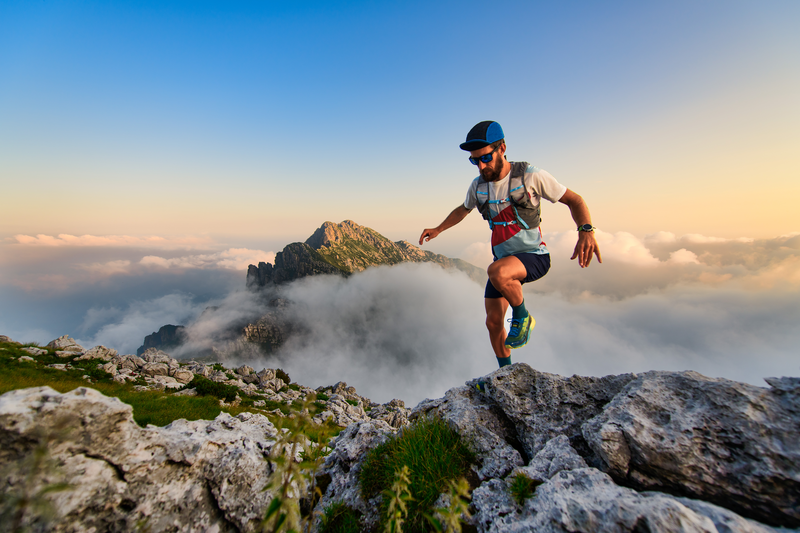
95% of researchers rate our articles as excellent or good
Learn more about the work of our research integrity team to safeguard the quality of each article we publish.
Find out more
ORIGINAL RESEARCH article
Front. Energy Res. , 13 September 2017
Sec. Carbon Capture, Utilization and Storage
Volume 5 - 2017 | https://doi.org/10.3389/fenrg.2017.00022
This article is part of the Research Topic Carbon Dioxide Utilisation View all 10 articles
A correction has been applied to this article in:
Corrigendum: Overcoming Barriers to Successfully Commercializing Carbon Dioxide Utilization
The successful transition to a low-carbon economy hinges on innovative solutions and collaborative action on a global scale. Sustainable entrepreneurship is thereby recognized as a key driver in the creation and transformation of ecologically and socially sustainable economic systems. The purpose of this article is to contribute to this topic by understanding commercialization barriers for strong sustainability-oriented new technology ventures and to derive recommendations to overcome them. A qualitative multilevel approach is applied to identify barriers and drivers within the internal dynamic capabilities of the organization and within the organization’s external stakeholders. A model of barriers has been developed based on semi-structured interviews with new carbon dioxide utilization ventures and associated industry players in Canada, the USA, and the European Economic Area. Resulting recommendations to facilitate the (re-)design of a dedicated support system are proposed on four levels: (a) actors, (b) resources, (c) institutional settings, and (d) the coordination of the support system.
Paris’s COP21 (UNFCCC, 2015) and the G7 Summit (G7 Germany, 2015) in 2015 emphasize the need for a transition to a low-carbon economy. Moreover, increasing amounts of money will be invested in clean technologies over the next decades (UNFCCC, 2015), potentially boosting sustainable-oriented innovation. However, effective allocation and support systems to leverage the sustainability transition of entire industries are still relatively unexplored.
In recent years, sustainable entrepreneurship (SE) has been recognized as key in the creation and transformation of ecologically and socially sustainable economic systems (Pacheco et al., 2010) such as a low-carbon economy. Furthermore, SE is identified as an essential driving force in the sustainability-oriented innovation (SOI) process (Jay and Gerard, 2015): the multidirectional sequence of inventing, developing and diffusing new sustainability-oriented technologies and ideas.
This work uses Schaltegger’s and Wagner’s definition of SE, because it ties SE and SOI together: “Sustainable entrepreneurship is in essence the realization of sustainability[-oriented] innovations aimed at the mass market and providing benefit to the larger part of society” (Schaltegger and Wagner, 2011, p. 225). The following section focuses on SE (realization of SOI) and its role in the sustainability transition (environmental and social benefits to society and market impact).
Literature increasingly deals with SE (cf. Schaefer et al., 2015), and entrepreneurship has been proposed as a solution to environmental problems (York and Venkataraman, 2010). SE identifies market opportunities and addresses market failures by aligning environmental, social, and economic aspects of sustainability (Cohen and Winn, 2007; Hall et al., 2010; Parrish, 2010; Thompson et al., 2011). Elkington (1998) coined this alignment as the “triple bottom line” principle. Porter and Kramer (2011) put Elkington’s triple bottom line in a business perspective, arguing that business should deal with society’s environmental and social issues such as resource depletion and climate change to create a shared value. The participation of multiple stakeholders and actors in the innovation process is required to create such a shared value. Hence, the involvement of stakeholders outside the organization becomes paramount for SOI and SE (Paech, 2007). This leads to the multilevel perspective on all stakeholder along the innovation value-added chain of this study to realize SOI.
The sustainability-orientation of an innovation determines the provided benefits for society, the environment, and the economy. Scholars often distinguish the degree of sustainability in two to three ascending categories (Klewitz and Hansen, 2014; Jay and Gerard, 2015; Muñoz and Dimov, 2015). The highest degree of sustainability may be achieved by radical (cf. Christensen, 1997) rather than incremental change (Roome, 2012). Therefore, this research focuses particularly on radical innovation which is either “(…) explicitly directed at a sustainability goal” or implicitly adhered to sustainability goals without having sustainability issues as a primary target (Blowfield et al., 2008, p. 2). Thus, strong sustainability-orientation and meaningful impact on sustainability transformation may be attained.
Although large incumbents struggle with radical innovation, incumbents seem key in diffusing sustainability innovations to mass markets because they have the necessary assets at their disposal (Schaltegger and Wagner, 2011) and often directly sell to other businesses (Parker, 2011). Hockerts and Wüstenhagen (2010) investigated the interplay of large incumbents and new technology ventures. Their work on “Greening Goliaths” and “Emerging Davids” describe the necessary interaction to attain sustainability transformations. Therefore, this study focuses on radical new technology ventures and their endeavor to bring sustainability-oriented products/services to the market whilst particularly acknowledging the interaction with external stakeholders such as large industry players.
The radical nature of an innovation is quite often best depicted in hardware-based technologies. The problem with hardware-based technologies is that a proof-of-concept on a technically relevant scale is essential when attracting external resources to sustain business operations and business growth. These proofs of a scale-up production for hardware-based sustainability-oriented technology applications range from demonstrators over pilot production facilities to large commercial plants and are mostly very capital-intensive (Bossink, 2014). Capital intensity, in turn, greatly influence investment risks for sustainability-oriented technologies such as renewable energy (Tietjen et al., 2016).
In this regard, Bürer and Wüstenhagen (2009) (based on Grubb, 2004) refine Murphy’s and Edwards’ (Murphy and Edwards, 2003) concept of the cash flow valley of death and describe this technology valley of death as the middle phase between publicly funded R&D and self-sustaining funding from (private) partners/customers, where a successful prototype needs to scale-up further to introduce a product/service to the market successfully. When this valley of death is not bridged, sustainability-oriented ventures are lacking and current societal challenges such as resource depletion and climate change are not being tackled through radical innovation.
Carbon dioxide (CO2) utilization technology ventures typically combine the underlying problem of high capital intensity and the preconditions for a sustainability transition due to a high level of technology radicalness for a strong sustainability-orientation and mass application potential. The author defines CO2 utilization as innovative approaches to convert CO2 molecules to other molecules. This definition builds on Styring’ and Jansen’s (Styring and Jansen, 2011) definition by adding the innovation aspect of not being fully commercialized yet.
The sustainability effect of CO2 utilization is reflected by the fact that the use of CO2 as a raw material may not only be a door-opener for large emission abatement technologies but may also be a potent resource efficiency technology because it feeds CO2 back into the carbon-based economic system (Armstrong and Styring, 2015; Styring et al., 2015; Naims, 2016). Furthermore, CO2-based products such as CO2-based fuel are about to reach larger markets (Aresta et al., 2013) and thereby gaining increasingly importance for sustainability transitions.
However, a successful diffusion of a technology in society is crucial to have meaningful SOI (Hall and Clark, 2003; Boons and Lüdeke-Freund, 2013). Hence, this article has a particular focus on the commercialization phase (cf. Pellikka, 2014) and looks at the critical process step between product development and commercialization, as it is at this stage that most sustainable entrepreneurs fail.
Barriers, drivers, and success factors of SE have already been drawn to the center of attraction of business scholars (Bernauer et al., 2007; Walker et al., 2008; Pinkse and Dommisse, 2009; Kennedy et al., 2013; Pinkse and Groot, 2015). Pinkse and Groot (2015), for example, focus on market barriers, namely inefficiency, externalities and imperfect information, identifying SE as a possible way to overcome these barriers. They argue that market barriers, once overcome by entrepreneurs, who are involved in political collective activities via industry associations, can reveal entrepreneurial opportunity (ibid). However, most of these research findings are adding sustainability-specific characteristics to findings from conventional innovation or entrepreneurship studies (Walker et al., 2008; Driessen et al., 2013; Jay and Gerard, 2015).
