- 1Department of Forest and Natural Resources Management, SUNY College of Environmental Science and Forestry, Syracuse, NY, United States
- 2Vicerrectoría de Investigación y Postgrado - Centro del Secano, Universidad Católica del Maule, Talca, Chile
- 3Department of Paper and Bioprocess Engineering, SUNY College of Environmental Science and Forestry, Syracuse, NY, United States
Shrub willows are being developed as a short rotation woody crop (SRWC) that can grow on marginal agricultural land. Willow has a high net energy ratio (energy produced/ fossil fuel energy consumed), low greenhouse gas footprint and high carbohydrate production potential. Willow biomass can be combined with forest biomass, but willow often has a higher proportion of bark that creates challenges because it increases the ash content and decreases the melting point. Hot water extraction is a pretreatment that has been shown to improve the quality of chipped material while producing a marketable stream of byproducts. This study evaluated how the amount of bark (0, 33, 66, and 100%) on three willow cultivars and sugar maple impact the output of hot water extraction in terms of mass removal and extract composition, as well as its influence on the heating value, ash and elemental content. The hot water extraction process resulted in ash content up to 50% for sugar maple and willow, but there was variation among the willow varieties. The heating value after hot water extraction was about 5% higher because of the removal of mostly hemicelluloses, which have relatively low heating value. HWE led to significant reductions of calcium, potassium, magnesium and sulfur contents. The hot water extraction provides a fermentable sugar stream and other coproducts after multiple separation and treatment steps, and improves the characteristics of willow and sugar maple biomass for combined heat and power. This paper demonstrates how biomass with higher bark content can generate a useable sugar stream while improving the quality of the biomass for combined heat and power by managing its ash content while simultaneously producing other valuable products.
Introduction
Biomass feedstock in the United States has the potential to displace and supplement a significant portion of the present petroleum consumption in the form of biofuels, bioenergy and bioproducts (USDOE, 2011). However, the variability in the quality of these materials has limited their adoption. In order for them to increase market share, effective and efficient pretreatment methods for lignocellulosic biomass need to be developed (Chaturvedi and Verma, 2013). The specific role of pretreatment steps varies among feedstocks and conversion pathways. Broadly, pretreatments are seen as a means to reduce variability in feedstock characteristics in order to deliver a uniform feedstock to end users. Pretreatments can also improve the efficiency of conversion by altering the physical and chemical attributes of woody biomass (Liu, 2015). Activities during pretreatment range from changing the particle size to the modification of the structural component of the biomass feedstock.
Hot Water Extraction (HWE) has been studied and proposed as a pretreatment step into a multi-product biorefinery. This process consists of cooking biomass materials, such as wood chips, at high temperature and pressure in water (Amidon and Liu, 2009). The treated lignocellulosic biomass, or extracted wood chip, can be valued as an improved-quality raw material for fuels, manufacture of wood products or wood derivatives (Amidon et al., 2011). Multiple separation steps and treatments of the extract solution yield wood-based chemicals and materials such as acetic acid, methanol, lignin and fermentable sugars. The effect of HWE on various biomass feedstocks has been extensively studied during the last decade (Amidon and Liu, 2009; Amidon et al., 2011; Duarte et al., 2011; Pu et al., 2011; Lu et al., 2012; Wang et al., 2012; Ma et al., 2013; Mante et al., 2014; Corbett et al., 2015). By removing some biomass components, HWE alters the chemical components of biomass and the surface morphology of biomass. A recent study (Ma et al., 2013) on HWE of bamboo has shown that oxygen/carbon ratio decreases respectively from 0.43 to 0.34 on the exterior surface and to 0.37 on the interior surface. Hemicellulose, which has a higher oxygen/carbon ratio than cellulose and lignin, is dissolved into the water during HWE process. As a pretreatment, HWE increases the porosity of the cell wall (Duarte et al., 2011), which enhances sugar recovery and enzymatic hydrolysis (Wang et al., 2012).
Shrub willow (Salix sp.) is a potential feedstock for the bioenergy and bioproducts industry. It has a high net energy ratio, high yield, rapid growth rate, the ability to resprout after multiple harvests, and is compositionally similar to other hardwood species (USDOE, 2011; Volk et al., 2014). Willow is generally harvested every 3–4 years for up to 7 rotations without replanting, and it is suitable to grow on marginal land across the Northeast, Midwest and parts of the Southeastern United States; it has been grown for decades in Europe. The net energy ratio, which is the ratio of the total energy content of biomass at the gate of the farm by the total fossil fuels consumed, was estimated to be 55:1 for a typical agricultural production of willow biomass (Heller et al., 2003). The first rotation yields of top willow cultivars range from 9.4 to 13.6 odt/ha.yr across multiple sites (Volk et al., 2011). Sleight et al. (2016) demonstrates that plots with low yields during the first rotation had a significant yield increase during the second rotation. Eisenbies (Eisenbies et al., 2015) reports the characteristics of willow biomass chips harvested at two sites are similar to other woody biomass; the ash content ranges from 0.8 to 3.5%; the moisture content ranges from 37 to 51% and the higher heating value ranges from 18.3 to 19.1 MJ/kg. The stem diameters of three willow cultivars, which were 4 years old on a 5 year root system, were less than 45 mm (Eisenbies et al., 2015). Bark content of willow crop varies with stem diameters and site conditions. For instance, as diameter of willow stems increases bark content decreases. Mean whole stem bark percentage of willow in two sites was 12.1% and 18.9% (Eich et al., 2015).
Willow is typically harvested using single-pass cut-and-chip forage harvesters that have been developed to harvest short-rotation crop. The machine can cut willow stems of up to 120 mm in diameter and produce 10–45 mm long chips (Eisenbies et al., 2014). Due to the small diameter of willow crop at harvest, the prospect of debarking this feedstock is challenging, even infeasible with current technology, and would increase the overall processing time and cost of this feedstock (Magagnotti et al., 2011). Thus, bioenergy and bioproducts industries will have access to willow as bark-on feedstocks. To date most of research on HWE of wood chips used debarked wood (Duarte et al., 2011; Lu et al., 2012; Mante et al., 2014). Forest residues with bark, often called dirty chips, are considerably cheaper than debarked chips and if the HWE process can be used to improve their quality and/or extend their storage, these materials could be effectively utilized at an acceptable cost. A further option with feedstocks would be to blend different sources of material to ultimately provide a year round supply with consistent quality and minimal cost variation (Williams et al., 2016). Examining the performance of HWE on bark-on woodchips, as well as woodchips with varying amounts of bark removed, is an important step in understanding how this feedstock and pretreatment could be incorporated into the feedstock supply chain.
The objective of this study was to evaluate the effect of hot water extraction on the current bark-on willow biomass in term of mass removal and composition of the extract, as well as its influence on the heating value and ash content of three willow cultivars using sugar maple as an operational control. A second objective was to compare the degree to which bark removal would impact changes in biomass quality (ash content, heating value, elemental content) when HWE is applied.
Materials and Experimental Methods
Source of Material
Shrub willow used for this study was hand harvested as whole stems in SUNY ESF's genetic field station in Tully, NY. Sugar maple (Acer saccharum Marshall) stems were obtained from SUNY ESF's field station in Lafayette, NY. Three cultivars of willow (SX64 - Salix sachalinensis F. Schmidt, SV1 - Salix x dasyclados Wimm. and Sherburne - Salix miyabeana Seemen) were used in addition with sugar maple. Both shrub willow and sugar maple are hardwood species.
