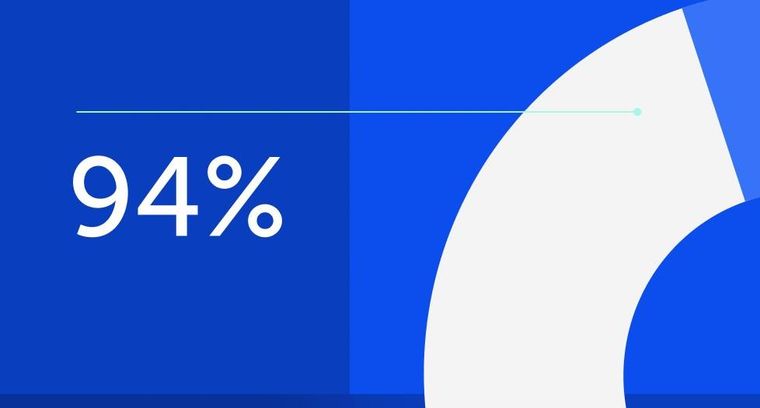
94% of researchers rate our articles as excellent or good
Learn more about the work of our research integrity team to safeguard the quality of each article we publish.
Find out more
REVIEW article
Front. Endocrinol., 03 April 2025
Sec. Obesity
Volume 16 - 2025 | https://doi.org/10.3389/fendo.2025.1558194
This article is part of the Research TopicMulti-organ Linkage Pathophysiology and Therapy for MASLD and MASH Volume IIView all 3 articles
Metabolic dysfunction-associated steatotic liver disease is a metabolic disease with an increasing incidence. Its pathogenesis involves the interaction of multiple factors. There is currently no specific treatment, so early prevention and treatment are crucial. Mesenchymal stem cells are a type of cell with the ability to self-renew and differentiate in multiple directions. They have a wide range of sources, including umbilical cords, bone marrow, and fat, and have various biological functions such as anti-inflammation, immune regulation, anti-oxidation, and inhibition of fibrosis. They have shown significant potential in the treatment of non-alcoholic fatty liver disease. In recent years, mesenchymal stem cells derived exosomes have been shown to be rich in bioactive substances, and to be involved in intercellular communication, regulating metabolism, reducing inflammatory responses, improving lipid metabolism, inhibiting fibrosis, and other processes that contribute to the treatment of metabolic dysfunction-associated steatotic liver disease. Mesenchymal stem cells and mesenchymal stem cell-derived exosomes play an important role in the pathogenesis and treatment of metabolic dysfunction-associated steatotic liver disease and provide new potential and direction for the treatment of Metabolic dysfunction-associated steatotic liver disease. This article reviews the role and effects of mesenchymal stem cells and mesenchymal stem cell-derived exosomes from different sources in Metabolic dysfunction-associated steatotic liver disease and discusses their prospects as potential therapeutic strategies.
Metabolic dysfunction-associated steatotic liver disease (MASLD) is the most common chronic disease with a prevalence approaching 25% worldwide (1–3). The disease was once known as non-alcoholic fatty liver disease (NAFLD), in 2023, a consensus panel led by the Liver Association proposed to redefine NAFLD as MASLD (4). This new definition better reflects the pathophysiological characteristics of fatty liver and metabolic abnormalities and provides a new perspective for clinical diagnosis and treatment. MASLD is a clinical and pathological syndrome with no history of excessive alcohol consumption, multiple causes, and characterized by lipid accumulation and fat degeneration in the liver (5, 6). Studies have shown that about 3% to 5% of patients with fatty liver disease can develop Metabolic dysfunction-associated steatohepatitis (MASH), which is characterized by liver inflammation and hepatocellular injury (6). As the disease progresses, hepatic steatosis can further develop into MASH and liver fibrosis, ultimately leading to cirrhosis and hepatocellular carcinoma (HCC) (7). As a complex metabolic disease, there is currently no specific treatment for MASLD (8). Lifestyle interventions are still recognized as the first choice of treatment. In addition, drug interventions can regulate glucose and lipid metabolism, mitigate inflammation, and delay liver fibrosis progression, providing an effective strategy to slow the development of MASLD (9).
Mesenchymal stem cells (MSCs) are a type of pluripotent progenitor cell with the ability to self-renew and differentiate into multiple lineages, including adipocytes, osteocytes, osteoblasts, and chondrocytes (10–12). Initially, MSCs were isolated from bone marrow mesenchymal stem cells (BM-MSCs) (BM-MSCs) (13). With the deepening of research, it was gradually discovered that MSCs also exist in adipose tissue,skeletal muscle, umbilical cord blood, lung and other tissues (14–17). MSCs from different sources have various biological functions such as anti-inflammatory, anti-oxidative, immune regulation and tissue regeneration, which makes them show unique advantages in the treatment of various diseases (18, 19). In recent years, mesenchymal stem cell-derived exosomes (MSCs-Exo) have become a research hotspot because it contains a variety of bioactive substances. Exosomes are small extracellular vesicles with diameters ranging from 30 to 150 nanometers, which are secreted by various types of cells, including stem cells, immune cells, adipocytes, hepatocytes, tumor cells, etc. (20–28). Exosomes are generated through the inward budding of the cell membrane, resulting in the formation of vesicles, which contain biologically active substances such as nucleic acids, proteins and growth factors. They play a crucial role in intercellular communication and signal transduction by merging with the cell membrane and releasing their contents into the extracellular space (29). They reach distant cells and tissues through body fluids such as blood, lymphatic fluid and saliva to regulate metabolic processes in the body (30–33). An increasing number of studies have shown that exosome-mediated drug delivery has the advantages of low toxicity, low immunogenicity and high engineering, and has broad application prospects in the treatment of diseases in the future. This article will summarize the role and therapeutic potential of microRNAs derived from MSC- Exo, especially those derived from mesenchymal stem cells, in MASLD.
The pathogenesis of MASLD involves the interaction of multiple factors, including insulin resistance (IR), excessive accumulation of fatty acids, oxidative stress, inflammatory response, intestinal microbiota imbalance, adipose tissue and mitochondrial dysfunction, and liver fibrosis (34, 35). With the innovation of detection technology and the emergence of new research results, the “second hit” hypothesis proposed by James and Day can no longer summarize the complex and ever-changing pathogenesis of MASLD (34). However, the emergence of new theories such as the “three-hit hypothesis” in recent years has well compensated for the deficiencies of the “two-hit” theory, which includes steatosis, lipotoxicity and inflammation (6, 36). IR is one of the core pathogenesis mechanisms of MASLD (37). Insulin normally inhibits lipolysis in adipose tissue. However, when the sensitivity of insulin-targeted organs (such as the liver, muscle and adipose tissue) to insulin decreases, the inhibitory effect of insulin is weakened, leading to fat accumulation in the liver. IR leads to increased fat accumulation in the liver by stimulating lipolysis and inducing hyperinsulinemia, thereby setting the stage for the excessive buildup of fatty acids (38).
Excessive accumulation of fatty acids in the liver is a key link in the progression of MASLD. In the context of insulin resistance, the breakdown and oxidation of fatty acids increases, leading to many free fatty acids entering hepatocytes, promoting lipotoxicity and mitochondrial dysfunction in hepatocytes, thereby triggering hepatocyte apoptosis. These fatty acids cannot be completely oxidized, thus accumulating in the liver and forming steatosis (39, 40). Excessive accumulation of fatty acids not only increases the pressure inside liver cells, but also provides conditions for the occurrence of oxidative stress and inflammation. In addition, dysfunction of adipose tissue can lead to an imbalance of adipokines, such as abnormal secretion of leptin and adiponectin, which exacerbates lipid accumulation in the liver. At the same time, these adipokines play a critical role in mediating the inflammatory response and driving the fibrosis process (41). Excessive accumulation of fatty acids and abnormal metabolism directly lead to increase in reactive oxygen species (ROS) in liver cells, which activates oxidative stress (42). Oxidative stress exacerbates the inflammatory response in the liver by damaging liver cells, promoting the peroxidation of fatty acids, and increasing the release of cytokines, leading to chronic low-grade chronic inflammation, infiltration of immune cells such as adipocytes, macrophages, and T cells, and production of inflammatory mediators such as cytokines and chemokines. These inflammatory reactions further promote cell damage and fibrosis in the liver, which may eventually progress to cirrhosis or hepatocellular carcinoma (43, 44).
