation among national and local governments proved challenging (Huh and Kim, 2018). While technology transfer and financial assistance, from developed countries to developing countries, are still hard to obtain, the Clean Development Mechanism may provide important opportunities. Under the Clean Development Mechanism, emissions-reduction projects in developing countries can earn certified emission reduction credits. Industrialized countries can meet a part of their emission reduction targets with these transferable credits (UNFCCC, 2018).
The implementation of Aikan Technology in Mexico City presents an important challenge that calls for further research, particularly in terms of economic and political feasibility. Such information is beyond the aim of this study. However, the information generated from this study provides a preliminary overview and is addressed to policymakers seeking to improve the environmental performance of Mexico. This study should also represent a starting point for policies intending to reach bilateral agreements with more developed countries such as Denmark (Coelho et al., 2018), and thus beginning the international exchange of information and strategies. Or, it may represent a first step toward an exchange of knowledge able to promote the development of new technologies aimed to achieve a more sustainable waste management.
Conclusions
This paper evaluated the environmental performance of the existing waste management system in Mexico City and compared it to the potential scenarios that implement Aikan Technology for recovering biogas used to produce electricity, heat, and biofuel. We concluded that the implementation of Aikan Technology in Mexico City would result in environmental impact reductions, such as climate change mitigation (−155 and −89 kg CO2eq ton−1 of organic waste for S1 and S2, respectively), fossil fuel depletion (−52 and −10 kg oil eq ton−1 of organic waste for S1 and S2, respectively), and cumulative energy demand (−3557 and −452 MJ ton−1 of organic waste for S1 and S2, respectively). The best option investigated would utilize the Aikan Technology to produce compost and biogas that is subsequently used to generate electricity and heat. However, the impact reductions are lower when biogas is exploited for generating biofuel.
This paper represents a first step toward a complete assessment of a potential technology transfer between Denmark and Mexico. As such, economic, political, and socio-cultural aspects are not considered in this study and further examinations are therefore needed. This study provides relevant information in terms of environmental performances and thus constitutes a preliminary reference point demonstrating the potential environmental benefits of technology transfer between developed and developing countries.
Author Contributions
CC: data analysis and paper writing; DC: paper writing; MT: paper writing and general supervision.
Conflict of Interest Statement
The authors declare that the research was conducted in the absence of any commercial or financial relationships that could be construed as a potential conflict of interest.
Acknowledgments
The authors appreciate financial support from the National Autonomous University of Mexico and the Department of Environmental Science at Aarhus University.
Supplementary Material
The Supplementary Material for this article can be found online at: https://www.frontiersin.org/articles/10.3389/fenrg.2019.00030/full#supplementary-material
References
Abarca, L. G., Maas, G., and Hogland, W. (2013). Solid waste management challenges for cities in developing countries. Waste Manage. 33, 220–232. doi: 10.1016/j.wasman.2012.09.008
Alemán, G., Casiano, V., Cárdenas, D., Díaz, R., Scarlat, N., Mahlknecht, J., et al. (2014). Renewable energy research progress in Mexico: a review. J. Renew. Sustain. Energy Rev. 32, 140–153. doi: 10.1016/j.rser.2014.01.004
Atlas (2018). The Global Carbon Atlas. Available online at: http://www.globalcarbonatlas.org/en/CO2-emissions 2-emissions (accessed September 2018).
Borjesson, P., and Berglund, M. (2006). Environmental systems analysis of biogas systems—Part I: fuel-cycle emissions. Biomass Bioenergy. 30, 469–485. doi: 10.1016/j.biombioe.2005.11.014
Börjesson, P., and Mattiasson, B. (2008). Biogas as a resource-efficient vehicle fuel. Trends Biotechnol. 26, 7–13. doi: 10.1016/j.tibtech.2007.09.007
Buenrostro, O., and Bocco, G. (2003). Solid waste management in municipalities in Mexico: goals and perspectives. J. Resour. Conserv. Recycling. 39, 251–263. doi: 10.1016/S0921-3449(03)00031-4
Bundhoo, Z. M. A. (2018). Solid waste management in least developed countries: current status and challenges faced. J. Mater. Cycles Waste Manag. 20, 1867–1877. doi: 10.1007/s10163-018-0728-3
Cancino, Y., Paredes, J., Gutiérrez, J., and Xiberta, J. (2016). The development of renewable energy resources in the State of Veracruz, Mexico. J. Utilities Pol. 39, 1–4. doi: 10.1016/j.jup.2016.01.001
Chuffart, S. (2013). Technology Transfer and Dissemination Under the UNFCCC: Achievements and New Perspectives. Columbia University Academic Commons. (accessed September 2018).