This article contributes to the literature by addressing the following primary research question: What are the barriers and drivers for successful commercialization of strong sustainability-oriented new technology ventures?
The aim of this work is twofold: firstly, to gain a better understanding of the internal and external barriers to the commercial success of new CO2 utilization technology ventures; and secondly, to use the identified barriers to derive hands-on recommendations to (re-)design a dedicated support system for these technologies. It brings together different research on SE in the light of sustainability transition to illustrate the complex and dynamic process to commercialize new sustainability-oriented technologies. By researching new CO2 utilization ventures in Canada, the USA, and the European Economic Area (EEA) a broad set of barriers and drivers have been identified. Subsequently, detailed recommendations for strong sustainability-oriented new technology ventures, policy makers, and support providers are derived to facilitate the implementation of strong SOI such as CO2 utilization.
Previous research noted that the commercialization process of a small firm has both an internal (e.g., managerial actions and decision making) and an external (e.g., interaction with commercialization environments) dimension of activities (cf. Pellikka, 2014). Especially, the dependency of small firms, such as new technology ventures on external resources, has been recognized (e.g., Oakey, 2007). Pellikka’s (Pellikka, 2014) framework of the commercialization process in small high-technology ventures sheds light on the interface between internal and external commercialization dimensions and thus may help to discover how firms can receive these external resources.
Other scholars also acknowledge these internal and external dimensions. Walker et al. (2008), for example, performed a literature review on barriers and drivers of small and medium enterprises to engage in good environmental practice. They point out two perspectives how barriers are perceived: the firm perspective, representing the internal dimension and the government perspective, as one of the main representations of the external dimension (ibid). Acknowledging such of a multilevel perspective can also help to better understand ambiguous findings such as barrier of company A is a driver for company B (Hueske and Guenther, 2015). Hueske and Guenther (2015) propose a barrier framework that enables a more encompassing identification, on different level of analysis, of innovation barriers and draws from theory.
This work builds on a multilevel perspective from current relevant research (Hueske and Guenther, 2015) to investigate all barriers to commercial success. A dynamic capabilities approach (Teece et al., 1997; Eisenhardt and Martin, 2000), in combination with stakeholder theory (Freeman, 2004; Tantalo and Priem, 2016), was chosen to analyze both internal and external barriers that exist alongside the innovation value-added chain. Applying this analysis on multiple levels enables a focus on interactions of barriers within and across the levels of analysis. Furthermore, it acknowledges the specificity of context and thereby allows for a broad set of recommendations (Hueske and Guenther, 2015) and an increased contextual understanding. Both, the organizational and the external level define (cross-)barrier categories and sub-categories are set out within the different levels. Figure 1 pictures the applied barrier framework.
Figure 1. Applied barrier framework (adapted from Hueske and Guenther, 2015).
Freeman et al. (2007, 2010) argue that stakeholders have competing goals when creating value that need to be addressed by managerial trade-off decision making. In contrast to Freeman’s mind-set, the stakeholder synergy approach addresses how value can be created simultaneously for stakeholder groups that are essential for a firm (Tantalo and Priem, 2016). This approach helps to identify value creation opportunities both within and across relevant stakeholder groups (ibid). Seven key stakeholder groups can be observed: (1) investors, (2) future employees, (3) partners and suppliers in research, engineering and manufacturing, (4) competitors, (5) customers, (6) governments, and (7) society (Hueske and Guenther, 2015).
Dynamic capabilities may be used to create new resource configuration and update the competitive position of an organization. Furthermore, dynamic capabilities include processes such as collaboration and product development that may turn resources into value-creating strategies (Eisenhardt and Martin, 2000).
An explorative qualitative interview study was used to investigate the new CO2 utilization venture landscape worldwide. This research approach is suitable for early stage research such as SE in CO2 utilization (Silverman, 2013). Furthermore, the approach was chosen to enable the enfoldment of existing theory by analyzing capability-driven, internal barriers and stakeholder-driven, external barriers (Eisenhardt, 1989).
The empirical setting is carefully chosen and looks specifically on the EEA, Canada, and the USA. This choice was made to exclude too much variation due to differences between industries.
The author choses a theory-based purposeful sampling approach to identify and select information-rich interviews (Palinkas et al., 2015; Patton, 2015). Experienced and knowledgeable individuals, which were available and willing to participate, have been identified to make an effective use of limited research resources (Palinkas et al., 2015). New CO2 utilization ventures were identified globally via (1) the author’s participation at several events (such as conferences and workshops) with a CO2 utilization theme and (2) an extensive online desk research on past conferences, privately and publicly funded projects/programmes/prizes, and aggregated news platforms. A population of N = 48 existing new CO2 utilization venture companies (as of October 2015) were identified with a great concentration in the EEA, Canada, and the USA.
Smit et al. (2014) divide carbon utilization into four sections: enhanced oil recovery, CO2 to chemicals, CO2 to fuels, and incorporating CO2 into construction and building material. This categorization is based on the output of the various CO2 utilization technologies (Peters et al., 2011; see also Styring and Jansen, 2011; Hendriks et al., 2013). The author of this work adapted the categorization by application class of CO2 utilization technologies, but included only those categories that are in line with the CO2 utilization definition of this paper. Incorporating CO2 into construction and building material is mainly carried out by mineralization of CO2. Hence, the population and sample were divided into CO2 to chemicals, CO2 to fuels, or CO2 mineralization.
Typically, these categories contain various technologies that address different industry sectors:
• CO2 mineralization turns CO2, minerals, and industrial waste mainly into aggregates or fillers for the building industry (Styring et al., 2015).
• CO2 to chemicals primarily cover applications such as intermediates, specialty chemicals or precursors such as polyols for the plastic production (ibid).
• CO2 to fuels include primary power-to-x technologies. Power-to-x processes—in the context of CO2 utilization—use electricity to transform CO2 into liquid or gaseous fuels, e.g., for the transportation sector or as seasonal storage for the energy market (ibid).
Other possible transformation pathways of power-to-x are chemicals with more complex molecular structure such as pharmaceuticals (ibid). Hence, also a hybrid category of “CO2 to chemicals” and “CO2 to fuels” is possible. The use of microorganisms is another method to convert CO2 into fuels and/or chemicals (Styring and Jansen, 2011).
Within the population of N = 48 companies, 19 initial interviews were conducted with 18 different ventures. The geographical distribution and the shares within the categories of the identified CO2 utilization ventures of the population were well reflected in the sample (cf. Figure 2). The interviewees’ position ranged from CEOs, CTOs, and CFOs to R&D managers, business developers, and operations and sales persons. Most of the interviewees were intentionally the (co-)founders of the company because they potentially have the best overview of the company and can share in-depth insight into business operations and strategies. 30- to 80-min (Ø 45 min) interviews were conducted face-to-face and/or via telecommunication between October 2015 and April 2016. In addition to the initial 19 interviews, two more interviews with new CO2 utilization ventures were conducted in July and November 2016, adding up to n = 20 companies with a total of 900 min of recording time. This enabled further insights and the ability to test propositions derived from previous interviews. All interviews were semistructured and a semiopen questionnaire was adapted from interview studies on similar technology fields (e.g., Matus et al., 2012). The semiopenness allowed for an ongoing adjustment of the questionnaire in the course of the interview series; in combination with the sequenced interview process over several months, interviews were carried out until no new phenomenon were discovered and therefore theoretical saturation was reached (Strauss and Corbin, 2015). In addition, a ranking of perceived success factor categories, which were identified in the literature, was carried out in twelve interviews. All initial interviews were recorded, transcribed, and send to the respective interviewee for validation prior to the anonymized data analysis process. The study was carried out in accordance with the German law. No ethics approval was required for this type of research as per German laws and regulations. In compliance with these laws and regulations, oral informed consent was obtained from all research participants. Their answers were anonymized and it is not possible to link the statements back to individual subjects. Figure 2 provides further aggregated information about the sample on the ordinate.
To enhance contextual understanding, additional data sources such as publicly available reports were used to triangulate the venture’s positions (proposition testing). Furthermore, during the data collection process four interviews with a steel producer, utility, infrastructure provider, and chemical company were conducted in the EEA to give a broader perspective from additional stakeholders. These four interviews with five interviewees were surveyed over a total of 140 min.
Data were analyzed using the software Atlas.ti 7 (ATLAS.ti, 2016). An open and axial coding process (Strauss and Corbin, 2015) was performed to extract internal and external commercialization barriers and increase the reliability of the analysis. Following the coding process, codes of each code family were manually extracted. The codes were further aggregated and duplicates were removed. This extraction revealed cross-family and cross-level occurrences of barriers and contextualized the data from the interview by comparing institutional settings and company types: the EEA vs. Canada and the USA, and large incumbents vs. new technology ventures. Table 1 states an exemplary overview of the data analysis process.
Table 1. Exemplary overview of the data analysis process (adapted from Hahn and Ince, 2016).