The final stage of MASLD is liver fibrosis, which is usually caused by an imbalance between hepatocyte death and reduced regenerative capacity. Persistent harmful factors (such as oxidative stress, inflammation, and intestinal microbiota imbalance) cause activation of hepatic stellate cells, which in turn leads to excessive extracellular matrix accumulation, ultimately leading to changes in liver structure, accompanied by fibrosis, regenerative nodules, and inflammatory cell infiltration. Although the current treatment of choice for MASLD is lifestyle intervention (e.g., diet, exercise, and weight loss), there is still a lack of specific drugs for MASLD (9). New therapeutic strategies focus on mitigating pathological processes, including lipid accumulation, inflammation, and oxidative stress in the liver. In addition, novel therapies based on non-coding RNAs, and MSCs-Exo are becoming a research hotspot and hold the potential to bring new breakthroughs in the treatment of MASLD.
Mesenchymal stem cells (MSCs) affect the occurrence and development of MASLD through multiple pathways. The main mechanisms include: (1) improving metabolic disorders; (2) reducing inflammation and oxidative stress; (3) antifibrotic effects; (4) inducing autophagy; and (5) immunosuppressive effects (Figure 1).
Figure 1. The schematic role of MSC in MASLD. Mechanisms and pathways of mesenchymal stem cells (MSCs) in the treatment of MASLD. This figure outlines the mechanisms and signaling pathways by which MSCs exert their therapeutic effects, specifically targeting liver function to alleviate MASLD, and it outlines the mechanisms and pathways associated with the therapeutic efficacy of different sources of MSCs and combination therapies.
ADSCs show great potential in the research and treatment of MASLD due to their rich sources, easy availability, and low impact on the body. Studies have shown that ADSCs can effectively improve the symptoms of MASLD through mechanisms such as regulating lipid metabolism, reducing inflammation, relieving oxidative stress, and anti-fibrosis. For example, Watanabe et al. found in a Mc4r-KO knockout MASH mouse and a LPS-induced MASH mouse model that ADSC intervention significantly reduced the levels of serum ALT and inflammatory markers, while increasing the proportion of anti-inflammatory macrophages in the liver, providing a new basis for the treatment of MASLD (45). Saleh et al. also studied obesity-related MASLD and showed that ADSC treatment can reverse liver fat accumulation in obese mice and decrease TNF-α and IL-6 expression levels, improving MASLD symptoms (46). In addition to reducing inflammation, antifibrosis is also an important target in the treatment of MASLD (47). Yano et al. found that in a mouse model of MASH, u-ADSCs were able to reduce liver inflammation and cell infiltration and showed similar therapeutic effects to wild-type u-ADSCs in terms of fibrosis. In particular, MASH (12 weeks) u-ADSCs performed better in regulating metabolism and intracellular transport, while MASH (4 weeks) u-ADSCs were more significant in reducing inflammation (48). In addition, the therapeutic potential of modified ADSCs in MASLD has also received widespread attention. For example, Domingues et al. used an adenovirus to construct the antioxidant gene Sod2 and upregulate it in ADSCs. The study found that modified ADSCs significantly improved the liver fat content of obese mice, mainly by reducing liver inflammation and oxidative stress (49). This was also verified in an in vivo experiment by Afarin et al., who found that by injecting LPS-stimulated ADSCs into injecting LPS-stimulated ADSCs into a MASLD rat model, it was found that they not only effectively corrected liver enzyme levels (such as ALT and AST), it not only significantly decreased the expression of transforming growth factor β (TGF-β) and inflammation-related genes but also lowered the levels of ROS, demonstrating the potential of ADSCs in the treatment of MASLD (50).
UCMSCs, as another source of mesenchymal stem cells, are not only easy to obtain, but also exhibit stronger differentiation potential and lower immune rejection compared to mesenchymal stem cells from other sources, making it a potential therapeutic strategy for treating MASLD. In particular, human-derived umbilical cord mesenchymal stem cells (HUCMSCs) have attracted widespread attention for their use in MASLD. Existing studies have shown that hUCMSCs mainly function in the treatment of MASLD through mechanisms such as anti-inflammation, anti-fibrosis, autophagy induction, regulation of lipid metabolism, and improvement of insulin resistance. In a mouse model of MASLD, HUCMSCs significantly improved liver function and reduced lipid deposition by tail vein injection, and improved lipid metabolism by upregulating the HNF4α-CES2 pathway and regulating genes related to fatty acid oxidation (51). Impaired autophagy is another important mechanism of MASLD (52). Studies have found that HUCMSCs can promote autophagy by suppressing the expression of mTOR and P62, and promoting the levels of AMPK and LC3BII/α, thereby accelerating the degradation of body fat and alleviating the symptoms of MASLD (53). In addition, HUCMSCs also improve fat metabolism and reduce inflammatory responses by regulating the PPAR signaling pathway, enhancing the expression of PPAR-α, and targeting CPT1A. Further studies found that in mice treated with HUCMSCs, the expression of α-SMA protein and various fibrosis-related collagens (such as Col1a1, Col1a2, and Col3a1) was significantly downregulated, reducing the degree of liver fibrosis (54). In recent years, researchers have explored the potential of combining HUCMSCs with other treatments to further improve the therapeutic effect of MASLD. For example, one study showed that the combination of HUCMSCs and liraglutide significantly improved liver function damage and pathological changes in rats with MASLD associated with type 2 diabetes mellitus (T2DM). After 8 weeks of combined treatment, HbA1c, HOMA-IR, ALT and AST levels were significantly reduced, and glucometabolic, insulin resistance and liver damage were significantly improved. At the same time, they inhibited the TLR4/NF-κB inflammatory pathway, resulting in a significant reduction in the expression of IL-6, TNF-α, and the antioxidant enzyme superoxide dismutase (SOD). These results suggest that combination therapy with hUCMSCs and other drugs may be an effective strategy to enhance the therapeutic effect, providing new ideas for the clinical management of MASLD (55).
BMSCs are one of the most extensively researched types of MSCs. Similar to other MSC types, BMSCs have the advantages of being easy to obtain, having multi-directional differentiation potential and low immunogenicity. They are also ideal for treating a variety of diseases because they do not involve ethical issues and can be autologous transplanted. Existing studies have shown that BMSCs can significantly improve MASLD through multiple mechanisms, such as anti-inflammation, anti-fibrosis, inhibition of T cell proliferation, alleviation of mitochondrial dysfunction, alleviation of endoplasmic reticulum stress, and regulation of glycolipid metabolism.The study shows that that BMSCs have a significant role on obese mice. Compared with untreated mice, BMSCs-treated mice can effectively prevent the occurrence of liver fibrosis. This is manifested in the fact that the gene expression levels of inflammatory factors and fibrosis markers in the liver (such as IL-1β, INF-γ, TNF-α, TGF-β1 and collagen type I) are close to normal or even lower than normal, thereby reducing liver tissue damage (56).
