- 1Department of Geriatrics, Shenzhen People’s Hospital (The Second Clinical Medical College, Jinan University; The First Affiliated Hospital, Southern University of Science and Technology; Shenzhen Clinical Research Center for Geriatrics; Guangdong Provincial Clinical Research Center for Geriatrics), Shenzhen, Guangdong, China
- 2Department of Neurology, Shenzhen People’s Hospital, Shenzhen, Guangdong, China
Metabolic syndrome (MetS) encompasses a cluster of interrelated conditions, including obesity, hyperglycemia, hyperlipidemia, and hypertension, and has been established as a significant risk factor for cardiovascular events and heightened mortality. At its core, insulin resistance serves as the primary underlying mechanism driving the development of MetS. The prevalence of MetS is rising at an alarming rate, posing a significant public health challenge worldwide. Even in the absence of overt obstructive coronary artery disease or valvular heart disease, patients with MetS often exhibit adverse cardiac remodeling and myocardial dysfunction. Left ventricular hypertrophy (LVH) and left ventricular diastolic dysfunction (LVDD) are the leading manifestations of heart failure with preserved ejection fraction (HFpEF). Abnormal myocardial substrate utilization, neurohormonal activation, interstitial fibrosis, coronary microvascular dysfunction, and metabolic inflammation have all been implicated in the development and progression of adverse cardiac remodeling associated with MetS. However, despite the tremendous research produced on this subject, HFpEF remains highly prevalent in such a population. The early diagnosis of abnormal cardiac remodeling would enable optimal effective therapies to prevent the progression of the disease to the symptomatic phase. HFpEF encompasses a diverse range of pathological processes. In these patients, LVDD and elevated left ventricular filling pressure are the primary manifestations. Echocardiography remains the popular imaging modality for the assessment of LVDD and LV filling pressure. The article aims to review recent articles covering the association between MetS components or MetS and LVDD in HFpEF.
1 Introduction
Metabolic syndrome (MetS) appears to be important in the development of left ventricular diastolic dysfunction (LVDD) and its progression towards heart failure with preserved ejection fraction (HFpEF), as the increasing prevalence of obesity, hypertension, chronic kidney disease, and diabetes is closely associated with the incidence of HFpEF. HFpEF is commonly defined as a condition in which a person with heart failure (HF) has a left ventricular (LV) ejection fraction (EF) of ≥50%. The European Society of Cardiology (ESC) provides a more detailed definition: HFpEF is characterized by symptoms and signs of heart failure, evidence of structural and/or functional cardiac abnormalities, and/or elevated natriuretic peptides (NPs), along with an LVEF of ≥50% (1). Findings from previous studies have suggested a novel paradigm. In the new paradigm, multiple comorbidities, including obesity, hypertension, hyperlipidemia, diabetes, and insulin resistance, cause a systemic pro-inflammatory state that leads to oxidative stress, endothelial dysfunction, ectopic fat accumulation, and coronary microvascular dysfunction. Eventually, these disease mechanisms contribute to LVDD and, ultimately, HFpEF.
Throughout this review, we will focus on the association between MetS and its components with changes in LVDD to offer updated information on this emerging issue. Using the following keywords, we searched PubMed, Medline, OVID, and EMBASE databases for English-language studies published from January 1963 to December 2024: “left ventricle diastolic dysfunction”, “metabolic syndrome”, “arterial hypertension”, “systemic hypertension”, “obesity”, “overweight”, “central obesity”, “body mass index”, “diabetes”, “increased glucose level”, “dyslipidemia”, “triglycerides”, and “high-density lipoprotein cholesterol”, and “heart failure with preserved ejective fraction”.
2 Definitions of MetS
There have been several definitions proposed for the MetS so that clinicians and researchers can use the tool effectively. An international consultation group on the definition of diabetes for the World Health Organization (WHO) proposed the first formalized definition of metabolic syndrome in 1998 (2). Several insulin resistance markers and two additional risk factors, including obesity, hypertension, high triglyceride level, reduced high-density lipoprotein (HDL) cholesterol level, or microalbuminuria, could be used to diagnose the syndrome by the WHO criteria. Based on the National Cholesterol Education Program-Third Adult Treatment Panel (NCEP ATP III) definition (3), a diagnosis is based on the presence of three of the following five factors: abdominal obesity, elevated triglyceride, reduced HDL cholesterol, elevated blood pressure (BP), and elevated fasting glucose (impaired fasting glucose or diabetes). In 2005, according to the International Diabetes Federation (IDF) (4), abdominal obesity was one of five factors that must be taken into account in the diagnosis of diabetes, with waist measurement serving as the primary screening tool. The remaining criteria were essentially the same as those in ATP III. While the American Heart Association (AHA)/National Heart, Lung, and Blood Institute (AHA-NHLBI) definition (5) slightly modified the ATP III criteria, abdominal obesity was not mandated as a risk factor. In 2009 (6), both the IDF and AHA/NHLBI recognized abdominal obesity as one of five criteria for a diagnosis, but not a prerequisite. As a result, the presence of three of five risk factors constitutes a diagnosis of MetS. Table 1 shows the common definition.
3 Echocardiographic variables for evaluating LVDD
LVDD is primarily assessed using echocardiography. The methodology is the most extensively validated, and it has the highest temporal resolution. We have summarized the main advantages, disadvantages, and indications for utilizing different echo parameters in Table 2. LVDD is widely evaluated with echocardiography due to its availability and relative low cost. The first recommendations for LVDD by echocardiography were published in 2009 (7). In patients with abnormal diastolic function, the severity of LVDD was determined by comparing the maximum diastolic velocity of the early(E) and late(A) waves, E/A ratios, E-wave deceleration time, diastolic velocities (tissue Doppler) in the mitral annulus (e’ and a’), and pulmonary vein inflow. More recently, updated recommendations from the European Association of Cardiovascular Imaging (EACVI) and the American Society of Echocardiography (ASE) for the evaluation of diastolic function by echocardiography were published in 2016 (8). These recommendations tried to apply the most feasible and reproducible measurements from the 2009 recommendations to simplify the evaluation of LVDD. The 2016 recommendations proposed a new algorithm to assess LVDD, as shown in Figure 1. A recent study has revealed a new LVDD indicator, left atrial (LA) reservoir strain, which has excellent feasibility of ~95% and can detect LV diastolic alterations and elevated LV filling pressure even when LA volume index (LAVI) is normal (9, 10).