Coelho, S. T., Carmo, B. S., Holm-Nielsen, J. B., Lybæk, R., Garcilasso, V. P., Mariano, M., et al. (2018). “Challenges for biogas production and use in Brazil and lessons to learn from Denmark,” in European Biomass Conference and Exhibition Proceedings 2018 (26thEUBCE) (Copenhagen), 1493–1494.
Cong, R.-G., Caro, D., and Thomsen, M. (2017). Is it beneficial to use biogas in the Danish transport sector? An environmental-economic analysis. J. Cleaner Product. 165, 1025–1035. doi: 10.1016/j.jclepro.2017.07.183
Denmark Country Report (2017). IEA Bioenergy Task 37 Energy from Biogas. Available online at: file:///C:/Users/AU552179/Downloads/Denmark_Task%2037%20Country%20Report_Sep_2017.pdf
Duran Moreno, A., Garcés, M., Velasco, A., Marín, J., Gutiérrez, R., Moreno, A., et al. (2013). Mexico City's municipal solid waste characteristics and composition analysis. Rev. Int. Contam. Ambient. 29, 39–46. Available online at: http://www.scielo.org.mx/pdf/rica/v29n1/v29n1a4.pdf
European Commission (2017). Mexico Country Page. Available Local Programs or Funds That Could Provide Support to Mexican Horizon 2020 Participants. Available online at: http://ec.europa.eu/research/participants/data/ref/h2020/other/hi/h2020_localsupp_mexico_en.pdf
European Parliament (2017). Towards a Circular Economy – Waste Management in the EU. Scientific Foresight Unit (STOA). Available online at: at: http://www.europarl.europa.eu/RegData/etudes/STUD/2017/581913/EPRS_STU%282017%29581913_EN.pdf
G20 (2017). Mexico's Efforts to Phase Out and Rationalise its Fossil Fuel Subsidies. Available online at: https://www.oecd.org/site/tadffss/Mexico-Peer-Review.pdf
Goedkoop, M., Heijungs, R., Huijbregts, M., De Schryver, A., Struijs, J., and van Zelm, R. (2013). ReCiPe 2008, a Life Cycle Impact Assessment Method Wich Comprises Harmonised Category Indicators at the Midpoint and the Endpoint Level Report 1, Characterization. Ministry of housing, special planning and the environment, pp 133.
Huh, T., and Kim, H. J. (2018). Korean experimentation of knowledge and technology transfer to address climate change in developing countries. Sustainability. 10:41263. doi: 10.3390/su10041263
IPCC (2006). “2006 IPCC Guideline for National Greenhouse Gas Inventories,” in eds H. S. Eggleston, L. Buendia, K. Miwa, T. Ngara, and K. Tanabe (IGES: Japan). Available online at: http://www.ipcc-nggip.iges.or.jp/public/2006gl/index.html (accessed November 2017).
ISO (2006a). Environmental Management. Life Cycle Assessment. Principle and Framework. ISO14040:2006. International Organization for Standardization, Geneva.
ISO (2006b). Environmental Management. Life Cycle Assessment. Requirements and Guidelines. ISO14044:2006. International Organization for Standardization (ISO), Geneva.
Kristensen, B. M. (2014). Aikan Agri To-Faset Biogas og Kompostering af Organisk Affald Fra by og Land. Miljøprojekt nr. 1586. Available online at: https://www2.mst.dk/Udgiv/publikationer/2014/07/978-87-93178-74-8.pdf (accessed September 2018)
Lybæk, R., and Kyær, T. (2017). Enhancing identified circular economic benefits related to the deployment of the Solrød biogas plant. Eng. Appl. Sci. Res. 44, 97–105. doi: 10.14456/easr.2017.14
Mejia-Dugand, S., Hjelm, O., and Baas, L. (2013). Sustainable Business and Clean Technology Market in Megacities: An Explorative Research and Their Dynamics in Cairo and Mexico City (44 pp.). Available online at: https://www.diva-portal.org/smash/get/diva2:645158/FULLTEXT01.pdf (accessed September 2018).
Meng, Y., Ling, T. C., and Mo, K. H. (2018). Recycling of wastes for value-added applications in concrete blocks: an overview. Resour. Conserv. Recycl. 138, 298–312. doi: 10.1016/j.resconrec.2018.07.029
Mereki, D., Baldwin, A., and Li, B. (2016). A comparative analysis of solid waste management in developed, developing and lesser developed countries. Environ. Technol. Rev. 5, 120–141. doi: 10.1080/21622515.2016.1259357
Mexico Institute (2018). Mexico's Renewable Energy Future. Working Paper by Lisa Viscidi. Available online at: https://www.wilsoncenter.org/sites/default/files/mexico_renewable_energy_future_0.pdf
Persson, M., Jonsson O, and Wellinger, A. (2006). Biogas Upgrading to Vehicle Fuel Standard and Grid Injection. IEA Bioenergy. Task 37 - Energy from Biogas and Landfill Gas. Available online at: http://task37.ieabioenergy.com/files/daten-redaktion/download/publi-task37/upgrading_report_final.pdf
Petersson, A., and Wellinger, A. (2009). Biogas Upgrading Technologies – Developments and Innovations. IEA Bioenergy, task 37. Energy from biogas and landfill gas, 13.