Endoplasmic reticulum (ER) stress is considered an important trigger of MASLD (57). Li et al. found that BMSCs intervention can improve steatosis, insulin resistance and dyslipidemia, and further revealed its potential molecular mechanism. Specifically, BMSCs alleviate endoplasmic reticulum stress in MASLD rats and PA-induced HepG2 cells by regulating SERCA (calcium pump) to restore intracellular Ca²+ homeostasis, inhibit cell pyroptosis, and improve metabolic dysfunction (58). In recent years, MSC has gradually become an emerging way to improve disease progression through the mitochondrial transfer mechanism. Bi et al. found that BMSCs significantly reduced ROS production and enhanced oxidative phosphorylation (OXPHOS) activity and ATP production by transferring mitochondria to fat cells, thereby effectively maintaining the cell’s energy balance and restoring liver function. This mechanism can inhibit the inflammatory response and lipid and glucose metabolism disorders associated with MASLD (59). Nickel et al. further verified this finding in their study, which showed that by transplanting the mitochondria of human BMSCs into the hepatocytes of MASH mice, the lipid decomposition capacity can be enhanced, thereby significantly improving the lipid load of MASH mice (60). This innovative mechanism repairs damaged cells through mitochondrial transfer, independent of cell differentiation or paracrine effects, providing new research direction for cell therapy.
In addition to the aforementioned common adipose tissue-, bone marrow- and umbilical cord-derived MSCs, other sources of MSCs, such as bone-derived MSCs and menstrual blood-derived endometrial stem cells (MenSCs), have also shown potential in the treatment of MASLD. For example, Wang et al. showed that bone-derived MSCs inhibit the proliferation of CD4+ T cells through immunomodulatory effects, thereby reducing inflammation in MASLD (61). In addition, they conducted another study to demonstrate that in a mouse model of MCD diet-induced MASH, this protective effect may be related to the activation of bone-derived MSCs to inhibit the secretion of IFN-γ and IL-6 by CD4+ T cells (62). MenSCs, on the other hand, target the AMPK-mTOR signaling pathway by secreting the novel regulatory factor Rnf186, which improves lipid metabolism and insulin resistance in MASLD mice, thereby alleviating the symptoms of MASLD (63). In summary, mesenchymal stem cells of different origins affect the development and progression of MASLD through multiple mechanisms, providing more options for the future treatment of MASLD. Future research will further explore the therapeutic potential of different MSC sources and promote their clinical application.
In summary, mesenchymal stem cells from different sources have their own advantages in the treatment of MASLD and can influence the onset and progression of MASLD through distinct mechanisms. This provides more treatment choices for MASLD, especially in the context of individualized treatment. The appropriate source of MSCs can be selected according to specific circumstances to achieve more precise and effective treatment. At present, there are still many gaps in the application of MSCs from other sources in the treatment of MASLD, and their specific mechanisms need to be further explored to promote the clinical application of MSCs in treating MASLD (Supplementary Table 1).
MSCs-Exo play an important role in the treatment of MASLD, mainly through the following mechanisms: (1) inhibiting oxidative stress and inflammatory response; (2) regulating glycolipid metabolism; (3) inhibiting apoptosis and inducing autophagy; (4) alleviating liver fibrosis (Figure 2).
Figure 2. The schematic role of MSC exosomes in MASLD. Mechanisms and pathways of exosomes derived from different MSCs in the treatment of MASLD therapy. Exosomes derived from different MSCs have anti-inflammatory, oxidative stress inhibiting, lipid metabolism regulating, apoptosis inhibiting, autophagy inducing, and liver fibrosis attenuating effects to protect MASLD.
In recent years, ADSC-Ex has emerged as a novel therapeutic strategy that has unique advantages in the occurrence, development and treatment of MASLD. Number of studies have shown that ADSC-Exo not only affects the onset and progression of MASLD through multiple pathways, but also effectively relieves the symptoms of MASLD, including regulating glycolipid metabolism, inhibiting inflammatory response and liver fibrosis. Abnormal liver lipid metabolism and abnormal accumulation of lipids are key factors in the development of MASLD. Studies have found that ADSC-Exo can regulate lipid metabolism in multiple ways to reduce lipid accumulation and thereby improve MASLD symptoms. For example, a study by Qinghui Niu et al. showed that ADSC-Exo carrying miR-223-3p can target and inhibit the expression of E2F1, thereby inhibiting lipid accumulation and liver fibrosis and improving MASLD symptoms (64). In addition, studies have indicated that adipose tissue plays a key role in a number of diseases associated with insulin resistance, particularly MASLD. Togliatto et al. found that ADSC-Exo derived from obese individuals may have therapeutic potential in the process of angiogenesis, and also showed that ADSC-Exo plays an important role in obesity-related metabolic complications (65). A Baranova et al. believe that miRNAs such as miR-122 and other miRNAs may play a positive role in preventing MASLD -related hepatocellular carcinoma, and MSC-derived exosomes derived from adipose tissue are an ideal vehicle for these miRNAs (66). Qinghui Niu’s research also showed that miR-223-3p carried by ADSC-Exo can delay the progression of MASLD, suggesting that ADSC-Exo loaded with miR-223-3p may be a potential strategy for the treatment of MASLD.
HUCMSCs-Exo is an exosome with a wide range of sources and low immunogenicity. Meanwhile, current research shows that PPARα can improve steatosis, inflammation and fibrosis, and is a potential new therapeutic target for MASLD (67). Studies have shown that hUCMSCs-Exo can significantly improve the symptoms of MASLD by regulating lipid metabolism, reducing inflammation, and reducing oxidative stress, inhibiting apoptosis, inducing autophagy, and inhibiting liver fibrosis. These functions mainly depend on the miRNA carried by it to regulate related signal pathways. In the early stages of MASLD, abnormal lipid deposition and steatosis are its main pathological features (68). HUCMSCs-Exo improves MASLD by regulating lipid metabolism to reduce lipid accumulation. Fuji Yang et al. showed that h UCMSCs-Exo can significantly reduce lipid accumulation and improve hepatic steatosis by activating the AMPK-dependent pathway to inhibit SREBP-1c-mediated fatty acid synthesis and enhance peroxisome proliferator-activated receptor (PPARα) mediated fatty acid oxidation (69). In addition, Lidan Cheng and others found that miR-627-5p carried by hUCMSCs-Exo can inhibit the expression of genes associated with fat synthesis,(such as G6Pc, PEPCK, FAS and SREBP-1c) in palmitic acid-treated L-O2 cells, while upregulating the expression of PPARα, thereby improving glycolipid metabolism and reducing lipid accumulation (70). Inflammation and oxidative stress are key mechanisms in the development of MASLD, and hUCMSCs-Exo also showed significant efficacy in inhibiting inflammation and oxidative stress. Studies have shown that hUCMSCs-Exo alleviates inflammatory and oxidative stress responses by reducing the secretion of TNF-α and IL-6,and activating the Nrf2/NQO-1 antioxidant signaling pathway (71). In addition, miR-24-3p in hUCMSCs-Exo can further alleviate inflammation and oxidative stress by targeting and inhibiting Keap-1 to reduce ROS production (72).
As MASLD progresses, liver fibrosis becomes another key pathological feature. Recent studies have found that hUCMSCs-Exo can effectively inhibit liver fibrosis and delay the progression of MASLD through the anti-fibrosis miRNAs it carries. For example, Sani et al. showed that anti-miR-17-5p-enriched hUCMSCs-Exo can inhibit the progression of liver fibrosis by downregulating the expression of TGF-β1, IL-1β, and IL-6, thereby reducing the accumulation of extracellular matrix (73). Exosomes can enhance hepatocyte autophagy through the AMPK/mTOR or ei24-related autophagy pathway, thereby reducing liver fat and collagen deposition (53). In addition, He et al. found that hUCMSCs-Exo can promote the formation of autophagosomes in T2DM rats and palmitic acid-treated L-O2 cells, and improve glycolipid metabolism through the autophagy pathway (74). It is worth noting that Tawfeek et al. found that curcumin-pretreated hUCMSCs-Exo improved lipid accumulation and reduced liver inflammation and oxidative stress in MASH mice. This study shows that the pretreated hUCMSCs-Exo further enhances its efficacy, providing greater potential for clinical treatment (75). These studies provide an important theoretical basis and new research directions for the future clinical treatment of MASLD, and provide more possibilities for the clinical application of MSCs-Exo.
