- 1Institute of Batteries, Astana, Kazakhstan
- 2Nazarbayev University Research and Innovation System, Astana, Kazakhstan
- 3Nazarbayev University, Astana, Kazakhstan
Although sulfur has a high theoretical gravimetric capacity, 1672 mAh/g, its insulating nature requires a large amount of conducting additives: this tends to result in a low mass-loading of active material (sulfur), and thereby, a lower capacity than expected. Therefore, an optimal choice of conducting agents and of the method for sulfur/conducting-agent integration is critically important. In this paper, we report that the areal capacity of 4.9 mAh/cm2 was achieved at sulfur mass loading of 4.1 mg/cm2 by casting sulfur/polyacrylonitrile/ketjenblack (S/PAN/KB) cathode composite into carbon fiber paper. This is the highest value among published/reported ones even though it does not contain expensive nanosized carbon materials such as carbon nanotubes, graphene, or graphene derivatives, and competitive enough with the conventional LiCoO2-based cathodes (e.g., LiCoO2, <20 mg/cm2 corresponding to <2.8 mAh/cm2). Furthermore, the combination of sulfur/PAN-based composite and PAN-based carbon fiber paper enabled the sulfur-based composite to be used even in carbonate-based electrolyte solution that many lithium/sulfur battery researchers avoid the use of it because of severer irreversible active material loss than in electrolyte solutions without carbonate-based solutions, and even at the highest mass-loading ever reported (the more sulfur is loaded, the more decomposed sulfides deposit at an anode surface).
Introduction
Although sulfur is considered one of the promising candidate materials for the next generation lithium-ion batteries, lithium/sulfur (Li/S) batteries because of its high theoretical gravimetric capacity, 1672 mAh/g (LiCoO2 has a theoretical capacity of 274 mAh/g; however, it can practically offer only 140 mAh/g), and its extremely low cost, USD150/t (LiCoO2 typically costs USD40/kg), there are several challenging issues in order to realize Li/S batteries.
First, since elemental sulfur is a very insulating material, sulfur-based cathodes need a large amount of conducting additives (usually more than 50 wt. %) such as conducting polymer [e.g., polypyrrole (PPY) (Zhang et al., 2013a,b,c), cyclized polyacrylonitrile (PAN) (Zhang et al., 2013d,e; Li et al., 2014), etc.], and conducting carbon materials [e.g., acetylene black (AB), carbon nanotube (CNT), graphene etc.]; therefore, practically (=economically and technically), optimal choice of conducting agents and of the method for sulfur/conducting-agent integration is critically important in order to achieve a high gravimetric capacity and a high mass-loading of active materials. Although the theoretical gravimetric capacity of sulfur is 1672 mAh/g, the addition of 50 wt. % of a conducting agent and a binder, etc. can decrease the realistic capacity of the cathode composite down to 836 mAh/g or less. In addition, the mass-loading of sulfur-based composites tends to be 2–4 mg/cm2 corresponding to the capacity of 1.67–3.34 mAh/cm2 [in Zhang et al. (2013a,b,c,d,e), 0.63–1.7 mAh/cm2 after the initial gradual capacity fading], it does not give us any great merits compared to conventional ones (e.g., LiCoO2, <20 mg/cm2, <2.8 mAh/cm2). A S/PAN/reduced graphene oxide (RGO) composite shows the mass-loading of 7.0 mg/cm2 (sulfur, 2.5 mg/cm2) (coated onto Ni foams) and offers the capacity of 3.2 mAh/cm2 (Li et al., 2014); however, the stable and wide supply of graphene or graphene derivatives at a cheap price have not yet achieved (typically USD4,000/kg or higher, corresponding to the cathode composite cost increase of USD120/kg when assuming 3 wt. % of graphene in the cathode composites). Hopefully, a sulfur-based cathode capacity of 3–6 mAh/cm2 should be achieved at a cost of USD20–40/kg or less.
