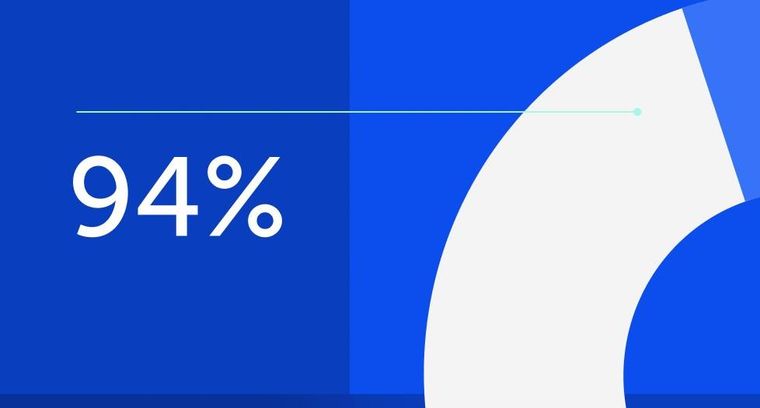
94% of researchers rate our articles as excellent or good
Learn more about the work of our research integrity team to safeguard the quality of each article we publish.
Find out more
ORIGINAL RESEARCH article
Front. Endocrinol., 08 April 2025
Sec. Clinical Diabetes
Volume 16 - 2025 | https://doi.org/10.3389/fendo.2025.1545393
Objective: This study aimed to investigate the effect of mid-pregnancy lipid levels on adverse outcomes in women with gestational diabetes mellitus (GDM) under adequate glycemic control. Whether this effect is independent of factors such as blood glucose was also analyzed.
Methods: We retrospectively analyzed 1,001 women with normal glucose tolerance (NGT) and 1,078 women with GDM under adequate glycemic control from 2015 to 2024. Logistic regression analysis was used to explore the relationship between blood lipids and adverse outcomes. Those with GDM were further classified according to their pre-pregnancy body mass index (BMI), gestational weight gain, glycosylated hemoglobin A1c (HbA1c), and fasting blood glucose (FBG). An interaction model between triglyceride (TG) and pre-pregnancy BMI, gestational weight gain, HbA1c, and FBG on adverse outcomes was constructed.
Results: In GDM, high levels of TG were independent risk factors for preeclampsia (OR = 1.51, 95%CI = 1.18–1.93), preterm birth (OR = 1.68, 95%CI = 1.30–2.18), macrosomia (OR = 1.48, 95%CI = 1.14–1.92), postpartum hemorrhage (OR = 1.33, 95%CI = 1.10–1.61), and intrauterine fetal distress (OR = 1.68, 95%CI = 1.13–2.51). Furthermore, TG had a greater impact on GDM women than on NGT women. In addition, in GDM, high levels of TG were independent risk factors for the above adverse outcomes in the subgroups of pre-pregnancy BMI, gestational weight gain, HbA1c, and FBG (interaction p > 0.05).
Conclusions: High levels of TG promoted the occurrence of preeclampsia, preterm birth, macrosomia, postpartum hemorrhage, and intrauterine fetal distress in women with GDM. Furthermore, TG had a greater effect on adverse outcomes in GDM than in NGT women.
Gestational diabetes mellitus (GDM), a condition in which carbohydrate intolerance first develops during pregnancy (1), severely affects pregnant women and infants, with a prevalence of 17.5% in China (2). GDM women and infants are at great risk of perinatal and long-term complications (3, 4). In light of this unfavorable condition, effective intervention strategies are urgently needed to prevent adverse outcomes in women with GDM.
The Hyperglycemia and Adverse Pregnancy Outcome study showed that hyperglycemia positively increases the risk of adverse pregnancy outcomes (5). However, some scholars found that, even if blood glucose control during pregnancy is within the normal range, the risk of adverse pregnancy outcomes is still high (6). Indeed, women with GDM often have a combination of other risk factors for adverse pregnancy outcomes, including advanced maternal age, obesity, and a family history of diabetes mellitus (7, 8). Recent evidence has shown that maternal dyslipidemia is associated with adverse pregnancy outcomes such as preterm birth, large for gestational age (LGA), macrosomia, and intrauterine fetal distress (9, 10). An appropriate increase in maternal triglyceride (TG) and total cholesterol (TC), considered as a physiological adaptation, has a positive impact on the maintenance of pregnancy (11, 12). However, as a result of insulin resistance and placental hormones, women with GDM may easily progress to pathological hyperlipidemia compared with women with normal glucose tolerance (NGT) (13), resulting in numerous adverse outcomes.
