- DBT-IOC Centre for Advanced Bioenergy Research, Research and Development Centre, Indian Oil Corporation Ltd., Faridabad, India
Multiple enzymes are required for efficient hydrolysis of lignocellulosic biomass and no wild type organism is capable of producing all enzymes in desired levels. In this study, steam explosion of wheat straw was carried out at pilot scale and a synthetic enzyme mixture (EnzMix) was developed by partially replacing the cellulase with critical dosages of commercially available accessory enzymes (β-glucosidase, xylanase and laccase) through central composite design. Highest degree of synergism (DS) was observed with β-glucosidase (1.68) followed by xylanase (1.36). Finally, benchmarking of EnzMix (Celluclast, β-glucosidase and xylanase in a protein ratio of 20.40: 38.43: 41.16, respectively) and other leading commercial enzymes was carried out. Interestingly, hydrolysis improved by 75% at 6 h and 30% at 24 h, respectively in comparison of control. By this approach, 25% reduction in enzyme dosage was observed for obtaining the same hydrolysis yield with opitimized enzyme cocktail. Thus, development of enzyme cocktail is an effective and sustainable approach for high hydrolysis efficiency.
Introduction
Second generation bioethanol has the potential to decrease our dependence on non-renewable fossil fuels (Satlewal et al., 2017, 2018a; Frankó et al., 2018; Kane and French, 2018). Since ligno-cellulosic biomass is naturally recalcitrant in nature, pretreatment at high temperature/pressure is usually required to improve hydrolysis (Agrawal et al., 2015b,c, 2017b; Sharma et al., 2015a). Steam explosion (SE) has been widely implemented as a pretreatment approach for a wide variety of lignocellulosic biomasses like wheat straw, rice straw, sugarcane bagasse, corn stover, poplar chips barley straw, eucalyptus, switchgrass, miscanthus etc. (Janzon et al., 2014; Sun et al., 2014; Jacquet et al., 2015; Pengilly et al., 2016; Zhai et al., 2016; Álvarez et al., 2017). The major advantage of SE is that, it is auto-catalyzed and considered as non-toxic and environment friendly method suitable for commercial application (Janzon et al., 2014; Sharma et al., 2015b) due to no addition or recovery/neutralization of chemicals during pretreatment, no corrosion issues and simple and economical operations (Ruiz et al., 2013).
The mechanism of SE has been discussed earlier suggesting that biomass cellular structure gets swollen with water at elevated temperature and pressures, causing cellulase hydration and hemicellulase depolymerization resulting in the formation of oligomers and monomers. Further, H-bonding starts weakening, allowing autoionization of water into acidic hydronium ions (H3O+) that act as catalysts and basic hydroxide ions (OH−). Moreover, hydronium ions are also generated from acetyl groups and uronic acid present in the biomass (Ruiz et al., 2017). In comparison to acid or alkali based pretreatments the capital expenditure is considered to be low with SE because no special kind of material of construction is required for SE. In addition very little or no inhibitors are generated during SE (Pielhop et al., 2016; Walker et al., 2018).
The heterogeneous nature of pretreated biomass requires synergistic actions of multiple and functionally different ligno-cellulolytic enzymes (like endoglucanases, exoglucanases, xylanases, mannases, esterases, arabinofuranosidases, etc.) for its efficient hydrolysis(Loman and Ju, 2016; Lee et al., 2017; Kane and French, 2018; Sanhueza et al., 2018). Although a number of factors are responsible for lower biomass hydrolysis high cellulase crystallinity, low enzyme accessibility, non-productive enzyme-lignin binding, competitive, and feedback inhibition of the enzyme have been predominantly reported (Agrawal et al., 2015b; Parnthong et al., 2018). Thus, usually a high amount of enzyme is required to achieve high biomass hydrolysis but it negatively affects the commercial viability of the process.
Development of synergistic enzyme cocktail is an emerging strategy for improving the biomass hydrolysis (Satlewal et al., 2008; Sánchez-Cantú et al., 2018; Sanhueza et al., 2018). It has been reported earlier that both exo and endo glucanases work synergistically to degrade cellulase into cellobiose and finally β-glucosidase hydrolyze cellobiose into glucose. Cellobiose is a stronger inhibitor than glucose and its accumulation causes feedback inhibition against most cellobiohydrolases and endoglucanases, and thus it becomes important to supply enough β-glucosidases to hydrolyze cellobiose to glucose in order to eliminate such inhibition (Wang and Lu, 2016). The sufficient amount of β-glucosidase may lead to alleviation of the feedback inhibition by cellobiose (de Oliveira Rodrigues et al., 2017). Other accessory enzymes like hemicelluloses, esterases, arabinofuranosidases, laccases remove the physical protective covering of the cellulase microfibrils thereby, improving the enzyme accessibility (Gonçalves et al., 2015). Since the xylo-oligomers produced from hemicelluloses inhibit the hydrolysis by either physical blocking of the enzyme or by competitive inhibition of the cellulases; an optimal amount of xylanase is also essential (Qing et al., 2010; Boyce and Walsh, 2018). The commercially available first generation enzyme preparations (like Spezyme CP, Celluclast, and Novozyme 188) have been found to be underperforming in hydrolyzing the xylo-oligomers and overcoming their inhibitory effects at reasonable dosages (Qing et al., 2010; Agrawal et al., 2015b). This is due to the fact that these commercial enzymes are usually derived from the filamentous fungi (Trichoderma) and contains high amount of exo and endo glucanases but with lower amounts accessory enzymes like (β-glucosidases and hemicellulose) (Agrawal et al., 2015b; Pierce et al., 2017; Wu et al., 2017). In addition to this, type of ligno-cellulosic biomass and the pretreatment approach bring in differences in their structural properties (Satlewal et al., 2018b). Hence, development of tailor-made enzyme cocktail according to the pretreatment approach and the type of biomass might assist in improving the biomass hydrolysis. Although, few studies have been carried out on enzyme synergy but very few have evaluated the commercial enzymes and steam pretreated wheat straw at pilot scale level. A few published reports are discussed here in-brief which might be referred for further details. Takano and Hoshino (2018) who optimized the commercial enzyme reagents for hydrolysis of the alkali pretreated rice straw have reported that a combination of “Cellulase Onozuka 3S,” “Cellulase T Amano 4,” and “Pectinase G Amano” improved the hydrolysis efficiency with a yield of 94%. Soleimani and Ranaei-Siadat (2017) reported that an optimize enzyme cocktail optimized via response surface methodology involved enzymes, including Exo-1,4-β-D-glucanases II (CBHII), Endo-1,4-β-D-glucanases II (EGII) and 1,4-β-D-glucosidases I (BGI) improved the degradation of pretreated bagasse. In a similar report, 14 commercially available cellulolytic and hemicellulolytic enzymes were procured and mixed together to generate many cocktail combinations. This approach of blending crude commercially available enzyme cocktails allowed a 4-fold reduction in total enzyme requirements to achieve high hydrolysis yields (Chundawat et al., 2017).