Second, lithium polysulfides, reduced forms of sulfur, dissolve into an electrolyte solution, resulting in capacity fading; therefore, the stabilization of polysulfides is imperative. So far, S/PAN composites show relatively stable cycle life performance even in LiPF6/carbonate-based electrolyte solutions (note that any other composites cannot be used in LiPF6/carbonate-based electrolyte solutions because of irreversible polysulfides-carbonate reactions) (Wang et al., 2002, 2003; Yu et al., 2004; Fanous et al., 2011; Doan et al., 2013, 2014; Zhang et al., 2013d,e; Konarov et al., 2014; Li et al., 2014; Zhang, 2014): it has been explained that the nanoscopic (or sub-nanoscopic) distribution of sulfur/polysulfides in cyclized PAN contributes to the polysulfide stabilization (Wang et al., 2002, 2003; Yu et al., 2004; Fanous et al., 2011; Doan et al., 2013; Zhang, 2014). S/PAN binary composites (Wang et al., 2002) or S/PAN/conducting carbon ternary composites (Wang et al., 2003) were first suggested by Wang et al. Recently, S/PAN composites with nanosized additives, such as S/PAN/nanosized Mg0.6Ni0.4O (MNO) (Zhang et al., 2013d), S/PAN/graphene (Zhang et al., 2013e), and S/PAN/reduced graphene oxide (RGO) (Li et al., 2014), were intensively investigated by the research groups of University of Waterloo (Canada) and Nazarbayev University (Kazakhstan), and it is likely that the use of nanosized filler (either conducting or non-conducting; conducting ones tend to show better rate performance, of course) can contribute to a better cycle life performance than the composites without nanosized fillers probably owing to the more homogeneous sulfur distribution. However, as mentioned earlier, this challenging issue must also be solved in an economically reasonable manner.
Regarding the high mass-loading of S/PAN-based composites, Konarov et al. (2014) reported that S/PAN offers 2.7 mAh/cm2 at a cathode mass-loading of 7.0 mg/cm2 (sulfur, 2.7 mg/cm2) when coated onto Ni foams; however, Ni foams are too heavy as current collectors (much heavier than Al foils). Note that even Al foils are the dominant contributor on the total electrode mass (e.g., a 15-μm-thick Al foil weighs 56 mg/cm2). Doan et al. (2014) tried to increase the mass-loading of S/PAN/MNO/carbon black composite coated onto Al foils: it was likely that the cycle life performance was not a problem at a sulfur mass-loading of 3.1 mg/cm2 or less (capacity, 1.86 mAh/cm2 or less), but severely deteriorated at 5.2 mg/cm2 or higher sulfur mass-loading. Regarding current collectors, Zhang et al. (2014) tested carbon-fiber-cloth current collectors, and found that the electrochemical performance of sulfur-based cathode was improved compared to the cathode coated onto/into Ni foams. Note that the mass-loading of cathode composites in a carbon fiber cloth can be much greater than that in a Ni foam since the pore volume of the former (0.35 cm3/g) is higher than that of the latter (0.02 cm3/g), although Zhang et al. (2014) did not disclose the mass-loading of the cathode composites. Carbon-fiber-based current collectors can be lighter than Al foils and Ni foams (note that a carbon fiber cloth is heavier than any other current collectors just because it is thick, e.g., millimeter level), can accommodate larger amount of cathode composites than Ni foams, and offer better electrochemical performance than Al foils and Ni foams; therefore, they can be promising candidates as current collectors. The cost of carbon fiber cloth is about USD15/m2 that is less expensive than that of Ni foam, USD30/m2. Carbon fiber papers can be much lighter (e.g., 190 μm thick, 1 mg/cm2) at an adequate thickness for sulfur-based cathodes, and much cheaper than carbon fiber cloth because of its simpler manufacturing process than carbon fiber cloth, although small-scale sample transactions tend to cost a lot (e.g., USD3100/m2, Toray Carbon Paper, TGP-H-60). Thus, we chose carbon fiber papers as current collectors.
In this paper, we show the progress on the increase in sulfur mass-loading and on the polysulfide stabilization.
Experimental
Materials
Sulfur (S) (Sigma-Aldrich, 100-mesh particle size powder), polyacrylonitrile (PAN) (Sigma-Aldrich), and ketjenblack (KB) (Ketjen Black International Co.) were manually mixed in the weight ratio of 80:19.7:0.3 for 2 min (Konarov et al., 2014). The mixture was heat treated at 350°C for 6 h in a tubular furnace in Ar gas to melt sulfur and react it with PAN.
Material Characterization
The sulfur content (47 wt. %) in S/PAN/KB was determined using an element analyzer (Vario Micro Cube, Elementar).