A qualitative multilevel model on the internal and external barriers to successful commercialization has been developed based on the families from the coding process (cf. Figure 3). External barrier categories focus on the external stakeholders of the value-added chain of SOI and the internal categories reflect the sustainability-oriented venture itself. External stakeholder are public and private investors, future employees, partners in R&D and manufacturing, competitors, customers, governments, and society. The internal organization is divided into strategy, size and structure, and resources of the organization. Resources can be further broken down into infrastructural, technological and financial resources, knowledge and networks, and management and team in terms of social and human capital. Furthermore, a third main category was derived from the analysis, because of the reoccurring nature of three barrier categories at the immediate interface of internal and external barriers: promoter, location, and risk. They are referred to as cross-linkage barriers.
The following part provides an overview of the barriers of each category and their main drivers. Moreover, it indicates cross-barrier relationships across the three main categories: external, internal and cross-linkage barriers and compares the predominant institutional settings and company types.
All barriers and drivers in this section are extracted from the underlying data of the qualitative research, unless stated otherwise. They represent the individual perception of the new CO2 utilization ventures and industry player.
New CO2 utilization ventures encounter various commercialization barriers in their external environment (cf. Table 2). This environment is mainly determined by investors, future employees, partners in R&D and manufacturing, competitors, customers, governments, and society.
Barriers when dealing with investors in the field of CO2 utilization fall within investment behavior, requirements dilemma, operational and strategic involvement, and in access to investments. The risk taking of investors is not much different to other hardware-based technology investments. However, uncertainties in regulations, the preference for asset-backed investments, and the fact that there is often yet no market with a minimum assurance were reported to discourage the risk affinity for CO2 utilization further. In addition, study participants stated that the clean tech sector still suffers from a loss of reputation due to investment decisions during the mid-2000s: institutional investors without suitable sector-specific experience invested heavily into clean energy technologies (e.g., Rai et al., 2015) contributing to poor financial returns between 2000 and 2010 (e.g., Bygrave et al., 2014).
These experiences led to a set of requirements that put new technology ventures to the test. A proof-of-concept on an industrial scale (actual system prototype or proven technology in its final form) is often mandatory and existing agreements with off-takers/customers are required as described by the interviewees.
Moreover, the degree of an investor’s involvement can result in a trade-off decision: the investor may bring crucial market and management expertise but might also curtail the new venture’s freedom and fail to acknowledge its expertise as reported differently by the study participants. Either way, the interviewees highlighted that institutional investors are necessary to scale-up and grow. Hence, access to these investments were noted to be of upmost importance and new CO2 utilization ventures stated to often lack the network to gain that investment access.
Barriers in the context of future employees are competitive job markets and the staffing process. Job markets like any other market are influenced by world economics. Especially the oil market developments pre and post the 2000s were pointed out to have shaped the availability of technical personal in the USA and also in Europe. In general, the risk affinity of future employees and thereby the willingness to work in a firm with a high probability to fail is claimed to be low. New CO2 utilization ventures also began to strategize and move to certain locations to get access to greater talent pools. Close ties to university can facilitate the staffing process. However, there is also a trade-off between proximity for partnerships and recruitment. Overall, fluctuation in the management, and the internal development of necessary skillsets and the organizational fit of future employees were described to remain challenging.
Additional barriers related to external stakeholders concern partners in R&D and manufacturing. The partner’s alignment with new CO2 utilization ventures emerges as a barrier, when it comes to the strategy for a technology development that takes at least 3–5 years and the motivation that depends on the position within the value chain. Furthermore, there are proclaimed challenges in collaboration and resource sharing (knowledge transfer and infrastructure). New CO2 utilization ventures need to assess and identify potential partnerships to engage in a collaboration. The roles of partner, however, are not necessarily easy to define, the coordination of partnerships is resource intensive and the matchmaking process requires the presence of extensive networks as described by the interviewee. Nevertheless, outsourcing strategies and approaches to build entire synergetic ecosystems with crucial value chain stakeholders involved were identified as attempts to overcome shortcomings in resources. Knowledge transfer—by means of market and industry intelligence, and management expertise—and the provision of infrastructure, offer opportunities for building up intellectual property (IP) portfolios and testing capabilities under real life conditions, but were recognized as very challenging to establish.
The competitor category reveals market entry barriers in form of lock out, subsidization and scale effects. New CO2 utilization ventures claim to face threats of being locked out of the market by competitors temporarily lowering prices for commoditized products. Such entry deterrence strategies of large incumbents in high volume markets with little product margins are reported to require significant price advantages of new technology ventures over current prices to be competitive. Furthermore, inconsistencies within the subsidization of renewable energy or fossil fuels directly influence competing technologies when certain technologies are favored (e.g., Victor, 2009; Hope et al., 2015) or exemptions for certain companies are made [e.g., the special equalization scheme under the renewable energy sources act in Germany (§§ 63 ff. Erneuerbare-Energien-Gesetz—EEG 2017)]. Aside from these subsidies, scale effects cater for low costs of mature technologies such as fossil-based productions as described by the interviewees.
During the interaction with customers new CO2 utilization ventures encounter barriers regarding a proof-of-concept, the participation of potential customers, specific customer characteristics, and global market dynamics. A proof-of-concept in form of a pilot production facility with a high quality of product samples is mandatory for most potential customers to sign any kind of offtake agreement; this in turn is often necessary for investors as recurrently pointed out by the study participants. However, risk profiles of customers are reported to vary depending on the position within the value chain (e.g., 1/10 of scale for several months vs. full scale for several years). Moreover, new CO2 utilization ventures can share customers’ risks by issue commissioning warranties. A financial challenge yet remains: either as a proof-of-concept or as the backing of warranties. Furthermore, customer’s problems need to be acknowledged and the solutions need to be understood from both, the customer and the new CO2 utilization venture as pointed out by the participants. To do so, an early participation and specific customer characteristics are identified drivers that must be addressed. New CO2 utilization ventures have experienced challenges in complying with industry requirements such as ownership models, investment abilities, and customers’ intrinsic motivations to lower the environmental impact. Policy intervention and market dynamics were described to further influence these specific characteristics and to be potential barriers in themselves. Overall there is little perceived willingness to pay a premium for environmentally and socially superior products in the field of CO2 utilization: Only one out of 14 interviewees sees a general willingness to pay a premium for carbon-based products; one interviewee does not know and 12 interviewee see no or very limited willingness of a small fraction of customers to pay premium prices.
Further barriers that are related to governments are in the categories competences, regulation, and other incentives. In terms of competences, the study participants claim that there is a lack of foresight and most regulation is based on current rather than novel technologies. Moreover, responsibilities are often not clearly defined and passed on between different authorities (e.g., between the different Directorate-Generals of the European Union). With respect to regulatory frameworks translation, harmonization, and sovereignty is often an issue for rolling-out CO2 utilization technologies globally. Regulation frameworks are perceived as too complex, volatile, and can even create market perversion, e.g., by encouraging the generation of more and more energy rather than focusing on a more efficient use (e.g., via storage). The barriers for funding applications are noted to be considerable for small companies and thereby limit the funding access. Other incentives like a general sponsorship for the implementation of CO2 utilization technologies are underrepresented and long-term strategies and global agendas for CO2 utilization were identified as still missing by the interviewees.
When it comes to society, the main barrier is public perception and acceptance. There is a perceived negative misconception of CO2, especially when new CO2 utilization ventures are connected to the emitting industries. Dedicated marketing strategies and education programmes are identified as widely missing.
New CO2 utilization ventures experience also barriers within their organization (cf. Table 3). These barrier categories range from size and structure over strategy to resources. Like other new technology ventures, CO2 utilization ventures encounter challenges because of their size. The competitiveness is affected by capabilities to compete on market prices and even below-market (dumping) prices as reported by the study participants. Moreover, scale effects such as the fossil industry built up over decades, complex regulatory framework, and limitations to take on multiple roles simultaneously such as investor, owner, manufacturer, and operator influence the perceived competitiveness even further. The internal structure of new CO2 utilization ventures greatly influence the decision making of investors. An understanding of the investment rationale is noted to enhance the chances of survival and future growth.
Strategy-related barriers relate to market characteristics, value creation, IP protection, and project financing. The market entry strategy must meet the specific market requirements and characteristics. Hence, a focus on the technological application is observed to be necessary. Opportunities may arise when focusing on bridging applications to decarbonize the transportation sector or to store energy. New CO2 utilization ventures need to target customers that are at a particular stage in the value chain, even though their technology can have different outlets for different value chain positions. Moreover, they need to balance relevance in R&D and marketability of their technology and need to decide on how much value they want to capture and how to acknowledge and build on the existing competencies of their potential customers as reported by the interviewees. When approaching a customer, study participants noted that challenges regarding the IP arise. A rigid patenting strategy might help to facilitate immediate interaction, but is also very capital intensive as pointed out by the interviewees. Having a sound strategy to finance and to implement collaborative projects holds further perceived hurdles that may be driven by staged project approaches.
Internal barriers in the resource domain are grouped into knowledge and networks, management and team, infrastructure, finance, and technology. New CO2 utilization ventures encounter challenges to obtain certain expertise such as management and market expertise, and application know-how. They often report to lack access to networks to build-up knowledge and approach key persons for innovation processes in external organizations, so-called gatekeepers (Allen, 1970). Within the management and team category, capabilities such as adaptation abilities for dynamic team settings and changing environments and the management composition were reported by the study participants to hinder successful commercialization.