In this study, a comprehensive analysis of synergism between cellulase and accessory enzymes (xylanase, pectinase, β-glucosidases etc.) has been investigated to quantitatively evaluate their impact on hydrolysis of steam pretreated wheat straw (SEWS) at pilot scale. This work established the hypothesis that accessory enzymes have a critical role in biomass hydrolysis and their optimization is a significant tool to enhance fermentable sugar production (Sharma et al., 2017). Further, the in-house developed enzyme mixture (EnzMix) was benchmarked with the latest commercial enzyme preparations of the leading manufacturers.
Materials and Methods
Biomass, Chemicals, and Enzyme Preparations
Wheat straw (WS) was procured locally from Faridabad, Haryana, India. It was milled to 1–2 mm sizes using cutting mill (Texol, Pune, India) and stored in airtight plastic bags at room temperature. All analytical grade chemicals (like cellobiose, glucose, xylose, arabinose, furfural, hydroxymethyl furfural, acetic acid, and BCA-1) and ligno-cellulolytic enzymes cellulase (Celluclast 1.5L), β-glucosidase (i.e., BGL or Novozym 188 in this study), xylanase, pectinase, and laccase were procured from Sigma Aldrich, India. Other enzyme preparations like Cellic from Novozymes, Denmark; Accellerase from Genencor Dupont, USA and by Indian manufacturers like Sacchari-SEB-C6 from Advanced Enzyme, Mumbai; Bioconvert L1 and Bioconvert P 10 from Noor Enzymes, West Bengal; were either procured or kindly provided by them as a gift.
Pilot Scale Steam Explosion
Steam explosion plant contains in-house designed high pressure reactor of 10 L capacity, a steam generator (~300°C) equipped with quick feeding valve, cyclone separator and noise absorber. In order to quickly attain the desired temperature, steam explosion digester was flushed 3–4 times with high pressure steam (15 bar). Impregnation of WS was carried out in batches of 15 kg by placing it in a perforated mesh bin made up of stainless steel (SS) and dipping it into the water. The liquid was circulated using a pneumatic pump for each experiment for 30 min at room temperature (30°C). The soaked wheat straw was dewatered using the hydraulic press at a pressure of 100 bars. The pressed wheat straw was properly mixed to determine moisture content using a moisture analyzer (MA 150 Sartorius, Germany). 0.4 kg of pressed wheat straw on a dry basis was fed to reactor and temperature was increased by injecting of high pressure steam. The WS was pretreated at 200°C temperature for 10 min (Agrawal et al., 2014; Sharma et al., 2015b). After pretreatment, the wheat straw slurry was recovered from the cyclone separator, and separated into two fractions, xylose rich liquid hydrolysate and glucan rich solid residue. Thorough washing of SEWS residue was carried out with about five times of the distilled water to remove free sugars and inhibitors. The washed SEWS was kept in air tight plastic bags at 4°C till further use. Since, this is customized pilot plant and we have designed our process in such a way that minimum delay takes place in achieving the desired temperature and pressure within 2 min and cooling down to ambient temperature/pressure almost instantly within few seconds. A pre flushing of the reactor with high pressure steam (15 bar) also help in reducing the time delay. Further, we have conducted a series of experiments earlier and optimized our process. A study on process optimization has been published earlier and might be referred for any details (Sharma et al., 2015b).
Analysis
Biomass composition analysis to determine the cellulase, hemicelluloses, lignin ash, and extractives was carried out by using a standard analytical protocol by Sluiter et al. (2008). Sugar oligomers were estimated by a method developed by National Renewable Energy Laboratory (NREL) (Sluiter et al., 2006, 2008). All high performance liquid chromatography analysis was carried out with HPLC system from Waters, USA equipped with a refractive index detector (Model No. 2,414, Waters) and Aminex HPX-87H column 300 × 7.8 mm ID (Bio-Rad Labs, Hercules, CA). All analysis was conducted by preparing calibration curves at different concentrations of high purity sugar standards (D-cellobiose, D-(+)glucose, D-(+)xylose, D-(+)galactose, L-(+)arabinose, and D-(+)mannose) in the HPLC (Sluiter et al., 2008). Miller (1959) protocol was used to determine the total reducing sugar using glucose as a standard and absorbance was observed at 540 nm. All analyses were conducted in triplicate and the standard deviation determined.
Enzyme Activities and Protein Estimation
Standard assay procedures were used to determine the enzyme activities present in enzyme preparations used in this study. Cellulase activities such as, BGL, filter paper unit (FPU) and endo-glucanase were estimated with p-nitrophenyl-beta-D-glucoside (pNPG), filter paper, and carboxy-methyl cellulase as substrates, respectively (Ghose and Bisaria, 1979; Agrawal et al., 2013a, 2017a). The xylanase, pectinase and laccase assay was conducted by using oatspelt xylan, pectin and 2,2′-azinobis(3-ethylbenzothiazoline-6-sulfonic acid) diammonium salt (ABTS) as substrates as described by Ibrahim et al. (2014) and Zhao et al. (2014). BCA-1 kit purchased from Sigma, USA was used for protein estimation in all enzymes (Walker, 1994; Agrawal et al., 2012).
Enzyme Cocktail Development
A comparison of SEWS enzymatic hydrolysis was carried out with basic cellulase preparation obtained from Trichoderma reesei (Celluclast) and its combination with different concentrations (10–125 mg protein/g biomass) of accessory enzymes (BGL, Xylanase, Pectinase, and Laccase) in 500 mL Erlenmeyer flasks at 10% (w/w) solid loading in a total volume of 150 mL and pH was maintained by adding sodium citrate buffer of 0.05 molarity (4.8 pH) (Agrawal et al., 2017b,c).
The percent hydrolysis yields were determined by estimating the total reducing sugars by following formula (Satheeja Santhi et al., 2014):
Hydrolysis (%) = Concentration of reducing sugar (g) × 0.9 × 100
Carbohydrate content of the biomass (g)
A statistical approach of response surface methodology with central composite design (CCD) with replicates at the center and star points were used with Design-Expert (Stat-Ease, Inc., Minneapolis, USA) software to optimize the enzyme cocktail/mixture (EnzMix) The level of factors for RSM are tabulated as Table S1. Here, Celluclast was partially replaced with accessory enzymes and its impact on hydrolysis yield was observed by using a method discussed in Agrawal et al. (2015b). We have determined the free sugars present in the commercial enzyme samples and subtracted this amount while calculating the hydrolysis yield.
Benchmarking With Commercial Enzyme Preparations
The in-house developed enzyme mixture (EnzMix) was benchmarked with its commercially available counterparts at same conditions (10% biomass loading, 50°C temperature, and 4.8 pH), feedstock (SEWS) and concentration i.e., 49 mg protein/g biomass.