Electrochemical Characterization
Composite cathodes were prepared by mixing 80 wt. % of S/PAN/KB composite, 10 wt. % of AB (MTI Co.), and 10 wt. % of polyvinilydenefluoride (PVdF) (MTI Co.). All components were dispersed in N-methyl-2-pyrrolidone (NMP): the solid/NMP weight ratio was 1:2. The resulting slurry was filled into stacked carbon fiber papers (Alfa Aesar Co.) (each carbon fiber paper was 100 μm thick; the number of stacked carbon fiber papers was varied depending on the mass-loading of the cathode composite) by using a squeegee, vacuum dried at 60°C for 12 h, and cut into disks of 15 mm diameter. The cathode composite mass-loading was varied ranging from 7.7 to 11 mg/cm2 (sulfur, 2.9–4.1 mg/cm2). The electrodes were pressed in order to decrease the thicknesses down to half by using a roll-press (Hohsen Corp.) and thereby to achieve good contacting between the active material and the current collector. Coin type cells (CR2032) were assembled in a high-purity argon (99.9995% purity) filled gloved box (M. Braun Inertgas-Systeme GmbH), using lithium metal disks as anode electrodes, porous polypropylene membranes (Celgard, USA) as separator films, and 1 M LiPF6 solution in ethylene carbonate/ethylmethyl carbonate/dimethylene carbonate (EC/EMC/DMC, volume ratio = 1:1:1) as a liquid electrolyte. The cells were discharged and charged galvanostatically in a computer controlled battery tester (BT-2000, Arbin Instruments) at C-rates ranging from 0.2 to 2 C (1 C = 1672 mAh/g) between 1 and 3 V vs. Li+/Li at 25°C.
Results
Figure 1 shows voltage profiles of the first to third cycles at 0.2 C of coin cells that comprise the S/PAN/KB-based cathodes with (Figure 1A) a cathode composite mass-loading of 11 mg/cm2 (sulfur, 4.1 mg/cm2) and (Figure 1B) a cathode composite mass-loading of 7.7 mg/cm2 (sulfur, 2.9 mg/cm2) that were cast into carbon fiber papers and lithium foils as anodes. As shown in Figure 1, the double-plateau profiles disappeared after the first lithiation: this is due to the nanoscopic redistribution of sulfur/polysulfides that is a well-known phenomenon among S/PAN-based composites (Wang et al., 2002; Yu et al., 2004; Fanous et al., 2011; Doan et al., 2013; Zhang, 2014).
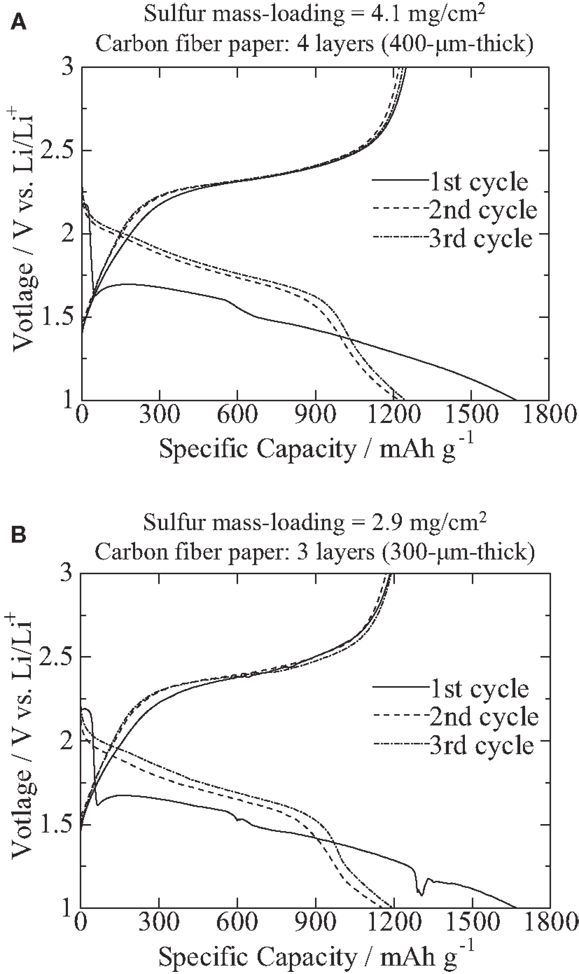
Figure 1. Voltage profiles of the first to the third cycles at 0.2 C. Specimens were composed of the S/PAN/KB-based cathodes with (A) a cathode composite mass-loading of 11 mg/cm2 (sulfur, 4.1 mg/cm2; carbon fiber paper, 400 μm thick) and (B) a cathode composite mass-loading of 7.7 mg/cm2 (sulfur, 2.9 mg/cm2; carbon fiber paper, 300 μm thick) that were cast into carbon fiber papers, and lithium foils as anodes.