BM-MSCs are one of the most used sources of MSCs. Recent studies have shown that BM-MSCs-Exo also play an important role in the treatment of MASLD. BM-MSCs-Exo alleviate the progression of MASLD through multiple pathways, including anti-apoptosis, improving lipid metabolism, enhancing mitochondrial autophagy, anti-inflammation and improving insulin resistance. Insulin resistance is the “first blow” in the hypothesis of the pathogenesis of MASLD. Studies have found that BM-MSCs-Exo can alleviate the progression of MASLD by activating the PI3K/AKT signaling pathway, improving IR and lipid droplet accumulation, and promoting the expression of glucose transporter 4 (GLUT4) (76). In addition, BM-MSCs-Exo can improve steatosis in MASH rats by regulating lipid metabolic disorders. For example, El-Derany et al. found that BM-MSCs-Exo treatment significantly downregulated the expression of genes related to fatty acid synthesis and uptake, significantly upregulated the expression of genes related to fatty acid oxidation, and improved the steatosis of MASH (77). BM-MSCs-Exo can also reduce the Bax/Bcl2 ratio in the liver of MASH rats and protect hepatocytes from apoptosis through its anti-apoptotic effect. Further studies have shown that BM-MSCs-Exo can alleviate the effects of a high-fat diet on the liver by activating the expression of mitochondrial autophagy genes (77). In addition, miRNA-96-5p plays an important role in BM-MSCs-Exo, and its regulatory mechanism is closely related to the therapeutic effect of MASLD.
In addition to the above sources, other types of MSC-Exos (such as MSC-Exo derived from umbilical cord blood, placenta, and dental pulp) also show good prospects in the treatment of MASLD. Although these studies are still in their infancy, there is growing interest in their potential therapeutic effects on MASLD. This is confirmed by the study by Chang, Chao-Yuan et al., which demonstrated that treatment with exosomes derived from human placental chorionic mesenchymal stem cells can reduce inflammation and inhibit the expression of NF-κB and HIF-1α in the liver tissue of obese mice (78). In addition, let-7i-5p miRNA is highly expressed in exosomes derived from human placental chorionic mesenchymal stem cells. Studies of its associated mechanisms have found that it mediates the treatment of obesity-associated sepsis, but whether it plays a role in the treatment of obesity-associated MASLD is unknown (Supplementary Table 2).
MSC-Exo has important potential in the treatment of MASLD. The above studies have shown that MSC-Exo improves the symptoms of MASLD through mechanisms such as regulating glycolipid metabolism, inhibiting inflammation and oxidative stress, inducing autophagy, and reducing fibrosis (Figure 3). Compared with traditional MSC therapy, MSC-Exo, with its low immunogenicity, small size, and higher biosafety, provides a new idea for precision intervention in MASLD. However, current research on MSC-Exo for the treatment of MASLD is still in the experimental stage, and its clinical translation still faces many challenges. Studies have shown that there are differences in the efficacy of MSCs and MSC-Exos from different sources (fat, umbilical cord, bone marrow, etc.), which may be related to donor factors (age, health status), culture conditions, and exosome isolation methods (ultracentrifugation, size exclusion chromatography, etc.) (79, 80). In addition, there is a lack of uniform standards for the isolation and characterization of MSC-Exo, which affects the comparability between different studies and poses certain challenges in assessing the optimal applicability of different MSC sources (81). Therefore, establishing a standardized MSC isolation, culture and delivery protocol is crucial to improving its clinical translation. In addition, current studies on MSCs and MSC-Exo in the field of MASLD generally have small sample sizes, lack randomized controlled trials, and have few primate models and clinical investigations, which are mostly limited to animal models and in vitro experiments. Although existing animal models (such as obesity models) and in vitro models can simulate some of the disease characteristics of MASLD that are similar to those in humans, the effects of in vivo and in vitro studies cannot be directly and effectively applied to the clinic because animal models and in vitro models differ from humans in terms of metabolism, immunity and disease development. This step limits their clinical application to a certain extent.
Figure 3. Graphical abstract. The different sources of MSCs and MSCs-exo. MSCs are harvested from various tissue sources, including adipose tissue, bone marrow, umbilical cord, endometrium, long bones, and placenta. This figure illustrates the evaluation of the therapeutic potential of MSCs and their exosomes.
The safety and efficacy of MSCs and MSC-exos in different MASLD patient populations still need to be determined by robust clinical trials (82). Clinical studies of MSC and MSC-Exo therapy for MASLD are still in their early stages. Most of these studies are still in phase I or II, and there are no large-scale phase III studies yet. Therefore, only short-term effects can be used as a reference, and the long-term efficacy and safety need to be verified (83). As pointed out by Jinag et al., the long-term safety of MSC and MSCs-Exo therapy is also a key issue (84). Although MSC and MSC-Exo did not cause significant rejection or tumorigenesis in early clinical studies, MSCs have a certain degree of plasticity. When MSCs and MSC-Exo are used for a long time or in high doses, we still need to fully evaluate the risk of their possible unintended differentiation (such as fibroblast-like differentiation), immune rejection, tumorigenesis, and long-term adverse effects on liver tissue (85, 86). A meta-analysis showed that hUCMSC therapy can improve glucose metabolism and insulin secretion in T2DM, providing some hope for hUCMSC therapy for DN (87). This was also confirmed by another meta-analysis study, which reported that MSC and MSCs-Exo therapy is a relatively safe treatment that can significantly improve liver function compared to conventional therapy (88). However, the inherent heterogeneity of MSCs results in significant variability in the therapeutic effects of these cells depending on their source (bone marrow, adipose tissue, or umbilical cord) and the methods used for isolation and preparation. Therefore, strategies to improve their safety, including but not limited to autologous MSC transplantation, immunosuppressive therapy, and gene editing techniques, are being explored to reduce their potential therapeutic risks. In addition, MSC and its MSC-Exo are biological agents, and various countries have strict regulatory requirements for the use of MSC-Exo in disease treatment, which is a major challenge to be solved in the future. Although MSC and MSCs-Exo have great potential in the treatment of MASLD, there is a lack of uniform standards for MSC and MSCs-Exo isolation protocols, effective doses, efficacy, cargo content, and information on their heterogeneous populations, making it difficult to draw conclusions (89). These issues pose major obstacles to current research on MSCs and their derived exosomes, and need to be addressed through further research. In the future, to promote the clinical application of MSCs and MSCs-Exo in the treatment of MASLD, in addition to the above challenges, optimizing miRNA delivery strategies is also an important issue. Enhancing the expression of specific miRNAs through genetic engineering or using engineered exosomes to improve the efficiency of targeted delivery can enhance their therapeutic effects (90). At the same time, exploring biomarkers that can predict efficacy is also a key direction, such as exosomes protein or miRNA indicators in the blood circulation, in order to screen for patients who are more suitable for MSC-Exo treatment and achieve personalized medicine (91). In addition, the production efficiency and quality of exosomes still face technical challenges. In the future, the yield and quality control can be improved by microfluidic chip separation or bioreactor culture technology to meet clinical needs (92).The above issues are the main challenges currently facing the research on MSCs and their derived exosomes. Future research needs to address these challenges and conduct large-scale, multi-center clinical studies. Integrating MSC and MSCs-Exo therapies into clinical practice can provide new hope for the effective treatment of MASLD patients and improve patient outcomes.