Results and Discussion
Wheat Straw Pretreatment and Chemical Composition
Steam explosion (SE) pretreatment of milled WS was carried out at high temperature and pressure usually between 180 and 230°C (Ruiz et al., 2008) with a sudden release steam in a flash tank. It is an “auto catalyzed” process, where acetic acid released from hemicellulose and self-ionization of water at elevated temperatures causes a drop in pH which acts as a catalyst (Sharma et al., 2015b). SE has been reported to break down biomass structure and develop pores for higher enzymatic accessibility (van der Zwan et al., 2017). During SE most of the hemicellulose is hydrolyzed to soluble sugar monomers and some fraction of cellulase also gets depolymerized into glucose while, a small fraction of lignin is dissolved and/or redistributed (Kumar and Murthy, 2011; Agrawal et al., 2013b). The composition of untreated and pretreated wheat straw is depicted in Figure 1.
After steam explosion pretreatment, about 31% increase was observed in cellulase content of SEWS with a slight increase in lignin (2%) however, the hemicellulose content was reduced substantially (11%) in comparison to untreated wheat straw and extractives were almost fully removed. Previously it has been reported that after steam pretreatment of rice straw (180°C, 0.5% sulfuric acid and 10 min residence time), cellulase content was increased by 21% and hemicellulose content was decreased by 9% with a slight increase in lignin (3%) (Sharma et al., 2015b). Kristensen et al. (2008) found that after SE of wheat straw (190°C, 3.5% of sulfuric acid/biomass and 6 min residence time), xylan content was reduced to about 17% as compared to the native biomass. Consequently, the cellulase content was increased by 17% while lignin was increased by 1%. In another study with sweet potato vine as a substrate, cellulase content increased from 17% (untreated) to 40–49% (SE treated), accompanied with the reduction of “lignin” from 43% to 20–25% and hemicellulose from 10 to 16% (Liu et al., 2017).
Analysis of Different Enzyme Preparations
Comparative analysis of different enzyme preparations was carried out and shown in Table 1. Both Cellulclast and BGL were available in liquid form and contained almost similar protein content, while xylanase was present in solid form and contained lower protein. Maximum cellulase activity (FPU) was found in Cellulclast followed by less than half in BGL and negligible activity in other accessory enzymes. This showed that accessory enzymes have very low cellulolytic potential. Pectinase and laccase contained 3,785 U/ml and 83 U/g of specific activity, respectively.
SEWS Hydrolysis
Hydrolysis With Celluclast
A rapid increase in hydrolysis was observed with increasing Celluclast concentration from 10 to 25 mg protein/g biomass but only marginal increase was observed at high protein dosage (more than 40 mg protein/g biomass) (Figure S1). It showed that even very high dosage of cellulase alone is not sufficient to achieve the high hydrolysis. Similar to above results, Hu et al. (2011) have reported that hydrolysis yields increased linearly with Celluclast dosage up to 35 mg/g and reached to a stationary phase after which no substantial increase was found above this dosage. This might be due to a lower amount of accessory enzymes in Celluclast.
Effect of Supplementing Celluclast With Accessory Enzymes
As Celluclast concentration of 10 mg protein/g biomass is sufficient to achieve 60% SEWS hydrolysis yield in 24 h, it was used as the baseline for any further improvement by supplementing the accessory enzymes. The primary objective of this experiment is to identify the amount of accessory enzymes to obtain at least 80% hydrolysis yield. Results showed that supplementation of even small amount of BGL (10 mg protein) in Celluclast is sufficient to achieve >80% hydrolysis and no further substantial increase was found by increasing the BGL dosage after 48 h (Figures 2A–C). Supplementation of Celluclast with BGL at 10 mg protein enhanced the biomass hydrolysis by 34% after 6 h. Berlin et al. (2007) also reported that corn stover hydrolysis has been improved by 37% by BGL supplementation.
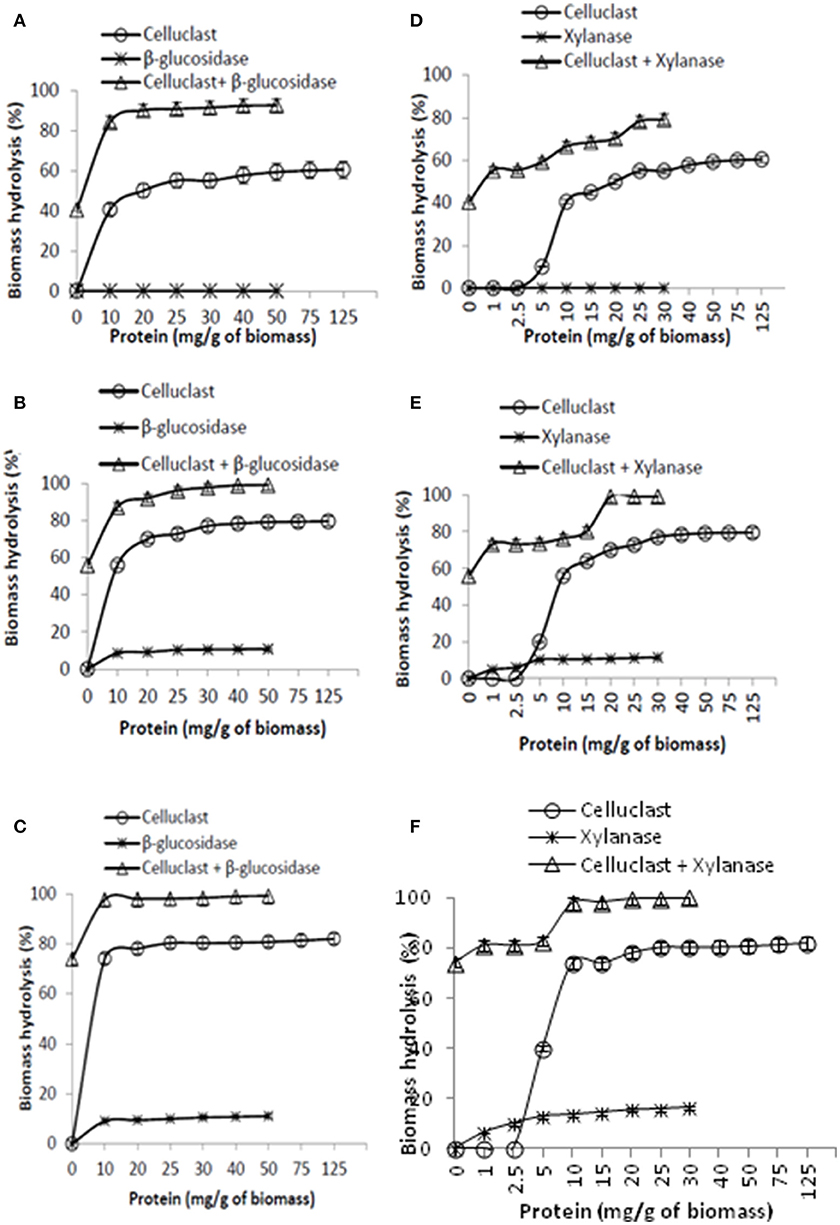
Figure 2. Supplementation of Celluclast with β-glucosidase (A–C) and xylanase (D–F). The corresponding hydrolysis time for (A,D), (B,E), and (C,F) is 6, 24, and 48 h, respectively.
