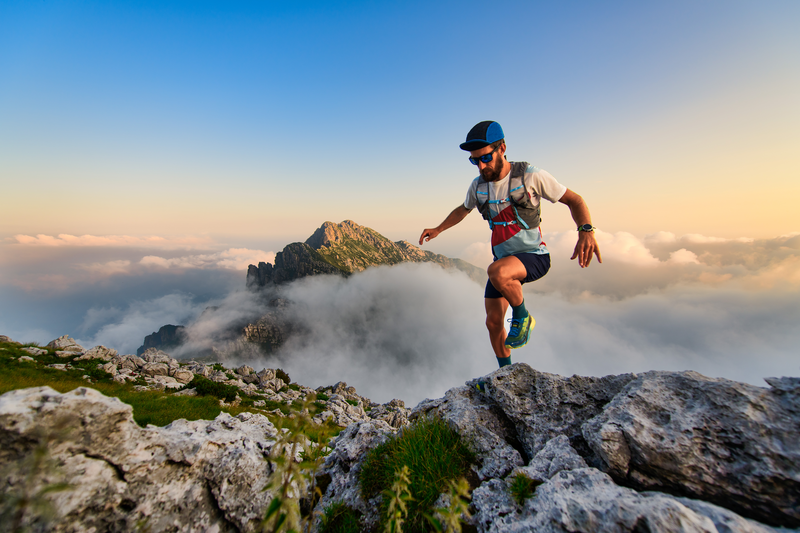
94% of researchers rate our articles as excellent or good
Learn more about the work of our research integrity team to safeguard the quality of each article we publish.
Find out more
SYSTEMATIC REVIEW article
Front. Endocrinol.
Sec. Cancer Endocrinology
Volume 16 - 2025 | doi: 10.3389/fendo.2025.1562851
The final, formatted version of the article will be published soon.
You have multiple emails registered with Frontiers:
Please enter your email address:
If you already have an account, please login
You don't have a Frontiers account ? You can register here
Background: Patients with thyroid cancer (TC) are commonly treated with radioactive iodine therapy (RIA) to prevent neoplastic transformation and the re-emergence of cancer cells.However, it has major side effects on blood cells. However, the degree of change in hematological parameters reported varies across studies. Therefore, the aim of this review was to assesses the mean differences in hematological parameters after RAI therapy.Methods: The relevant articles for this review were identified through extensive searches of databases and the Google search engine. The identified articles were subsequently selected using predetermined eligibility criteria. All relevant information from the screened articles was extracted. The pooled standardized mean differences (SMDs) of the parameters were assessed via a random effects model. The heterogeneity was determined by I 2 statistics test. Funnel plots and Egger's test were utilized to assess publication bias.Results: A total of 17 studies including 4,112 and 3,766 study participants before and after RAI therapy, respectively, were included. The pooled SMDs of the total leucocyte count (TLC) (*10 9 /L) at one, three, six and 12 months and the last follow-up period were 2.39, 2.46, 5.84, 3.19, and 0.53, respectively. Changes in the TLC after one, three and six months of therapy were statistically significant. In terms of the absolute neutrophil count (ANC; *10 9 /L) and absolute lymphocyte count (ALC; *10 9 /L), the pooled SMDs at the last follow-up period were 6.32 and 7.37, respectively. In addition, statistically significant changes in the platelet count (PLT; *10 9 /L) were observed at one, three, six and 12 months and at the last follow-up, with pooled SMDs of 7. 01, 0.22, 2.63, 6.61, and 8.76, respectively. Furthermore, statistically significant changes in red blood cells (RBCs; *10 12 /L) and hemoglobin (Hgb; g/dl) were detected after three and six months of therapy, with pooled SMDs of -1.088 and 2.4, respectively.According to the current systematic review and meta-analysis, radioiodine therapy had a significant effect on hematological parameters. Thus, early screening and correction of hematological toxicity may be helpful for improving quality of life in thyroid cancer patients receiving radioiodine therapy.
Keywords: hematological parameters, Radioactive iodine, RAI (radioiodine) ablation, therapy, thyriod cancer
Received: 18 Jan 2025; Accepted: 24 Feb 2025.
Copyright: © 2025 Berta, Teketelew, Cherie, Tamir, Abriham, Ayele, Tarekegne, Chane, Mulatie and Walle. This is an open-access article distributed under the terms of the Creative Commons Attribution License (CC BY). The use, distribution or reproduction in other forums is permitted, provided the original author(s) or licensor are credited and that the original publication in this journal is cited, in accordance with accepted academic practice. No use, distribution or reproduction is permitted which does not comply with these terms.
* Correspondence:
Dereje Mengesha Berta, School of Biomedical and Laboratory Sciences, College of Medicine and Health Sciences, University of Gondar, Gondar, Ethiopia
Disclaimer: All claims expressed in this article are solely those of the authors and do not necessarily represent those of their affiliated organizations, or those of the publisher, the editors and the reviewers. Any product that may be evaluated in this article or claim that may be made by its manufacturer is not guaranteed or endorsed by the publisher.
Research integrity at Frontiers
Learn more about the work of our research integrity team to safeguard the quality of each article we publish.