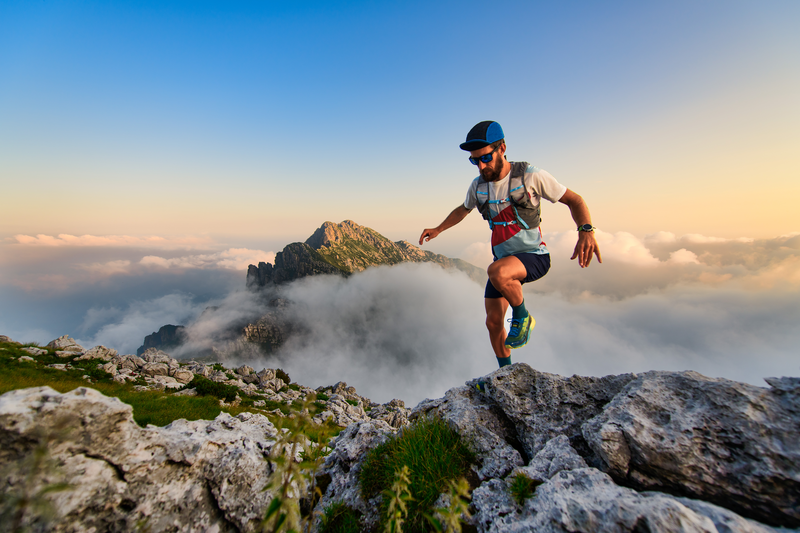
94% of researchers rate our articles as excellent or good
Learn more about the work of our research integrity team to safeguard the quality of each article we publish.
Find out more
CASE REPORT article
Front. Endocrinol.
Sec. Bone Research
Volume 16 - 2025 | doi: 10.3389/fendo.2025.1553032
This article is part of the Research Topic Phosphate handling: from cells to human View all 5 articles
The final, formatted version of the article will be published soon.
You have multiple emails registered with Frontiers:
Please enter your email address:
If you already have an account, please login
You don't have a Frontiers account ? You can register here
We report on a 3-year and 5-month-old boy who was referred for suspected rickets, due to knee valgus deformity developed over the previous year. The child had a history of epilepsy wellcontrolled with phenobarbital. His psychomotor development and growth metrics were appropriate for his age. On admission, laboratory work-up revealed elevated alkaline phosphatase (1289 U/L) and parathyroid hormone (PTH) (417 pg/ml), normal corrected calcium (9,3 mg/dl) and creatinine (0,21 mg/dl), low phosphate (3,2 mg/dl), 25-hydroxy vitamin D (6 ng/ml) and 1-25 hydroxy vitamin D (13.4 pg/mL, nv 20-80) concentrations. Urinalysis indicated low tubular reabsorption of phosphate (TRP % 10,7), along with bicarbonate, uric acid and amino acid loss, consistent with renal Fanconi syndrome. Based on these results, a genetic form of renal tubular dysfunction was suspected, and thus a clinical exome sequencing was requested. In the meanwhile, the child was commenced on Joulie solution (70 mg/kg/day of phosphate), calcitriol (0.03 mcg/kg/die), and ergocalciferol (1000 IU daily). FGF-23 concentrations were found to be within the normal range, thus ruling out FGF23dependent forms of rickets. Surprisingly, we observed a dramatic improvement in laboratory parameters within two weeks from the treatment initiation, including normalisation of phosphate and PTH concentrations and resolution of Fanconi syndrome, prompting discontinuation of phosphate supplements. Molecular analysis identified a de novo monoallelic mutation (C.1006+1 G>A) in the solute carrier family 34 member 1(SLC34A1) gene encoding a protein involved in actively transporting phosphate into cells via Na+ cotransport in the renal brush border membrane. However, even without phosphate substitution no further drops in serum phosphate concentrations and persistently normal proximal renal tubular function were observed. Moreover the rickets changes had almost healed six months after starting vitamin D supplementation. This case provides further evidence that vitamin D deficiency may rarely cause renal Fanconi syndrome, reversible upon vitamin D replacement. This is particularly relevant in children with risk factors for vitamin D deficiency, including use of anticonvulsants.
Keywords: Hypophosphatemic rickets, Vitamin D Deficiency, Renal Fanconi syndrome, Nutritional rickets, Phosphate Word count: 2.492
Received: 29 Dec 2024; Accepted: 12 Mar 2025.
Copyright: © 2025 Improda, Rosanio, Annicchiarico Petruzzelli, Ambrosio, Malgieri, Mandato and Licenziati. This is an open-access article distributed under the terms of the Creative Commons Attribution License (CC BY). The use, distribution or reproduction in other forums is permitted, provided the original author(s) or licensor are credited and that the original publication in this journal is cited, in accordance with accepted academic practice. No use, distribution or reproduction is permitted which does not comply with these terms.
* Correspondence:
Nicola Improda, AORN Santobono-Pausilipon, Naples, Italy
Disclaimer: All claims expressed in this article are solely those of the authors and do not necessarily represent those of their affiliated organizations, or those of the publisher, the editors and the reviewers. Any product that may be evaluated in this article or claim that may be made by its manufacturer is not guaranteed or endorsed by the publisher.
Research integrity at Frontiers
Learn more about the work of our research integrity team to safeguard the quality of each article we publish.