Bark was removed at four levels (0, 33, 66, and 100%) to simulate the effect of bark removal. Four replicates were prepared for each experimental level. The bark of willow stems and sugar maple stems were manually peeled and the ratio of bark to wood on each of the stems was determined. The stem and the bark were chipped separately and remixed using 0, 33, 66, and 100% of the predetermined proportions. The 0% sugar maple was used as the control because this is the standard used in previous HWE studies (Duarte et al., 2011; Lu et al., 2012; Mante et al., 2014). The sample with 0% bark refers to wood with no bark and sample with 100% bark refers to wood with all its bark attached. All samples were screened in a Williams's classifier conforming to mesh size of 3/8 to 7/8 inches prior to HWE. This was done to remove fine particles that would create blockages in the vessels used for HWE. Heating value, ash content, alkali metals, silica and sulfur content were determined on wood samples prior to HWE and after HWE.
Hot Water Extraction and Analysis
Hot water extraction was performed in a 4.7 L M/K digester using 500 g oven dried chips and 4:1 water to wood ratio at 160°C for 120 min. At the completion of the extraction, the liquor was collected and the extracted chips were washed twice. For the washing process, the equivalent volume of the liquor was added to the digester and cooked at 80°C for 15 min which fully removes dissolved substances from the mass of chips (Wang et al., 2017). The mass removal was reported as the ratio of the mass removed by HWE by the initial weight of oven dried wood chips. The hydrolyzate was subjected to chemical composition analysis as described in Kiemle et al. (2003) AND Mittal et al. (2009a). A sample of the hydrolyzate, for the maple and SV1 samples, was mixed with 96% sulfuric acid to obtain a 4wt.% sulfuric acid solution. The solution was autoclaved for 45 min at 121°C. Then, 0.1 ml of 72% deuterated sulfuric acid was added to 1 g of filtrated sample. After the hydrolysis, sugars (xylose, glucose, arabinose, rhamnose and galactose) as well as acetic acid and furfural concentrations in the sample were determined by 1H NMR (Mittal et al., 2009a). The higher heating value was determined on samples prior and following HWE in accordance with ASTM method D5865-13: Standard test method for gross calorific value of coal and coke by using a Parr 6200 Oxygen bomb calorimeter (ASTM, 2013). The ash content was determined by combustion in a muffle furnace in accordance with TAPPI method T 211 om-02: Ash in wood, pulp, paper and paperboard: combustion at 525°C (TAPPI1) on samples prior to HWE and following HWE. An acid (HCl) solution was prepared with the ash as described by Wild et al. (1979). Potassium, sodium, magnesium, calcium, silicon and sulfur were measured by Inductively Coupled Plasma Atomic Emission Spectroscopy technique (Schulte and Huang, 1985) on samples prior to HWE and following HWE.
A repeated analysis was performed using Statistical Analysis Software (SAS version 9.4) using the mixed procedure with an alpha level of 0.05.There were four varieties of biomass (three willow cultivars and one sugar maple), four bark levels (0, 33, 66, and 100%) and two periods (prior to HWE, following HWE). (Model Parameter = Variety Bark Period Variety*Bark Variety*Period Bark*Period Variety*Bark*Period).
Results and Discussions
Mass Removed by Hot Water Extraction (HWE) and Composition of the Hydrolyzate
The majority of HWE research to date has been conducted on woody biomass that has been debarked. In this study, the mass removal of 0% bark samples was not significantly different among the sugar maple and all three willow cultivars, and ranged from 20.0 to 21.7%. These mass removals were similar to other studies on HWE of hardwood species; HWE of debarked maple chips, under the same conditions described in this study, resulted in 21.1% mass removal (Duarte et al., 2011). Similarly, HWE of debarked aspen woodchips at 160°C for 210 min with a water to wood ratio of 4:1 produced a mass removal of 21.4% (Lu et al., 2012). HWE conducted for only 1 h, under approximately the same conditions, yields lower mass removal than what is found in this present study. Guan et al. found mass removal of 15.7% when HWE is performed on debarked hybrid poplar for 1 h at 170°C (Guan et al., 2018). HWE of sugar maple removed 14.2% of the initial weight, when operated at 160°C for 1 h (Mittal et al., 2009a). Mass removal is impacted by many factors, including—but not limited to—temperature, water-to-wood ratio and duration of extraction (Lu et al., 2012).
As the bark content of sugar maple increased, mass removal increased (Table 1). However, the mass removal of each willow cultivar did not match sugar maple with increasing bark content. Variation of bark content did not change the mass removal of Sherbune (p > 0.19) as it did for SX64 and SV1. Bark-on SV1 differed significantly from both SX64 and sugar maple. Differences of mass removal were 3.22% between SX64 and SV1 (p < 0.0001), and 3.52% between sugar maple and SV1 (p < 0.0001). These results suggest the impact of bark content on the mass removed by HWE varies among willow cultivars and sugar maple. The reason for the varied responses among samples is not entirely clear but may be partially related to the initial ash content and ash removal (see section Ash Content, below), the bark to wood ratio, and the variability in relative amounts of hemicellulose, cellulose, lignin and extractives. The relative proportion of bark-to-wood decreases as the diameter of the willow stems increases (Eich et al., 2015). Bark contains higher ash and lignin (Mészáros et al., 2004), and higher extractives than wood. Chemical composition analysis of 25 willow cultivars shows that hemicellulose and cellulose are greater in debarked wood than whole stem biomass (100% bark attached) (Serapiglia et al., 2009).
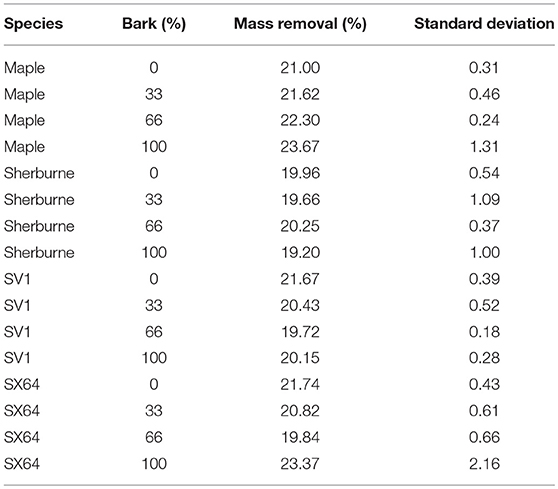
Table 1. Mass removal following hot water extraction of sugar maple and three willow cultivars with different bark content.
The mechanism of HWE for hardwood species has been described (Mittal et al., 2009a,b; Liu, 2010). HWE uses the hydrolytic property of water and in situ-generated organic acid to activate the depolymerization reaction. The depolymerization of carbohydrates, mainly hemicellulose, leads to the formation of oligomers and monomers of xylose, mannose, galactose and glucose, in addition to acetic acid, formic acid and other degradation products. Glucose may in fact come either from the decomposition of cellulose or hemicellulose. However, xylose is derived from hemicellulose depolymerization. Furfural is the result of the degradation of xylose at high temperature and pressure. Also, the degradation of hexoses leads to the formation of hydroxymethyl furfural (HMF), which could further converted into levulinic acid and formic acid (Palmqvist and Hahn-Hägerdal, 2000).
Glucose, xylose, mannose, arabinose, rhamnose, galactose—including both oligomers and monomers—in addition to acetate and furfural concentrations were determined in the hydrolyzate (Table 2). Only the concentration for sugar maple and SV1 were reported due to technical issues with the analysis of the hydrolyzate of SX64 and Sherburne. The total concentration, which is the sum of the concentration of these components, for sugar maple and SV1 was not significantly different (p > 0.47) at any of the bark percentages. Concurrently, the total concentration of sugars decreased steadily and at almost the same rate. The total concentration in the extract of sugar maple ranged from 45.29 g/L (0% bark) to 32.60 g/L (100% bark). Similarly, it ranged from 44.72 g/L (0% bark) to 28.97 g/L (100% bark) for SV1. As for other parameters reported in this study these responses may be different for other willow cultivars, particularly since previous studies have shown some degree of variation in cellulose, hemicellulose and lignin content across willow cultivars (Serapiglia et al., 2013).