A gradual increase in hydrolysis yield was also observed by increasing the xylanase concentration from 1 mg protein to 30 mg/g biomass (Figures 2D–F). It has been found that a 15% increase was observed at low xylanase dosage (1 mg protein). Results also indicate that higher concentration of xylanase is required to achieve maximum hydrolysis yield in minimum time like 30 mg of xylanase is required to obtain 80% hydrolysis in 6 h but 10 mg of xylanse is sufficient to achieve >95% hydrolysis in 48 h. Previous reports have also discussed that a substantial increase in steam pretreated corn stover (16%) and sugarcane baggase (31%) hydrolysis yield was observed with xylanase supplementation (Hu et al., 2011; Li et al., 2014). Surprisingly, pectinase, and laccase showed a negative effect on SEWS hydrolysis (data not shown) hence, only BGL and xylanase were selected for further optimization in this study. The negative effect of pectinase and laccase might be due to intensive competition between enzymes for cellulase binding sites and a slight inhibition of β-glucosidase activity. Recently, Oliva-Taravilla et al. (2014) reported that the relative glucose recovery in presence of laccase with cellulosic substrate (Sigmacell and steam exploded wheat straw) was almost 10% lower than in the absence of laccase. Yang et al. (2016) reported that the ratios and cooperation of cellulases are crucial in the hydrolysis and the efficiency could be further improved after adding accessory enzymes such as oxidoreductases, expansions, for which the bacterial enzyme pool is so abundant to excavate in the future(Agrawal et al., 2015d).
Synergistic Enzyme Cooperation
The degree of synergism (DS) was used to elucidate the synergistic cooperation of cellulases and accessory enzymes to improve the biomass hydrolysis yields. It was calculated as the proportion of hydrolysis yield obtained with synergistic enzyme mixture to the sum of the hydrolysis yield achieved with respective enzymes when used separately in the same amounts as in the mixture (Li et al., 2014). Maximum synergism was found between Celluclast and β-glucosidase (1.68) followed by xylanase (1.36) (Figure 3). These results indicate that the addition of β-glucosidase and xylanases substantially boost cellulases hydrolyzing ability and maximum synergism was obtained at 6 h of hydrolysis and it decreased with time. The reason for this might be the rate of hydrolysis in initial hours is in log phase where a maximum effect of the enzyme is observed. Similarly, previous report suggested that high DS (1.05–1.19) was found between Celluclast and xylanase for the hydrolysis of steam exploded sugarcane bagasse (Li et al., 2014). In another report, 1.43 DS was reported in an optimized enzyme mixture containing cellulase, xylanase, and pectinase (Bunterngsook et al., 2017). Recently, published report also suggested that better DS was observed during the initial hydrolysis stage but it decreased in the later hydrolysis stage (Huang et al., 2018). In addition, it was observed that the digestibility of cellulase was increased with the digestibility of xylan in the hydrolysis of pretreated sugarcane bagasse substrates (Huang et al., 2018). Similarly, supplementing commercial cellulase (Sigma-Aldrich, USA) with BGL was compared for the hydrolysis of microcrystalline cellulase, native corn straw and steam pretreated corn straw and the results showed an increase in glucose release by 62, 94, and 95%, respectively (Zhang et al., 2017).
Enzyme Cocktail Optimization
Optimization of enzyme cocktail was carried out by using a statistical tool known as response surface methodology. Since, only two enzymes i.e., β-glucosidase and xylanase showed synergism with Celluclast; these two have been used for further enzyme cocktail development. The experimental design and experimental results are depicted in Table S1. Equations (1) and (2) depict the correlation between the significant variables and response i.e., hydrolysis in 6 and 24 h.
Hydrolysis % (6h) = 79.39 + 2.38*A + 17.11*B - 0.76*AB - 4.17*A2 - 0.44*B2 (1)
Hydrolysis % (24 h) = 84.81 + 4.94*A + 15.68*B - 3.20*AB - 4.47*A2 - 2.56*B2 (2)
Where, A is BGL and B is xylanase concentration in terms of protein mg/g of biomass.
This model was found to be statistically significant as the p-value for 6 h (<0.0001) and 24 h (0.00) hydrolysis was greater than α = 0.05. ANOVA was used to assess the impacts of variables and their interactions for 6 and 24 h saccharification (Tables S2A,B). Model terms (A, B, and A2) were significant for both 6 h and 24 h periods. This model could be used to navigate the design space since the predicted R2 value was in agreement with the adjusted R2 value. Also the adequate precision value, which measures the signal to noise ratio, was above the threshold limit of 4 for both 6 h (18.1) and 24 h (19.8) models.
As predicted by the model and validated by experiments, 80% SEWS hydrolysis was observed by using very high amount of Celluclast (>100 mg protein/g biomass). But partial replacement of Celluclast with small amounts of accessory enzymes [Celluclast (10 mg), BGL (18.83 mg), and xylanase (20.17 mg)] as determined with the help of statistical RSM model is helpful in improving the SEWS hydrolysis yields up to 95% at low total protein loadings (49 mg) (Figure S1). The previous report also suggests that partial replacement of cellulase with accessory enzymes like BGL and xylanase has improved the pretreated wheat straw hydrolysis up to 80% as compared to cellulase alone (Qing and Wyman, 2011).
To find out the reason behind this substantial increase, enzymatic hydrolysate after hydrolysis was evaluated to determine the inhibitors like cellobiose and oligomers concentrations. Results suggest that 2.3% of cellobiose and oligomers were present after hydrolysis with Celluclast alone while, their concentration was reduced with EnzMix (Figure 4). It has been reported earlier that presence of even low amounts of oligomeric sugars and cellobiose act as strong enzyme inhibitors (Rajan and Carrier, 2014; Resch et al., 2014; Zhai et al., 2016). Qing et al. (2010) reported that 0.2–1% of oligomers may cause 15–38% decrease in hydrolysis yields.
An interesting finding of this study was the variation observed between optimized enzyme cocktail compositions based on different pretreatment methods on the same feedstock. In the previous study, the optimized cocktail developed for dilute acid pretreated wheat straw was composed of Celluclast (10 mg), beta-glucosidase (25 mg), xylanase (4 mg), pectinase (3.25 mg), and laccase (8 mg) (Agrawal et al., 2015b). However, the steam exploded wheat straw cocktail is composed of Celluclast (10 mg), β-glucosidase (18.83 mg), and xylanase (20.17 mg). It showed that a slightly higher amount of enzyme dosage is required for dilute acid pretreatment (50.25 mg) in comparison of steam explosion (49 mg). Another, important observation is that the pectinase and laccase have improved the hydrolysis yields with dilute acid pretreatment but these enzymes had negative impact during enzymatic hydrolysis of steam pretreated wheat straw. This might be due to the efficient disruption of wheat straw cell wall structure and lignin-carbohydrate linkages via steam explosion pretreatment at high temperature and pressure conditions while, the dilute acid pretreatment is mainly dependent upon removal of hemicellulose at relatively low temperature and pressure conditions.