Furthermore, infrastructure availability and dependence on existing infrastructure cause perceived internal challenges. In this regard, modifying existing equipment or using external facilities to rapidly deploy new technologies was described as greatly beneficial. However, compliance requirements from customers to build-up R&D facilities internally and the access to external infrastructure hinders the development of new CO2 utilization ventures. Moreover, centralized production systems with established infrastructure and the upkeep of an influx of required inputs such as CO2 and energy apply further pressure on these ventures.
Financial barriers are reflected by costs, pricing, and funding. The capital costs for proofs-of-concepts and other testing actions are noticed as very high in the field of CO2 utilization. In addition, new CO2 utilization ventures report to be often incapable of either scaling down at capital expenditure rates that would enable technological applications (see economies of scale) or building the facilities large enough to make the capital expenditure feasible, because raw materials are not available in sufficient quantities. Furthermore, their pricing capabilities are limited and they are unable to compete on under-market (dumping) prices. Funding opportunities and cost or availability of inputs such as CO2 and energy were stated to vary from one geographic location to another (see also Hendriks et al., 2013). Moreover, it is the experience of CO2 utilization ventures that the availability of funding sources is limited and that the exploitation of these sources is also challenging in terms of administration.
The last categories of internal barriers are of technological nature. They are related to application, feasibility, up-scaling, performance and technology development. Some new CO2 utilization ventures in the sample use platform technologies and therefore have a broad range of possible technological applications. This application range was identified by interviewees to bring challenges; research expertise and experience often determine the focus on an initial product rather than marketability or other market drivers. The study participants observed that new CO2 utilization ventures need to focus on a market driven applications whilst ensuring a maximized flexibility to adapt to different CO2 sources such as diluted or concentrated and large or small CO2 sources. Furthermore, when showing the feasibility of their CO2-based products and processes they must comply with time and resource-intensive testing and sampling requirements (e.g., life-cycle assessments, large simulations or pretests under real life conditions) of potential customers. Moreover, to get to a so-called proof-of-concept new CO2 utilization ventures have to up-scale their lab-scale processes over demonstrators to pilot plants. In the light of experiences from the participants, this up-scaling requires extensive engineering expertise and necessary capital.
A poor performance of CO2-based products can hinder a successful commercialization as well. Industry requirements such as the reported high level of reliability of power plant operations must be acknowledged. The willingness to pay a premium for CO2-based products was highlighted as very limited, hence, the interviewees conveyed product performance needs to be superior to be competitive. Developing CO2-based products takes time, especially when involving other stakeholders in the development process, coordination, and feedback loops (e.g., external evaluation rounds). These stages were reported to be time consuming and very challenging.
The third main category of the identified barriers is referred to as cross-linkage barriers (cf. Table 4). Barriers in this category can relate to both, internal and external barriers. They are re-occurring connecting elements within the commercialization process and are grouped into three sub-categories: location, risk, and promoter.
New CO2 utilization ventures face various challenges in terms of the geographic location. The location does not only determine incentives and des-incentives such as regional or national regulation and funding opportunities, but it also feeds into the requirements for partnerships: the proximity to portfolio venture, the possibilities for joint ventures, and the existence of markets for a potential exit depend on the location of a collaboration as study participants have reported.
When it comes to risk, the barriers are ownership and world economics. Owning capital-intensive assets is described to be too risky for most new CO2 utilization ventures, whereas the interviewees stated large industry players often own too many assets to take risks. Furthermore, the perceived risk averseness of their potential customers (and investors) can corner them into additional risk taking via warranty agreements. Global market developments and dynamics such as the economic crisis and the shift of the energy sector toward decentralized energy production were found to create further uncertainties and hinder long time commitments of several stakeholders.
Promoters are individuals that actively and intensively support the innovation process (cf. Witte, 1973) and thus are playing an important role in the commercialization process of CO2 utilization. However, they are most of the times noted to be absent and identifying and convincing gatekeepers at any stakeholder organization was recognized as considerably challenging. New CO2 utilization ventures reported that they face multiple organizational levels approaching large companies and find it difficult to identify key contacts. Hence, not only the identification but also the advocacy for a collaboration represent main barriers for these ventures. However, promoters for CO2 utilization do already exit internally within the founding and management teams of new technology ventures and externally among investors, partners and governments. Examples of this highlighted by the study participants include:
• Founders of new CO2 utilization ventures can have proven entrepreneurial skillsets and vast industry knowledge and networks from previous work experiences to enable the access to investors and potential customers and partner. Moreover, they cannot only bring specific know-how, but also have intrinsic sustainability-oriented motivation from previous experiences and see CO2 as (market) opportunity.
• Effective technology and market knowledge transfer can also be performed by investors and partners. Moreover, investors and universities can provide R&D infrastructure or universities can grant access to extensive IP portfolios and talent pools.
• Manufacturing partners can manufacture to the specific requirements of new CO2 utilization ventures. Existing expertise and equipment allows for a quicker project implementation, bigger scale-up, and more value capture by being able to offer turnkey solutions.
• Government can administer sponsorship for new CO2 utilization ventures. This sponsorship may cover different forms, from R&D funding schemes, to dedicated knowledge transfer and infrastructure programmes.
The study results do not only identify the internal, external and cross-linkage barriers of new CO2 utilization ventures, but also indicate the (perceived) relevance of the barrier categories. Cross-barrier relations were discovered first, by triangulating the reported barriers of new CO2 utilization ventures with the reported barriers of the four industry players (steel producer, utility, infrastructure provider, and chemical company). Second, by comparing two geographic location, representing two different institutional settings: Canada, the USA, and the EEA. Third, by considering ties between internal, external, and cross-linkage barriers. Fourth, by having four categories of success factor (Song et al., 2008) ranked by the new CO2 utilization ventures. Lastly, by comparing the sample on an application class level.
Barriers in the categories customer, partner, technology, government, finance, infrastructure, and strategy were reported the most by both new technology ventures and large incumbents, whereas there was less reporting in the categories society, risk, and knowledge and network. Differences were mainly in the categories investor and competitor, and promoter and team and management. The industry players reported more barriers in the first two categories, while the new ventures reported more in the latter two. Figure 4 gives a graphical overview of this data triangulation by plotting the relative reported barrier categories of the industry players against the relative reported barrier categories of new ventures.
Figure 4. Reported barrier category matrix: new ventures vs. incumbents. *Perceived main barrier reported in category.
Comparing the two regions, the reported barrier categories are primarily similar. However, the categories risk and society were more often reported in the EAA and the categories team and management, investor, competitor, knowledge and network and future employee more often in Canada and the USA (cf. Figure 5). Figure 5 shows the relative reported barrier categories with regard to the two observed regions. Categories that are closer to the ordinate (EEA) than to the abscissa (Canada and the USA) indicate a higher importance/relevance in the EEA and vice versa. The closer a category is to the bisector, the less distinct its importance/relevance for one region is.
Figure 5. Reported barrier category matrix: Canada and USA vs. EEA. *Perceived main barrier reported in category.
The amount of cross-barrier relationships between the three main categories are also depicted in Figures 4 and 5. The external categories customer, investor, government, partner; the internal categories strategy and all resource subcategories; and the cross-linkage categories promoter and location have the most links with ties to more than 50% of the other main categories.
The emphasis on main barriers has been captured by coding terms such as “challenge,” “hurdle,” “issue,” “barrier,” or “problematic” in combination with “main,” “biggest,” or “most.” These perceived main barriers are in the categories partner, customer, investor, government, society, promoter, location, finance, and technology (cf. Tables 2–4). Moreover, the perceived main barrier categories cover those barrier categories that have been reported by all new CO2 utilization ventures in the sample and include those barrier categories that have the most cross-barrier relationships (cf. Figures 4 and 5). However, the perceived main barrier category society is only reported by the minority of new CO2 utilization ventures in the sample and has little relationships to other barrier categories.
Figure 6 reveals the ranking of success factor categories. Thus, market and opportunity are the highest ranked categories followed by team, resources, and strategic and organizational fit. The ranking slightly changes when breaking it down to the two geographic locations. For Canada and the USA, the entrepreneurial opportunity is on the same rank as entrepreneurial team.
Figure 6. Success factor ranking according to Song et al. (2008).
Other comparisons can be made between the different application classes. For this matter the application class CO2 to fuels was further broken down, when a new CO2 utilization venture aimed at technological outlets in CO2 to fuels and CO2 to chemicals. These ventures often pursue platform technologies that allow for the conversion of CO2 to synthetic fuels and other more complex chemicals such as pharmaceuticals. Table 5 shows differences and relations of technology readiness level (TRL) and application classes. CO2 mineralization has the highest technological maturity, on average, followed by CO2 to fuels, and CO2 to chemicals.
Pursuing multiple application classes CO2 to fuels and CO2 to chemicals seem to impact the TRL. In most of these cases the venture has not decided on a business model or initial product yet. This is also depicted in the lowest averaged venture age of 4.8 years in this application class.