Figures 2 and 3 show C-rate performance: as shown in Figure 2, it looks C-rate performance is not good particularly at 2.0°C. However, as shown in Figure 3, when an additional carbon fiber paper is inserted between a current-collecting coin cell casing (cathode side) and the cathode in which the cathode composite is cast into carbon fiber papers, C-rate is improved. Therefore, it is the matter of the contact resistance between the cathodes and current-collection casings of coin cells, and it is easily improved. As shown in Figures 2 and 3, those cathodes can offer the specific gravimetric capacity of about 1200 mAh/g without deteriorating after high C-rate tests even at high mass-loadings such as a cathode composite mass-loading of 11 mg/cm2 (sulfur, 4.1 mg/cm2) and a cathode composite mass-loading of 7.7 mg/cm2 (sulfur, 2.9 mg/cm2), offering 4.9 and 3.5 mAh/cm2, respectively. These values have reached a competitive level with the conventional LiCoO2 (2.5–2.8 mAh/cm2) although the output voltage per cell of the sulfur-based battery can be 55% of the conventional LiCoO2-based ones.
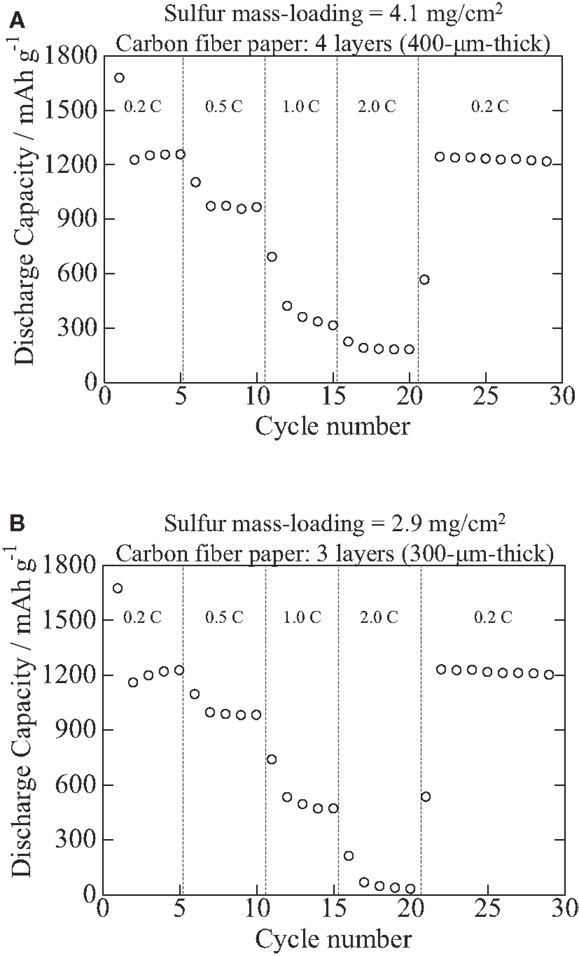
Figure 2. C-rate performance of specimens comprised the S/PAN/KB-based cathodes with (A) a cathode composite mass-loading of 11 mg/cm2 (sulfur, 4.1 mg/cm2; carbon fiber paper, 400 μm thick) and (B) a cathode composite mass-loading of 7.7 mg/cm2 (sulfur, 2.9 mg/cm2; carbon fiber paper, 300 μm thick) that were cast into carbon fiber papers, and lithium foils as anodes.