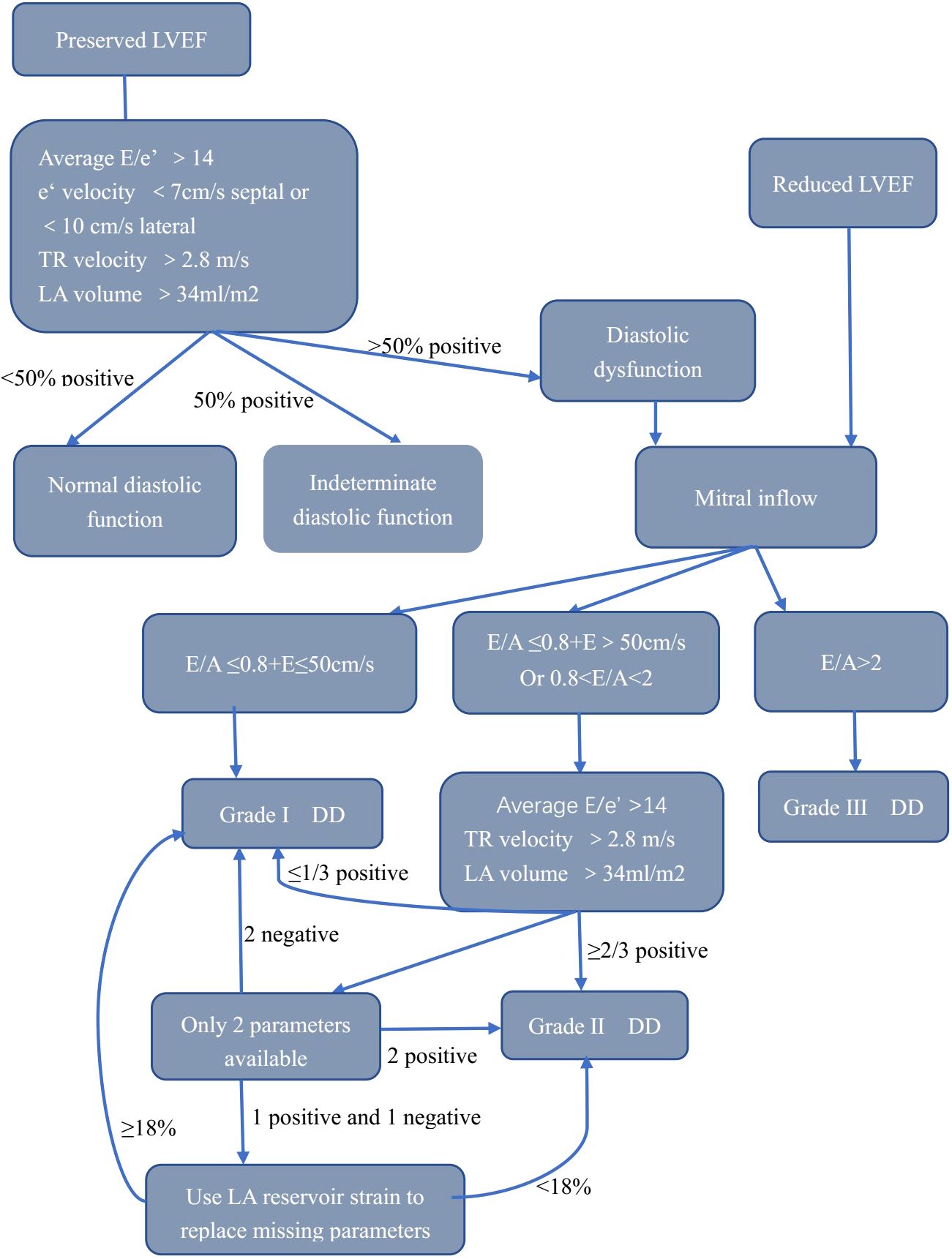
Figure 1. Algorithm for estimation of LVDD. LVEF, left ventricular ejection fraction; E, early filling; e’, early diastolic; LA, left atrium; TR, tricuspid regurgitation; LVDD, left ventricular diastolic dysfunction.
4 Hypertension and LVDD
A link between LVDD and hypertension was found (11), in which insulin resistance, LV concentric remodeling/hypertrophy (12), abnormalities of the renin–angiotensin–aldosterone system (RAAS) (13), endothelial dysfunction, and changes of coronary microcirculation (14) were factors. High sodium intake may affect BP parameters and arterial wall damage in hypertensive individuals, contributing to LVDD impairment (15). Sympathetic nerve system (SNS) activity causes LVDD in hypertension patients, and sympathoinhibition prevents the development or delays the progression of LVDD. Diastolic dysfunction was presumed to be a surrogate marker of myocardial fibrosis in hypertensive patients who were untreated. Matrix metalloproteinases type I (TIMP-1) tissue inhibitors correlate with diastolic dysfunction (16).
LVDD can occur across a range of blood pressure states, including normal blood pressure, hypertension, or masked hypertension. Grade 1 DD was more frequent in subjects with prehypertension, and grade 2 DD was significantly frequent in hypertension. Asymptomatic and newly diagnosed hypertensive patients showed reduced E/A and lower e’ velocity compared to normal BP individuals. Inter-visit systolic blood pressure (SBP) variability was more correlated with LVDD than mean SBP. Hypertensive patients have high pulse pressure and arterial stiffness, with significant effects on LVDD through ventricle-arterial coupling. Using the 2009 recommendations, Grade 1 DD was documented in 24.4%, grade 2 DD in 19.3%, and no patients were diagnosed with grade 3 LVDD. After applying the 2016 recommendations, LVDD was documented in 12.3% of patients (17). The prevalence of LVDD is 1.2%–2.7% in adults. An early stage of impaired glucose metabolism and diabetes in hypertensive patients may specifically deteriorate diastolic function. Obesity-related insulin resistance amplifies the effect of hypertension on LVDD. In elderly hypertensive women, LVDD occurs earlier and estimated filling pressures are higher, which indicates a greater likelihood of HFpEF. Primary hypertension patients experience a decrease in LA strain conduit and reservoir function before the diagnosis of LVDD is established.