On average, the CO2 mineralization class has the highest TRL but less capital has been attracted and fewer employees are working in this field than in the CO2 to fuels class.
Overall, the evaluation via various comparisons of the observed barrier phenomena remains challenging because of the qualitative nature of this study. However, the results at least indicate different levels of relevance. Figures 4–6 and Table 5 thereby provide an effective way to highlight these indications.
For all the identified barrier categories, the interviewees provided several barriers and drivers. However, some categories have been reported more frequently than others and may be contextualized with Song et al.’s (Song et al., 2008) ranking:
Song et al.’s (ibid) market and opportunity factors are mainly determined by the external environment of new CO2 utilization ventures. Hence, the proportion of reported barriers in the external categories as well as the emphasis on them are consistent and indicate the relevance of these categories. Song et al.’s interpretation of entrepreneurial team is best reflected in the internal barrier categories of team and management and knowledge and networks. Both seem especially relevant in Canada and the USA (cf. Figure 4). This fact is also being backed by the higher ranking of entrepreneurial team in this region (cf. Figure 6). Although, the resource-related categories cannot be clearly distinct from Song et al.’s entrepreneurial resources: Whereas factors such as financial resources are clearly related to the financial barrier category, patent protection and supply chain integration are more related to the strategy category. However, both internal categories (finance and strategy) seem to be of high relevance as well as technology and infrastructure. The less prominently ranked strategic and organizational fit would be best connected to the internal size and structure category. Even though cross-linkage barriers cannot being clearly related to Song et al.’s success factors, location and promoter seem to play a mediating role.
A support system in the context of entrepreneurship is defined as a system that “[…] comprises all actors, institutional settings and resources that help entrepreneurs in innovating successfully” (Fichter et al., 2016).
The barriers of new CO2 utilization ventures relate to their stakeholders or actors (perceived main categories: partner, customer, and investor), their internal organization including resources (perceived main categories: finance, strategies, and technology), specific contextual settings such as institutional settings (perceived main categories: government and society), and relational settings (perceived main categories: promoter and location). Hence, the concept of a coordinated, dedicated support systems could be applied to provide effective leverage for a sustainability transformation of industries (Fichter et al., 2013).
The capital intensity is paramount in CO2 utilization technologies. New CO2 utilization ventures need to proof their technology in costly demonstrators and pilot plants. Shared infrastructure and cost-efficient business models have been recognized to be of help in overcoming capital challenges for these kind of technologies (Cleantech Incubation Europe, 2014). New CO2 utilization ventures that invest primarily in people and not in bricks may be better able to adapt an organizational structure that comply with conventional requirements of institutional investor such as investment volumes.
Furthermore, there is a need for an immediate collaboration to enhance knowledge transfer and facilitate learning for new CO2 utilization ventures. Regional collaborative alliances with multiple stakeholder alongside the CO2 value chain from CO2 emission, capture, and utilization to the consumption of CO2-based products are wanted. Entire partnership systems could bring together (local) stakeholder to create specific business opportunities for CO2 utilization.
However, new CO2 utilization ventures also seem to lack the effective coordination of these approaches. Intermediating third parties (cf. van Lente et al., 2003; Altenburg and Pegels, 2012; Kivimaa, 2014) could ensure motivational and strategic alignment of different partners and coordinate the collaboration. Furthermore, these parties could help to overcome the (causality) dilemma where both, potential off-taker and investor, require a proof-of-concept and existing contracts by mediating between the different actors.
New CO2 utilization ventures face challenges when approaching conservative industries with radical solutions. The potentially disruptive nature of these solutions (Christensen, 1997) may be the reason for a restraining behavior of customers, investors, and other partners (cf. Gauthier and Gilomen, 2016).
Large incumbents do not only hesitate when developing sustainability-oriented solutions themselves (cf. Ihlen and Roper, 2014), but also are often reluctant to establish new facilities for a CO2-based production. Building-up a new production can be disruptive for established companies, whereas retrofitting an existing production line is rather sustaining for them.
These different innovation natures may also be represented in the sample: an application class with a higher TRL and, at the same time, fewer capital attracted than another class could indicate that, e.g., capital efficient retrofitting approaches are more prominent in the first class (cf. Table 5: CO2 mineralization vs. CO2 to fuels). However, differences in TRL, attracted capital and number of employees could also be explained by different technology requirements (e.g., exothermic vs. endothermic) or market conditions (e.g., resource availability).
Beside the disruptive or sustaining nature, the degree of the sustainability-orientation of an innovation seems to influence the commercial success of new CO2 utilization ventures. Hockerts and Wüstenhagen (2010) identified two dimensions of entrepreneurial activity that can lead to a sustainability transition of an industry: environmental and social performance, and market share. Hörisch (2015) builds on these dimensions and argues that sustainability-oriented new technology ventures need to master a coordinated interplay between sustainability effect and market impact to meaningfully contribute to sustainability transitions.
However, the findings of this study show that these dimensions tend to have a negative effect upon each other. An optimum CO2 reduction is often not economically viable and market impact is achieved at the expense of the sustainability effect.
Consequently, new CO2 utilization ventures need to initially focus on the product’s performance (economic value creation) rather than on its sole sustainability aspect (ecological and social value creation) to successfully commercialize their products in competitive mass markets. This focus may diminish the strong sustainability-orientation by emphasizing innovation which does not have sustainability as a primary target, but even products or materials with very high sustainability effect could be positioned as more durable, robust, or cheaper (cf. Driessen et al., 2013) to attain greater market impact.
Nevertheless, staged approaches such as a staged market entry strategy (cf. Clay, 2013) could help to target niche markets with a high sustainability effect and move toward mass markets with increased economies of scale and knowledge about industry requirements. Trade-offs between sustainability dimensions may thereby be avoided.
However, some technologies cannot be economically viable on a niche-market scale. That is why an early application focus in consideration of the market circumstances is crucial. Platform technologies with various application options might help to diversify and dynamically adapt to new market developments, but the choice often overburdens new CO2 utilization ventures. Staged project strategies can help to generate initial revenue and get a better understanding of customers by starting with advisory projects on a potential CO2 utilization implementation.
The acknowledgment of regulatory systems and institutional settings are essential for CO2 utilization and shape support systems for entrepreneurship (Fichter et al., 2013). In this work two geographic regions with different institutional settings have been looked at: Canada and the USA and the EEA. However, there were more similarities than differences. Whereas risk averseness and societal acceptance seemed to play a bigger role in the EEA, institutional investors, human capital and knowledge transfer seemed to be more prominent in Canada and the USA.
The public perception of CO2 utilization influence new technology ventures. Even though only the minority of the new CO2 utilization ventures reported barriers in the society category, social acceptance has been recognized as a necessity for a successful commercialization of SOI such as CO2 utilization (Jones et al., 2017). Dedicated marketing strategies enable these ventures to counteract the misperception of CO2 utilization, especially in the EEA (cf. van Heek et al., 2017).
Policy interventions and regulatory pressure might be another way to overcome barriers (Jaffe and Palmer, 1997; Brunnermeier and Cohen, 2003; Parker et al., 2009; Kneller and Manderson, 2012) but represent dependency on rather short-term mechanism. Interventions such as feed-in tariffs for renewable energy technologies affect institutional investors in clean technologies (cf. Bürer and Wüstenhagen, 2009; Ghosh and Nanda, 2010). Due to the larger number of private investments in the clean technology sector in Canada and the USA than in the EEA, a different importance of institutional investors might be attributed to the two institutional settings.
CO2-based products can substitute fossil-based products, however, the technologies for the substitution are often not yet economically viable without evolving production systems or new regulations (Bocken et al., 2014). Some of these CO2 utilization technologies currently need excess energy from renewable energy production. This dependency of current inefficiencies in the distribution systems of renewable energies can lead to a shorten vision as interim solutions.
However, clear responsibilities within existing regulatory frameworks and less complexity could already reduce barriers regardless of the institutional setting and the time horizon.
The cross-linkage barrier categories of promoter and location especially stress the relevance of context and mediation for new CO2 utilization ventures.
Not only proximity aspects when dealing with external stakeholders (for investments: cf. Knight, 2012; for partnerships: cf. Hansen, 2014) and location-dependent (des-)incentives, but also the technological diversity within CO2 utilization call for a dynamic adoption to the specific needs of a new CO2 utilization venture. Hence, tailored support solutions for individual technological and geographic context are needed (see also Fichter et al., 2016).
The promoter model is a recognized concept to overcome innovation barriers (Gemünden et al., 2007). In this work, gatekeepers are key people in any commercialization process of an external organization whereas promoters are key to drive a specific innovation on different levels (Hauschildt and Schewe, 2000).
With regard to the four person promoter model (Gemünden et al., 2007), the identified promoters in CO2 utilization are mainly power and expert promoters. Process and relationship promoters remain mainly unidentified or are missing. This is especially reflected in the challenge to identify and engage with gatekeepers outside the new CO2 utilization venture’s organization.
An intermediating third party could step in to facilitate the matching process and act as relationship promoter (ibid).