As mentioned above, the progression of MASLD involves multiple pathogenesis mechanisms, including the result of the combined effects of various factors such as environment and genetics. We summarize the research progress of the effects of MSCs and MSCs-Exo on MASLD and provide a brief overview of the involved mechanisms. MSCs and MSCs-Exo are important for the treatment of MASLD due to their differentiation ability and other promising properties, by inducing anti-inflammation, improving lipid metabolism, enhancing insulin sensitivity, balancing oxidation and anti-fibrosis. However, there are still many gaps that need to be further explored and filled. In addition, the preparation, quality control and safety of MSCs and MSCs-Exo are all practical and important issues that will face future clinical applications. In the future, promoting the application of MSCs and MSCs-Exo in the treatment of MASLD will require optimizing miRNA delivery, screening for biomarkers to achieve personalized treatment, and improving production efficiency and quality control of exosomes to meet clinical needs. In summary, MSCs and MSCs-Exo have great potential in the treatment of MASLD. We need to continue to explore and improve the therapeutic strategies of MSCs and their derived exosomes for the treatment of MASLD. This will provide more effective, safe and reliable options for the prevention and treatment of MASLD.
DQ: Visualization, Writing – original draft, Writing – review & editing. PH: Visualization, Writing – original draft, Writing – review & editing. JC: Writing – review & editing. CW: Writing – review & editing. YL: Resources, Supervision, Writing – review & editing.
The author(s) declare that financial support was received for the research and/or publication of this article. This work was supported by National Natural Science Foundation of China (82360160) and Guangxi Nature Foundation Joint Submission Project (2024GXNSFAA010239).
We thank figure draw for Medpeer and Biorender.
The authors declare that the research was conducted in the absence of any commercial or financial relationships that could be construed as a potential conflict of interest.
The author(s) declare that no Generative AI was used in the creation of this manuscript.
All claims expressed in this article are solely those of the authors and do not necessarily represent those of their affiliated organizations, or those of the publisher, the editors and the reviewers. Any product that may be evaluated in this article, or claim that may be made by its manufacturer, is not guaranteed or endorsed by the publisher.
The Supplementary Material for this article can be found online at: https://www.frontiersin.org/articles/10.3389/fendo.2025.1558194/full#supplementary-material
1. Loomba R, Sanyal AJ. The global NAFLD epidemic. Nat Rev Gastroenterol Hepatol. (2013) 10:686–90. doi: 10.1038/nrgastro.2013.171
2. Younossi ZM, Koenig AB, Abdelatif D, Fazel Y, Henry L, Wymer M. Global epidemiology of nonalcoholic fatty liver disease—Meta-analytic assessment of prevalence, incidence, and outcomes. Hepatology. (2016) 64:73. doi: 10.1002/hep.28431
3. Zhou F, Zhou J, Wang W, Zhang X-J, Ji Y-X, Zhang P, et al. Unexpected rapid increase in the burden of NAFLD in China from 2008 to 2018: A systematic review and meta-analysis. Hepatology. (2019) 70:1119–33. doi: 10.1002/hep.30702
4. Lazarus JV, Newsome PN, Francque SM, Kanwal F, Terrault NA, Rinella ME. Reply: A multi-society Delphi consensus statement on new fatty liver disease nomenclature. Hepatology. (2024) 79:E93–4. doi: 10.1097/HEP.0000000000000696
5. Review Team, LaBrecque DR, Abbas Z, Anania F, Ferenci P, Khan AG, et al. World Gastroenterology Organisation global guidelines: Nonalcoholic fatty liver disease and nonalcoholic steatohepatitis. J Clin Gastroenterol. (2014) 48:467–73. doi: 10.1097/MCG.0000000000000116
6. Cobbina E, Akhlaghi F. Non-alcoholic fatty liver disease (NAFLD) - pathogenesis, classification, and effect on drug metabolizing enzymes and transporters. Drug Metab Rev. (2017) 49:197–211. doi: 10.1080/03602532.2017.1293683
7. Pafili K, Maltezos E, Papanas N. Ipragliflozin and sodium glucose transporter 2 inhibitors to reduce liver fat: will the prize we sought be won? Expert Opin Pharmacother. (2018) 19:185–7. doi: 10.1080/14656566.2017.1413346
8. European Association for the Study of the Liver (EASL), European Association for the Study of Diabetes (EASD), European Association for the Study of Obesity (EASO). EASL-EASD-EASO Clinical Practice Guidelines on the management of metabolic dysfunction-associated steatotic liver disease (MASLD). J Hepatol. (2024) 81:492–542. doi: 10.1016/j.jhep.2024.04.031
9. Armstrong MJ, Gaunt P, Aithal GP, Barton D, Hull D, Parker R, et al. Liraglutide safety and efficacy in patients with non-alcoholic steatohepatitis (LEAN): a multicentre, double-blind, randomised, placebo-controlled phase 2 study. Lancet. (2016) 387:679–90. doi: 10.1016/S0140-6736(15)00803-X
11. Pittenger MF, Mackay AM, Beck SC, Jaiswal RK, Douglas R, Mosca JD, et al. Multilineage potential of adult human mesenchymal stem cells. Science. (1999) 284:143–7. doi: 10.1126/science.284.5411.143
12. Zepp JA, Zacharias WJ, Frank DB, Cavanaugh CA, Zhou S, Morley MP, et al. Distinct mesenchymal lineages and niches promote epithelial self-renewal and myofibrogenesis in the lung. Cell. (2017) 170:1134–1148.e10. doi: 10.1016/j.cell.2017.07.034
13. Friedenstein AJ, Piatetzky-Shapiro II, Petrakova KV. Osteogenesis in transplants of bone marrow cells. J Embryol Exp Morphol. (1966) 16:381–90. doi: 10.1242/dev.16.3.381
14. Costela Ruiz VJ, Melguizo Rodríguez L, Illescas Montes R, García Recio E, Arias Santiago S, Ruiz C, et al. Human adipose tissue-derived mesenchymal stromal cells and their phagocytic capacity. J Cell Mol Med. (2022) 26:178–85. doi: 10.1111/jcmm.17070
15. Gao X, Ren H, Zhang Z, Cao S, Zhang B, Sun Q, et al. Human lung cancer-derived mesenchymal stem cells promote tumor growth and immunosuppression. Biol Direct. (2024) 19:39. doi: 10.1186/s13062-024-00479-w
16. Hao X, Zhu H, Qin C, Li L, Lin Z, Jiang H, et al. Study on preclinical safety and toxic mechanism of human umbilical cord mesenchymal stem cells in F344RG rats. Stem Cell Rev Rep. (2024) 20:2236–52. doi: 10.1007/s12015-024-10780-w