Previous studies have shown that dyslipidemia is a risk factor for adverse outcomes such as macrosomia, cesarean section, and neonatal admission to the care unit in women with GDM (14, 15). The relationship between TG levels and pregnancy outcomes has also been investigated in GDM women under glycemic control, but mostly in terms of newborn weight and adiposity accumulation (16, 17). The effect of lipids on adverse pregnancy outcomes in women with GDM in relation to blood glucose remains unknown. Considering that the increase in lipid levels in early pregnancy is not evident, and that the study of lipids in late pregnancy has certain time constraints for clinicians to continuously monitor and intervene in a timely manner, in this study, mid-pregnancy lipids were selected as the study indicators (18).
Thus, women with GDM who had good glycemic control were selected to analyze the role of mid-pregnancy lipids in the development of adverse pregnancy outcomes. An interaction model was further constructed to explore whether the effect of lipids on adverse pregnancy outcomes was associated with other factors such as blood glucose.
A total of 2,079 pregnant women who were routinely examined and who delivered from 2015 to 2024 were retrospectively analyzed. The participants were divided into NGT and GDM according to the oral glucose tolerance test (OGTT). The eligibility criteria were as follows: 1) the diagnosis of GDM was made using the 75-g OGTT results at 24–28 weeks of gestation (fasting glucose ≥5.1 mmol/L and/or 1-h glucose ≥10.0 mmol/L and/or 2-h glucose ≥8.5 mmol/L, as recommended by the International Association of Diabetes and Pregnancy Study Groups) (19); 2) the enrolled women with GDM were those with good glycemic control during pregnancy (HbA1c <6% and self-monitored fasting blood glucose <5.3 mmol/L during late pregnancy) (20); and 3) women with natural conception singleton pregnancy. The exclusion criteria were as follows: 1) use of insulin therapy or hypoglycemic drugs; 2) use of drugs that may interfere with lipid metabolism; 3) women with pre-pregnancy diabetes; 4) overt diabetes during pregnancy; 5) multiple fetuses; 6) women with uterine abnormalities or reproductive tract infections; and 7) those with severe chronic diseases such as cardiovascular disease, abnormal liver and kidney function, infection, and mental illness.
Ultimately, 1,001 women with NGT and 1,078 women with GDM were recruited into the study. The study was performed in accordance with the Declaration of Helsinki and was carried out with the approval of the Institutional Ethics Committee of Drum Tower Hospital affiliated with Nanjing University Medical School (reference no. 2019-284-01).
Pregnancy data were collected through pregnancy cards and electronic medical records, which included the demographic characteristics, the laboratory test indicators, the blood glucose and lipid levels, and the adverse outcomes during pregnancy. The lipid levels were recorded during pregnancy weeks 20–24. The HbA1c levels were recorded at 28–34 weeks of pregnancy. The OGTT was performed at 24–28 weeks of pregnancy. Adverse pregnancy outcomes consisted of preeclampsia, preterm birth, macrosomia, low birth weight (LBW), LGA, small for gestational age (SGA), postpartum hemorrhage, intrauterine fetal distress, and neonatal infection.
Preeclampsia is characterized by the onset of hypertension (systolic blood pressure of 140 mmHg or higher or diastolic blood pressure of 90 mmHg or higher on two occasions at least 4 h apart) and proteinuria or in the absence of proteinuria but with the end-organ dysfunction after 20 weeks of gestation (21). Preterm birth is defined as delivery at less than 37 weeks’ gestational age (22). Macrosomia is defined as growth weight beyond 4,000 g or 4,500 g (23). LBW refers to a birth weight less than 2,500 g (24). LGA refers to a birth weight equal to or more than the 90th percentile for a given gestational age, while SGA refers to a birth weight equal to or less than the 10th percentile for a given gestational age (25).
Continuous variables were summarized as the mean ± SD or median (interquartile range), while categorical variables were expressed as number of cases (percentage). Continuous variables between the two groups were analyzed using Student’s t test or the Mann–Whitney U test, while categorical variables were analyzed using Pearson’s chi-squared test or Fisher’s exact test. Multivariate logistic regression analysis was used to estimate the incidence of adverse outcomes using odds ratios (ORs) and 95% confidence intervals (CIs). Moreover, the interaction model was utilized to explore the relationship between the lipid levels and adverse pregnancy outcomes. A p < 0.05 was considered statistically significant. IBM SPSS 26.0 and Empower Stats statistical software were used for the data processing and analysis in this study.
Table 1 shows that GDM women were older and had higher pre-pregnancy BMI, lower gestational weight gain, and higher rate of family history of diabetes than NGT women (p < 0.05). For previous miscarriages, there were no statistical differences between NGT and GDM (p > 0.05).