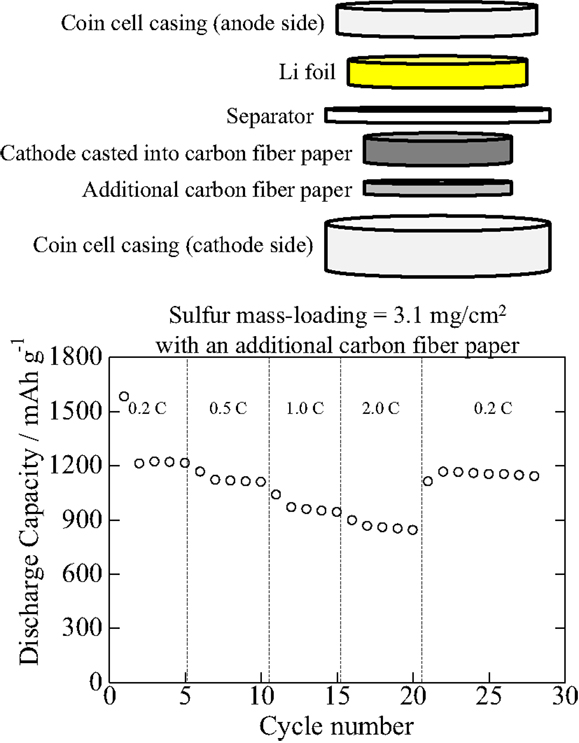
Figure 3. C-rate performance of the specimen comprised the S/PAN/KB-based cathodes with a cathode composite mass-loading of 8.1 mg/cm2 (sulfur, 3.1 mg/cm2; carbon fiber paper, 300 μm thick) that was cast into carbon fiber papers, and lithium foils as anodes. A 100-μm-thick additional carbon fiber paper was inserted between a current-collecting coin cell casing (cathode side) and the cathode.
Figures 4 shows cycle performance: as shown in Figure 4, all the specimens offer more than 900 mAh/g of capacitance up to around 70th cycle. Considering the use of a LiPF6/carbonate-based electrolyte solution and of the highest mass-loading of sulfur ever reported, the results are not so bad compared with ever reported ones; however, at least 2000 cycles or more cycle life will be required for practical applications. Many lithium/sulfur battery researchers avoid the use of carbonate-based electrolyte solutions because of severer irreversible active material loss than in electrolyte solutions that does not contain carbonate-based solutions (e.g., polyether). Note that the more sulfur is loaded, the more decomposed sulfides deposit (irreversible active material loss) at an anode surface. Although we expected the cycle life to be further improved by the combination of sulfur/PAN-based composite and carbon fiber paper, which is also synthesized from PAN, we have not reached the target cycle life (80% capacity retention at 2000th cycle). Extended cycle life will be investigated in the near future by using (1) more practical anodes than Li foils such as pre-lithiated graphite, Hard carbon, various types of nanosized silicon/carbon composites, etc. that have more active surface areas than Li foils, (2) polysulfide trap layers that may be formed at the surface of anode particles or at the surface of separators or at the surface of cathodes, and/or (3) other electrolyte solutions such as lithium bis(trifluoromethane sulfonyl) imide (LiTFSI)/tetraglyme.
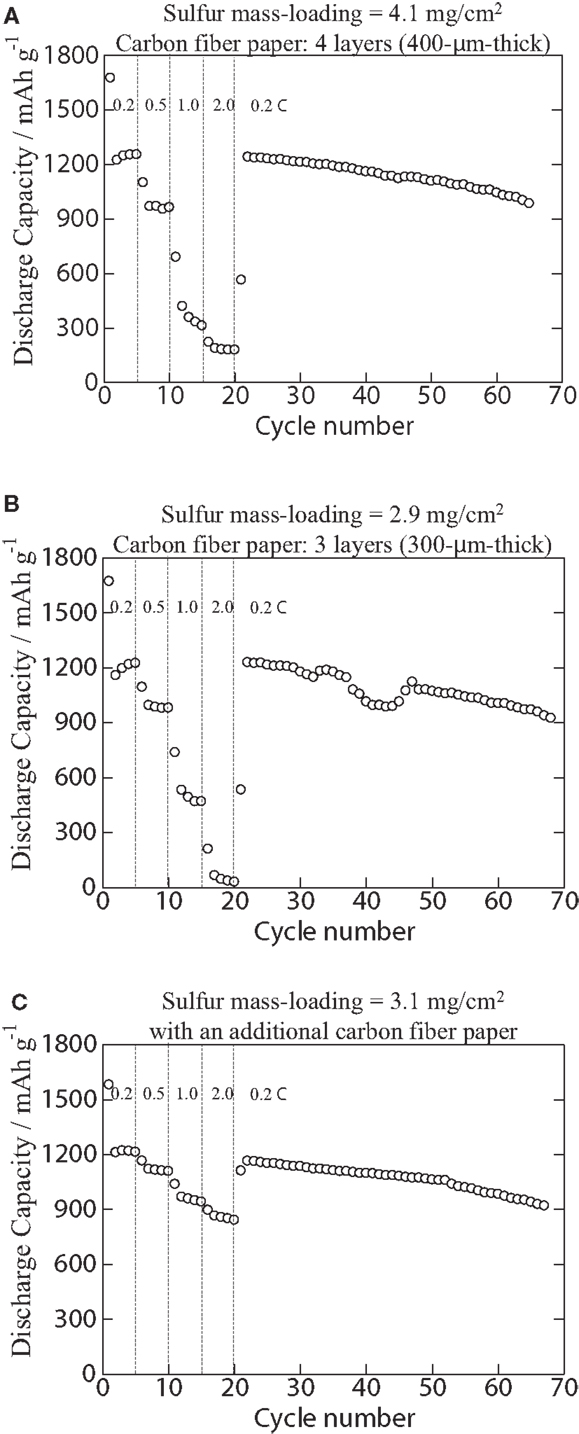
Figure 4. Cycle performance of specimens comprised the S/PAN/KB-based cathodes with (A) a cathode composite mass-loading of 11 mg/cm2 (sulfur, 4.1 mg/cm2; carbon fiber paper, 400 μm thick), (B) a cathode composite mass-loading of 7.7 mg/cm2 (sulfur, 2.9 mg/cm2; carbon fiber paper, 300 μm thick), and (C) a cathode composite mass-loading of 8.1 mg/cm2 (sulfur, 3.1 mg/cm2; carbon fiber paper, 400 μm thick) with a 100-μm-thick additional carbon fiber paper inserted between a current-collecting coin cell casing (cathode side) and the cathode.