17. Tamaki T, Natsume T, Katoh A, Shigenari A, Shiina T, Nakajima N, et al. Skeletal muscle-derived stem cell transplantation accelerates the recovery of peripheral nerve gap injury under 50% and 100% Allogeneic compatibility with the swine leucocyte antigen. Biomolecules. (2024) 14:939. doi: 10.3390/biom14080939
18. Meng F-Q, Li C-C, Xu W-J, Deng J-H, Guan Y-J, Zhang T-Y, et al. Induced pluripotent stem cell-derived mesenchymal stem cells enhance acellular nerve allografts to promote peripheral nerve regeneration by facilitating angiogenesis. Neural Regener Res. (2024). doi: 10.4103/NRR.NRR-D-22-00311
19. Tian C, Ye L, Zhao X, Zhu X, Xu J, Pan X. Umbilical cord mesenchymal stem cells: A novel approach to intervention of ovarian ageing. Regener Ther. (2024) 26:590–8. doi: 10.1016/j.reth.2024.08.006
20. Johnstone RM, Adam M, Hammond JR, Orr L, Turbide C. Vesicle formation during reticulocyte maturation. Association of plasma membrane activities with released vesicles (exosomes). J Biol Chem. (1987) 262:9412–20. doi: 10.1016/S0021-9258(18)48095-7
21. da Silva Novaes A, Borges FT, Maquigussa E, Varela VA, Dias MVS, Boim MA. Influence of high glucose on mesangial cell-derived exosome composition, secretion and cell communication. Sci Rep. (2019) 9:6270. doi: 10.1038/s41598-019-42746-1
22. Yao X, Wei W, Wang X, Chenglin L, Björklund M, Ouyang H. Stem cell derived exosomes: microRNA therapy for age-related musculoskeletal disorders. Biomaterials. (2019) 224:119492. doi: 10.1016/j.biomaterials.2019.119492
23. Li H, Zeng C, Shu C, Cao Y, Shao W, Zhang M, et al. Laminins in tumor-derived exosomes upregulated by ETS1 reprogram omental macrophages to promote omental metastasis of ovarian cancer. Cell Death Dis. (2022) 13:1028. doi: 10.1038/s41419-022-05472-7
24. Zhang Q, Deng T, Zhang H, Zuo D, Zhu Q, Bai M, et al. Adipocyte-derived exosomal MTTP suppresses ferroptosis and promotes chemoresistance in colorectal cancer. Adv Sci (Weinh). (2022) 9:e2203357. doi: 10.1002/advs.202203357
25. Zhang W, Zhang J, Shi H, Liu F, Yu H, Shi H. Exosome GLUT1 derived from hepatocyte identifies the risk of non-alcoholic steatohepatitis and fibrosis. Hepatol Int. (2023) 17:1170–81. doi: 10.1007/s12072-023-10520-1
26. Qin M, Xing L, Wu J, Wen S, Luo J, Chen T, et al. Skeletal muscle-derived exosomal miR-146a-5p inhibits adipogenesis by mediating muscle-fat axis and targeting GDF5-PPARγ Signaling. Int J Mol Sci. (2023) 24:4561. doi: 10.3390/ijms24054561
27. Sitbon A, Delmotte P-R, Pistorio V, Halter S, Gallet J, Gautheron J, et al. Mesenchymal stromal cell-derived extracellular vesicles therapy openings new translational challenges in immunomodulating acute liver inflammation. J Transl Med. (2024) 22:480. doi: 10.1186/s12967-024-05282-9
28. Yu Y, Li T, Ou M, Luo R, Chen H, Ren H, et al. OX40L-expressing M1-like macrophage exosomes for cancer immunotherapy. J Control Release. (2024) 365:469–79. doi: 10.1016/j.jconrel.2023.11.051
29. Théry C, Zitvogel L, Amigorena S. Exosomes: composition, biogenesis and function. Nat Rev Immunol. (2002) 2:569–79. doi: 10.1038/nri855
30. Zhan Y, Du L, Wang L, Jiang X, Zhang S, Li J, et al. Expression signatures of exosomal long non-coding RNAs in urine serve as novel non-invasive biomarkers for diagnosis and recurrence prediction of bladder cancer. Mol Cancer. (2018) 17:142. doi: 10.1186/s12943-018-0893-y
31. Herrmann IK, Wood MJA, Fuhrmann G. Extracellular vesicles as a next-generation drug delivery platform. Nat Nanotechnol. (2021) 16:748–59. doi: 10.1038/s41565-021-00931-2
32. Li Y-J, Wu J-Y, Liu J, Xu W, Qiu X, Huang S, et al. Artificial exosomes for translational nanomedicine. J Nanobiotechnol. (2021) 19:242. doi: 10.1186/s12951-021-00986-2
33. Zhou X, Zhao S, Li W, Ruan Y, Yuan R, Ning J, et al. Tubular cell-derived exosomal miR-150-5p contributes to renal fibrosis following unilateral ischemia-reperfusion injury by activating fibroblast in vitro and in vivo. Int J Biol Sci. (2021) 17:4021–33. doi: 10.7150/ijbs.62478
34. Day CP, James OF. Steatohepatitis: a tale of two “hits”? Gastroenterology. (1998) 114:842–5. doi: 10.1016/s0016-5085(98)70599-2
35. Conlon BA, Beasley JM, Aebersold K, Jhangiani SS, Wylie-Rosett J. Nutritional management of insulin resistance in nonalcoholic fatty liver disease (NAFLD). Nutrients. (2013) 5:4093–114. doi: 10.3390/nu5104093
36. Haas JT, Francque S, Staels B. Pathophysiology and mechanisms of nonalcoholic fatty liver disease. Annu Rev Physiol. (2016) 78:181–205. doi: 10.1146/annurev-physiol-021115-105331
37. Wu J, Wang K, Wang X, Pang Y, Jiang C. The role of the gut microbiome and its metabolites in metabolic diseases. Protein Cell. (2021) 12:360–73. doi: 10.1007/s13238-020-00814-7
38. Quek J, Chan KE, Wong ZY, Tan C, Tan B, Lim WH, et al. Global prevalence of non-alcoholic fatty liver disease and non-alcoholic steatohepatitis in the overweight and obese population: a systematic review and meta-analysis. Lancet Gastroenterol Hepatol. (2023) 8:20–30. doi: 10.1016/S2468-1253(22)00317-X
39. Buzzetti E, Pinzani M, Tsochatzis EA. The multiple-hit pathogenesis of non-alcoholic fatty liver disease (NAFLD). Metabolism. (2016) 65:1038–48. doi: 10.1016/j.metabol.2015.12.012
40. Kitade H, Chen G, Ni Y, Ota T. Nonalcoholic fatty liver disease and insulin resistance: new insights and potential new treatments. Nutrients. (2017) 9:387. doi: 10.3390/nu9040387
41. Geng Y, Faber KN, de Meijer VE, Blokzijl H, Moshage H. How does hepatic lipid accumulation lead to lipotoxicity in non-alcoholic fatty liver disease? Hepatol Int. (2021) 15:21–35. doi: 10.1007/s12072-020-10121-2
42. Henry L, Paik J, Younossi ZM. Review article: the epidemiologic burden of non-alcoholic fatty liver disease across the world. Aliment Pharmacol Ther. (2022) 56:942–56. doi: 10.1111/apt.17158
43. Schindhelm RK, Heine RJ, Diamant M. Prevalence of nonalcoholic fatty liver disease and its association with cardiovascular disease among type 2 diabetic patients. Diabetes Care. (2007) 30:e94; author reply e95. doi: 10.2337/dc07-0982
44. Pafili K, Roden M. Nonalcoholic fatty liver disease (NAFLD) from pathogenesis to treatment concepts in humans. Mol Metab. (2021) 50:101122. doi: 10.1016/j.molmet.2020.101122
45. Watanabe T, Tsuchiya A, Takeuchi S, Nojiri S, Yoshida T, Ogawa M, et al. Development of a non-alcoholic steatohepatitis model with rapid accumulation of fibrosis, and its treatment using mesenchymal stem cells and their small extracellular vesicles. Regener Ther. (2020) 14:252–61. doi: 10.1016/j.reth.2020.03.012