Furthermore, compared with NGT women, GDM women had higher levels of TG [2.18 (1.74–2.84) vs. 1.82 (1.44–2.33) mmol/L, p < 0.001], TC [5.52 (4.85–6.20) vs. 5.41 (4.87–5.98) mmol/L, p = 0.030], and LDL-C [2.71 (2.25–3.28) vs. 2.63 (2.16–3.10) mmol/L, p = 0.001] and lower levels of HDL-C [1.88 (1.57–2.19) vs. 2.00 (1.72–2.30) mmol/L, p < 0.001]. GDM women had lower glutamic–oxaloacetic transaminase (aspartate aminotransferase, AST) levels. There were no statistical differences with respect to glutamic–pyruvic transaminase (alanine aminotransferase, ALT), kidney function, and electrolytes between the two groups.
Table 2 shows the data of NGT and GDM women divided into two groups according to the occurrence of adverse outcomes. With regard to the clinical characteristics of NGT women, there were no statistically significant differences in age, pre-pregnancy BMI, gestational weight gain, and previous miscarriages between the groups with and without adverse outcomes. NGT women with adverse outcomes had a higher rate of a family history of diabetes compared with the group without adverse outcomes. In terms of the lipid levels in mid-pregnancy, NGT with adverse outcomes showed higher TG [1.90 (1.51–2.57) vs. 1.80 (1.42–2.24) mmol/L, p = 0.004] and lower HDL-C [1.97 (1.67–2.24) vs. 2.02 (1.74–2.32) mmol/L, p = 0.020] levels. The levels of TC and LDL-C showed no statistically significant differences between the two groups. In addition, NGT with adverse outcomes had higher levels of ALT and AST. The blood glucose values in the OGTT results, the kidney function, and electrolytes were not determined between the two groups in women with NGT.
With regard to the clinical characteristics of GDM women, the group with adverse outcomes had higher pre-pregnancy BMI and gestational weight gain than the group without adverse outcomes. The two groups showed no significant differences in age, previous miscarriages, and family history of diabetes. For the lipid levels in mid-pregnancy, GDM with adverse outcomes had higher TG levels [2.31 (1.80–2.96) vs. 2.13 (1.71–2.71) mmol/L, p = 0.002] compared with GDM without adverse outcomes. Among the GDM women, TC, LDL-C, and HDL-C showed no significant differences in the two groups. GDM with adverse outcomes had higher FBG levels [4.63 (4.35–5.12) vs. 4.58 (4.29–5.05) mmol/L, p = 0.047] than GDM without adverse outcomes. In GDM, the two groups showed no significant differences in terms of other laboratory indicators.
To compare the differences in the impact of blood lipids on adverse pregnancy outcomes between NGT and GDM women and to analyze whether the effect of blood lipids on adverse pregnancy outcomes in GDM is associated with blood glucose or other factors, multivariate logistic regression analyses on the lipids in mid-pregnancy and the risk of adverse outcomes were performed. The results are given in Table 3. Moreover, three regression models including different covariates were constructed, and the difference between GDM 1 (ORs adjusted for pre-pregnancy BMI and gestational weight gain) and GDM 2 (ORs adjusted for pre-pregnancy BMI, gestational weight gain, and FBG) lies in the adjustment for FBG. The results showed that, compared with that in NGT women, elevated TG levels in GDM women had greater impact on preeclampsia [OR = 1.51 (95% CI = 1.18–1.93) vs. OR = 1.31 (95%CI = 0.90–1.90)], preterm birth [OR = 1.68 (95%CI = 1.30–2.18) vs. OR = 1.45 (95%CI = 1.02–2.06)], macrosomia [OR = 1.48 (95%CI = 1.14–1.92) vs. OR = 1.48 (95%CI = 1.11–1.98)], postpartum hemorrhage [OR = 1.33 (95%CI = 1.10–1.61) vs. OR = 0.93 (95%CI = 0.73–1.18)], and intrauterine fetal distress [OR = 1.68 (95%CI = 1.13–2.51) vs. OR = 1.58 (95%CI = 0.79–3.19)]. This significant effect still existed after adjusting for FBG.
The results showed that TG was significantly positively correlated with pre-pregnancy BMI (R = 0.273, p < 0.001), HbA1c (R = 0.140, p < 0.001), and FBG (R = 0.186, p < 0.001) and negatively correlated with gestational weight gain (R = −0.089, p = 0.001) in GDM women (Figure 1).
Figure 1. Pearson’s correlation analysis between triglyceride (TG) and various other factors in women with gestational diabetes mellitus (GDM). (A) Scatter plot of TG and gestational weight gain. TG was significantly negatively correlated with gestational weight gain (R = −0.089, p = 0.001). (B) Scatter plot of TG and pre-pregnancy BMI. TG was significantly positively correlated with pre-pregnancy BMI (R = 0.273, p < 0.001). (C) Scatter plot of TG and HbA1c. TG was significantly positively correlated with HbA1c (R = 0.140, p < 0.001). (D) Scatter plot of TG and FBG. TG was significantly positively correlated with FBG (R = 0.186, p < 0.001). R, relevant coefficients; BMI, body mass index; FBG, fasting blood glucose; HbA1c, glycosylated hemoglobin A1c.