Discussion
Another way of increasing the mass-loading of sulfur is to prepare free-standing cathodes without using any current collectors that are main contributors regarding the total mass of electrode, such as binder-free nanosulfur/CNT papers (Sun et al., 2014) (no description on the mass-loading), binder-free nanosulfur/RGO papers (0.9 mAh/cm2) (Wang et al., 2015). Current problems are (1) an electrical contact with a low conductance will be, anyway, required in order to output current/voltage to external load. (note that coin cells do not cause any problems even when free-standing electrodes are used since the largest area of an electrode can contact with a “current-collecting” casings of coin cells); (2) mass-loading tends to be extremely low (2 mg/cm2 or less), resulting in a low capacity per unit area. Sulfur/micro-mesoporous carbon (MC)/Teflon (PTFE)/CNT free-standing sheets (80 μm thick) offer 2.3 mAh/cm2, and the preparation process of the sheets (roll-press) is suitable for mass production (Thieme et al., 2013); however, the free-standing cathode sheet was laminated onto a carbon-coated expanded aluminum current collector in order to reduce contact resistance. Lu et al. (2014) reported that sulfur/graphene oxide (GO) sponges can offer a 12 mg/cm2 of mass-loading and a 4.5 mAh/cm2 or higher capacity at 0.1 C up to 300 cycles; however, the preparation method of the sponge (hydrothermal reduction of GO hydrogel in an autoclave) is problematic for the mass production of electrodes.
So far, it seems that the cathode composite slurry coating onto a rigidly connected current-collecting network (i.e., current collectors such as Al foils, Ni foams, and carbon fiber papers, etc.) is the most appropriate process particularly when using active materials that can experience severe volume/morphology change such as sulfur. In this research, by casting sulfur-based cathode composite into carbon fiber papers, unit capacities per area of 3.5 mAh/cm2 (sulfur mass-loading = 2.9 mg/cm2) and 4.9 mAh/cm2 (sulfur mass-loading = 4.1 mg/cm2) were obtained, which are almost compatible with conventional graphite (theoretical capacity = 372 mAh/g, 9 mg/cm2 offers 3.3 mAh/cm2) and hard carbon (HC) (e.g., theoretical capacity = 1000 mAh/g, 4.5 mg/cm2 offers 4.5 mAh/cm2.) anodes, respectively. Particularly, the latter is competitive enough with conventional lithium-ion batteries, graphite/LiCoO2, even allowing for the low output voltage of sulfur-based batteries (55% of conventional graphite/LiCoO2), as shown in Table 1.