Synergism Between Celluclast and Accessory Enzymes
To establish the synergistic action between Celluclast and other accessory enzymes, actual enzyme activity in the reaction mixture of Celluclast with accessory enzymes was determined and compared with the theoretical summation of enzyme activities (FPU, CMCase, BGL, and xylanase) present in the mixture. If actual enzyme activities in the reaction mixture are greater than the summation of theoretical activities, it is known as synergistic effect (Hu et al., 2013) otherwise it is an additive effect. As depicted in Table 2, actual enzyme activities were found to be more than the addition of individual enzymes. This proves that Celluclast and accessory enzymes work in a synergistic manner. Highest synergism was found in EnzMix where, BGL activity has been enhanced by 15.5-folds followed by CMCase and FPU by 6.4 & 6-fold and xylanase by 4-fold, respectively. A similar report by (Adsul et al., 2014) has shown that FPU and CMCase activities were enhanced by 1.3 and 2 times.
Benchmarking With Commercial Enzyme Preparations
The hydrolysis of steam pretreated wheat straw by EnzMix containing Celluclast, β-glucosidase, and xylanase in a protein ratio of 20.40: 38.43: 41.16, respectively and commercial enzyme samples was compared on a similar substrate and protein concentration (i.e., 49 mg protein/g biomass) as in EnzMix (Figure 5). Highest hydrolysis yields (>95%) were obtained with Cellic and EnzMix. This study showed that 82% of hydrolysis yield was obtained with 125 mg protein/g biomass of Celluclast however; only 49 mg/g protein of EnzMix was needed to obtain 99% yields. Thus, only 16% of the total enzyme protein cost was needed to achieve high hydrolysis yields with both EnzMix and Cellic but the protein costs were substantially enhanced to 42% with sub-optimized enzyme such as Celluclast and other inferior enzymes. This comparison was based on an assumption that the commercial cost of the cellulase enzyme protein remained same while their efficiency might vary depending upon the type of its components.
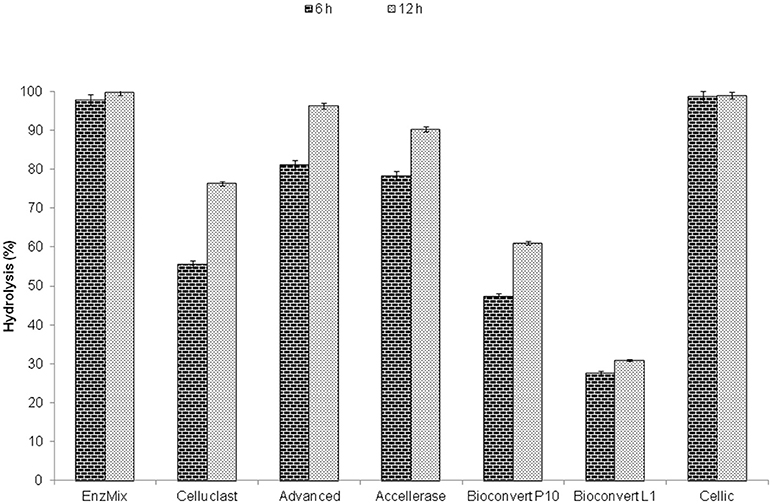
Figure 5. Benchmarking of commercial enzymes with EnzMix using steam pretreated wheat straw and same conditions.
Conclusions
This study established that accessory enzymes play a synergistic and critical role in enzymatic hydrolysis of ligno-cellulosic biomass. Development of enzyme cocktail based on substrate properties and pretreatment is a sustainable approach in reducing the enzyme concentration required to obtain high hydrolysis yields. In-house developed (EnzMix) was found at par or better than its commercial counterparts.
Author Contributions
RA and AS performed the research, data analysis and drafted the manuscript. SS and RK planned and conducted the pretreatment experiments. AS, RG, and DT reviewed the data and participated in the manuscript revisions. All authors read and approved the final manuscript.
Conflict of Interest Statement
RA, SS, RK, AM, RG, DT, and AS worked in the DBT-IOC Centre for Advanced Bioenergy Research, Indian Oil Corporation Ltd., Haryana, India.
Acknowledgments
The infrastructure and analytical support by IOCL R&D is duly acknowledged along with financial assistance by DBT, India. Enzyme manufacturers are greatly acknowledged for providing samples.
Supplementary Material
The Supplementary Material for this article can be found online at: https://www.frontiersin.org/articles/10.3389/fenrg.2018.00122/full#supplementary-material
Abbreviations
EnzMix, enzyme mixture; DS, degree of synergism; WS, wheat straw; SE, steam explosion; SEWS, steam pretreated wheat straw; SS, stainless steel; BGL, β-glucosidase activity; FPU, filter paper unit; CMCase, endo-glucanase, CCD, central composite design.
References
Adsul, M., Sharma, B., Singhania, R. R., Saini, J. K., Sharma, A., Mathur, A., et al. (2014). Blending of cellulolytic enzyme preparations from different fungal sources for improved cellulose hydrolysis by increasing synergism. RSC Adv. 4, 44726–44732. doi: 10.1039/c4ra08129c
Agrawal, R., Satlewal, A., Chaudhary, M., Verma, A., Singh, R., Verma, A. K., et al. (2012). Rapid detection of cadmium-resistant plant growth promotory rhizobacteria: a perspective of ELISA and QCM-based immunosensor. J. Microbiol. Biotechnol. 22, 849–855. doi: 10.4014/jmb.1108.08055
Agrawal, R., Satlewal, A., Gaur, R., Anshu, M., Kumar, R., Gupta, R. P., et al. (2015b). Improved saccharification of pilot-scale acid pretreated wheat straw by exploiting the synergistic behavior of lignocellulose degrading enzymes. RSC Adv. 5, 71462–71471. doi: 10.1039/c5ra13360b
Agrawal, R., Satlewal, A., Gaur, R., Mathur, A., Kumar, R., Gupta, R. P., et al. (2015c). Pilot scale pretreatment of wheat straw and comparative evaluation of commercial enzyme preparations for biomass saccharification and fermentation. Biochem. Eng. J. 102, 54–61. doi: 10.1016/j.bej.2015.02.018
Agrawal, R., Satlewal, A., Kapoor, M., Mondal, S., and Basu, B. (2017b). Investigating the enzyme-lignin binding with surfactants for improved saccharification of pilot scale pretreated wheat straw. Bioresour. Technol. 224, 411–418. doi: 10.1016/j.biortech.2016.11.026
Agrawal, R., Satlewal, A., Mathur, A. S., Gupta, R. P., Raj, T., Kumar, R., et al. (2014). Kinetic and enzyme recycling studies of immobilized β-glucosidase for lignocellulosic biomass hydrolysis. Environ. Eng. Manag. J. 17, 1–6.