Due to their ability to reduce both preload and afterload, angiotensin-converting enzyme inhibitors (ACEIs) and angiotensin-II receptor blockers (ARBs) are conceptually the most effective treatments for LVDD. Furthermore, these drugs reverse the concentric geometry of the left ventricle and reduce myocardial fibrosis and LV hypertrophy (LVH). Recent studies have examined the clinical effect of ARBs on LVDD. The E/e′ ratio of uncomplicated hypertensives was reduced by irbesartan (18). Angiotensin receptor-neprilysin inhibitors (ARNI) are recommended for the treatment of HFpEF. In addition to LVH regression, telmisartan therapy induced a parallel decrease in LAV and a shortening of IVRT (19). LV filling time is prolonged when the heart rate is lower, which counterbalances the resistance of a stiffened left ventricle to the diastolic inflow. In hypertension patients with LVDD, controlling heart rate is therefore an important objective. There was a strong correlation between e′ velocity and the extent of SBP reduction (SBP target <130 mm Hg or <140 mm Hg), and patients with the lowest achieved SBP values tended to have the highest velocity (20).
5 Diabetes and glucose intolerance and LVDD
Even patients with well-controlled diabetes without overt macrovascular complications, LV structural changes, and systolic and diastolic dysfunction have all been observed. It may be triggered by insulin resistance, abnormal substrate utilization by the myocardium, and uncoupling of mitochondrial oxidative phosphorylation. Researchers have identified decreased cardiomyocyte function in diabetic animal models, caused by impaired mitochondrial calcium handling and decreased levels of free matrix calcium, as a key mediator of heart failure. Inflammatory signaling and collagen metabolism are also affected by hyperglycemia via oxidative stress, protein kinase C activation, and advanced glycosylation end-products (AGEs). Additionally, insulin resistance may contribute to SNS activity. As a result of SNS activity, the RAAS may be stimulated and may promote LVDD via adrenergic-mediated hypertrophy and fibroblast growth, as well as apoptosis and necrosis in myocytes. A high level of insulin resistance, hyperglycemia, and increased metabolism of free fatty acids (FFAs) may contribute to diabetes-related altered cardiac phenotypes (21). Diabetic patients who had microvascular complications were most likely to develop cardiomyopathy, and several of them displayed alterations within the coronary arteries of the myocardium. Diabetic hearts in humans also display thickened capillary basement membranes and capillary microaneurysms (22). In a study by Zoneraich et al. (23) in type 1 diabetic patients with normotensive blood pressure levels, small vessel disease was present in 72%, but it was not found in non-diabetic patients with normal blood pressure levels.
Glucose interacts with collagen to form AGEs, and in diabetes, the process is accelerated, leading to interstitial fibrosis. In chronic hyperglycemia, vascular and membrane proteins are non-enzymatically glycated, resulting in reactive oxygen species (ROS) and AGEs. As a result of diabetes, AGEs of collagen are formed more frequently in the myocardium of the heart, which causes stiffness. Furthermore, glucose enhances the production of the extracellular matrix in fibroblasts by activating the collagen gene promoter sequence and increasing the level of angiotensin II type 1 receptors (24). The RAAS and SNS are major neurohormonal systems that affect cardiac remodeling; therefore, drugs that affect them are vital in preventing or reversing it (24). Diabetes-related changes in the heart include hypertrophy of myocytes, the addition of extracellular collagen, interstitial fibrosis, and microangiopathy within the myocardium (25).
In individuals with type 1 or 2 diabetes, LVDD is considered to be the first manifestation of diabetic cardiomyopathy, especially in patients with poorer glycemic control. In the Strong Heart Study, which enrolled 2,411 Native Americans, type 2 diabetes was frequently associated with an abnormal LV relaxation pattern, independent of age, BP, LV mass, and LV systolic function. There is a greater degree of abnormal LV relaxation in the diabetes-hypertension combination. HbA1c concentrations correlate with abnormal LV relaxation (26). In the context of LVDD, microalbuminuria is an independent risk factor, perhaps as a marker for intramyocardial microangiopathy. It appears that LVDD is more commonly associated with aging and chronic diabetes in patients with well-controlled diabetes than hypertension or LV hypertrophy. Diabetes patients’ E/A ratios in their 40s were not significantly different from those of control subjects, but in their 50s, 60s, and 70s, the ratio was significantly lower. Diabetes patients without overt heart disease are more susceptible to diastolic function deterioration when they are older, have retinopathy, and have increased BP over time (27). Diabetes duration was strongly and positively associated with larger LAVI. Worsening in e’ (5.4 vs. 7.3 cm/s) and E/e’ ratio (13.6 vs. 10.3) was observed in patients with diabetes or impaired glucose tolerance who also had cardiovascular autonomic neuropathy (28). Impaired LV longitudinal systolic and diastolic strains were documented in diabetes. One study found the diabetes susceptibility locus, HNF1B, is associated with prevalent diastolic dysfunction and incident cardiovascular disease. This may provide future drug targets (29). Those with a higher HbA1c level and obesity status showed a high prevalence of LVDD (30). Decreased LA reservoir, conduit, and booster strain was found in adolescents and young adults with obesity and diabetes, although LA volume was normal (31). Peak diastolic strain rate from cardiovascular magnetic resonance feature tracking was decreased in diabetes (32).
Glycemic control can partially prevent or reverse LVDD. Canagliflozin can improve LV diastolic function within 3 months, and those with significantly improved hemoglobin values showed the greatest benefit (33). Animal research found that PPAR-α (fenofibrate) or -γ agonists (pioglitazone) prevented LVDD, possibly by improving the fatty acid metabolism in the myocardium or by modifying hyperglycemia and/or hyperlipidemia (34). After 4 years of treatment with pioglitazone on LVDD in diabetes, a study found an increase in both E/e’ and LAVI (35). Liraglutide therapy had favorable effects on E/e’ regardless of body weight. The DPP-4 inhibitor, alogliptin, prevents cardiac diastolic dysfunction by inhibiting ventricular remodeling, which can be explained by enhanced mitochondrial function and increased mitochondrial biogenesis in diabetic rabbits (87). LVEF and E/e’ in individuals with diabetes improved after 6 months of treatment with tofogliflozin, a sodium-dependent glucose transporter 2 (SGLT2) inhibitor. A previous study has indicated that empagliflozin improves diastolic function, preserves calcium handling and growth signaling pathways, and reduces myocardial insulin resistance in obese mice (36). SGLT2 inhibitors (SGLT2is) are efficacious and safe in treating HFpEF in patients with comorbid chronic kidney disease with and without T2DM (37). SGLT2is work by inhibiting the sodium-glucose co-transporter 2 in the proximal renal tubules, reducing glucose reabsorption and increasing glucose excretion in urine. This leads to lower blood glucose levels, improved insulin sensitivity, and additional benefits such as reduced sodium retention, blood pressure, and cardiac workload, which are particularly beneficial in managing heart failure and diabetic conditions. The ESC 2023 HF guideline update has given SGLT2is for chronic HFpEF a class 1a recommendation (38). However, in patients with diabetes and hypertension without overt heart failure, metformin treatment did not affect LV mass or diastolic function after 1 year (39).