Ruiz, B., Rodríguez-Padilla, V., and Martínez, J. (2008). Renewable energy sources in the Mexican electricity sector. J. Renew. Energy. 33, 1346–1353. doi: 10.1016/j.renene.2007.07.016
Santibáñez, J. E., Ponce, J. M., González, J., Serna, M., and El-Halwagi, M. (2013). Optimal planning for the sustainable utilization of municipal solid waste. J. Waste Manag. 33, 2607–2622. doi: 10.1016/j.wasman.2013.08.010
SEDEMA. (2014). Inventario de Residuos Sólidos CDMX - 2014. Secretaria del Medio Ambiente. Anexos. Páginas: 91–115. Available online at: https://www.sedema.cdmx.gob.mx/storage/app/media/programas/residuos-solidos/inventario-residuos-solidos-2014/IRS-2014.pdf (accessed November 2017)
Starr, K., Villalba, G., and Gabarrell, X. (2015). Upgraded biogas from municipal solid waste for natural gas substitution and CO2 reduction – A case study of Austria, Italy and Spain. J. Waste Manage. 38, 105–116. doi: 10.1016/j.wasman.2015.01.001
Thomsen, M., Romeo, D., Caro, D., Seghetta, M., and Cong, R.-G. (2018). Environmental-economic analysis of integrated organic waste and wastewater management systems: a case study from Aarhus City (Denmark). Sustainability. 10:3742. doi: 10.3390/su10103742
Thomsen, M., Seghetta, M., Mikkelsen, M., Gyldenkærne, S., Becker, T., and Caro, D. (2017). Comparative life cycle assessment of biowaste to resource management systems – A Danish case study. J. Cleaner Product. 2017, 1–9. doi: 10.1016/j.jclepro.2016.10.034
Tsydenova, N., Morillas, A. V., and Salas, A. A. C. (2018). Sustainability assessment of waste management system for Mexico City (Mexico)—based on analytic hierarchy process. Recycling. 3:45. doi: 10.3390/recycling3030045
UNFCCC (2018). United Nations Framework Convention on Climate Change. Available online at: https://cdm.unfccc.int/
Valencia-Vazquez, R., Pérez-López, M. E., Vicencio-de-la-Rosa, M. G., Martínez-Prado, M. A., and Rubio-Hernández, R. (2014). Knowledge and technology transfer to improve the municipal solid waste management system of Durango City, Mexico. Waste Manage. Res. 32, 848–856. doi: 10.1177/0734242X14546035
Velenturf, A. P. M., and Jopson, J. S. (2019). Making the business case for resource recovery. Sci. Total Environ. 651, 1495–1504. doi: 10.1016/j.scitotenv.2018.08.224
Weidema, B. P., Bauer, C., Hischier, R., Mutel, C., Nemecek, T., Reinhard, J., et al. (2013). Overview and Methodology. Data Quality Guideline for the Ecoinvent Database Version 3. Ecoinvent Report 1(v3). St. Gallen: The ecoinvent Centre.
WPR (2018). World Population Review. Available online at: http://worldpopulationreview.com/
Keywords: waste management, biowaste, biogas, life cycle (impact) assessment, Aikan technology
Citation: Chávez CS, Caro D and Thomsen M (2019) Environmental Assessment of Alternatives for Biowaste Treatment in Mexico City. Front. Energy Res. 7:30. doi: 10.3389/fenrg.2019.00030
Received: 06 August 2018; Accepted: 06 March 2019;
Published: 26 March 2019.
Edited by:
Federico Maria Pulselli, University of Siena, ItalyReviewed by:
Feni Agostinho, Paulista University, BrazilPriscila De Morais Lima, University of São Paulo (USP) at São Carlos School of Engineering, Brazil
Copyright © 2019 Chávez, Caro and Thomsen. This is an open-access article distributed under the terms of the Creative Commons Attribution License (CC BY). The use, distribution or reproduction in other forums is permitted, provided the original author(s) and the copyright owner(s) are credited and that the original publication in this journal is cited, in accordance with accepted academic practice. No use, distribution or reproduction is permitted which does not comply with these terms.
*Correspondence: Dario Caro, ZGFjQGVudnMuYXUuZGs=