Agrawal, R., Satlewal, A., Sharma, B., Mathur, A., Gupta, R., Tuli, D., et al. (2017c). Induction of cellulases by disaccharides or their derivatives in Penicillium janthinellum EMS-UV-8 mutant. Biofuels 8, 615–622. doi: 10.1080/17597269.2016.1242692
Agrawal, R., Satlewal, A., and Varma, A. (2015d). “Characterization of plant growth-promoting rhizobacteria (PGPR): a perspective of conventional versus recent techniques,” in Heavy Metal Contamination of Soils: Monitoring and Remediation, eds S. Irena and V. Ajit (Basel: Springer International Publishing), 471–485.
Agrawal, R., Satlewal, A., and Verma, A. (2013b). Development of a β-glucosidase hyperproducing mutant by combined chemical and UV mutagenesis. 3 Biotech 3, 381–388. doi: 10.1007/s13205-012-0095-z
Agrawal, R., Satlewal, A., and Verma, A. K. (2013a). Production of an extracellular cellobiase in solid state fermentation. J. Microbiol. Biotechnol. Food Sci. 2, 2339–2350.
Agrawal, R., Satlewal, A., and Verma, A. K. (2017a). Utilization of citrus sinensis waste for the production of β-glucosidase by solid-state fermentation using a Bacillus subtilis mutant. Environ. Eng. Manag. J. 16, 1465–1471.
Álvarez, C., González, A., Negro, M. J., Ballesteros, I., Oliva, J. M., and Sáez, F. (2017). Optimized use of hemicellulose within a biorefinery for processing high value-added xylooligosaccharides. Ind. Crops Prod. 99(Supplement C), 41–48. doi: 10.1016/j.indcrop.2017.01.034
Berlin, A., Maximenko, V., Gilkes, N., and Saddler, J. (2007). Optimization of enzyme complexes for lignocellulose hydrolysis. Biotechnol. Bioeng. 97, 287–296. doi: 10.1002/bit.21238
Boyce, A., and Walsh, G. (2018). Purification and characterisation of a thermostable β-Xylosidase from aspergillus niger van tieghem of potential application in lignocellulosic bioethanol production. Appl. Biochem. Biotechnol. 186, 712–730. doi: 10.1007/s12010-018-2761-z
Bunterngsook, B., Laothanachareon, T., Natrchalayuth, S., Lertphanich, S., Fujii, T., Inoue, H., et al. (2017). Optimization of a minimal synergistic enzyme system for hydrolysis of raw cassava pulp. RSC Adv. 7, 48444–48453. doi: 10.1039/c7ra08472b
Chundawat, S. P. S., Uppugundla, N., Gao, D., Curran, P. G., Balan, V., and Dale, B. E. (2017). Shotgun approach to increasing enzymatic saccharification yields of ammonia fiber expansion pretreated cellulosic biomass. Front. Energy Res. 5:9. doi: 10.3389/fenrg.2017.00009
de Oliveira Rodrigues, P., de Cássia Pereira, J., dos Santos, D. Q., Alves Gurgel, L. V., Pasquini, D., and Baffi, M. A. (2017). Synergistic action of an Aspergillus (hemi-)cellulolytic consortium on sugarcane bagasse saccharification. Ind. Crops Prod. 109(Supplement C), 173–181. doi: 10.1016/j.indcrop.2017.08.031
Frankó, B., Carlqvist, K., Galbe, M., Lidén, G., and Wallberg, O. (2018). Removal of water-soluble extractives improves the enzymatic digestibility of steam-pretreated softwood barks. Appl. Biochem. Biotechnol. 184, 599–615. doi: 10.1007/s12010-017-2577-2
Ghose, T. K., and Bisaria, V. S. (1979). Studies on the mechanism of enzymatic-hydrolysis of cellulosic substances. Biotechnol. Bioeng. 21, 131–146.
Gonçalves, G. A. L., Takasugi, Y., Jia, L., Mori, Y., Noda, S., Tanaka, T., et al. (2015). Synergistic effect and application of xylanases as accessory enzymes to enhance the hydrolysis of pretreated bagasse. Enzyme Microb. Technol. 72, 16–24. doi: 10.1016/j.enzmictec.2015.01.007
Hu, J., Arantes, V., Pribowo, A., and Saddler, J. N. (2013). The synergistic action of accessory enzymes enhances the hydrolytic potential of a cellulase mixture but is highly substrate specific. Biotechnol. Biofuels 6:112. doi: 10.1186/1754-6834-6-112
Hu, J., Arantes, V., and Saddler, J. N. (2011). The enhancement of enzymatic hydrolysis of lignocellulosic substrates by the addition of accessory enzymes such as xylanase: is it an additive or synergistic effect? Biotechnol. Biofuels 4, 36–49. doi: 10.1186/1754-6834-4-36
Huang, C., Zhao, C., Li, H., Xiong, L., Chen, X., Luo, M., et al. (2018). Comparison of different pretreatments on the synergistic effect of cellulase and xylanase during the enzymatic hydrolysis of sugarcane bagasse. RSC Adv. 8, 30725–30731. doi: 10.1039/c8ra05047c
Ibrahim, D., Weloosamy, H., and Sheh-Hong, L. (2014). Potential use of nylon scouring pad cubes attachment method for pectinase production by Aspergillus niger HFD5A-1. Process Biochem. 49, 660–667. doi: 10.1016/j.procbio.2014.01.012
Jacquet, N., Maniet, G., Vanderghem, C., Delvigne, F., and Richel, A. (2015). Application of steam explosion as pretreatment on lignocellulosic material: a review. Ind. Eng. Chem. Res. 54, 2593–2598. doi: 10.1021/ie503151g
Janzon, R., Schutt, F., Oldenburg, S., Fischer, E., Korner, I., and Saake, B. (2014). Steam pretreatment of spruce forest residues: optimal conditions for biogas production and enzymatic hydrolysis. Carbohydr. Polym. 100, 202–210. doi: 10.1016/j.carbpol.2013.04.093
Kane, S. D., and French, C. E. (2018). Characterisation of novel biomass degradation enzymes from the genome of Cellulomonas fimi. Enzyme Microb. Technol. 113, 9–17. doi: 10.1016/j.enzmictec.2018.02.004
Kristensen, J. B., Thygesen, L. G., Felby, C., Jorgensen, H., and Elder, T. (2008). Cell-wall structural changes in wheat straw pretreated for bioethanol production. Biotechnol. Biofuels 1, 1–5. doi: 10.1186/1754-6834-1-5
Kumar, D., and Murthy, G. S. (2011). Impact of pretreatment and downstream processing technologies on economics and energy in cellulosic ethanol production. Biotechnol. Biofuels 4:27. doi: 10.1186/1754-6834-4-27
Lee, H., Lee, Y. M., Heo, Y. M., Lee, J., Kim, J. S., Kang, K. Y., et al. (2017). Utilization of agricultural residues for enhancement of cellulolytic enzyme production and enzymatic saccharification by Trichoderma harzianum KUC1716. Ind. Crops Prod. 109(Supplement C), 185–191. doi: 10.1016/j.indcrop.2017.08.042
Li, J., Zhou, P., Liu, H., Xiong, C., Lin, J., Xiao, W., et al. (2014). Synergism of cellulase, xylanase, and pectinase on hydrolyzing sugarcane bagasse resulting from different pretreatment technologies. Bioresour. Technol. 155, 258–265. doi: 10.1016/j.biortech.2013.12.113
Liu, L.-Y., Qin, J.-C., Li, K., Mehmood, M. A., and Liu, C.-G. (2017). Impact of moisture content on instant catapult steam explosion pretreatment of sweet potato vine. Bioresour. Bioprocess. 4:49. doi: 10.1186/s40643-017-0179-z
Loman, A. A., and Ju, L.-K. (2016). Towards complete hydrolysis of soy flour carbohydrates by enzyme mixtures for protein enrichment: a modeling approach. Enzyme Microb. Technol. 86, 25–33. doi: 10.1016/j.enzmictec.2016.01.010
Miller, G. L. (1959). Use of dinitrosalicylic acid reagent for determination of reducing sugar. Anal. Chem. 31, 426–428.