6 Obesity and LVDD
Previous histological analysis showed that the capillary length density of obese patients was lower (40), cardiomyocyte width was reduced, and the pulmonary capillary wedge pressure was greater. A reduction in phosphocreatine/adenosine triphosphate (ATP) is further exacerbated in obese individuals during inotropic stress, leading to continuing diastolic dysfunction. There is evidence that myocardial energetics may play a key role in obesity-related diastolic dysfunction. The obesity-related increase in LV filling pressure was associated with a lower coronary microvascular density, which could account for the lower maximal myocardial blood flow, impaired myocardial metabolism impairment, LVDD, and a greater risk of HF in obese individuals. Excess adipose accumulation in peripheral obesity increases total and central blood volume, resulting in an increase in cardiac output. Peripheral vascular resistance is decreased, which facilitates this process. An increased amount of visceral adipose tissue is associated with low-grade inflammation (serum C-reactive protein) (41). Obesity is associated with various neurohormonal and metabolic abnormalities that may alter cardiac morphology in humans. Obesity is associated with LVH (42), which alters cardiac morphology. The combination of insulin resistance and hyperinsulinemia has been linked to increased LV mass in obese animals and humans. LVH contributes the most to LV diastolic function. In obesity, RAAS activation may increase sympathetic tone and directly affect the LV myocardium, which can lead to LVH (43). LVDD and myocardial fibrosis were also exacerbated by obesity and hypertension in a synergistic manner (44). It is often difficult to determine whether obesity is independently responsible for LVDD in obese patients due to the presence of insulin resistance, impaired glucose tolerance, or overt diabetes (43).
Impairment of LV diastolic filling or relaxation in obesity was revealed. LVDD appears to be related to obesity level and fasting insulin levels and reduced exercise capacity (45, 46), however, obesity duration was not considered (47). Elderly patients with severe prolonged obesity had elevated plasma volume, eccentric LVH, and systolic and diastolic dysfunction. HF is more likely to develop in obese women. The adverse effect of central adiposity on LV diastolic function was independent of general adiposity and more prominent among women (48). Only visceral fat, other than total body fat, was significantly associated with LVDD. The relationship between LV diastolic function and visceral fat was significantly mediated by triglycerides and sex hormone-binding globulin, possibly through a metabolic pathway that involves blood lipids and ectopic fat accumulation (49). A significant association exists between adipocyte fatty acid-binding protein (FABP4) levels and LVDD in obese subjects who have MetS. FABP4 may thus play a role in obesity and cardiometabolic disorders (50). In morbidly obese individuals, the growth-differentiation factor (GDF)-15 level, a marker of inflammation, was better correlated with diastolic dysfunction. GDF-15 levels increase in relation to different degrees of LVDD (51). One study showed obese patients with a reduced diffusing capacity of the lungs for carbon monoxide had an increased prevalence of moderate or severe LVDD (52). Sarcopenic obesity was associated with impaired diastolic function and decreased exercise capacity (53, 54).
Weight loss, whether achieved through diet and exercise or bariatric surgery, can improve myocardial metabolism, left ventricular structure, and diastolic function, all of which are affected by obesity. As BMI decreased longitudinally, LAVI decreased significantly, and e’ velocity increased significantly. Surgical weight loss resulted in a 23% decrease in LV mass, a 33% increase in E/e, and a 28% improvement in relaxation. A reduction of BMI, insulin resistance, total oxygen consumption of the heart, and LV mass was associated with an improvement in LV relaxation but not the reduction of fatty acid utilization. These changes can be reversed more effectively by bariatric surgery than by diet and exercise alone. Bariatric surgery, which is primarily used for severely obese patients, results in significant weight loss and improves the neurohormonal and metabolic milieu to a greater extent than the weight loss modalities of diet and exercise alone (55).
High- and moderate-intensity training can prevent diet-induced obesity-related LV remodeling with diastolic and systolic dysfunction in mice, which suggests that physical activity can alleviate obesity-related cardiac disorders. An obese, insulin resistance, and hypertension rodent model showed that nebivolol attenuated diastolic dysfunction and myocardial remodeling by blunting myocardial oxidative stress and promoting insulin metabolic signaling (56). Recent evidence indicates that GLP-1 RA may play a significant role in preventing HFpEF in patients with obesity, MS, or obesity and T2DM (57). According to a recent study, Epithelial Sodium Channel (EnNaC) activation induces endothelium permeability, which promotes macrophage infiltration and oxidative stress, resulting in cardiac fibrosis and LVDD in female mice with diet-induced obesity. Western diet-induced impairments of left ventricular filling rate and relaxation time were attenuated by amiloride, an EnNaC antagonist (58). A significant reduction in cardiomyocyte area, interstitial and perivascular fibrosis, and collagen deposition was observed after clostazol treatment. The inflammatory milieu in the hearts of obese mice was also reduced by cilostazol. There may be a therapeutic role for clostazol in treating obesity-related diastolic dysfunction and preventing overt heart failure (59).
7 Hyperlipidemia and LVDD
There is an association between LVDD and increased myocardial lipid storage. Diastolic dysfunction can be induced by lipid intermediates, which generate ROS. Mitochondrial dysfunction and impaired energetics may lead to cardiac dysfunction since the heart requires most of its ATP from mitochondrial oxidative phosphorylation. Reduced ATP availability may be caused by mitochondrial dysfunction but could also be caused by myocardial lipid buildup. It has been discussed previously that mitochondrial dysfunction leads to an increase in mitochondrial ROS, which damages cellular components and causes subsequent dysfunction (60). Increased oxidative stress and inflammation in a high-fat and high-cholesterol diet in rats led to cardiac fibrosis, endothelial dysfunction, and LVDD (61).