Figure 1 shows that the TG levels were associated with pre-pregnancy BMI, gestational weight gain, HbA1c, and FBG. On this basis, the women with GDM were grouped by pre-pregnancy BMI, gestational weight gain, HbA1c, and FBG (Figures 2-1, 2-2). In addition, a model was constructed to investigate the interaction between pre-pregnancy BMI, gestational weight gain, HbA1c, and FBG and the effect of TG on adverse pregnancy outcomes. The results showed that there was no interaction between the effect of TG on adverse pregnancy outcomes and pre-pregnancy BMI, gestational weight gain, HbA1c, and FBG, with the interaction p-value >0.05.
Figure 2. Analysis of the interaction between triglyceride (TG) and various factors in gestational diabetes mellitus (GDM) women. (1, 2) Analysis of the interaction between TG and various other factors in women with GDM. Adjusted odds ratios were adjusted for pre-pregnancy BMI, gestational weight gain, and FBG. The p-value for interaction was >0.05. There was no significant distinction in the impact of TG on adverse pregnancy outcomes between individuals with different levels of pre-pregnancy BMI (<24 or ≥24 kg/m2), gestational weight gain (appropriate or inappropriate), HbA1c (<5.2% or ≥5.2%), and FBG (<5.1 or ≥5.1 mmol/L). BMI, body mass index; FBG, fasting blood glucose; HbA1c, glycosylated hemoglobin A1c.
In this retrospective study, it was observed that GDM women with good blood glucose control appeared to have higher lipid levels than NGT women. Furthermore, elevated TG levels during mid-pregnancy were positively associated with adverse outcomes. Notably, GDM women experienced a greater effect of the TG levels on adverse outcomes than NGT women. This suggests that, for women with GDM, on the basis of managing blood glucose, attention should also be paid to the blood lipids, particularly to the levels of TG.
There are several factors that cause elevated blood lipids during pregnancy. Due to the special metabolic state during pregnancy, the blood lipid levels of pregnant women show dynamic changes. Compared with those in non-pregnant individuals, the blood lipids levels during pregnancy may increase physiologically (26). The results of this study showed that GDM women had higher levels of TG and TC compared with NGT women, which is consistent with previous studies (27, 28). Yazıcı et al. demonstrated that increased lipid levels and an altered intracellular signaling metabolism are associated with insulin resistance (29). As a result of insulin resistance, the main sources of TG in the liver are abnormally elevated (13). Therefore, pathological hyperlipidemia is more likely to occur among GDM women. Moreover, owing to the widespread application of assisted reproductive practice such as intrauterine insemination and autologous platelet-rich plasma, women undergoing these procedures may face physiological changes (30, 31). Research shows that women who have received assisted reproduction may experience ovarian stimulation and changes in hormone levels. This could exacerbate insulin resistance and cause disturbances in the glucose and lipid metabolism among pregnancies and the offspring (32, 33). Hence, in this study, women who received assisted reproduction were excluded.
Previous studies have shown that dyslipidemia is a risk factor for preterm birth, macrosomia, and preeclampsia (34, 35). Similarly, we found that the TG levels in the group with adverse outcomes were higher than those in the group without adverse outcomes in both NGT and GDM women. A number of scholars have noted that the TG levels in GDM women are associated with macrosomia and preeclampsia and that the effect is more significant than that in NGT women (14). However, the aforementioned study did not take into account the relationship between the impact of lipids on adverse outcomes and the blood glucose factors. The American Diabetes Association (ADA) recommends an ideal HbA1c target of <6.0% in the absence of hypoglycemia (20). In order to investigate whether glycemic factors are involved in the effect of lipids on adverse pregnancy outcomes in women with GDM, the included GDM population all met the glycemic control target. The logistic regression analysis revealed that, after adjustment for glycemic factors (GDM 2), TG remained an independent risk factor for preeclampsia, preterm birth, macrosomia, postpartum hemorrhage, and intrauterine fetal distress. Furthermore, consistent with the study by Shi et al. (14), it was found that, compared with that in NGT women, the effect of TG on the aforementioned adverse outcomes was more pronounced in GDM women. The development of preeclampsia is influenced by numerous factors, and we have considered the effect of pre-pregnancy BMI, gestational weight gain, and blood glucose via the nadir criteria and multifactorial analyses. Currently, there is insufficient evidence to explain the mechanism of preeclampsia caused by TG. Chen et al. (36) established a preeclampsia mouse model using hypoxia-inducible factor 1α and found high levels of blood lipids and urinary protein in the mouse placenta, accompanied by mitochondrial dysfunction. After improvement of mitochondrial function, the blood lipids and urinary protein levels decreased accordingly, hinting that placental mitochondrial dysfunction may be involved in the occurrence and the development of preeclampsia caused by blood lipids. Several studies have indicated that an abnormally elevated TG increases blood viscosity and the risk of thrombosis, leading to inadequate placental perfusion, nutrient transfer disorder, fetal ischemia, and hypoxia. As a result, high levels of TG induce preterm birth and intrauterine fetal distress (37, 38).