Xylose was the predominant component in the hydrolyzate after HWE of sugar maple and willow woodchips. Total xylose concentration—in the form of monomer and oligomer—in the hydrolyzate after HWE ranged from 14.36 to 27.51 g/L for sugar maple and 15.94 to 23.93 g/L for SV1. Guan et al. (2018) found total xylose concentration of 16.8 g/L from HWE of hybrid poplar (hardwood), mostly present in the form of oligosaccharides, and 4.1 g/L from HWE of southern pine (softwood). It has been documented that glucomannan is the predominant unit of hemicellulose in softwood while Glucuronoxylan is the major one in hardwood (Liu, 2010). There was a steady decline in the xylose concentration in the extract as the amount of bark in the sample increases for both maple and SV1. There was no significant difference in the xylose concentration between maple and SV1 at any of the bark percentages (P > 0.16). The ratio of xylose to glucose was high across all the samples, ranging from 3.9 (SV1 0% bark) to 12.4 (Maple 0% bark). This confirms that hemicellulose is the main component that is removed during HWE of sugar maple and willow across the range of bark samples.
Acetic acid, furfural, and other carbohydrate and lignin degradation products can inhibit fermentation, but have value as byproducts. Bark content did not affect the formation of furfural during HWE of sugar maple and SV1 samples (p > 0.48). The mean of furfural concentration in the hydrolyzate after HWE for sugar maple and SV1 were respectively 1.09 and 0.96 g/L. Thomsen reported furfural concentration ranging from 0.03 to 1.2 g/L during hydrothermal treatment of wheat straw (Thomsen et al., 2009). The relative cellular redox activity of Candida shehatae, a xylose fermenting yeast strain, was reduced to 65% when furfural concentration was above 2 g/L and 55% when acetic acid concentration was 4 g/L (Zhao et al., 2005). Beside their inhibition capability on fermentation, furfural and acetic acid hold valuable promise for the development of a biorefinery that includes the process of HWE. Acetic acid has been used for centuries by society for food preservation and is used in the manufacture of a range of products. Furfural is the precursor of many valuable chemicals in the category of furyl, furfuryl, furoyl, furfuryldiene as well as dimethyl furan and ethyl levulinate (Yan et al., 2014). Furfuryl alcohol, a product of catalytic hydrogenation of furfural, is being used in the production of foundry resin and furan fiber reinforced plastics (Schneider and Phillips; Yan et al., 2014). Recently, a new fuel blend that contains furfuryl alcohol and ionic liquid has been developed to be used in missiles and satellite launch vehicles (Bhosale et al., 2016).
Ash Content
Before HWE, the ash content of sugar maple woodchips was significantly less than all three willow cultivars for all bark contents (P < 0.001). There were general differences among the willow cultivars at each of the bark percentages with Sherburne > SX64 > SV1. However, there were no statistical differences with three pairs of samples among all willow and bark percentage combinations; 33% bark for SV1 and Sherburne (p = 0.097), 66% bark for SV1 and Sherburne (p = 0.798), and 100% bark SV1 and SX64 (p = 0.22). At 0% bark level, SV1 had significantly lower ash content than Sherburne and SX64. Sherburne had significantly higher ash content than SV1 and SX64 at 100% bark level (Figure 1). The ash content values for Sherburne and SX64 with 100% bark were very similar to the values reported by Serapiglia et al. (2013), despite different sampling and analytical techniques being used. However, the ash values for SV1 in this study were about three times higher than reported values for samples from the Tully site, but the ash content in this study was about 40% lower than samples from another site in Belleville in the Serapiglia et al. (2013) study. Ash content has been shown to vary by site and willow cultivar but the values here are in the range of values reported for hand harvested plots.
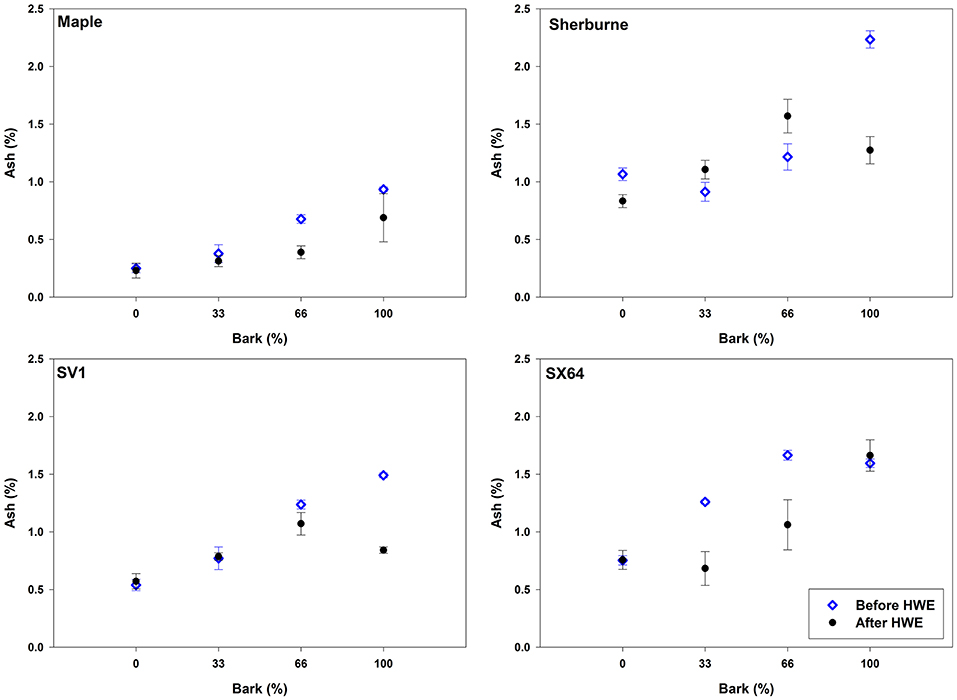
Figure 1. Ash content of sugar maple and three willow cultivars with bark levels ranging from 0 to 100% before and after HWE.
After HWE, ash contents of the three willow cultivars were more uniform at low bark level. There were no significant differences between the ash content of SV1, SX64 and Sherburne samples at 0% bark level (p > 0.11). But, at 100% bark level SX64 had significantly higher ash content than Sherburne, which itself had higher ash content than SV1. Sugar maple had significantly lower ash content than all three willow cultivars across the four levels of bark except for 100% SV1, which was not significantly different (p = 0.35).
Overall, the ash content of sugar maple and willow samples decreased after HWE (Figure 1). The mean ash content of sugar maple samples across all bark levels decreased from 0.56% prior to HWE to 0.40% after HWE. Similarly, SV1 and SX64 had lower ash content after HWE. But, it is important to notice that ash content of 0% bark sugar maple, SV1 and SX64 was not impacted by HWE (p > 0.77). These results suggest that inorganic elements that are transferred into the water during the process of HWE originated mostly from the bark structure. The reduction in ash levels varied among the three willow cultivars. SV1 had no or small reductions in ash content until the 100% bark sample where ash was reduced from 1.49 to 0.84%. Previous studies have shown that washing alone can reduce ash content in biomass (Jenkins et al., 1996; Deng et al., 2013). The reduction of ash generation has the advantage of allowing the removal of ash less frequently, decreasing the risk of slagging and reducing the emissions problem. It was suggested that removal efficiency of ash increases as the water temperature increases (Deng et al., 2013). In addition to the reduction of ash, which is an important step for thermochemical conversion of biomass, the HWE process provides a liquid stream that contains key components to be recovered as products.