Intramyocardial fat deposition may partly contribute to LVH and impaired diastolic function in humans. When all components of the MetS and visceral adiposity tissue were adjusted for, an increase in hepatic triglyceride content was associated with a change in mean E/A in obese individuals. In healthy subjects or those with diabetes, a short-term very low-calorie diet (VLCD) induced the accumulation of myocardial triglycerides and was associated with a decrease in LV diastolic function (62, 63). An increase in intramyocardial triglycerides is associated with LVDD. Myocardial steatosis is associated with accelerated deterioration of left ventricular diastolic function over time. In children with heterozygous familial hypercholesterolemia (FH), reduced e’ and higher E/e’ ratios were observed (64). In one Mendelian randomization (MR) analysis, HDL cholesterol showed no significant connection with any LV parameter. LDL cholesterol and triglycerides were independently associated with adverse changes in LV mass in another MR analysis (65).
There is controversy regarding the effect of lipid-lowering therapy on improving diastolic function. Compared with healthy volunteers, patients with hypercholesterolemia showed lower E/A ratios, higher Tei indexes, and lower e’/a’ ratio in both the septum and laterally. After 6 months of rosuvastatin (RSV) treatment, a significant improvement of longitudinal global systolic and diastolic function (Tei index) was registered (66). The subjects received treatment with RSV or pitavastatin (PTV) for 24 weeks, however, the result showed statin treatment did not significantly alter the E/e′ ratio (67). Multiple diastolic parameters should be included in this research to avoid bias. In rabbits, HDL cholesterol infusions accompany a rapid improvement of LVDD with reductions of LV macrophage accumulation, coronary atherosclerosis, cardiomyocyte apoptosis, and remodeling (68).
8 MetS and LVDD
Although the effect of the MetS on LV remodeling has been extensively studied, the effect of MetS on LVDD has been less studied (69–73) (Table 3). Previous studies have revealed that patients with MetS have LVDD independent of LV mass (74, 75). In patients with MS, insulin resistance plays a key role in LVDD and HFpEF (76). Additionally, SNS excitement, RAAS activation, oxidative stress, endothelial dysfunction, and inflammation, which are common symptoms of MetS, could also explain the worsening of LVDD in these patients. In MetS, excess salt induces LVDD through the upregulation of mineralocorticoid receptor signals and increased oxidative stress. Myocardial fibrosis is a key contributor to subclinical LVDD and HFpEF in patients with MetS. In patients with MetS, those with increased abdominal fat deposition exhibited higher levels of procollagen peptides and cardiac fibrosis and more severe LVDD manifested by lower myocardial remodeling and e’ and higher E/e’ ratios (77). MetS and higher insulin resistance were significantly related to impaired diastolic function in one study using cardiac magnetic resonance imaging (CMR), independent of the myocardial extracellular matrix (ECV). The severity of LVDD was strongly related to the abundance of MetS components. Importantly, diabetes and obesity affect LV function even in the absence of coronary artery disease and hypertension (78). LVDD was more pronounced in individuals with obesity and MS than in those without (79). LVDD can also occur in patients with MetS without hypertension. Impaired LV diastolic and systolic synchronization were found in patients with MS, in which obesity, hyperglycemia, and age play key roles, whereas hypertension was not a contributor to impaired synchronicity. A previous study has shown that the impact of the MetS on preclinical myocardial abnormalities were not accounted for by differences in age, gender, or 24-h BP and can be reasonably ascribed to the interplay of MetS components, making MetS in itself a relevant clinical problem in non-diabetic patients or in those never treated with antihypertensive or lipid-lowering drugs (80). Additionally, the coexistence of MS with hypertension or diabetes can further worsen LVDD (81, 82).
A previous study confirmed a significant improvement in LVDD after a 1-year lifestyle intervention program in abdominally obese men with MS, which worked through improved exercise tolerance, enhanced heart rate variability (HRV), and decreased insulin levels (83). One study showed that adding spironolactone to standard angiotensin II inhibition therapy significantly improved myocardial fibrosis in patients with MS (84). In a rat model of metabolic syndrome, improving endothelial dysfunction could improve LVH and diastolic function (85, 86). The DPP-4 inhibitor linagliptin reduces left ventricular stiffness and improves LV relaxation, which was related to decreased cardiac fibrosis and cardiomyocyte passive stiffness in MetS rats (87). In MetS rats, calorie restriction alleviates the incidence of obesity, hypertension, LV remodeling, and diastolic dysfunction by reducing cardiac oxidative stress and inflammation (88). Statins treatment reverses myocardial remodeling and enhances ventricular relaxation via AMPK (amplifier-activated protein kinase)-mediated antifibrotic effects (89). Attenuation of the inflammatory and oxidative stress process, reduced insulin levels, and decreased cardiac fibrosis may provide a novel therapeutic strategy in treating metabolic cardiomyopathy.
9 Clinical consequences of LVDD in individuals with metabolic syndrome
LVDD is an independent predictor of adverse cardiovascular events in hypertensive patients. Of importance, the prognostic value of e′ and the E/e’ ratio for heart failure has been recognized in the hypertensive setting with normal EF. The Framingham Heart Study also provided the earliest evidence of diabetes and heart failure independent of coronary artery disease (90). In individuals with overt cardiovascular diseases, MetS increases the risk of adverse events; however, in HF patients, MetS was not an independent predictor for all-cause mortality or cardiovascular mortality. Few studies have investigated the prognostic value of LVDD in patients with MetS. The coexistence of MetS with diastolic dysfunction showed obvious incremental value in predicting cardiac events. Overall, few prospective studies have explored the association between LVDD and HFpEF in patients with MetS. Much of the current evidence is based on the characterization of patients with HFpEF. MetS refers to a group of interrelated disorders. More studies have explored the prognosis of LVDD in patients with hypertension or diabetes. Along with an aging population and rising rates of cardiometabolic comorbidities, HFpEF has a tremendous global burden and is poised to increase in prevalence, particularly the MetS phenotype. Future research should focus on the prognostic value of LVDD in patients with metabolic syndrome. A previous study revealed that the number of MetS criteria fulfilled and the presence of 4–5 criteria was associated with incident HF, implying that metabolic disturbances also contribute to an elevated risk of HF through pathways other than insulin resistance (76).