A summary of 46 studies revealed that high maternal TG and low HDL-C increased the risk of macrosomia (39). This may be attributed to the point that placental lipase hydrolyzes TG into fatty acids, which in turn leads to the abnormal accumulation of fetal fat and to excessive growth (40, 41). However, an association between HDL-C and macrosomia was not found, which may be due to differences in the distribution of population characteristics.
It was found that TG was associated with gestational weight gain, pre-pregnancy BMI, HbA1c, and FBG. In order to further explore whether the effect of TG on adverse pregnancy outcomes was associated with the above factors, interaction analyses based on the subgroups were performed. There was no interaction observed between TG and gestational weight gain, pre-pregnancy BMI, HbA1c, and FBG (interaction p-value >0.05), indicating that the impact of TG on adverse pregnancy outcomes was not affected by the above factors. There was no significant distinction in the impact of TG on adverse pregnancy outcomes between individuals with different levels of pre-pregnancy BMI (<24 or ≥24 kg/m2), gestational weight gain (appropriate or inappropriate), HbA1c (<5.2% or ≥5.2%), and FBG (<5.1 or ≥5.1 mmol/L). This further suggests that TG had an independent effect on adverse pregnancy outcomes. Hence, even for individuals with relatively suitable levels of pre-pregnancy BMI, gestational weight gain, HbA1c, and FBG, attention should still be paid to the management of lipids. Indeed, a meta-analysis demonstrated that high levels of TG have a more significant impact on macrosomia in pregnant women who are overweight or obese before pregnancy (39). In this study, the ORs of macrosomia in GDM pregnant women with pre-pregnancy BMI ≥24 kg/m2 were higher than those in pregnant women with BMI <24 kg/m2; however, there were no statistically significant differences in the interaction between the groups. We speculate that the difference in the results may be related to the limitation in the sample size.
This study has several limitations. Firstly, the blood lipids indicators did not include free fatty acids. Secondly, although we adjusted for blood glucose factors in women with GDM, there are still other unknown confounding factors. Finally, considering that the lipid levels are related to dietary habits and regional distribution and this study only included the population of the Nanjing area, further research encompassing wider areas should be performed.
In summary, this study found that high levels of TG are independent risk factors for preeclampsia, preterm birth, macrosomia, postpartum hemorrhage, and intrauterine fetal distress. In addition, TG had a greater impact on GDM than on NGT women. Clinicians should monitor the blood lipid levels early and dynamically in women with GDM and to recommend timely lifestyle interventions, which can help reduce the incidence of adverse outcomes (42).
The raw data supporting the conclusions of this article will be made available by the authors, without undue reservation.
The studies involving humans were approved by the institutional ethics committee of Drum Tower Hospital affiliated with Nanjing University Medical School. The studies were conducted in accordance with the local legislation and institutional requirements. Written informed consent for participation was not required from the participants or the participants’ legal guardians/next of kin in accordance with the national legislation and institutional requirements.
RZ: Writing – original draft, Data curation, Formal Analysis, Investigation, Methodology, Writing – review & editing. JH: Data curation, Writing – original draft, Formal Analysis. YL: Data curation, Writing – original draft, Investigation. XC: Data curation, Writing – original draft. QW: Data curation, Writing – original draft. TW: Data curation, Writing – original draft. WZ: Writing – review & editing. YB: Writing – review & editing. SS: Writing – review & editing. ZG: Supervision, Writing – review & editing, Conceptualization.
The author(s) declare that financial support was received for the research and/or publication of this article. This work was supported by the National Natural Science Foundation of China Grant Awards (82030026, 82270883, 82370841, 82374554, 82270887, 81970689, 82070837, 81970704, 81900787, 82000735, 82000775, 82300877, 82303721, 82200924, and 82100868); the National Key Research and Development Program of China (2021YFC2501600 and 2022YFC2505306); the Jiangsu Provincial Medical Key Discipline Cultivation Unit (JSDW202201); the Key Project of Nanjing Clinical Medical Science, the Key Research and Development Program of Jiangsu Province of China (BE2022666); the Scientific Research Project of the Sixth Phase of “333 Project” of Jiangsu Province of China, the Science and Technology Plan Project of Ili Kazak Autonomous Prefecture of Xinjiang Uyghur Autonomous Region of China (YJC2023A35); and Fundings for Clinical Trials from the Affiliated Drum Tower Hospital, Medical School of Nanjing University (2022-LCYJ-ZD-03 and 2021-LCYJ-ZD-04).