The population size was determined by the fact that CO2 utilization is a relatively new and emerging field. The population of new CO2 utilization ventures was identified with little under 50 worldwide and the companies within the sample are on average 6.5 years old (cf. Table 5). The sample size made a full comparison of the application classes challenging and led to a lack of contrasting juxtaposition within the classes.
Additionally, comparisons between different conversion processes such as catalysis, artificial photosynthesis, photocatalysis, and electrochemical reduction (cf. Styring and Jansen, 2011) for the same product and different sustainable business models such as create value from waste or substitute with renewables and natural processes (cf. Bocken et al., 2014) are missing.
Comparisons of top management and operating staff within a single new CO2 utilization venture to view the phenomena from different perspectives (Eisenhardt and Graebner, 2007) were rarely performed, because of the limited amount of knowledgeable people (cf. Palinkas et al., 2015) for this study. From the 23 interviewees, nine had not been with the company since its start, accounting for an average of being 4.8 years with the venture (cf. Table 5). Therefore, internal barriers have been aggregated to the organizational level only.
Although theoretical saturation was reached in the course of this analysis, future research should look at sustainability-oriented organizations in their growth phase in-depth to identify differences in the individual, group and organizational level (cf. Hueske and Guenther, 2015). Such an in-depth analysis could also enrich data on the social dimension of sustainability, that is currently underrepresented due to the predominant focus on the technology development of most of the investigated new technology ventures.
In addition, qualitative comparisons could shed light on the differences in location and output- and process-based categorizations of new CO2 utilization ventures and longitudinal studies on sustainability-oriented ventures could contribute to a better understanding of success and failure factors of strong sustainability-oriented new technology ventures.
Furthermore, case studies on system intermediaries for the sustainability transition and their role in supporting these new ventures could help to further shape effective support mechanism.
This work brings together SE, sustainability transition and barriers to successful commercialize radical SOI. These radical SOI show increased complexity when compared to conventional innovation. Multidimensional (economic, environment, and social) focal points such as the coordinated interplay of sustainability effect and market impact (cf. Hörisch, 2015) or the problem of “double externalities” (cf. Beise and Rennings, 2005) and an extended external orientation toward more diverse stakeholders (including society or partnership ecosystems), e.g., to overcome resource constraints, to bring novel technology to the market or to share risks (cf. Jay and Gerard, 2015) differentiate the commercialization process of strong sustainability-oriented new technology ventures from conventional new technology ventures. Hence, this study supports the notion of describing radical SOI processes by adding sustainability-specific characteristics to conventional innovation processes (cf. Walker et al., 2008; Driessen et al., 2013; Jay and Gerard, 2015).
An internal and external barrier framework (Hueske and Guenther, 2015) has been applied and further developed for strong sustainability-oriented new technology ventures based on a qualitative interview study with 24 new ventures and large incumbents in CO2 utilization. A new cross-linkage barrier category has been added to the main barrier categories to highlight the relevance of connections between internal and external barriers. This category contributes to a better understanding of the importance of context for strong SOI such as CO2 utilization.
Stakeholder synergies and the creation of shared values (cf. Tantalo and Priem, 2016) are experienced throughout the barrier analysis but especially in the partner category where entire stakeholder ecosystems drive the successful commercialization of CO2 utilization. Hence, stakeholder synergies (Tantalo and Priem, 2016) rather than stakeholder theory (Freeman et al., 2007, 2010) could be supported by strong sustainability-oriented new technology ventures for external barriers.
Value-creating strategies through collaboration also occur internally with external interactions (Eisenhardt and Martin, 2000). Organizational learning (cf. Teece et al., 1997) is represented foremost as driver in expertise gain in the knowledge and network category. Thus, dynamic capabilities seem appropriate for internal barriers of strong sustainability-oriented new technology ventures.
Both, synergies and learnings can be facilitated by cross-linking promoters that can reveal gatekeepers and mediate between stakeholder groups (cf. Hauschildt and Schewe, 2000). In combination with the proximity dimension (Knight, 2012; Hansen, 2014) these intermediaries can lay the groundwork for local new venture support systems paving the way for multilevel perspectives on the entire system of strong SOI such as CO2 utilization (cf. Geels, 2011).
Recommendations for a dedicated support system are derived from a commercial barrier analysis of new CO2 utilization ventures in the institutional settings of Canada and the USA, and the EEA. There are four levels of recommendations for strong sustainability-oriented new technology ventures, policy makers, and support providers: on (a) actors, (b) resources, (c) institutional settings, and (d) the coordination of a support system.
a. Strong sustainability-oriented new technology ventures and other actors from the entire value adding path of CO2 utilization (e.g., from CO2 and energy supplier, R&D and upscaling partners to off-taker/customers) should take part in partnership ecosystems that are aligned and facilitated by intermediating third parties to exploit synergies such as increased value capture and knowledge transfer. Policy makers can encourage these ecosystems by providing necessary resources and means to pursue fruitful collaborations.
b. Strong sustainability-oriented new technology ventures should foster their focus on the CO2-based product’s performance, for example, by managing platform technologies and facilitate staged project approaches such as staged market entry strategies.
c. Policy makers should pursue in sponsorship for CO2 utilization such as regulatory frameworks with clear responsibilities and little complexity, for example, to ease access to funding opportunities and encourage internationalization for strong sustainability-oriented new technology ventures.
d. Intermediaries should coordinate support systems, for example, to identify gatekeepers, spark collaboration by aligning strategies and motivations and enable support providers to tailor support solutions to the specific needs of a new CO2 utilization venture in a given environment.
MK: main/single author, main contribution to the conception and execution of the work.
The author declares that the research was conducted in the absence of any commercial or financial relationship that could be construed as a potential conflict of interest.
This work has been accomplished in the frame of the EnCO2re program, funded by the European Institute of Innovation and Technology’s Climate-KIC. The author is particularly grateful to the companies that kindly accepted to participate in this study. Insightful comments and suggestions by the two reviewers and the editor helped to improve an earlier version of this manuscript. Furthermore, the author wants to express a special gratitude to Prof. Dr. Jan Kratzer and Dr. Ingo Michelfelder for their continuous supervision and Arno Zimmermann for his support in the interview process.
This work has been funded by the European Institute of Innovation and Technology (EIT) via the EIT Climate-KIC flagship program “EnCO2re—Enabling CO2 re-use.” The author acknowledges support by the German Research Foundation and the Open Access Publication Funds of Technische Universität Berlin.
Allen, T. J. (1970). Communication networks in R & D laboratories. R&D Manage. 1, 14–21. doi: 10.1111/j.1467-9310.1970.tb01193.x
Altenburg, T., and Pegels, A. (2012). Sustainability-oriented innovation systems – managing the green transformation. Innov. Dev. 2, 5–22. doi:10.1080/2157930X.2012.664037
Aresta, M., Dibenedetto, A., and Angelini, A. (2013). The changing paradigm in CO2 utilization. J. CO2 Util. 3-4, 65–73. doi:10.1016/j.jcou.2013.08.001
Armstrong, K., and Styring, P. (2015). Assessing the potential of utilization and storage strategies for post-combustion CO2 emissions reduction. Front. Energy Res. 3:8. doi:10.3389/fenrg.2015.00008
ATLAS.ti. (2016). ATLAS.ti: The Qualitative Data Analysis & Research Software. Available at: http://atlasti.com/
Beise, M., and Rennings, K. (2005). Lead markets and regulation: a framework for analyzing the international diffusion of environmental innovations. Ecol. Econ. 52, 5–17. doi:10.1016/j.ecolecon.2004.06.007
Bernauer, T., Engel, S., Kammerer, D., and Sejas Nogareda, J. (2007). “Explaining green innovation. Ten years after porter’s win-win proposition: how to study the effects of regulation on corporate environmental innovation?” in Politik und Umwelt, eds K. Jacob, F. Biermann, P.-O. Busch, and P. H. Feindt (Wiesbaden: VS, Verl. für Sozialwiss), 323–341.
Blowfield, M., Visser, W., and Livesey, F. (2008). Sustainability Innovation: Mapping the Territory. UK: Cambridge University Press.
Bocken, N., Short, S. W., Rana, P., and Evans, S. (2014). A literature and practice review to develop sustainable business model archetypes. J. Cleaner Prod. 65, 42–56. doi:10.1016/j.jclepro.2013.11.039
Boons, F., and Lüdeke-Freund, F. (2013). Business models for sustainable innovation: state-of-the-art and steps towards a research agenda. J. Cleaner Prod. 45, 9–19. doi:10.1016/j.jclepro.2012.07.007
Bossink, B. A. G. (2014). Demonstration projects for diffusion of clean technological innovation: a review. Clean Technol. Environ. Policy 17, 1409–1427. doi:10.1007/s10098-014-0879-4
Brunnermeier, S. B., and Cohen, M. A. (2003). Determinants of environmental innovation in US manufacturing industries. J. Environ. Econ. Manage. 45, 278–293. doi:10.1016/S0095-0696(02)00058-X
Bürer, M. J., and Wüstenhagen, R. (2009). Which renewable energy policy is a venture capitalist’s best friend? Empirical evidence from a survey of international cleantech investors. Energy Policy 37, 4997–5006. doi:10.1016/j.enpol.2009.06.071
Bygrave, W. D., Lange, J., Marram, E., Brown, D., and Marquis, J. (2014). No financial cleanup: a study of venture capital returns on cleantech IPOs. SSRN J. doi:10.2139/ssrn.2412959
Christensen, C. (1997). The Innovator’s Dilemma: When New Technologies Cause Great Firms to Fail. Boston, USA: Harvard Business Review Press.