10 Conclusion
The prevalence of MetS is constantly growing. MetS-related LVDD or HFpEF also leads to a high risk of cardiac events in this population. Echocardiography diagnosis techniques are rapidly developing, allowing for the early detection of cardiac structural or functional alterations, as summarized in Figure 2. Although the underlying mechanisms of HFpEF pathogenesis remain controversial, insulin resistance and subsequent changes in both cardiomyocytes and the myocardial interstitium and coronary microcirculation play critical roles in mediating LVDD in MetS. Considering this, in individuals with MetS, improving LVDD may serve as the therapeutic strategy to prevent or ameliorate HFpEF, especially for the MetS phenotype. Improving metabolic abnormalities associated with MetS, particularly through controlling insulin levels and reducing oxidative stress and inflammation, may help slow down or reverse the progression of LVDD, thereby improving the prognosis of HFpEF. Although lifestyle interventions (such as weight loss and exercise) and pharmacological treatments (such as statins and liraglutide) have been shown to have certain effects on improving LVDD, there is significant individual variability among patients with MetS, and the universality and long-term effects of these interventions are still unclear. The lack of standardized treatment guidelines and evidence-based support makes it difficult to implement clinical treatments broadly. With the continuous development of personalized medicine, future research should focus more on how to tailor treatment plans based on different MetS subtypes and patient characteristics (such as gender, age, and comorbidities). Further prospective large population studies are needed to integrate clinical data and optimize intervention strategies to enhance treatment outcomes.
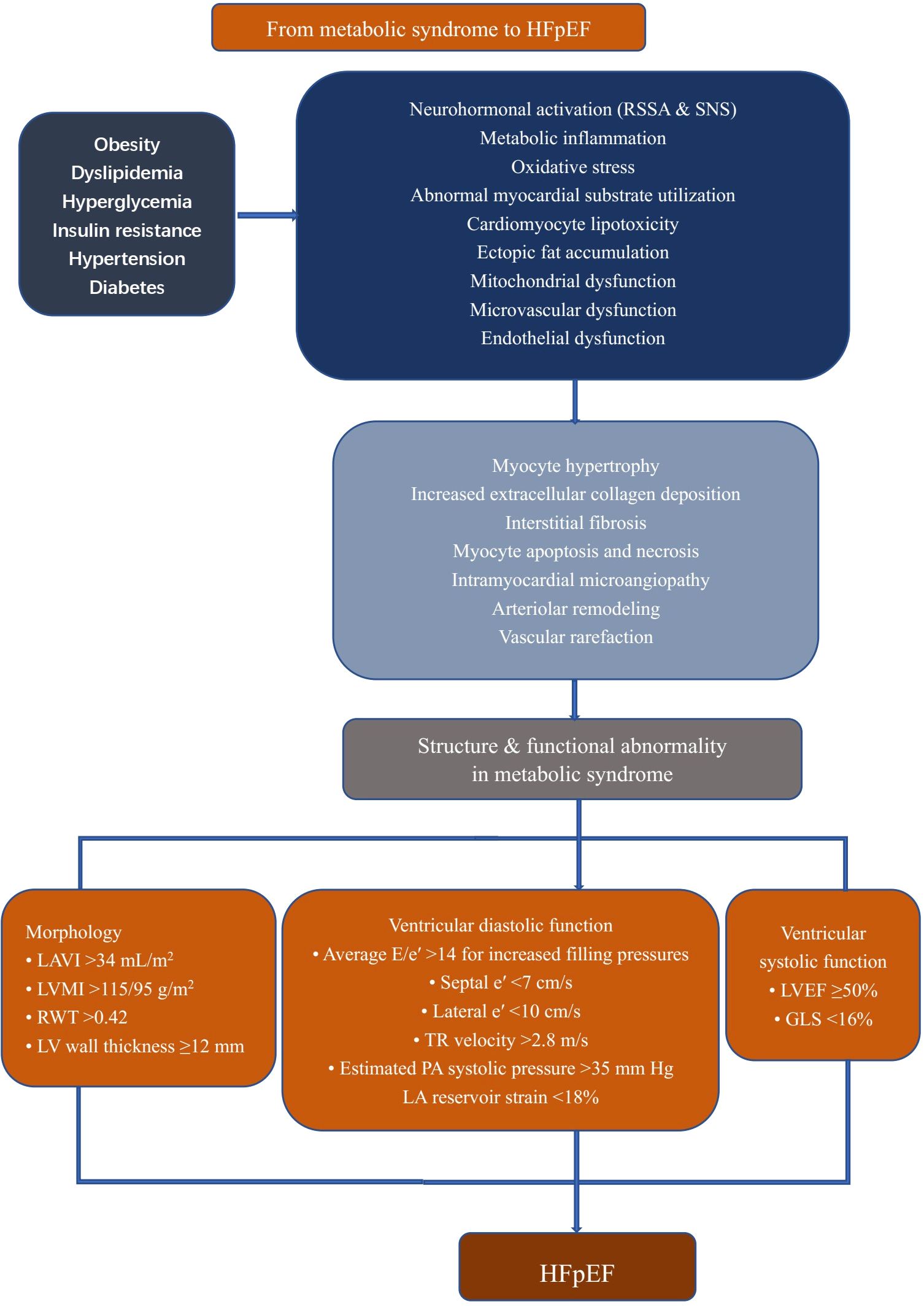
Figure 2. Cardiac remodeling in patients with metabolic syndrome. All components of metabolic syndrome can induce cardiac alteration to some extent. Their sum activates several pathophysiological pathways in the vessels and heart, including neurohormonal activation, mitochondrial dysfunction, and increased oxidative and inflammatory stress, leading to adverse cardiac remodeling. LVEF, left ventricular ejection fraction; E, early filling; e’, early diastolic; LA, left atrium; LAVI, left atrium volume index; LVMI, left ventricular mass index; RWT, relative wall thickness; TR, tricuspid regurgitation; PA, pulmonary artery; GLS, Global Longitudinal Strain; HFpEF, heart failure with preserved ejection fraction; RSSA, Renin Angiotensin Aldosterone System; SNS, sympathetic nervous system.
Author contributions
DZ: Conceptualization, Investigation, Supervision, Writing – original draft, Writing – review & editing. SL: Conceptualization, Investigation, Writing – original draft. ZL: Conceptualization, Investigation, Writing – original draft. JY: Conceptualization, Investigation, Writing – original draft. HR: Conceptualization, Investigation, Writing – review & editing. HT: Conceptualization, Investigation, Writing – review & editing. YG: Conceptualization, Investigation, Writing – review & editing. XJ: Conceptualization, Investigation, Writing – original draft, Writing – review & editing.