The authors gratefully acknowledge the invaluable contributions of doctors in Nanjing Drum Tower Hospital.
The authors declare that the research was conducted in the absence of any commercial or financial relationships that could be construed as a potential conflict of interest.
The author(s) declare that no Generative AI was used in the creation of this manuscript.
All claims expressed in this article are solely those of the authors and do not necessarily represent those of their affiliated organizations, or those of the publisher, the editors and the reviewers. Any product that may be evaluated in this article, or claim that may be made by its manufacturer, is not guaranteed or endorsed by the publisher.
1. American Diabetes Association. 14. Management of diabetes in pregnancy: standards of medical care in diabetes. Diabetes Care. (2021) 44:S200–10. doi: 10.2337/dc21-S014
2. Zhu W, Yang H, Wei Y, Wang Z, Li X, Wu H, et al. Comparing the diagnostic criteria for gestational diabetes mellitus of World Health Organization 2013 with 1999 in Chinese population. Chin Med J. (2015) 128:125–7. doi: 10.4103/0366-6999.147858
3. Sweeting A, Wong J, Murphy HR, Ross GPA. Clinical update on gestational diabetes mellitus. Endocrine Rev. (2022) 43:763–93. doi: 10.1210/endrev/bnac003
4. Ye W, Luo C, Huang J, Li C, Liu Z, Liu F. Gestational diabetes mellitus and adverse pregnancy outcomes: systematic review and meta-analysis. BMJ (Clinical Res ed.). (2022) 377:e067946. doi: 10.1136/bmj-2021-067946
5. HAPO Study Cooperative Research Group, Metzger BE, Lowe LP, Dyer AR, Trimble ER, Chaovarindr U, et al. Hyperglycemia and adverse pregnancy outcomes. New Engl J Med. (2008) 358:1991–2002. doi: 10.1056/NEJMoa0707943
6. Jensen DM, Damm P, Sørensen B, Mølsted-Pedersen L, Westergaard JG, Klebe J, et al. Clinical impact of mild carbohydrate intolerance in pregnancy: a study of 2904 nondiabetic Danish women with risk factors for gestational diabetes mellitus. Am J obstetrics gynecology. (2001) 185:413–9. doi: 10.1067/mob.2001.115864
7. Zhang C, Ning Y. Effect of dietary and lifestyle factors on the risk of gestational diabetes: review of epidemiologic evidence. Am J Clin Nutr. (2011) 94:1975S–9S. doi: 10.3945/ajcn.110.001032
8. Sweeting AN, Wong J, Appelblom H, Ross GP, Kouru H, Williams PF, et al. A novel early pregnancy risk prediction model for gestational diabetes mellitus. Fetal diagnosis Ther. (2019) 45:76–84. doi: 10.1159/000486853
9. Kaneko K, Ito Y, Ebara T, Kato S, Matsuki T, Tamada H, et al. Association of maternal total cholesterol with SGA or LGA birth at term: the Japan environment and children's study. J Clin Endocrinol Metab. (2022) 107:e118–29. doi: 10.1210/clinem/dgab618
10. Ottun AT, Odunsi MA, Jinadu FO, Olumodeji AM, Tijani F, Olalere HF, et al. Maternal hyperlipidemia and spontaneous preterm delivery: a multi-centre cohort study. J Matern Fetal Neonatal Med. (2022) 35:8530–5. doi: 10.1080/14767058.2021.1988071
11. Waage CW, Mdala I, Stigum H, Jenum AK, Birkeland KI, Shakeel N, et al. Lipid and lipoprotein concentrations during pregnancy and associations with ethnicity. BMC pregnancy childbirth. (2022) 22:246. doi: 10.1186/s12884-022-04524-2
12. Ghio A, Bertolotto A, Resi V, Volpe L, Di Cianni G. Triglyceride metabolism in pregnancy. Adv Clin Chem. (2011) 55:133–53. doi: 10.1016/b978-0-12-387042-1.00007-1
13. Ginsberg HN, Zhang YL, Hernandez-Ono A. Regulation of plasma triglycerides in insulin resistance and diabetes. Arch Med Res. (2005) 36:232–40. doi: 10.1016/j.arcmed.2005.01.005
14. Shi P, Tang J, Yin X. Association between second- and third-trimester maternal lipid profiles and adverse perinatal outcomes among women with GDM and non-GDM: a retrospective cohort study. BMC pregnancy childbirth. (2023) 23:318. doi: 10.1186/s12884-023-05630-5
15. Li Y, Wang X, Jiang F, Chen W, Li J, Chen X. Serum lipid levels in relation to clinical outcomes in pregnant women with gestational diabetes mellitus: an observational cohort study. Lipids Health disease. (2021) 20:125. doi: 10.1186/s12944-021-01565-y
16. Ortega-Senovilla H, Schaefer-Graf U, Meitzner K, Abou-Dakn M, Herrera E. Decreased concentrations of the lipoprotein lipase inhibitor angiopoietin-like protein 4 and increased serum triacylglycerol are associated with increased neonatal fat mass in pregnant women with gestational diabetes mellitus. J Clin Endocrinol Metab. (2013) 98:3430–7. doi: 10.1210/jc.2013-1614
17. Schaefer-Graf UM, Graf K, Kulbacka I, Kjos SL, Dudenhausen J, Vetter K, et al. Maternal lipids as strong determinants of fetal environment and growth in pregnancies with gestational diabetes mellitus. Diabetes Care. (2008) 31:1858–63. doi: 10.2337/dc08-0039
18. Wild R, Feingold KR. Effect of Pregnancy on Lipid Metabolism and Lipoprotein Levels. Endotext. South Dartmouth (MA): MDText.com, Inc (2023).