46. Saleh FA, Jaber H, Eid A. Effect of Adipose derived mesenchymal stem cells on multiple Organ Injuries in diet-induced obese mice. Tissue Barriers. (2021) 9:1952150. doi: 10.1080/21688370.2021.1952150
47. Tacke F, Puengel T, Loomba R, Friedman SL. An integrated view of anti-inflammatory and antifibrotic targets for the treatment of NASH. J Hepatol. (2023) 79:552–66. doi: 10.1016/j.jhep.2023.03.038
48. Yano M, Nasti A, Seki A, Ishida K, Yamato M, Inui H, et al. Characterization of adipose tissue-derived stromal cells of mice with nonalcoholic fatty liver disease and their use for liver repair. Regener Ther. (2021) 18:497–507. doi: 10.1016/j.reth.2021.11.005
49. Domingues CC, Kundu N, Kropotova Y, Ahmadi N, Sen S. Antioxidant-upregulated mesenchymal stem cells reduce inflammation and improve fatty liver disease in diet-induced obesity. Stem Cell Res Ther. (2019) 10:280. doi: 10.1186/s13287-019-1393-8
50. Afarin R, Aslani F, Asadizade S, Jaberian Asl B, Mohammadi Gahrooie M, Shakerian E, et al. The effect of lipopolysaccharide-stimulated adipose-derived mesenchymal stem cells on NAFLD treatment in high-fat diet-fed rats. Iran J Pharm Res. (2023) 22:e134807. doi: 10.5812/ijpr-134807
51. Li B, Cheng Y, Yu S, Zang L, Yin Y, Liu J, et al. Human umbilical cord-derived mesenchymal stem cell therapy ameliorates nonalcoholic fatty liver disease in obese type 2 diabetic mice. Stem Cells Int. (2019) 2019:8628027. doi: 10.1155/2019/8628027
52. Ren Q, Sun Q, Fu J. Dysfunction of autophagy in high-fat diet-induced non-alcoholic fatty liver disease. Autophagy. (2024) 20:221–41. doi: 10.1080/15548627.2023.2254191
53. Liang C, Gao S, Gao J, Xu Y, Li Q. Comparison of effects of HucMSCs, exosomes, and conditioned medium on NASH. Sci Rep. (2023) 13:18431. doi: 10.1038/s41598-023-45828-3
54. Hu J, Li S, Zhong X, Wei Y, Sun Q, Zhong L. Human umbilical cord mesenchymal stem cells attenuate diet-induced obesity and NASH-related fibrosis in mice. Heliyon. (2024) 10:e25460. doi: 10.1016/j.heliyon.2024.e25460
55. Xu X, Wang W, Lin L, Chen P. Liraglutide in combination with human umbilical cord mesenchymal stem cell could improve liver lesions by modulating TLR4/NF-kB inflammatory pathway and oxidative stress in T2DM/NAFLD rats. Tissue Cell. (2020) 66:101382. doi: 10.1016/j.tice.2020.101382
56. Ezquer M, Ezquer F, Ricca M, Allers C, Conget P. Intravenous administration of multipotent stromal cells prevents the onset of non-alcoholic steatohepatitis in obese mice with metabolic syndrome. J Hepatol. (2011) 55:1112–20. doi: 10.1016/j.jhep.2011.02.020
57. Lebeaupin C, Vallée D, Hazari Y, Hetz C, Chevet E, Bailly-Maitre B. Endoplasmic reticulum stress signalling and the pathogenesis of non-alcoholic fatty liver disease. J Hepatol. (2018) 69:927–47. doi: 10.1016/j.jhep.2018.06.008
58. Li L, Zeng X, Liu Z, Chen X, Li L, Luo R, et al. Mesenchymal stromal cells protect hepatocytes from lipotoxicity through alleviation of endoplasmic reticulum stress by restoring SERCA activity. J Cell Mol Med. (2021) 25:2976–93. doi: 10.1111/jcmm.16338
59. Bi Y, Guo X, Zhang M, Zhu K, Shi C, Fan B, et al. Bone marrow derived-mesenchymal stem cell improves diabetes-associated fatty liver via mitochondria transformation in mice. Stem Cell Res Ther. (2021) 12:602. doi: 10.1186/s13287-021-02663-5
60. Nickel S, Christ M, Schmidt S, Kosacka J, Kühne H, Roderfeld M, et al. Human mesenchymal stromal cells resolve lipid load in high fat diet-induced non-alcoholic steatohepatitis in mice by mitochondria donation. Cells. (2022) 11:1829. doi: 10.3390/cells11111829
61. Wang H, Zhang H, Huang B, Miao G, Yan X, Gao G, et al. Mesenchymal stem cells reverse high−fat diet−induced non−alcoholic fatty liver disease through suppression of CD4+ T lymphocytes in mice. Mol Med Rep. (2018) 17:3769–74. doi: 10.3892/mmr.2017.8326
62. Wang H, Wang D, Yang L, Wang Y, Jia J, Na D, et al. Compact bone-derived mesenchymal stem cells attenuate nonalcoholic steatohepatitis in a mouse model by modulation of CD4 cells differentiation. Int Immunopharmacol. (2017) 42:67–73. doi: 10.1016/j.intimp.2016.11.012
63. Du J, Jiang Y, Liu X, Ji X, Xu B, Zhang Y, et al. HGF secreted by menstrual blood-derived endometrial stem cells ameliorates non-alcoholic fatty liver disease through downregulation of hepatic rnf186. Stem Cells. (2023) 41:153–68. doi: 10.1093/stmcls/sxac091
64. Niu Q, Wang T, Wang Z, Wang F, Huang D, Sun H, et al. Adipose-derived mesenchymal stem cell-secreted extracellular vesicles alleviate non-alcoholic fatty liver disease via delivering miR-223-3p. Adipocyte. (2022) 11:572–87. doi: 10.1080/21623945.2022.2098583
65. Togliatto G, Dentelli P, Gili M, Gallo S, Deregibus C, Biglieri E, et al. Obesity reduces the pro-angiogenic potential of adipose tissue stem cell-derived extracellular vesicles (EVs) by impairing miR-126 content: impact on clinical applications. Int J Obes (Lond). (2016) 40:102–11. doi: 10.1038/ijo.2015.123
66. Baranova A, Maltseva D, Tonevitsky A. Adipose may actively delay progression of NAFLD by releasing tumor-suppressing, anti-fibrotic miR-122 into circulation. Obes Rev. (2019) 20:108–18. doi: 10.1111/obr.12765
67. Sinha RA, Rajak S, Singh BK, Yen PM. Hepatic lipid catabolism via PPARα-lysosomal crosstalk. Int J Mol Sci. (2020) 21:2391. doi: 10.3390/ijms21072391
68. Anwar SD, Foster C, Ashraf A. Lipid disorders and metabolic-associated fatty liver disease. Endocrinol Metab Clin North Am. (2023) 52:445–57. doi: 10.1016/j.ecl.2023.01.003
69. Yang F, Wu Y, Chen Y, Xi J, Chu Y, Jin J, et al. Human umbilical cord mesenchymal stem cell-derived exosomes ameliorate liver steatosis by promoting fatty acid oxidation and reducing fatty acid synthesis. JHEP Rep. (2023) 5:100746. doi: 10.1016/j.jhepr.2023.100746
70. Cheng L, Yu P, Li F, Jiang X, Jiao X, Shen Y, et al. Human umbilical cord-derived mesenchymal stem cell-exosomal miR-627-5p ameliorates non-alcoholic fatty liver disease by repressing FTO expression. Hum Cell. (2021) 34:1697–708. doi: 10.1007/s13577-021-00593-1
71. Kang Y, Song Y, Luo Y, Song J, Li C, Yang S, et al. Exosomes derived from human umbilical cord mesenchymal stem cells ameliorate experimental non-alcoholic steatohepatitis via Nrf2/NQO-1 pathway. Free Radic Biol Med. (2022) 192:25–36. doi: 10.1016/j.freeradbiomed.2022.08.037
72. Du X, Li H, Han X, Ma W. Mesenchymal stem cells-derived exosomal miR-24-3p ameliorates non-alcohol fatty liver disease by targeting Keap-1. Biochem Biophys Res Commun. (2022) 637:331–40. doi: 10.1016/j.bbrc.2022.11.012