HWE had a greater effect on bark-on wood compared to bark-off wood. Ash content for bark-off sugar maple was essentially unchanged 0.24 and 0.23% for 0% bark before and after HWE respectively; conversely, for 100% bark content ash content decreased from 0.93 to 0.69%. All three willow cultivars (SV1, SX64, and Sherburne) with 100% bark-on had significant ash reductions compared to their corresponding 0% bark samples. For instance, among all three willow cultivars and prior to HWE, ash content ranged from 0.54 to 1.06% for 0% bark samples, and from 1.27 to 2.23% for 100% bark samples. Following HWE, the reduced ash content ranged from 0.57 to 0.83% on debarked willow samples, and from 0.84 to 1.66% on willow samples with 100% bark attached. As with maple, the amount of ash removed by HWE was greater for samples with higher bark content. Based on these results, the ash content for most 0% bark samples would meet the 1.0% threshold set for class A1 graded wood chips; Sherburne would meet the A1.5 class (1.5% ash content threshold) (ISO, 2014). HWE resulted in grade increase for willow. Samples with 100% bark content at best qualify for a class B chip, with ash content less than 3.0%.
The willow used in this trial was hand harvested. Due to the logistics of mechanical harvesting, ash content at commercial scales could be slightly higher. Eisenbies et al. (2015) found that ash content of over 200 willow samples that were harvested with a commercial single pass cut and chip harvester range from 0.8 to 3.5%. Inorganic materials that contaminate the feedstock during harvesting and transportation operations could be easily washed out during the HWE. Beside the variability that can be caused by willow cultivar, bark content and harvesting method, ash content depend also on site condition (Stolarski et al., 2013).
Heating Value
Before HWE, there were significant differences between heating values of all three willow cultivars (p < 0.003) (Figure 2). Across all bark content (0–100%), the heating value of SV1 was about 2–3% lower than the heating value of SX64 and Sherburne. But, heating values of SV1 and sugar maple were not significantly different (p = 0.39). There were no significant differences in HHV for 0% bark (p = 0.91) and for 100% bark (p = 0.70) for SX64 and Sherburne. The difference observed among the heating values of different willow cultivars may be due to the difference in their structural composition. Serapiglia et al. (2009) studied the composition of 25 willow cultivars and found significant differences in their relative cellulose, hemicellulose and lignin contents. On a dry mass basis, SV1 had 1.6 percent point lower lignin content than SX64 (Serapiglia et al., 2009). Lignin has higher heating value than cellulose (White, 2007; Novaes et al., 2010), which itself has higher heating value than hemicellulose. Therefore, the lower heating value of SV1 aligns with the positively correlated relationship between higher heating value and lignin content in hardwood species (Demirbaş, 2001).
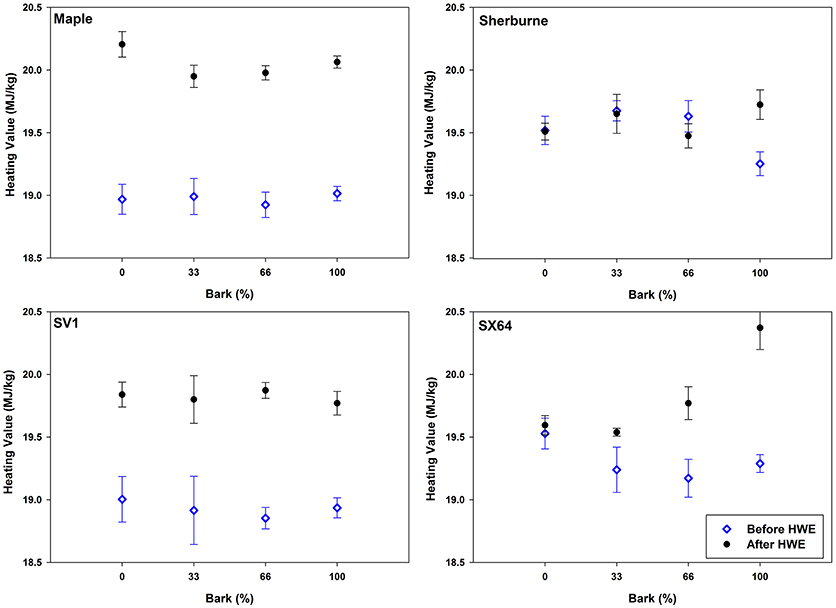
Figure 2. Higher heating value of sugar maple and three willow cultivars with bark levels ranging from 0 to 100% before and after HWE (errors bars represent standard deviations).
Both the amount of bark content and the specific cultivar had an impact on the higher heating value after HWE. For bark content ranging from 0 to 100% the heating values of SV1 and SX64 were not significantly different, but were significantly higher than the heating value of Sherburne. The heating value of debarked sugar maple and sugar maple with all its bark attached were significantly higher respectively than debarked SV1 (p = 0.004) and SV1 with all its bark attached (p < 0.0001).
Following HWE the heating value ranged from 19.5 to 20.4 MJ/kg across all species and different amount of bark, which is an increase compared to higher heating values prior to HWE. Heating values of sugar maple, SV1 and SX64 increase significantly after HWE across all bark levels except for 0% bark SX64, which had no significant change in HHV (p = 0.51). The means of the heating values of sugar maple across all bark treatments increases after HWE by 5.7%. Similarly, after HWE SV1 and SX64 have an increase of 4.7 and 2.7% of their heating value. The higher heating value of mixed hardwood species that were extracted using a similar process (160°C for 2 h) showed a 2.9% increase in HHV. The increase was slightly higher, 3.6%, at the conditions of 170°C for 2 h (Pu et al., 2011). During HWE, hemicellulose molecules in willow and sugar maple structures are being extracted and partially hydrolyzed. As it is shown in Table 1, 19.2–23.7% of the woodchips were transferred into the hot water. The analysis of the composition of the hydrolyzate shows that xylose, which is produced from the hydrolysis of hemicellulose, is the major component of the hydrolyzate. As a consequence, after HWE the wood had a lower proportion of hemicellulose and a higher proportion of lignin, which results in an increase of the heating value.
The effect of bark content on heating value before and after HWE varies between sugar maple and willow cultivars. The heating values for sugar maple and SV1 before HWE are similar across all the bark treatments. Following HWE the heating values for sugar maple and SV1 are higher for all bark treatments but the heating values do not vary across the bark treatments for either sugar maple or SV1. In contrast, the heating value of SX64 decreases with increasing bark content before HWE and increases with bark content after HWE. Studies of the effect of bark ratio on heating value of pellets made from five evergreen Mediterranean hardwoods species found that the heating value of bark was statistically lower in three species and was higher in one than the heating value of their debarked wood (Barmpoutis et al., 2015).
The increase of the heating value of woody biomass, including short rotation willow, can add value to the biomass feedstock for biopower and heating applications. The moisture content of the biomass will impact the lower heating value of the chips being used. Practically speaking, net (lower) heating value describes better the amount of energy available to be used. When the moisture content of a fuel increases, the lower heating value decreases. In general, the moisture content of chips immediately following HWE is higher than the moisture of the non-extracted chips. However, further investigation is needed to understand the drying process of extracted woodchips. HWE woodchips contain less hemicellulose and have higher porosity (Duarte et al., 2011), which suggests a propensity for more rapid drying and a lower capacity to retain water.
Potassium Content
Prior to HWE, the potassium content in all three willow cultivars increased significantly with increasing bark content (p < 0.0001) (Figure 3). Potassium content at 100% bark level was 1.6, 1.5, and 1.3 times higher than the potassium content at 0% bark level for SV1, SX64 and Sherburne respectively. The potassium content was significantly different among all four biomass categories across all bark levels except for at the 33% bark treatment for Sherburne and SX64 (p = 0.31), and 66% bark treatment for Sherburne and SX64 (p = 0.87). These results mirror the ash content data, SV1 with 0% bark had lower potassium content (0.68 ± 0.03 mg/g) than SX64 (0.91 ± 0.02 mg/g), which had lower potassium content than Sherburne (1.14 ± 0.04 mg/g). Observed values of potassium content for SV1, SX64 and Sherburne with all their bark attached are within the range of values reported in a previous study (Tharakan et al., 2003).