Funding
The author(s) declare that financial support was received for the research and/or publication of this article. This work was supported by Sustainable Development Science and Technology Project of Shenzhen Science and Technology Innovation Commission (KCXFZ20201221173411032; KCXFZ20201221173400001), “Five Threes” clinical research program of Shenzhen People’s Hospital (SYWGSLCYJ202204), the Natural Science Foundation of China (82371471), and Shenzhen Key Medical Discipline Construction Fund (No. SZXK012, No. SZXK005).
Conflict of interest
The authors declare that the research was conducted in the absence of any commercial or financial relationships that could be construed as a potential conflict of interest.
Generative AI statement
The author(s) declare that no Generative AI was used in the creation of this manuscript.
Publisher’s note
All claims expressed in this article are solely those of the authors and do not necessarily represent those of their affiliated organizations, or those of the publisher, the editors and the reviewers. Any product that may be evaluated in this article, or claim that may be made by its manufacturer, is not guaranteed or endorsed by the publisher.
References
1. McDonagh TA, Metra M, Adamo M, Gardner RS, Baumbach A, Böhm M, et al. 2021 ESC Guidelines for the diagnosis and treatment of acute and chronic heart failure. Eur Heart J. (2021) 42:3599–726. doi: 10.1093/eurheartj/ehab368
2. Alberti KG, Zimmet PZ. Definition, diagnosis and classification of diabetes mellitus and its complications. Part 1: diagnosis and classification of diabetes mellitus provisional report of a WHO consultation. Diabetes Med. (1998) 15:539–53. doi: 10.1002/(SICI)1096-9136(199807)15:7<539::AID-DIA668>3.0.CO;2-S
3. Expert Panel on Detection, Evaluation, and Treatment of High Blood Cholesterol in Adults. Executive summary of the third report of the national cholesterol education program (NCEP) expert panel on detection, evaluation, and treatment of high blood cholesterol in adults (Adult treatment panel III). JAMA (2001) 285(19):2486–97. doi: 10.1001/jama.285.19.2486
4. Alberti KG, Zimmet P, Shaw J. The metabolic syndrome–a new worldwide definition. Lancet (London England). (2005) 366:1059–62. doi: 10.1016/S0140-6736(05)67402-8
5. Grundy SM, Cleeman JI, Daniels SR, Donato KA, Eckel RH, Franklin BA, et al. Diagnosis and management of the metabolic syndrome: an American Heart Association/National Heart, Lung, and Blood Institute Scientific Statement. Circulation. (2005) 112:2735–52. doi: 10.1161/CIRCULATIONAHA.105.169404
6. Alberti KG, Eckel RH, Grundy SM, Zimmet PZ, Cleeman JI, Donato KA, et al. Harmonizing the metabolic syndrome: a joint interim statement of the International Diabetes Federation Task Force on Epidemiology and Prevention; National Heart, Lung, and Blood Institute; American Heart Association; World Heart Federation; International Atherosclerosis Society; and International Association for the Study of Obesity. Circulation. (2009) 120:1640–5. doi: 10.1161/CIRCULATIONAHA.109.192644
7. Nagueh SF, Appleton CP, Gillebert TC, Marino PN, Oh JK, Smiseth OA, et al. Recommendations for the evaluation of left ventricular diastolic function by echocardiography. J Am Soc Echocardiography: Off Publ Am Soc Echocardiography. (2009) 22:107–33. doi: 10.1016/j.echo.2008.11.023
8. Nagueh SF, Smiseth OA, Appleton CP, Byrd BF 3rd, Dokainish H, Edvardsen T, et al. Recommendations for the evaluation of left ventricular diastolic function by echocardiography: an update from the american society of echocardiography and the european association of cardiovascular imaging. J Am Soc Echocardiography: Off Publ Am Soc Echocardiography. (2016) 29:277–314. doi: 10.1016/j.echo.2016.01.011
9. Morris DA, Belyavskiy E, Aravind-Kumar R, Kropf M, Frydas A, Braunauer K, et al. Potential usefulness and clinical relevance of adding left atrial strain to left atrial volume index in the detection of left ventricular diastolic dysfunction. JACC Cardiovasc imaging. (2018) 11:1405–15. doi: 10.1016/j.jcmg.2017.07.029
10. Smiseth OA, Morris DA, Cardim N, Cikes M, Delgado V, Donal E, et al. Multimodality imaging in patients with heart failure and preserved ejection fraction: an expert consensus document of the European Association of Cardiovascular Imaging. Eur Heart J Cardiovasc Imaging. (2022) 23:e34–61. doi: 10.1093/ehjci/jeab154
11. Cai A, Zhou D, Liu L, Zhou Y, Tang S, Feng Y. Age-related alterations in cardiac and arterial structure and function in hypertensive women and men. J Clin Hypertens (Greenwich). (2021) 23:1322–34. doi: 10.1111/jch.14262
12. Ragosta M, Samady H, Isaacs RB, Gimple LW, Sarembock IJ, Powers ER. Coronary flow reserve abnormalities in patients with diabetes mellitus who have end-stage renal disease and normal epicardial coronary arteries. Am Heart J. (2004) 147:1017–23. doi: 10.1016/j.ahj.2003.07.029
13. Sciarretta S, Paneni F, Palano F, Chin D, Tocci G, Rubattu S, et al. Role of the renin-angiotensin-aldosterone system and inflammatory processes in the development and progression of diastolic dysfunction. Clin Sci (London England: 1979). (2009) 116:467–77. doi: 10.1042/CS20080390
14. Galderisi M. Diagnosis and management of left ventricular diastolic dysfunction in the hypertensive patient. Am J hypertension. (2011) 24:507–17. doi: 10.1038/ajh.2010.235