Clay, T. A. (2013). New venture: a new model for clean energy innovation. J. Appl. Corporate Finance 25, 56–61. doi:10.1111/jacf.12029
Cleantech Incubation Europe. (2014). Cleantech Incubation Policy and Practice: Recipes for Creating Cleantech Incubator Hotspos in Europe. Delft, Helsinki, Budapest, Paris, Turin, Peterborough: Cleantech Incubation Europe (CIE). Available at: http://cleantechincubation.eu/wp-content/uploads/2012/07/Cleantech-Incubation-Practice-and-Practice-Handbook.-June-2014.pdf
Cohen, B., and Winn, M. I. (2007). Market imperfections, opportunity and sustainable entrepreneurship. J. Bus. Venturing 22, 29–49. doi:10.1016/j.jbusvent.2004.12.001
Driessen, P. H., Hillebrand, B., Kok, R. A. W., and Verhallen, T. M. M. (2013). Green new product development: the pivotal role of product greenness. IEEE Trans. Eng. Manage. 60, 315–326. doi:10.1109/TEM.2013.2246792
Eisenhardt, K. M. (1989). Building theories from case study research. Acad. Manage. Rev. 14, 532–550. doi:10.2307/258557
Eisenhardt, K. M., and Graebner, M. E. (2007). Theory building from cases: opportunities and challenges. Acad. Manage. J. 50, 25–32. doi:10.5465/AMJ.2007.24160888
Eisenhardt, K. M., and Martin, J. A. (2000). Dynamic capabilities: what are they? Strateg. Manage. J. 21, 1105–1121. doi:10.1002/1097-0266(200010/11)21:10/11<1105::AID-SMJ133>3.0.CO;2-E
Elkington, J. (1998). Cannibals with forks: the triple bottom line of 21st-century business. Environ. Qual. Manage. 8, 37–51. doi:10.1002/tqem.3310080106
Fichter, K., Fuad-Luke, A., Hjelm, O., Klofsten, M., Backman, M., Bergset, L., et al. (2016). Redesigning the Support System for Innovation and Entrepreneurship: Work Package 8: Paradigm Shift. Berlin, Helsinki, Linköping: SHIFT.
Fichter, K., Fuad-Luke, A., Klofsten, M., Bergset, L., Bienkowska, D., Clausen, J., et al. (2013). Support Systems for Sustainable Entrepreneurship and Transformation (SHIFT): Work Package 1: Theoretical Foundation. Berlin, Linköping, Aalto: SHIFT.
Freeman, R. E., Harrison, J. S., and Wicks, A. C. (2007). Managing for Stakeholders: Survival, Reputation, and Success. New Haven, CT: Yale University Press.
Freeman, R. E., Harrison, J. S., Wicks, A. C., Parmar, B. L., and de Colle, S. (2010). Stakeholder Theory: The State of the Art. Cambridge, UK: Cambridge University Press.
G7 Germany. (2015). G7: Leaders’ Declaration G7 Summit: Think Ahead. Act Together. Germany: Schloss Elmau.
Gauthier, C., and Gilomen, B. (2016). Business models for sustainability: energy efficiency in urban districts. Organ. Environ. 29, 124–144. doi:10.1177/1086026615592931
Geels, F. W. (2011). The multi-level perspective on sustainability transitions: responses to seven criticisms. Environ. Innov. Soc. Transitions 1, 24–40. doi:10.1016/j.eist.2011.02.002
Gemünden, H. G., Salomo, S., and Hölzle, K. (2007). Role models for radical innovations in times of open innovation. Creativity & Inn Man 16, 408–421. doi:10.1111/j.1467-8691.2007.00451.x
Ghosh, S., and Nanda, R. (2010). Venture Capital Investment in the Clean Energy Sector. Harvard Business School Entrepreneurial Management Working Paper No. 11-020. Available at: https://ssrn.com/abstract=1669445
Grubb, M. (2004). Technology innovation and climate change policy: an overview of issues and options. Keio. Econ. Stud. 41, 103–132.
Hahn, R., and Ince, I. (2016). Constituents and characteristics of hybrid businesses: a qualitative, empirical framework. J. Small Bus. Manag. 54, 33–52. doi:10.1111/jsbm.12295
Hall, J., and Clark, W. W. (2003). Special issue: environmental innovation. J. Cleaner Prod. 11, 343–346. doi:10.1016/S0959-6526(02)00070-7
Hall, J. K., Daneke, G. A., and Lenox, M. J. (2010). Sustainable development and entrepreneurship: past contributions and future directions. J. Bus. Venturing 25, 439–448. doi:10.1016/j.jbusvent.2010.01.002
Hansen, T. (2014). Juggling with proximity and distance: collaborative innovation projects in the Danish cleantech industry. Econ. Geogr. 90, 375–402. doi:10.1111/ecge.12057
Hauschildt, J., and Schewe, G. (2000). Gatekeeper and process promotor: key persons in agile and innovative organizations. Int. J. Agile Manage. Syst. 2, 96–103. doi:10.1108/14654650010312624
Hendriks, C., Noothout, P., Zakkour, P., and Cook, G. (2013). Implications of the Reuse of Captured CO2 for European Climate Action Policies. Utrecht: ECOFYS Netherlands B.V. Available at: http://www.scotproject.org/sites/default/files/Carbon%20Count,%20Ecofys%20(2013)%20Implications%20of%20the%20reuse%20of%20captured%20CO2%20-%20report.pdf
Hockerts, K., and Wüstenhagen, R. (2010). Greening Goliaths versus emerging Davids—theorizing about the role of incumbents and new entrants in sustainable entrepreneurship. J. Bus. Venturing 25, 481–492. doi:10.1016/j.jbusvent.2009.07.005
Hope, C., Gilding, P., and Alvarez, J. (2015). Quantifying the Implicit Climate Subsidy Received by Leading Fossil Fuel Companies. Cambridge Judge Business School Working Papers. Working Paper No. 02/2015. Available at: https://www.jbs.cam.ac.uk/fileadmin/user_upload/research/workingpapers/wp1502.pdf
Hörisch, J. (2015). The role of sustainable entrepreneurship in sustainability transitions: a conceptual synthesis against the background of the multi-level perspective. Adm. Sci. 5, 286–300. doi:10.3390/admsci5040286
Hueske, A.-K., and Guenther, E. (2015). What hampers innovation? External stakeholders, the organization, groups and individuals: a systematic review of empirical barrier research. Manage. Rev. Q 65, 113–148. doi:10.1007/s11301-014-0109-5
Ihlen, Ø, and Roper, J. (2014). Corporate reports on sustainability and sustainable development: ‘we have arrived’. Sust. Dev. 22, 42–51. doi:10.1002/sd.524
Jaffe, A. B., and Palmer, K. (1997). Environmental regulation and innovation: a panel data study. Rev. Econ. Stat. 79, 610–619. doi:10.1162/003465397557196
Jay, J., and Gerard, M. (2015). Accelerating the Theory and Practice of Sustainability-Oriented Innovation. MIT Sloan. Research Paper No. 5148-15. Available at: https://ssrn.com/abstract=2629683
Jones, C. R., Olfe-Kräutlein, B., Naims, H., and Armstrong, K. (2017). The social acceptance of carbon dioxide utilisation: a review and research agenda. Front. Energy Res. 5:11. doi:10.3389/fenrg.2017.00011
Kennedy, S., Whiteman, G., and van den Ende, J. (2013). Presented at the Sustainability and the Corporation: Big Ideas Conference. Harvard Business School. Available at: http://www.hbs.edu/faculty/conferences/2013-sustainability-and-corporation/Documents/Enhancing_radical_innovation_using_sustainability_as_a_strategic_choice.pdf
Kivimaa, P. (2014). Government-affiliated intermediary organisations as actors in system-level transitions. Res. Policy 43, 1370–1380. doi:10.1016/j.respol.2014.02.007
Klewitz, J., and Hansen, E. G. (2014). Sustainability-oriented innovation of SMEs: a systematic review. J. Cleaner Prod. 65, 57–75. doi:10.1016/j.jclepro.2013.07.017
Kneller, R., and Manderson, E. (2012). Environmental regulations and innovation activity in UK manufacturing industries. Resour. Energy Econ. 34, 211–235. doi:10.1016/j.reseneeco.2011.12.001
Knight, E. (2012). The economic geography of financing clean energy technologies. Competit. Change 16, 77–90. doi:10.1179/1024529412Z.0000000009
Matus, K. J., Xiao, X., and Zimmerman, J. B. (2012). Green chemistry and green engineering in China: drivers, policies and barriers to innovation. J. Cleaner Prod. 32, 193–203. doi:10.1016/j.jclepro.2012.03.033
Muñoz, P., and Dimov, D. (2015). The call of the whole in understanding the development of sustainable ventures. J. Bus. Venturing 30, 632–654. doi:10.1016/j.jbusvent.2014.07.012
Murphy, L. M., and Edwards, P. L. (2003). Bridging the Valley of Death: Transitioning from Public to Private Sector Financing. Golden, CO: National Renewable Energy Laboratory.