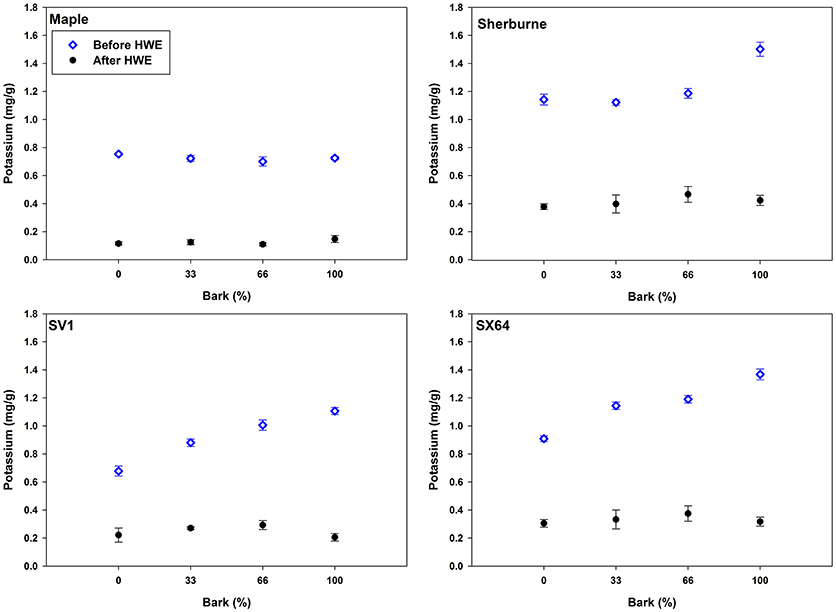
Figure 3. Potassium content of sugar maple and three willow cultivars with bark levels ranging from 0 to 100% before and after HWE (errors bars represent standard deviations).
After HWE, potassium content decreased to a uniform level across the percent bark treatments independently of their content before extraction (Figure 3). Among all bark content, following the extraction, the potassium content of SV1 varied from 0.20 to 0.29 mg/g while it varied from 0.30 to 0.37 mg/g in SX64 and 0.38 to 0.47 mg/g in Sherburne. HWE removed 70% of potassium in willow cultivars and 83% of potassium in sugar maple. The considerable reduction in potassium content by HWE is the result of potassium being in true solution and ionizable form in many plant tissues (Morris and Sayre, 1935). Potassium content along with the concentrations of sodium, magnesium, calcium and silicon determine the melting behavior of ash; therefore, potassium removal is a highly desirable benefit that reduces the risk of slagging and hard deposit that accumulates in furnaces and boilers (Obernberger and Thek, 2004). Although potassium contents were uniform within a given cultivar after HWE, there were significant differences in potassium content among willow cultivars and sugar maple across all bark levels (p < 0.03).
Magnesium Content
Prior to HWE the magnesium contents among all three willow cultivars and sugar maple samples across all bark content were within the range of 0.20–0.28 mg/g (Figure 4). Magnesium content was not significantly different with increasing bark content for maple but changed for each of the three willow cultivars. For Sherburne, the magnesium concentration was the same for 0, 33, and 66% bark treatments but was significantly higher at the 100% bark treatment. For SX64, the 0% bark treatment had a lower Magnesium concentration compared to the other three treatments. SV1 followed a slightly different pattern with slightly lower magnesium concentration values for the 0 and 100% bark treatments compared to the 33 and 66% bark treatments. For the 100% bark treatment, Sherburne had a significantly higher magnesium concentration than the other two willow cultivars (SX64 and SV1) and maple. Tharakan et al. (2003) reported magnesium content of 0.23 mg g−1 and 0.24 mg g−1 respectively for SV1 and SX64 cultivars with all their bark attached.
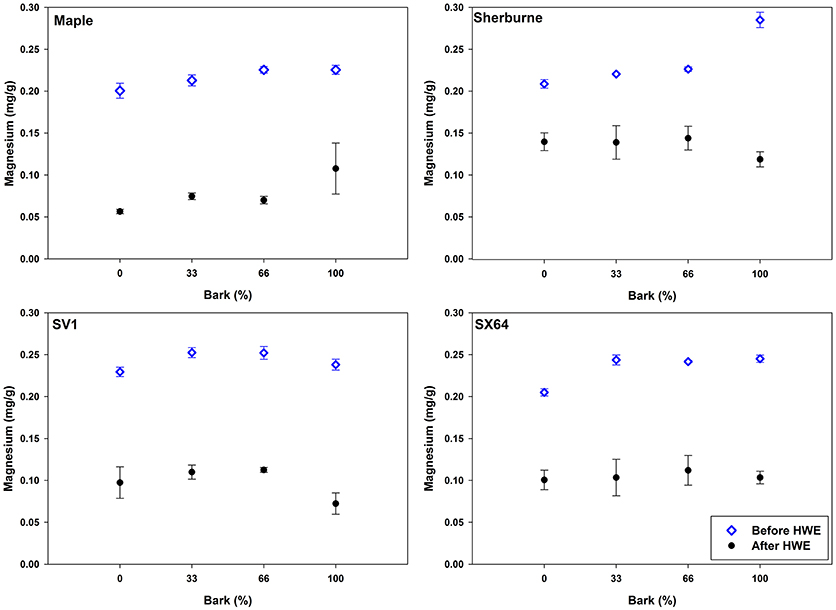
Figure 4. Magnesium content of sugar maple and three willow cultivars with bark levels ranging from 0% to 100% before and after HWE (errors bars represent standard deviations).
Similar to potassium content, the magnesium content of the three willow cultivars and sugar maple decreased after HWE to a stable value for bark treatments ranging from 0 to 66% (Figure 4). The average reduction of magnesium content was about 65% for sugar maple, 60% for SV1, and 55% for SX64 and 40% for Sherburne, for bark treatment ranging from 0 to 100%. Magnesium in the form of oxide plays a key role in the inhibition of alkali (K, Na) volatilization while burning biomass (Miles et al., 1996). Therefore, a reduction of magnesium would lead to an increase in the volatized alkali, but this effect would not be a concern as there was a greater decrease of alkali in the form of potassium.
Calcium Content
Calcium content of all three willow cultivars and sugar maple followed exactly the same trend as ash content. Calcium content of all three willow cultivars and sugar maple increased with increasing ash content (Table 3). Prior to HWE, sugar maple and willow samples with all their bark attached had 2.2–2.4 times more calcium than their corresponding samples with 0% bark. Sherburne with 100% bark treatment had the highest calcium content (7.932 ± 0.241 mg/g) and SV1 with 0% bark treatment had the lowest calcium (1.93 ± 0.04 mg/g), across all bark levels and willow cultivars (Table 3). Calcium concentrations reported in the literature (Tharakan et al., 2003) for SV1 and SX64 willow cultivars were slightly higher than those found in this present study.