19. International Association of Diabetes and Pregnancy Study Groups Consensus Panel, Metzger BE, Gabbe SG, Persson B, Buchanan TA, Catalano PA, et al. International association of diabetes and pregnancy study groups recommendations on the diagnosis and classification of hyperglycemia in pregnancy. Diabetes Care. (2010) 33:676–82. doi: 10.2337/dc09-1848
20. American Diabetes Association Professional Practice Committee. 15. Management of diabetes in pregnancy: standards of medical care in diabetes-2022. Diabetes Care. (2022) 45:S232–43. doi: 10.2337/dc22-S015
21. ACOG Practice Bulletin No. 202. Gestational hypertension and preeclampsia. Obstetrics gynecology. (2019) 133:1. doi: 10.1097/AOG.0000000000003018
22. Goldenberg RL, Culhane JF, Iams JD, Romero R. Epidemiology and causes of preterm birth. Lancet (London England). (2008) 371:75–84. doi: 10.1016/S0140-6736(08)60074-4
23. Macrosomia: ACOG Practice Bulletin, Number 216. Obstet Gynecol. (2020) 135:e18–35. doi: 10.1097/AOG.0000000000003606
24. He Z, Bishwajit G, Yaya S, Cheng Z, Zou D, Zhou Y. Prevalence of low birth weight and its association with maternal body weight status in selected countries in Africa: a cross-sectional study. BMJ Open. (2018) 8:e020410. doi: 10.1136/bmjopen-2017-020410
25. Dai L, Deng C, Li Y, Zhu J, Mu Y, Deng Y, et al. Birth weight reference percentiles for Chinese. PloS One. (2014) 9:e104779. doi: 10.1371/journal.pone.0104779
26. Neboh EE, Emeh JK, Aniebue UU, Ikekpeazu EJ, Maduka IC, Ezeugwu FO. Relationship between lipid and lipoprotein metabolism in trimesters of pregnancy in Nigerian women: Is pregnancy a risk factor? J Natural science biology Med. (2012) 3:32–7. doi: 10.4103/0976-9668.95944
27. Ryckman KK, Spracklen CN, Smith CJ, Robinson JG, Saftlas AF. Maternal lipid levels during pregnancy and gestational diabetes: a systematic review and meta-analysis. BJOG: an Int J obstetrics gynaecology. (2015) 122:643–51. doi: 10.1111/1471-0528.13261
28. Wang J, Li Z, Lin L. Maternal lipid profiles in women with and without gestational diabetes mellitus. Medicine. (2019) 98:e15320. doi: 10.1097/MD.0000000000015320
29. Yazıcı D, Sezer H. Insulin resistance, obesity and lipotoxicity. Adv Exp Med Biol. (2017) 960:277–304. doi: 10.1007/978-3-319-48382-5_12
30. Huniadi A, Bimbo-Szuhai E, Botea M, Zaha I, Beiusanu C, Pallag A, et al. Fertility predictors in intrauterine insemination (IUI). J Pers Med. (2023) 13:395. doi: 10.3390/jpm13030395
31. Zaha IA, Huniadi A, Bodog F, Seles L, Toma MC, Maghiar L, et al. Autologous platelet-rich plasma (PRP) in infertility-infusion versus injectable PRP. J Pers Med. (2023) 13:1676. doi: 10.3390/jpm13121676
32. Bals-Pratsch M, Grosser B, Seifert B, Ortmann O, Seifarth C. Early onset and high prevalence of gestational diabetes in PCOS and insulin resistant women before and after assisted reproduction. Exp Clin Endocrinol Diabetes. (2011) 119:338–42. doi: 10.1055/s-0030-1269883
33. Cui L, Zhou W, Xi B, Ma J, Hu J, Fang M, et al. Increased risk of metabolic dysfunction in children conceived by assisted reproductive technology. Diabetologia. (2020) 63:2150–7. doi: 10.1007/s00125-020-05241-1
34. Jin WY, Lin SL, Hou RL, Chen XY, Han T, Jin Y, et al. Associations between maternal lipid profile and pregnancy complications and perinatal outcomes: a population-based study from China. BMC pregnancy childbirth. (2016) 16:60. doi: 10.1186/s12884-016-0852-9