73. Sani F, Soufi Zomorrod M, Azarpira N, Soleimani M. The effect of mesenchymal stem cell-derived exosomes and miR17-5p inhibitor on multicellular liver fibrosis microtissues. Stem Cells Int. (2023) 2023:8836452. doi: 10.1155/2023/8836452
74. He Q, Wang L, Zhao R, Yan F, Sha S, Cui C, et al. Retraction Note: Mesenchymal stem cell-derived exosomes exert ameliorative effects in type 2 diabetes by improving hepatic glucose and lipid metabolism via enhancing autophagy. Stem Cell Res Ther. (2022) 13:505. doi: 10.1186/s13287-022-03191-6
75. Tawfeek GA-E, Kasem HA. Curcumin preconditioned mesenchymal stem cells derived exosomes transplantation ameliorate and protect against non- alcoholic steatohepatitis by regulation the expression of key genes of inflammation and oxidative stress. Transpl Immunol. (2023) 78:101837. doi: 10.1016/j.trim.2023.101837
76. Shi H, Hao X, Sun Y, Zhang H, Zhao Y, Wang B, et al. Bone marrow mesenchymal stem cell-derived exosomes reduce insulin resistance and obesity in mice via the PI3K/AKT signaling pathway. FEBS Open Bio. (2023) 13:1015–26. doi: 10.1002/2211-5463.13615
77. El-Derany MO, AbdelHamid SG. Upregulation of miR-96-5p by bone marrow mesenchymal stem cells and their exosomes alleviate non-alcoholic steatohepatitis: Emphasis on caspase-2 signaling inhibition. Biochem Pharmacol. (2021) 190:114624. doi: 10.1016/j.bcp.2021.114624
78. Chang C-Y, Chen K-Y, Shih H-J, Chiang M, Huang I-T, Huang Y-H, et al. Let-7i-5p mediates the therapeutic effects of exosomes from human placenta choriodecidual membrane-derived mesenchymal stem cells on mitigating endotoxin-induced mortality and liver injury in high-fat diet-induced obese mice. Pharm (Basel). (2021) 15:36. doi: 10.3390/ph15010036
79. Wu M, Zhang R, Zou Q, Chen Y, Zhou M, Li X, et al. Comparison of the biological characteristics of mesenchymal stem cells derived from the human placenta and umbilical cord. Sci Rep. (2018) 8:5014. doi: 10.1038/s41598-018-23396-1
80. Wilson A, Hodgson-Garms M, Frith JE, Genever P. Multiplicity of mesenchymal stromal cells: finding the right route to therapy. Front Immunol. (2019) 10:1112. doi: 10.3389/fimmu.2019.01112
81. Takakura Y, Hanayama R, Akiyoshi K, Futaki S, Hida K, Ichiki T, et al. Quality and safety considerations for therapeutic products based on extracellular vesicles. Pharm Res. (2024) 41:1573–94. doi: 10.1007/s11095-024-03757-4
82. Korkida F, Stamatopoulou A, Roubelakis MG. Recent advances in mesenchymal stem/stromal cell-based therapy for alcohol-associated liver disease and non-alcoholic fatty liver disease. Stem Cells Transl Med. (2024) 13:107–15. doi: 10.1093/stcltm/szad082
83. Zhao L, Chen S, Shi X, Cao H, Li L. A pooled analysis of mesenchymal stem cell-based therapy for liver disease. Stem Cell Res Ther. (2018) 9:72. doi: 10.1186/s13287-018-0816-2
84. Jiang Y, Yusoff NM, Du J, Moses EJ, Lin J-T. Current perspectives on mesenchymal stem cells as a potential therapeutic strategy for non-alcoholic fatty liver disease. World J Stem Cells. (2024) 16:760–72. doi: 10.4252/wjsc.v16.i7.760
85. Luo F, Sun Z, Han Q, Xue C, Bai C. Effect of human hepatocellular carcinoma hepG2 cell-derived exosome on the differentiation of mesenchymal stem cells and their interaction. Zhongguo Yi Xue Ke Xue Yuan Xue Bao. (2017) 39:312–7. doi: 10.3881/j.issn.1000-503X.2017.03.003
86. Song Y, Liang F, Tian W, Rayhill E, Ye L, Tian X. Optimizing therapeutic outcomes: preconditioning strategies for MSC-derived extracellular vesicles. Front Pharmacol. (2025) 16:1509418. doi: 10.3389/fphar.2025.1509418
87. Kassem DH, Kamal MM. Therapeutic efficacy of umbilical cord-derived stem cells for diabetes mellitus: a meta-analysis study. Stem Cell Res Ther. (2020) 11:484. doi: 10.1186/s13287-020-01996-x
88. Huang W-C, Li Y-C, Chen P-X, Ma KS-K, Wang L-T. Mesenchymal stem cell therapy as a game-changer in liver diseases: review of current clinical trials. Stem Cell Res Ther. (2025) 16:3. doi: 10.1186/s13287-024-04127-y
89. Li L, Liu Y, Wang K, Mo J, Weng Z, Jiang H, et al. Stem cell exosomes: new hope and future potential for relieving liver fibrosis. Clin Mol Hepatol. (2024). doi: 10.3350/cmh.2024.0854
90. Zhu X, Gao M, Yang Y, Li W, Bao J, Li Y. The CRISPR/cas9 system delivered by extracellular vesicles. Pharmaceutics. (2023) 15:984. doi: 10.3390/pharmaceutics15030984
91. Shakerian N, Darzi-Eslam E, Afsharnoori F, Bana N, Noorabad Ghahroodi F, Tarin M, et al. Therapeutic and diagnostic applications of exosomes in colorectal cancer. Med Oncol. (2024) 41:203. doi: 10.1007/s12032-024-02440-3
Keywords: metabolic dysfunction-associated steatotic liver disease, metabolic dysfunction-associated steatohepatitis, mesenchymal stem cells, exosomes, therapy
Citation: Qin D, Huang P, Chen J, Wu C and Liang Y (2025) The therapeutic potential of different mesenchymal stem cells and their derived exosomes in metabolic dysfunction-associated steatotic liver disease. Front. Endocrinol. 16:1558194. doi: 10.3389/fendo.2025.1558194
Received: 09 January 2025; Accepted: 14 March 2025;
Published: 03 April 2025.
Edited by:
Takefumi Kimura, Shinshu University, JapanReviewed by:
Iraj Alipourfard, Polish Academy of Sciences, PolandCopyright © 2025 Qin, Huang, Chen, Wu and Liang. This is an open-access article distributed under the terms of the Creative Commons Attribution License (CC BY). The use, distribution or reproduction in other forums is permitted, provided the original author(s) and the copyright owner(s) are credited and that the original publication in this journal is cited, in accordance with accepted academic practice. No use, distribution or reproduction is permitted which does not comply with these terms.
*Correspondence: Yuzhen Liang, bGlhbmd5dXpoZW4yNkAxNjMuY29t
†These authors have contributed equally to this work and share first authorship
Disclaimer: All claims expressed in this article are solely those of the authors and do not necessarily represent those of their affiliated organizations, or those of the publisher, the editors and the reviewers. Any product that may be evaluated in this article or claim that may be made by its manufacturer is not guaranteed or endorsed by the publisher.
Research integrity at Frontiers
Learn more about the work of our research integrity team to safeguard the quality of each article we publish.