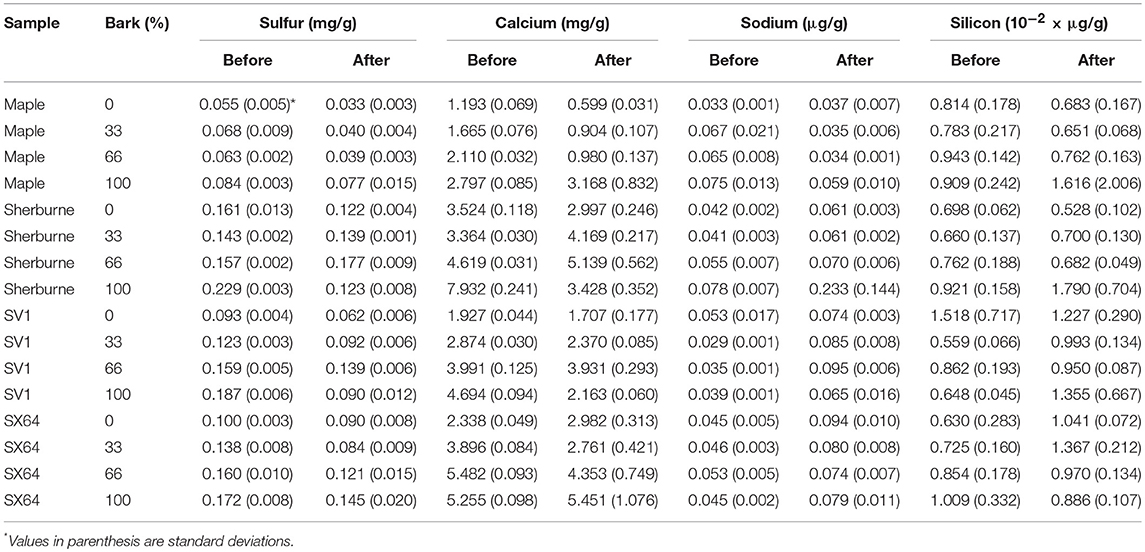
Table 3. Calcium, sodium, sulfur and silicon contents of sugar maple and three willow cultivars with bark levels ranging from 0 to 100% before and after HWE.
The effect of HWE on calcium content varied among the three willow cultivars and bark treatment. For 100% bark treatment, following HWE, calcium content in SV1 and Sherburne decrease by more than half of their calcium content prior to HWE while SX65 had no significant change. Additionally, HWE removed half of the initial calcium content in sugar maple with 0% bark treatment. No significant removal of calcium was observed for all three willow cultivars with 0% bark treatment (P > 0.15).
Sulfur, Sodium and Silicon Contents
As with other elements, sulfur content varied among cultivars, amount of bark and HWE treatment, following the same trend as ash (Figure 5). Prior to HWE, willow and sugar maple samples had significantly higher sulfur content than their corresponding samples with 0% bark treatment (Table 3). Prior to HWE, 100% bark treatment had 1.5 times more calcium than 0% bark treatment for sugar maple. But, it ranged from 1.4 to 2 times for the three willow cultivars. After HWE, there were no significant differences between 0% bark Sherburne and 100% bark Sherburne (P = 0.93). But, 100% bark treatment samples were 1.4–1.6 times higher than 0% bark treatment samples for SX64 and SV1, following HWE. Additionally, sulfur removal by HWE was the highest for 100% bark treatment SV1 and Sherburne.
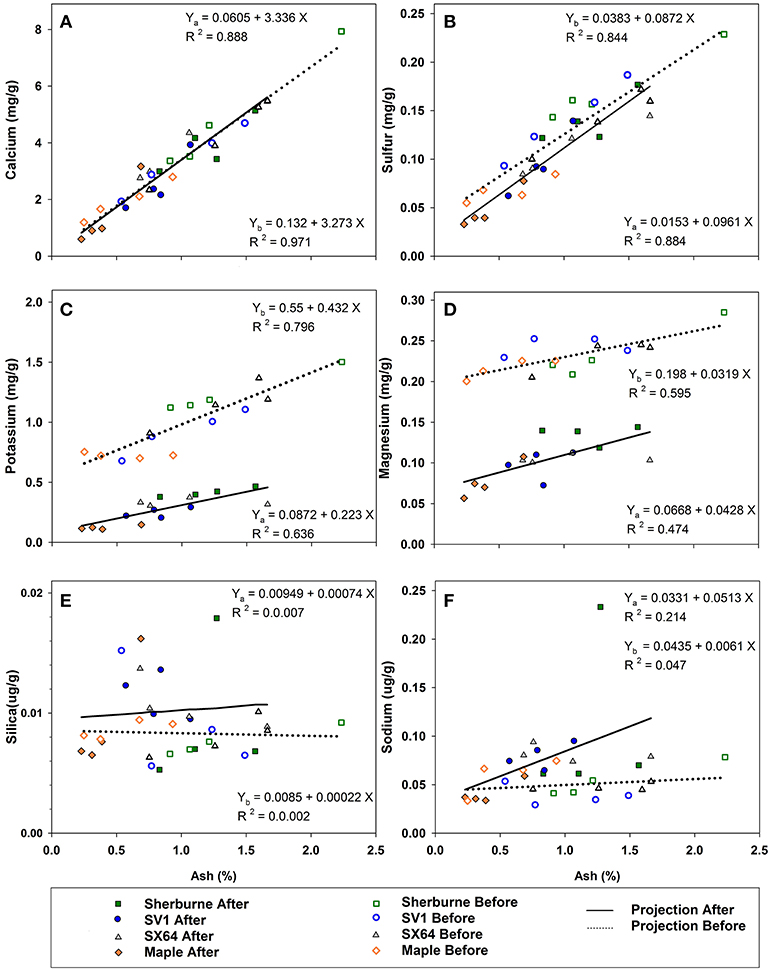
Figure 5. Changes in calcium (A), sulfur (B), potassium (C), magnesium (D), Silica (E) and sodium (F) contents with ash content and linear projections before and after HWE of sugar maple and three willow cultivars (Ya, dependent variable after HWE; Yb, dependent variable before HWE; and X, percentage of ash).
Unlike potassium, there were few differences in both sodium and silicon contents among willow cultivars and sugar maple samples, as well as different bark level and HWE treatment. Concentrations of sodium and silicon were the lowest for all three willow cultivars and sugar maple samples (Table 3), among all elements that were analyzed in this study.
Correlations
Calcium, sulfur, potassium and magnesium contents in willow and sugar maple had strong positive correlations with ash content before and after HWE (Table 4, Figure 5). Calcium, potassium and magnesium were strongly correlated to each other. There was no evidence of linear correlation among silica with ash across all the samples. Silica content in maple and willow is known to be relatively low compared to other elements, but soil contamination during harvesting can cause spikes (Tharakan et al., 2003). There was no evidence of significant linear correlation between ash and heating value for sugar maple (p = 0.13). However, prior to HWE, ash, potassium and magnesium had a negative correlation with heating value for SX64 and Sherburne cultivars. Ash content had a stronger correlation with potassium and magnesium in samples with all their bark attached than samples with 0% bark. As observed, ash was the lowest in samples with no bark.
Conclusion
The degree to which bark content impacts mass removal, sugars concentration in hot water extracts, and ash content, varies among willow cultivars and sugar maple. As bark content increased, the mass removal increased for sugar maple, but decreased for SV1 and SX64 at 0 to 66% bark treatment level. However, with increasing bark content, the total sugars concentration in the extract decreased, and the ash content increased for willow (hardwood) and sugar maple (hardwood). Hot water extraction improves the properties of the biomass as fuel source for bioenergy, and provides an extract that is rich in C5/C6 sugars. The residual material from this process still has the physical characteristics of a wood chip, but has chemical properties that make it more attractive than the original woodchip. The ash content of the extracted woodchip is lower and its energy density is greater than the original woodchip. The response among two key elements of ash (K, Mg) was that they were reduced to a consistent level following HWE across cultivars and bark treatments. The HWE process has been tested on debarked wood in the past; this research demonstrates that woodchips with bark can be used effectively in this process and that extracted woodchips from bark-on wood have significantly lower ash content and higher heating value. This will create new opportunities to make use of dirty wood, which has a significantly lower cost than debarked wood, while still yielding valuable products and a residual woodchip with improved properties. In the case of industries such as wood pellet manufacture, this would provide a significant cost savings and increase their profit margins. This work also demonstrates that willow biomass can be improved by this process and with improved characteristics that make them appealing for the production of wood pellets in a growing market. This will create new market outlets for willow biomass crops that were previously not available because of its high ash content.