Conclusion
For the next generation lithium-ion batteries, lithium/sulfur (Li/S) batteries, high mass-loading of a sulfur-based cathode composite and polysulfides stabilization were investigated by casting sulfur/polyacrylonitrile/ketjenblack (S/PAN/KB) cathode composite into carbon fiber papers. By using carbon fiber papers as current collectors, the capacity of 4.9 mAh/cm2 was achieved at a cathode composite mass-loading of 11 mg/cm2 (sulfur, 4.1 mg/cm2) that is the highest value among published/reported ones and competitive enough with the conventional LiCoO2-based cathodes. S/PAN/KB could offer stable electrochemical performance as is widely reported, even at high sulfur mass-loadings as first reported in this paper.
Conflict of Interest Statement
The authors declare that the research was conducted in the absence of any commercial or financial relationships that could be construed as a potential conflict of interest.
Acknowledgment
This publication has been made within the Sub-project #157-2013, which is funded under the Technology Commercialization Project, supported by the World Bank and the Government of the Republic of Kazakhstan.
References
Doan, T. N. L., Ghaznavi, M., Zhao, Y., Zhang, Y., Konarov, A., Sadhu, M., et al. (2013). Binding mechanism of sulfur and dehydrogenated polyacrylonitrile in sulfur/polymer composite cathode. J. Power Sources 241, 61–69. doi: 10.1016/j.jpowsour.2013.04.113
Doan, T. N. L., Gosselink, D., Hoang, T. K. A., and Chen, P. (2014). The effect of sulfur loading on the electrochemical performance of a sulfur-polymer composite cathode coated on aluminum foil. Phys. Chem. Chem. Phys. 16, 13843–13848. doi:10.1039/c4cp00974f
PubMed Abstract | Full Text | CrossRef Full Text | Google Scholar
Fanous, J., Wegner, M., Grimminger, J., Andersen, A., and Buchmeiser, M. R. (2011). Structure-related electrochemistry of sulfur-poly(acrylonitrile) composite cathode materials for rechargeable lithium batteries. Chem. Mater. 23, 5024–5028. doi:10.1021/cm202467u
Konarov, A., Gosselink, D., Doan, T. N. L., Zhang, Y., Zhao, Y., and Chen, P. (2014). Simple, scalable, and economical preparation of sulfur – PAN composite cathodes for Li/S batteries. J. Power Sources 259, 183–187. doi:10.1016/j.jpowsour.2014.02.078
Li, J., Li, K., Li, M., Gosselink, D., Zhang, Y., and Chen, P. (2014). A sulfur – polyacrylonitrile/graphene composite cathode for lithium batteries with excellent cyclability. J. Power Sources 252, 107–112. doi:10.1016/j.jpowsour.2013.11.088
Lu, S., Chen, Y., Wu, X., Wang, Z., and Li, Y. (2014). Three-dimensional sulfur/graphene multifunctional hybrid sponges for lithium-sulfur batteries with large areal mass loading. Sci. Rep. 4, 4629. doi:10.1038/srep04629
PubMed Abstract | Full Text | CrossRef Full Text | Google Scholar
Sun, L., Li, M., Jiang, Y., Kong, W., Jiang, K., Wang, J., et al. (2014). Sulfur nanocrystals confined in carbon nanotube network as a binder-free electrode for high-performance lithium sulfur batteries. Nano Lett. 14, 4044–4049. doi:10.1021/nl501486n
PubMed Abstract | Full Text | CrossRef Full Text | Google Scholar
Thieme, S., Bruckner, J., Bauer, I., Oschatz, M., Borchardt, L., Althues, H., et al. (2013). High capacity micro-mesoporous carbon – sulfur nanocomposite cathodes with enhanced cycling stability prepared by a solvent-free procedure. J. Mater. Chem. A 1, 9225–9234. doi:10.1039/c3ta10641a