Oliva-Taravilla, A., Moreno, A. D., Demuez, M., Ibarra, D., Tomas-Pejo, E., Gonzalez-Fernandez, C., et al. (2014). Unraveling the effects of laccase treatment on enzymatic hydrolysis of steam-exploded wheat straw. Bioresour. Technol. 175, 209–215. doi: 10.1016/j.biortech.2014.10.086
Parnthong, J., Kungsanant, S., and Chavadej, S. (2018). The influence of nonionic surfactant adsorption on enzymatic hydrolysis of oil palm fruit bunch. Appl. Biochem. Biotechnol. 186, 895–908. doi: 10.1007/s12010-018-2783-6
Pengilly, C., García-Aparicio, M. P., Diedericks, D., and Görgens, J. F. (2016). Optimization of enzymatic hydrolysis of steam pretreated triticale straw. BioEnergy Res. 9, 851–863. doi: 10.1007/s12155-016-9741-3
Pielhop, T., Amgarten, J., von Rohr, P. R., and Studer, M. H. (2016). Steam explosion pretreatment of softwood: the effect of the explosive decompression on enzymatic digestibility. Biotechnol. Biofuels. 9:152. doi: 10.1186/s13068-016-0567-1
Pierce, B. C., Agger, J. W., Wichmann, J., and Meyer, A. S. (2017). Oxidative cleavage and hydrolytic boosting of cellulose in soybean spent flakes by Trichoderma reesei Cel61A lytic polysaccharide monooxygenase. Enzyme Microb. Technol. 98, 58–66. doi: 10.1016/j.enzmictec.2016.12.007
Qing, Q., and Wyman, C. (2011). Supplementation with xylanase and beta-xylosidase to reduce xylo-oligomer and xylan inhibition of enzymatic hydrolysis of cellulose and pretreated corn stover. Biotechnol. Biofuels 4, 18–30. doi: 10.1186/1754-6834-4-18
Qing, Q., Yang, B., and Wyman, C. E. (2010). Xylooligomers are strong inhibitors of cellulose hydrolysis by enzymes. Bioresour. Technol. 101, 9624–9630. doi: 10.1016/j.biortech.2010.06.137
Rajan, K., and Carrier, D. J. (2014). Characterization of rice straw prehydrolyzates and their effect on the hydrolysis of model substrates using a commercial endo-cellulase, β-glucosidase and cellulase cocktail. ACS Sustain. Chem. Eng. 2, 2124–2130. doi: 10.1021/sc5002947
Resch, M. G., Donohoe, B. S., Ciesielski, P. N., Nill, J. E., Magnusson, L., Himmel, M. E., et al. (2014). Clean fractionation pretreatment reduces enzyme loadings for biomass saccharification and reveals the mechanism of free and cellulosomal enzyme synergy. ACS Sustain. Chem. Eng. 2, 1377–1387. doi: 10.1021/sc500210w
Ruiz, E., Cara, C., Manzanares, P., Ballesteros, M., and Castro, E. (2008). Evaluation of steam explosion pre-treatment for enzymatic hydrolysis of sunflower stalks. Enzyme Microb. Technol. 42, 160–166. doi: 10.1016/j.enzmictec.2007.09.002
Ruiz, H. A., Rodríguez-Jasso, R. M., Fernandes, B. D., Vicente, A. A., and Teixeira, J. A. (2013). Hydrothermal processing, as an alternative for upgrading agriculture residues and marine biomass according to the biorefinery concept: a review. Renew. Sustain. Energy Rev. 21, 35–51. doi: 10.1016/j.rser.2012.11.069
Ruiz, H. A., Thomsen, M. H., and Trajano, H. L. (2017). Hydrothermal Processing in Biorefineries: Production of Bioethanol and High Added-Value Compounds of Second and Third Generation Biomass. Berlin: Springer.
Sánchez-Cantú, M., Ortiz-Moreno, L., Ramos-Cassellis, M. E., Marín-Castro, M., and De la Cerna-Hernández, C. (2018). Solid-state treatment of castor cake employing the enzymatic cocktail produced from Pleurotus djamor fungi. Appl. Biochem. Biotechnol. 185, 434–449. doi: 10.1007/s12010-017-2656-4
Sanhueza, C., Carvajal, G., Soto-Aguilar, J., Lienqueo, M. E., and Salazar, O. (2018). The effect of a lytic polysaccharide monooxygenase and a xylanase from Gloeophyllum trabeum on the enzymatic hydrolysis of lignocellulosic residues using a commercial cellulase. Enzyme Microb. Technol. 113, 75–82. doi: 10.1016/j.enzmictec.2017.11.007
Santhi, V. S., Bhagat, A. K., Saranya, S., Govindarajan, G., and Jebakumar, S. R. D. (2014). Seaweed (Eucheuma cottonii) associated microorganisms, a versatile enzyme source for the lignocellulosic biomass processing. Int. Biodeter.Biodegr. 96, 144–151. doi: 10.1016/j.ibiod.2014.08.007
Satlewal, A., Agrawal, R., Bhagia, S., Das, P., and Ragauskas, A. J. (2018a). Rice straw as a feedstock for biofuels: availability, recalcitrance, and chemical properties. Biofuels Bioprod. Biorefin. 12, 83–107. doi: 10.1002/bbb.1818
Satlewal, A., Agrawal, R., Bhagia, S., Sangoro, J., and Ragauskas, A. J. (2018b). Natural deep eutectic solvents for lignocellulosic biomass pretreatment: recent developments, challenges and novel opportunities. Biotechnol. Adv. doi: 10.1016/j.biotechadv.2018.08.009. [Epub ahead of print].