Author Contributions
OT drafted the manuscript. AC performed a number of experiments and TA granted laboratory access for laboratory experiments. OT and ME performed the data analysis. TV, ME, OT, and TA revised the manuscript.
Funding
Funding to complete this research was provided by US Department of Energy Bioenergy Technologies Office under award number DE-EE0002992, the New York State Energy Research and Development Authority (NYSERDA) Award 30713, and USDA National Institute of Food and Agriculture under award 20161000825635.
Conflict of Interest Statement
The authors declare that the research was conducted in the absence of any commercial or financial relationships that could be construed as a potential conflict of interest.
Footnotes
1. ^TAPPI Ash in wood, pulp, paper and paperboard: combustion at 525°C.
References
Amidon, T. E., Bujanovic, B., Liu, S., and Howard, J. R. (2011). Commercializing biorefinery technology: a case for the multi-product pathway to a viable biorefinery. Forests 2, 929–947. doi: 10.3390/f2040929
Amidon, T. E., and Liu, S. (2009). Water-based woody biorefinery. Biotechnol. Adv. 27, 542–550. doi: 10.1016/j.biotechadv.2009.04.012
ASTM (2013). Standard Test Method for Gross Calorific Value of Coal and Coke. West Conshohocken, PA: American Society for Testing and Materials.
Barmpoutis, P., Lykidis, C., and Barboutis, I. (2015). Influence of stem diameter and bark ratio of evergreen hardwoods on the fuel characteristics of the produced pellets. Ligno 11, 673–679.
Bhosale, M. V. K., Kulkarni, S. G., and Kulkarni, P. S. (2016). Ionic liquid and biofuel blend: a low–cost and high performance hypergolic fuel for propulsion application. Chem. Sel. 1, 1921–1925. doi: 10.1002/slct.201600358
Chaturvedi, V., and Verma, P. (2013). An overview of key pretreatment processes employed for bioconversion of lignocellulosic biomass into biofuels and value added products. 3 Biotech 3, 415–431. doi: 10.1007/s13205-013-0167-8
Corbett, D., Kohan, N., Machado, G., Jing, C., Nagardeolekar, A., and Bujanovic, B. (2015). Chemical composition of apricot pit shells and effect of hot-water extraction. Energies 8, 9640–9654. doi: 10.3390/en8099640
Demirbaş, A. (2001). Relationships between lignin contents and heating values of biomass. Energy Convers. Manag. 42, 183–188. doi: 10.1016/S0196-8904(00)00050-9
Deng, L., Zhang, T., and Che, D. (2013). Effect of water washing on fuel properties, pyrolysis and combustion characteristics, and ash fusibility of biomass. Fuel Process. Technol. 106, 712–720. doi: 10.1016/j.fuproc.2012.10.006
Duarte, G. V., Ramarao, B. V., Amidon, T. E., and Ferreira, P. T. (2011). Effect of hot water extraction on hardwood kraft pulp fibers (Acer saccharum, Sugar Maple). Ind. Eng. Chem. Res. 50, 9949–9959. doi: 10.1021/ie200639u
Eich, S., Volk, T. A., and Eisenbies, M. H. (2015). Bark content of two shrub willow cultivars grown at two sites and relationships with centroid bark content and stem diameter. BioEnergy Res. 8, 1661–1670. doi: 10.1007/s12155-015-9617-y
Eisenbies, M. H., Volk, T. A., Posselius, J., Foster, C., Shi, S., and Karapetyan, S. (2014). Evaluation of a single-pass, cut and chip harvest system on commercial-scale, short-rotation shrub willow biomass crops. BioEnergy Res. 7, 1506–1518. doi: 10.1007/s12155-014-9482-0
Eisenbies, M. H., Volk, T. A., Posselius, J., Shi, S., and Patel, A. (2015). Quality and variability of commercial-scale short rotation willow biomass harvested using a single-pass cut-and-chip forage harvester. BioEnergy Res. 8, 546–559. doi: 10.1007/s12155-014-9540-7
Guan, W., Xu, G., Duan, J., and Shi, S. (2018). Acetone–Butanol–Ethanol production from fermentation of hot-water-extracted hemicellulose hydrolysate of pulping woods. Ind. Eng. Chem. Res. 57, 775–783. doi: 10.1021/acs.iecr.7b03953
Heller, M. C., Keoleian, G. A., and Volk, T. A. (2003). Life cycle assessment of a willow bioenergy cropping system. Biomass Bioenergy 25, 147–165. doi: 10.1016/S0961-9534(02)00190-3
ISO 17225-4 (2014). Solid Biofuels - Fuel Specifications and Classes. Geneva: International Organization for Standardization.
Jenkins, B. M., Bakker, R. R., and Wei, J. B. (1996). On the properties of washed straw. Biomass Bioenergy 10, 177–200. doi: 10.1016/0961-9534(95)00058-5
Kiemle, D. J., Stipanovic, A. J., and Mayo, K. E. (2003). “Proton NMR methods in the compositional characterization of polysaccharides,” in Hemicelluloses: Science and Technology ACS Symposium Series, eds P. Gatenholm and M. Tenkanen (Washington, DC: American Chemical Society), 122–139. doi: 10.1021/bk-2004-0864.ch009
Liu, S. (2010). Woody biomass: niche position as a source of sustainable renewable chemicals and energy and kinetics of hot-water extraction/hydrolysis. Biotechnol. Adv. 28, 563–582. doi: 10.1016/j.biotechadv.2010.05.006
Liu, S. (2015). A synergetic pretreatment technology for woody biomass conversion. Appl. Energy 144, 114–128. doi: 10.1016/j.apenergy.2015.02.021
Lu, H., Hu, R., Ward, A., Amidon, T. E., Liang, B., and Liu, S. (2012). Hot-water extraction and its effect on soda pulping of aspen woodchips. Biomass Bioenergy 39, 5–13. doi: 10.1016/j.biombioe.2011.01.054
Ma, X. J., Cao, S. L., Lin, L., Luo, X. L., Chen, L. H., and Huang, L. L. (2013). Surface characterizations of bamboo substrates treated by hot water extraction. Bioresour. Technol. 136, 757–760. doi: 10.1016/j.biortech.2013.03.120
Magagnotti, N., Nati, C., Pari, L., Spinelli, R., and Visser, R. (2011). Assessing the cost of stump-site debarking in eucalypt plantations. Biosyst. Eng. 110, 443–449. doi: 10.1016/j.biosystemseng.2011.09.009
Mante, O. D., Amidon, T. E., Stipanovic, A., and Babu, S. P. (2014). Integration of biomass pretreatment with fast pyrolysis: an evaluation of electron beam (EB) irradiation and hot-water extraction (HWE). J. Anal. Appl. Pyrolysis 110, 44–54. doi: 10.1016/j.jaap.2014.08.004
Mészáros, E., Jakab, E., Várhegyi, G., Szepesváry, P., and Marosvölgyi, B. (2004). Comparative study of the thermal behavior of wood and bark of young shoots obtained from an energy plantation. J. Anal. Appl. Pyrolysis 72, 317–328. doi: 10.1016/j.jaap.2004.07.009
Miles, T. R., Baxter, L. L., Bryers, R. W., Jenkins, B. M., and Oden, L. L. (1996). Boiler deposits from firing biomass fuels. Biomass Bioenergy 10, 125–138. doi: 10.1016/0961-9534(95)00067-4
Mittal, A., Scott, G. M., Amidon, T. E., Kiemle, D. J., and Stipanovic, A. J. (2009a). Quantitative analysis of sugars in wood hydrolyzates with 1H NMR during the autohydrolysis of hardwoods. Bioresour. Technol. 100, 6398–6406. doi: 10.1016/j.biortech.2009.06.107
Mittal, A., Siddharth, G. C., Gary, M. S., and Thomas, E. A. (2009b). Modeling xylan solubilization during autohydrolysis of sugar maple wood meal: Reaction kinetics. Holzforschung 63:307. doi: 10.1515/HF.2009.054
Morris, V. H., and Sayre, J. D. (1935). Solubility of potassium in corn tissues. Plant Physiol. 10:565. doi: 10.1104/pp.10.3.565
Novaes, E., Kirst, M., Chiang, V., Winter-Sederoff, H., and Sederoff, R. (2010). Lignin and biomass: a negative correlation for wood formation and lignin content in trees. Plant Physiol. 154, 555–561. doi: 10.1104/pp.110.161281
Obernberger, I., and Thek, G. (2004). Physical characterisation and chemical composition of densified biomass fuels with regard to their combustion behaviour. Biomass Bioenergy 27, 653–669. doi: 10.1016/j.biombioe.2003.07.006
Palmqvist, E., and Hahn-Hägerdal, B. (2000). Fermentation of lignocellulosic hydrolysates. II: inhibitors and mechanisms of inhibition. Bioresour. Technol. 74, 25–33. doi: 10.1016/S0960-8524(99)00161-3
Pu, Y., Treasure, T., Gonzalez, R., Venditti, R., and Jameel, H. (2011). Autohydrolysis pretreatment of mixed hardwoods to extract value prior to combustion. BioRes. 6, 4856–4870.Available online at: https://bioresources.cnr.ncsu.edu/resources/autohydrolysis-pretreatment-of-mixed-hardwoods-to-extract-value-prior-to-combustion/
Schneider, M. H., and Phillips, J. G. (2004). Furfuryl Alcohol and Lignin Adhesive Composition. Available online at: http://www.uspto.gov/ (Accessed August 29, 2016).