Wang, C., Wang, X., Wang, Y., Chen, J., Zhou, H., and Huang, Y. (2015). Macroporous free-standing nano-sulfur/reduced graphene oxide paper as stable cathode for lithium-sulfur battery. Nano Energy 11, 678–686. doi:10.1016/j.nanoen.2014.11.060
Wang, J., Yang, J., Xie, J., and Xu, N. (2002). A novel conductive polymer – sulfur composite cathode material for rechargeable lithium batteries. Adv. Mater. 14, 963–965. doi:10.1002/1521-4095(20020704)14:13/14<963::AID-ADMA963>3.0.CO;2-S
Wang, J., Yang, J., Wan, C., Du, K., Xie, J., and Xu, N. (2003). Sulfur composite cathode materials for rechargeable lithium batteries. Adv. Funct. Mater. 13, 487. doi:10.1002/adfm.200304284
Yu, X., Xie, J., Yang, J., Huang, H., Wang, K., and Wen, Z. (2004). Lithium storage in conductive sulfur-containing polymers. J. Electroanal. Chem. 573, 121–128. doi:10.1016/j.jelechem.2004.07.004
Zhang, S. S. (2014). Understanding of sulfurized polyacrylonitrile for superior performance lithium/sulfur battery. Energies 7, 4588–4600. doi:10.3390/en7074588
Zhang, Y., Bakenov, Z., Zhao, Y., Konarov, A., Wang, Q., and Chen, P. (2014). Three-dimensional carbon fiber as current collector for lithium/sulfur batteries. Ionics 20, 803–808. doi:10.1007/s11581-013-1042-7
Zhang, Y., Zhao, Y., Konarov, A., Gosselink, D., Li, Z., Ghaznavi, M., et al. (2013a). One-pot approach to synthesize PPy@S core – shell nanocomposite cathode for Li/S batteries. J. Nanopart. Res. 15, 1–7. doi:10.1007/s11051-013-2007-5
Zhang, Y., Zhao, Y., Doan, T. N. L., Konarov, A., Gosselink, D., Soboleski, H. G., et al. (2013b). A novel sulfur/polypyrrole/multi-walled carbon nanotube nanocomposite cathode with core – shell tubular structure for lithium rechargeable batteries. Solid State Ionics 238, 30–35. doi:10.1016/j.ssi.2013.03.006
Zhang, Y., Zhao, Y., Konarov, A., Gosselink, D., Soboleski, H. G., and Chen, P. (2013c). A novel nano-sulfur/polypyrrole/graphene nanocomposite cathode with a dual-layered structure for lithium rechargeable batteries. J. Power Sources 241, 517–521. doi:10.1016/j.jpowsour.2013.05.005
Zhang, Y., Zhao, Y., Yermukhambetova, A., Bakenov, Z., and Chen, P. (2013d). Ternary sulfur/polyacrylonitrile/Mg0.6Ni0.4O composite cathodes for high performance lithium/sulfur batteries. J. Mater. Chem. A 1, 295–301. doi:10.1039/C2TA00105E
Keywords: lithium, sulfur, battery, mass-loading, polysulfide
Citation: Hara T, Konarov A, Mentbayeva A, Kurmanbayeva I and Bakenov Z (2015) High mass-loading of sulfur-based cathode composites and polysulfides stabilization for rechargeable lithium/sulfur batteries. Front. Energy Res. 3:22. doi: 10.3389/fenrg.2015.00022
Received: 05 February 2015; Accepted: 22 April 2015;
Published: 07 May 2015
Edited by:
Mariusz Walkowiak, Institute of Non-Ferrous Metals, PolandReviewed by:
Xinbing Zhao, Zhejiang University, ChinaWang Xin, South China Normal University, China
Copyright: © 2015 Hara, Konarov, Mentbayeva, Kurmanbayeva and Bakenov. This is an open-access article distributed under the terms of the Creative Commons Attribution License (CC BY). The use, distribution or reproduction in other forums is permitted, provided the original author(s) or licensor are credited and that the original publication in this journal is cited, in accordance with accepted academic practice. No use, distribution or reproduction is permitted which does not comply with these terms.
*Correspondence: Toru Hara, Institute of Batteries, Nazarbayev University Research and Innovation System, Nazarbayev University, Technopark, 53 Kabanbay Batyr Avenue, Astana 010000, Kazakhstan, hara.toru@nu.edu.kz