Satlewal, A., Saini, J. K., Agrawal, R., Mathur, A., Tuli, D. K., and Adsul, M. (2017). “Indian biofuel progress, GHG emission and GHG savings by biofuels: comparative assessment with world,” in Sustainable Biofuels Development in India, eds K. Anuj Chandel and K. Rajeev Sukumaran (Cham: Springer International Publishing), 541–557.
Satlewal, A., Soni, R., Zaidi, M., Shouche, Y., and Goel, R. (2008). Comparative biodegradation of HDPE and LDPE using an indigenously developed microbial consortium. J. Microbiol. Biotechnol. 18, 477–482.
Sharma, B., Agrawal, R., Singhania, R. R., Satlewal, A., Mathur, A., Tuli, D., et al. (2015a). Untreated wheat straw: potential source for diverse cellulolytic enzyme secretion by Penicillium janthinellum EMS-UV-8 mutant. Bioresour. Technol. 196, 518–524. doi: 10.1016/j.biortech.2015.08.012
Sharma, N. K., Behera, S., Arora, R., and Kumar, S. (2017). Evolutionary adaptation of Kluyveromyces marxianus NIRE-K3 for enhanced xylose utilization. Front. Energy Res. 5:32. doi: 10.3389/fenrg.2017.00032
Sharma, S., Kumar, R., Gaur, R., Agrawal, R., Gupta, R. P., Tuli, D. K., et al. (2015b). Pilot scale study on steam explosion and mass balance for higher sugar recovery from rice straw. Bioresour. Technology. 175, 350–357. doi: 10.1016/j.biortech.2014.10.112
Sluiter, A., Hames, B., Ruiz, R., Scarlata, C., Sluiter, J., and Templeton, D. (2006). Determination of Sugars, Byproducts, and Degradation Products in Liquid Fraction Process Samples. Laboratory Anlytical Procedure from the National Renewlable Energy Laboratory Biomass Analysis Technology (NREL BAT) Team.
Sluiter, A., Hames, B., Ruiz, R., Scarlata, C., Sluiter, J., Templeton, D., et al. (2008). Determination of Structural Carbohydrates and Lignin in Biomass. Laboratory Analytical Procedure from the National Renewable Energy Laboratory Biomass Analysis Technology (NREL BAT) Team.
Soleimani, S., and Ranaei-Siadat, S.-O. (2017). Preparation and optimization of cellulase cocktail to improve the bioethanol process. Biofuels 8, 291–296. doi: 10.1080/17597269.2016.1224293
Sun, S.-N., Cao, X.-F., Li, H.-Y., Xu, F., and Sun, R.-C. (2014). Structural characterization of residual hemicelluloses from hydrothermal pretreated Eucalyptus fiber. Int. J. Biol. Macromol. 69(Supplement C), 158–164. doi: 10.1016/j.ijbiomac.2014.05.037
Takano, M., and Hoshino, K. (2018). Bioethanol production from rice straw by simultaneous saccharification and fermentation with statistical optimized cellulase cocktail and fermenting fungus. Bioresour. Bioprocess. 5:16. doi: 10.1186/s40643-018-0203-y
van der Zwan, T., Hu, J., and Saddler, J. N. (2017). Mechanistic insights into the liquefaction stage of enzyme-mediated biomass deconstruction. Biotechnol. Bioeng. 114, 2489–2496. doi: 10.1002/bit.26381
Walker, D. J., Gallagher, J., Winters, A., Somani, A., Ravella, S. R., and Bryant, D. N. (2018). Process optimization of steam explosion parameters on multiple lignocellulosic biomass using taguchi method—a critical appraisal. Front. Energy Res. 6:46. doi: 10.3389/fenrg.2018.00046
Walker, J. M. (1994). The bicinchoninic acid (BCA) assay for protein quantitation. Methods Mol. Biol. 32, 5–8. doi: 10.1385/0-89603-268-X:5
Wang, M., and Lu, X. (2016). Exploring the synergy between cellobiose dehydrogenase from phanerochaete chrysosporium and cellulase from Trichoderma reesei. Front. Microbiol. 7:620. doi: 10.3389/fmicb.2016.00620
Wu, Y., Sun, X., Xue, X., Luo, H., Yao, B., Xie, X., et al. (2017). Overexpressing key component genes of the secretion pathway for enhanced secretion of an Aspergillus niger glucose oxidase in Trichoderma reesei. Enzyme Microb. Technol. 106, 83–87. doi: 10.1016/j.enzmictec.2017.07.007
Yang, M., Zhang, K.-D., Zhang, P.-Y., Zhou, X., Ma, X.-Q., et al. (2016). Synergistic cellulose hydrolysis dominated by a multi-modular processive endoglucanase from clostridium cellulosi. Front. Microbiol. 7:932. doi: 10.3389/fmicb.2016.00932
Zhai, R., Hu, J., and Saddler, J. N. (2016). What are the major components in steam pretreated lignocellulosic biomass that inhibit the efficacy of cellulase enzyme mixtures? ACS Sustain. Chem. Eng. 4, 3429–3436. doi: 10.1021/acssuschemeng.6b00481
Zhang, Z., Wang, M., Gao, R., Yu, X., and Chen, G. (2017). Synergistic effect of thermostable β-glucosidase TN0602 and cellulase on cellulose hydrolysis. 3 Biotech 7:54. doi: 10.1007/s13205-017-0672-2
Keywords: steam explosion, hydrolysis, cellulase, wheat straw, inhibition
Citation: Agrawal R, Semwal S, Kumar R, Mathur A, Gupta RP, Tuli DK and Satlewal A (2018) Synergistic Enzyme Cocktail to Enhance Hydrolysis of Steam Exploded Wheat Straw at Pilot Scale. Front. Energy Res. 6:122. doi: 10.3389/fenrg.2018.00122
Received: 24 July 2018; Accepted: 29 October 2018;
Published: 19 November 2018.
Edited by:
Sachin Kumar, Sardar Swaran Singh National Institute of Renewable Energy, IndiaReviewed by:
Héctor A. Ruiz, Universidad Autónoma de Coahuila, MexicoKalavathy Rajan, University of Tennessee Institute of Agriculture, United States
Copyright © 2018 Agrawal, Semwal, Kumar, Mathur, Gupta, Tuli and Satlewal. This is an open-access article distributed under the terms of the Creative Commons Attribution License (CC BY). The use, distribution or reproduction in other forums is permitted, provided the original author(s) and the copyright owner(s) are credited and that the original publication in this journal is cited, in accordance with accepted academic practice. No use, distribution or reproduction is permitted which does not comply with these terms.
*Correspondence: Ruchi Agrawal, ZHIucnVjaGlhZ3Jhd2FsMDEwQGdtYWlsLmNvbQ==