Schulte, E. E., and Huang, C.-Y. L. (1985). Digestion of plant tissue for analysis by ICP emission spectroscopy. Commun. Soil Sci. Plant Anal. 16, 943–958. doi: 10.1080/00103628509367657
Serapiglia, M. J., Cameron, K. D., Stipanovic, A. J., and Smart, L. B. (2009). Analysis of biomass composition using high-resolution thermogravimetric analysis and percent bark content for the selection of shrub willow bioenergy crop varieties. BioEnergy Res. 2, 1–9. doi: 10.1007/s12155-008-9028-4
Serapiglia, M. J., Humiston, M. C., Xu, H., Hogsett, D. A., Orduña, R. M., de, Stipanovic, A. J., et al. (2013). Enzymatic saccharification of shrub willow genotypes with differing biomass composition for biofuel production. Front. Plant Sci. 4:57. doi: 10.3389/fpls.2013.00057
Sleight, N. J., Volk, T. A., Johnson, G. A., Eisenbies, M. H., Shi, S., Fabio, E. S., et al. (2016). Change in yield between first and second rotations in willow (Salix spp.) biomass crops is strongly related to the level of first rotation yield. BioEnergy Res. 9, 270–287. doi: 10.1007/s12155-015-9684-0
Stolarski, M. J., Szczukowski, S., Tworkowski, J., and Klasa, A. (2013). Yield, energy parameters and chemical composition of short-rotation willow biomass. Ind. Crops Prod. 46, 60–65. doi: 10.1016/j.indcrop.2013.01.012
Tharakan, P. J., Volk, T. A., Abrahamson, L. P., and White, E. H. (2003). Energy feedstock characteristics of willow and hybrid poplar clones at harvest age. Biomass Bioenergy 25, 571–580. doi: 10.1016/S0961-9534(03)00054-0
Thomsen, M. H., Thygesen, A., and Thomsen, A. B. (2009). Identification and characterization of fermentation inhibitors formed during hydrothermal treatment and following SSF of wheat straw. Appl. Microbiol. Biotechnol. 83, 447–455. doi: 10.1007/s00253-009-1867-1
USDOE (2011). U.S. Billion-ton Update: Biomass Supply for a Bioenergy and Bioproducts Industry, eds R. D. Perlack, B. J. Stokes. Oak Ridge, TN: Oak Ridge National Laboratory.
Volk, T. A., Abrahamson, L. P., Cameron, K. D., Castellano, P., Corbin, T., Fabio, E., et al. (2011). Yields of willow biomass crops across a range of sites in North America. Asp. Appl. Biol. 112, 67–74.
Volk, T. A., Abrahamson, L. P., Caputo, J., Eisenbies, M. H., and Buchholz, T. (2014). “Development and deployment of willow biomass crops,” in Cellulosic Energy Cropping Systems, ed D. L. Karlen (Chichester: John Willey and Sons), 201–217.
Wang, K.-T., Chengyan, J., Christopher, W., Aditi, N., Neil, K., Prajakta, D., et al. (2017). Toward complete utilization of miscanthus in a hot-water extraction-based biorefinery. Energies 11:39. doi: 10.3390/en11010039
Wang, W., Yuan, T., Wang, K., Cui, B., and Dai, Y. (2012). Combination of biological pretreatment with liquid hot water pretreatment to enhance enzymatic hydrolysis of Populus tomentosa. Bioresour. Technol. 107, 282–286. doi: 10.1016/j.biortech.2011.12.116
White, R. H. (2007). Effect of lignin content and extractives on the higher heating value of wood. Wood Fiber Sci. 19, 446–452.
Wild, S. A., Corey, R. B., Lyer, L. G., and Voigt, G. K. (1979). Soil and Plant Analysis for Tree Culture. New Delhi: Oxford & IBH Publishing Co.
Williams, C. L., Westover, T. L., Emerson, R. M., Tumuluru, J. S., and Li, C. (2016). Sources of biomass feedstock variability and the potential impact on biofuels production. BioEnergy Res. 9, 1–14. doi: 10.1007/s12155-015-9694-y
Yan, K., Wu, G., Lafleur, T., and Jarvis, C. (2014). Production, properties and catalytic hydrogenation of furfural to fuel additives and value-added chemicals. Renew. Sustain. Energy Rev. 38, 663–676. doi: 10.1016/j.rser.2014.07.003
Keywords: SRWC, willow, hot water extraction, heating value, ash, alkali metals, biomass
Citation: Therasme O, Volk TA, Cabrera AM, Eisenbies MH and Amidon TE (2018) Hot Water Extraction Improves the Characteristics of Willow and Sugar Maple Biomass With Different Amount of Bark. Front. Energy Res. 6:93. doi: 10.3389/fenrg.2018.00093
Received: 11 April 2018; Accepted: 24 August 2018;
Published: 11 September 2018.
Edited by:
Timothy G. Rials, University of Tennessee, Knoxville, United StatesReviewed by:
Ming Zhai, Harbin Institute of Technology, ChinaRichard Arthur Venditti, North Carolina State University, United States
Copyright © 2018 Therasme, Volk, Cabrera, Eisenbies and Amidon. This is an open-access article distributed under the terms of the Creative Commons Attribution License (CC BY). The use, distribution or reproduction in other forums is permitted, provided the original author(s) and the copyright owner(s) are credited and that the original publication in this journal is cited, in accordance with accepted academic practice. No use, distribution or reproduction is permitted which does not comply with these terms.
*Correspondence: Obste Therasme, b3RoZXJhc21Ac3lyLmVkdQ==