35. Xi F, Chen H, Chen Q, Chen D, Chen Y, Sagnelli M, et al. Second-trimester and third-trimester maternal lipid profiles significantly correlated to LGA and macrosomia. Arch gynecology obstetrics. (2021) 304:885–94. doi: 10.1007/s00404-021-06010-0
36. Chen G, Lin Y, Chen L, Zeng F, Zhang L, Huang Y, et al. Role of DRAM1 in mitophagy contributes to preeclampsia regulation in mice. Mol Med Rep. (2020) 22:1847–58. doi: 10.3892/mmr.2020.11269
37. Emet T, Ustüner I, Güven SG, Balık G, Ural UM, Tekin YB, et al. Plasma lipids and lipoproteins during pregnancy and related pregnancy outcomes. Arch gynecology obstetrics. (2013) 288:49–55. doi: 10.1007/s00404-013-2750-y
38. Scifres CM, Catov JM, Simhan HN. The impact of maternal obesity and gestational weight gain on early and mid-pregnancy lipid profiles. Obes (Silver Spring Md.). (2014) 22:932–8. doi: 10.1002/oby.20576
39. Wang J, Moore D, Subramanian A, Cheng KK, Toulis KA, Qiu X, et al. Gestational dyslipidaemia and adverse birthweight outcomes: a systematic review and meta-analysis. Obes reviews: an Off J Int Assoc Study Obes. (2018) 19:1256–68. doi: 10.1111/obr.12693
40. Chen Q, Chen H, Wang M, Qiu L, Xi F, Jiang Y, et al. The association between alteration of maternal lipid levels and birthweight at term: A within-family comparison. Front endocrinology. (2022) 13:989663. doi: 10.3389/fendo.2022.989663
41. Szabo AJ. Transferred maternal fatty acids stimulate fetal adipogenesis and lead to neonatal and adult obesity. Med hypotheses. (2019) 122:82–8. doi: 10.1016/j.mehy.2018.10.022
Keywords: lipids, gestational diabetes mellitus, adverse outcomes, risk factors, pregnancy
Citation: Zhao R, Hu J, Li Y, Chen X, Wang Q, Wu T, Zhou W, Bi Y, Shen S and Ge Z (2025) Influence of maternal lipid levels on adverse pregnancy outcomes in women with gestational diabetes mellitus. Front. Endocrinol. 16:1545393. doi: 10.3389/fendo.2025.1545393
Received: 14 December 2024; Accepted: 13 March 2025;
Published: 08 April 2025.
Edited by:
Ping Wang, Michigan State University, United StatesReviewed by:
Cosmin Mihai Vesa, University of Oradea, RomaniaCopyright © 2025 Zhao, Hu, Li, Chen, Wang, Wu, Zhou, Bi, Shen and Ge. This is an open-access article distributed under the terms of the Creative Commons Attribution License (CC BY). The use, distribution or reproduction in other forums is permitted, provided the original author(s) and the copyright owner(s) are credited and that the original publication in this journal is cited, in accordance with accepted academic practice. No use, distribution or reproduction is permitted which does not comply with these terms.
*Correspondence: Zhijuan Ge, Z2V6aGlqdWFuQG5qZ2x5eS5jb20=; Shanmei Shen, c2hhbm1laXNoZW5AMTI2LmNvbQ==; Yan Bi, Yml5YW5Abmp1LmVkdS5jbg==
†ORCID: Yan Bi, orcid.org/0000-0003-3914-7854
Shanmei Shen, orcid.org/0000-0003-4949-0162
Zhijuan Ge, orcid.org/0009-0009-4585-0985
Disclaimer: All claims expressed in this article are solely those of the authors and do not necessarily represent those of their affiliated organizations, or those of the publisher, the editors and the reviewers. Any product that may be evaluated in this article or claim that may be made by its manufacturer is not guaranteed or endorsed by the publisher.
Research integrity at Frontiers
Learn more about the work of our research integrity team to safeguard the quality of each article we publish.