Naims, H. (2016). Economics of carbon dioxide capture and utilization – a supply and demand perspective. Environ. Sci. Pollut. Res. Int. 23, 22226–22241. doi:10.1007/s11356-016-6810-2
Oakey, R. (2007). Clustering and the R&D management of high-technology small firms: in theory and practice. R&D Manage. 37, 237–248. doi:10.1111/j.1467-9310.2007.00472.x
Pacheco, D. F., Dean, T. J., and Payne, D. S. (2010). Escaping the green prison: entrepreneurship and the creation of opportunities for sustainable development. J. Bus. Venturing 25, 464–480. doi:10.1016/j.jbusvent.2009.07.006
Paech, N. (2007). “Directional certainty in sustainability-oriented innovation management,” in Innovations Towards Sustainability: Conditions and Consequences, ed. M. Lehmann-Waffenschmidt (Heidelberg: Physica-Verlag HD), 121–139.
Palinkas, L. A., Horwitz, S. M., Green, C. A., Wisdom, J. P., Duan, N., and Hoagwood, K. (2015). Purposeful sampling for qualitative data collection and analysis in mixed method implementation research. Adm. Policy Ment. Health 42, 533–544. doi:10.1007/s10488-013-0528-y
Parker, C. M., Redmond, J., and Simpson, M. (2009). A review of interventions to encourage SMEs to make environmental improvements. Environ. Plann. C Gov. Policy 27, 279–301. doi:10.1068/c0859b
Parker, S. C. (2011). Intrapreneurship or entrepreneurship? J. Bus. Venturing 26, 19–34. doi:10.1016/j.jbusvent.2009.07.003
Parrish, B. D. (2010). Sustainability-driven entrepreneurship: principles of organization design. J. Bus. Venturing 25, 510–523. doi:10.1016/j.jbusvent.w2009.05.005
Patton, M. Q. (2015). Qualitative Evaluation and Research Methods: Integrating Theory and Practice. Los Angeles, CA: SAGE.
Pellikka, J. (2014). “The commercialization process of innovation in small high-technology firms – theoretical review,” in Handbook of Research on Techno-Entrepreneurship, 2nd Edn, ed. F. Thérin (Cheltenham, UK; Northampton, MA: Edward Elgar Publishing), 91–109.
Peters, M., Köhler, B., Kuckshinrichs, W., Leitner, W., Markewitz, P., and Müller, T. E. (2011). Chemical technologies for exploiting and recycling carbon dioxide into the value chain. ChemSusChem 4, 1216–1240. doi:10.1002/cssc.201000447
Pinkse, J., and Dommisse, M. (2009). Overcoming barriers to sustainability: an explanation of residential builders’ reluctance to adopt clean technologies. Bus. Strategy Environ. 18, 515–527. doi:10.1002/bse.615
Pinkse, J., and Groot, K. (2015). Sustainable entrepreneurship and corporate political activity: overcoming market barriers in the clean energy sector. Entrepreneurship Theory Pract. 39, 633–654. doi:10.1111/etap.12055
Rai, V., Funkhouser, E., Udwin, T., and Livingston, D. (2015). Venture capital in clean energy innovation finance: insights from the U.S. market during 2005-2014. SSRN J. doi:10.2139/ssrn.2676216
Roome, N. (2012). “Looking back, thinking forward: distinguishing between weak and strong sustainability,” in The Oxford Handbook of Business and the Natural Environment, eds P. Bansal, and A. J. Hoffman (Oxford, England: Oxford University Press), 620–629.
Schaefer, K., Corner, P. D., and Kearins, K. (2015). Social, environmental and sustainable entrepreneurship research: what is needed for sustainability-as-flourishing? Organ. Environ. 28, 394–413. doi:10.1177/1086026615621111
Schaltegger, S., and Wagner, M. (2011). Sustainable entrepreneurship and sustainability innovation: categories and interactions. Bus. Strategy Environ. 20, 222–237. doi:10.1002/bse.682
Silverman, D. (2013). Doing Qualitative Research: A Practical Handbook. 4th Edn. London, UK: SAGE Publications Limited.
Smit, B., Park, A.-H. A., and Gadikota, G. (2014). The grand challenges in carbon capture, utilization, and storage. Front. Energy Res. 2:55. doi:10.3389/fenrg.2014.00055
Song, M., Podoynitsyna, K., Van Der Bij, H., and Halman, J. I. M. (2008). Success factors in new ventures: a meta-analysis*. J. Prod. Innov. Manage. 25, 7–27. doi:10.1111/j.1540-5885.2007.00280.x
Strauss, A. L., and Corbin, J. M. (2015). Basics of Qualitative Research: Techniques and Procedures for Developing Grounded Theory. 4th Edn. Thousand Oaks, CA: SAGE publications.
Styring, P., and Jansen, D. (2011). Carbon Capture and Utilisation in the Green Economy: Using CO2 to Manufacture Fuel, Chemicals and Materials. With assistance of H. de Coninck, H. Reith, K. Armstrong Sheffield, UK: CO2Chem Publishing.
Styring, P., Quadrelli, E. A., and Armstrong, K. (2015). “Preface,” in Carbon Dioxide Utilisation, eds P. Styring, E. A. Quadrelli, and K. Armstrong (Amsterdam: Elsevier), 15–24.
Tantalo, C., and Priem, R. L. (2016). Value creation through stakeholder synergy. Strateg. Manage. J. 37, 314–329. doi:10.1002/smj.2337
Teece, D. J., Pisano, G., and Shuen, A. (1997). Dynamic capabilities and strategic management. Strateg. Manage. J. 18, 509–533. doi:10.1002/(SICI)1097-0266(199708)18:7<509::AID-SMJ882>3.0.CO;2-Z
Thompson, N., Kiefer, K., and York, J. G. (2011). “Distinctions not dichotomies: exploring social, sustainable, and environmental entrepreneurship,” in Advances in Entrepreneurship, Firm Emergence and Growth, eds G. T. Lumpkin, and J. A. Katz. Social and Sustainable Entrepreneurship, Vol. 13 (Bingley, UK: Emerald Group Publishing Limited), 201–229.
Tietjen, O., Pahle, M., and Fuss, S. (2016). Investment risks in power generation: a comparison of fossil fuel and renewable energy dominated markets. Energy Econ. 58, 174–185. doi:10.1016/j.eneco.2016.07.005
UNFCCC. (2015). “Adoption of the Paris Agreement,” in 21st Conference of the Parties. Paris, France: United Nations Framework Convention on Climate Change.
van Heek, J., Arning, K., and Ziefle, M. (2017). Reduce, reuse, recycle: acceptance of CO2 – utilization for plastic products. Energy Policy 105, 53–66. doi:10.1016/j.enpol.2017.02.016
van Lente, H., Hekkert, M., Smits, R., and van Waveren, B. (2003). Roles of systemic intermediaries in transition processes. Int. J. Innov. Manage. 07, 247–279. doi:10.1142/S1363919603000817
Walker, B., Redmond, J., Sheridan, L., Wang, C., and Goeft, M. U. (2008). Small and Medium Enterprises and the Environment: Barriers, Drivers, Innovation and Best Practice. A Review of the Literature. Perth, Western Australia: Edith Cowan University.
Witte, E. (1973). Organisation für Innovationsentscheidungen: Das Promotoren-Modell. Göttingen: O. Schwartz.
Keywords: sustainable entrepreneurship, sustainability transition, barriers to commercial success, CO2 utilization, new technology venture, commercialization, sustainability-oriented innovation, support system
Citation: Kant M (2017) Overcoming Barriers to Successfully Commercializing Carbon Dioxide Utilization. Front. Energy Res. 5:22. doi: 10.3389/fenrg.2017.00022
Received: 30 March 2017; Accepted: 23 August 2017;
Published: 13 September 2017
Edited by:
Katy Armstrong, University of Sheffield, United KingdomReviewed by:
Meng Lu, The Commonwealth Scientific and Industrial Research Organization, AustraliaCopyright: © 2017 Kant. This is an open-access article distributed under the terms of the Creative Commons Attribution License (CC BY). The use, distribution or reproduction in other forums is permitted, provided the original author(s) or licensor are credited and that the original publication in this journal is cited, in accordance with accepted academic practice. No use, distribution or reproduction is permitted which does not comply with these terms.
*Correspondence: Marvin Kant, bWFydmluLmthbnRAdHUtYmVybGluLmRl
Disclaimer: All claims expressed in this article are solely those of the authors and do not necessarily represent those of their affiliated organizations, or those of the publisher, the editors and the reviewers. Any product that may be evaluated in this article or claim that may be made by its manufacturer is not guaranteed or endorsed by the publisher.
Research integrity at Frontiers
Learn more about the work of our research integrity team to safeguard the quality of each article we publish.