15. Cwynar M, Gasowski J, Stompor T, Barton H, Wizner B, Dubiel M, et al. Blood pressure and arterial stiffness in patients with high sodium intake in relation to sodium handling and left ventricular diastolic dysfunction status. J Hum hypertension. (2015) 29:583–91. doi: 10.1038/jhh.2015.1
16. Lindsay MM, Maxwell P, Dunn FG. TIMP-1: a marker of left ventricular diastolic dysfunction and fibrosis in hypertension. Hypertension (Dallas Tex: 1979). (2002) 40:136–41. doi: 10.1161/01.HYP.0000024573.17293.23
17. Zhou D, Yan M, Cheng Q, Feng X, Tang S, Feng Y. Prevalence and prognosis of left ventricular diastolic dysfunction in community hypertension patients. BMC Cardiovasc Disord. (2022) 22:265. doi: 10.1186/s12872-022-02709-3
18. Müller-Brunotte R, Kahan T, Malmqvist K, Ring M, Edner M. Tissue velocity echocardiography shows early improvement in diastolic function with irbesartan and atenolol therapy in patients with hypertensive left ventricular hypertrophy. Results form the Swedish Irbesartan Left Ventricular Hypertrophy Investigation vs Atenolol (SILVHIA). Am J hypertension. (2006) 19:927–36. doi: 10.1016/j.amjhyper.2006.02.009
19. Mattioli AV, Zennaro M, Bonatti S, Bonetti L, Mattioli G. Regression of left ventricular hypertrophy and improvement of diastolic function in hypertensive patients treated with telmisartan. Int J Cardiol. (2004) 97:383–8. doi: 10.1016/j.ijcard.2003.10.018
20. Solomon SD, Verma A, Desai A, Hassanein A, Izzo J, Oparil S, et al. Effect of intensive versus standard blood pressure lowering on diastolic function in patients with uncontrolled hypertension and diastolic dysfunction. Hypertension (Dallas Tex: 1979). (2010) 55:241–8. doi: 10.1161/HYPERTENSIONAHA.109.138529
21. Karnik AA, Fields AV, Shannon RP. Diabetic cardiomyopathy. Curr hypertension Rep. (2007) 9:467–73. doi: 10.1007/s11906-007-0086-3
22. Fischer VW, Barner HB, Leskiw ML. Capillary basal laminar thichness in diabetic human myocardium. Diabetes. (1979) 28:713–9. doi: 10.2337/diab.28.8.713
23. Zoneraich S, Silverman G, Zoneraich O. Primary myocardial disease, diabetes mellitus, and small vessel disease. Am Heart J. (1980) 100:754–5. doi: 10.1016/0002-8703(80)90243-4
24. Asbun J, Villarreal FJ. The pathogenesis of myocardial fibrosis in the setting of diabetic cardiomyopathy. J Am Coll Cardiol. (2006) 47:693–700. doi: 10.1016/j.jacc.2005.09.050
25. Gaasch WH, Cole JS, Quinones MA, Alexander JK. Dynamic determinants of letf ventricular diastolic pressure-volume relations in man. Circulation. (1975) 51:317–23. doi: 10.1161/01.cir.51.2.317
26. Liu JE, Palmieri V, Roman MJ, Bella JN, Fabsitz R, Howard BV, et al. The impact of diabetes on left ventricular filling pattern in normotensive and hypertensive adults: the Strong Heart Study. J Am Coll Cardiol. (2001) 37:1943–9. doi: 10.1016/S0735-1097(01)01230-X
27. Bergerot C, Davidsen ES, Amaz C, Thibault H, Altman M, Bellaton A, et al. Diastolic function deterioration in type 2 diabetes mellitus: predictive factors over a 3-year follow-up. Eur Heart J Cardiovasc Imaging. (2018) 19:67–73. doi: 10.1093/ehjci/jew331
28. Dinh W, Futh R, Lankisch M, Bansemir L, Nickl W, Scheffold T, et al. Cardiovascular autonomic neuropathy contributes to left ventricular diastolic dysfunction in subjects with Type 2 diabetes and impaired glucose tolerance undergoing coronary angiography. Diabetes Med. (2011) 28:311–8. doi: 10.1111/j.1464-5491.2010.03221.x
29. Molvin J, Jujic A, Nilsson PM, Leosdottir M, Lindblad U, Daka B, et al. A diabetes-associated genetic variant is associated with diastolic dysfunction and cardiovascular disease. ESC Heart failure. (2020) 7:348–56. doi: 10.1002/ehf2.12573
30. Maiello M, Zito A, Cecere A, Ciccone MM, Palmiero P. Left ventricular diastolic dysfunction in normotensive postmenopausal women with type 2 diabetes mellitus. Cardiol J. (2017) 24:51–6. doi: 10.5603/CJ.a2016.0064
31. Steele JM, Urbina EM, Mazur WM, Khoury PR, Nagueh SF, Tretter JT, et al. Left atrial strain and diastolic function abnormalities in obese and type 2 diabetic adolescents and young adults. Cardiovasc Diabetol. (2020) 19:163. doi: 10.1186/s12933-020-01139-9
32. Zhou S, Zhang Z, Zhang Z, Gao Y, Li G, Lou M, et al. Evaluation of left ventricular systolic and diastolic function in subjects with prediabetes and diabetes using cardiovascular magnetic resonance-feature tracking. Acta Diabetol. (2022) 59:491–9. doi: 10.1007/s00592-021-01822-7
33. Matsutani D, Sakamoto M, Kayama Y, Takeda N, Horiuchi R, Utsunomiya K. Effect of canagliflozin on left ventricular diastolic function in patients with type 2 diabetes. Cardiovasc Diabetol. (2018) 17:73. doi: 10.1186/s12933-018-0717-9
34. Kim SK, Zhao ZS, Lee YJ, Lee KE, Kang SM, Choi D, et al. Left-ventricular diastolic dysfunction may be prevented by chronic treatment with PPAR-alpha or -gamma agonists in a type 2 diabetic animal model. Diabetes Metab Res Rev. (2003) 19:487–93. doi: 10.1002/dmrr.v19:6
35. Investigators AES, Group AC. Effects of perindopril-indapamide on left ventricular diastolic function and mass in patients with type 2 diabetes: the ADVANCE Echocardiography Substudy. J hypertension. (2011) 29:1439–47. doi: 10.1097/HJH.0b013e3283480fe9
36. Hammoudi N, Jeong D, Singh R, Farhat A, Komajda M, Mayoux E, et al. Empagliflozin improves left ventricular diastolic dysfunction in a genetic model of type 2 diabetes. Cardiovasc Drugs Ther. (2017) 31:233–46. doi: 10.1007/s10557-017-6734-1
37. Mentz RJ, Brunton SA, Rangaswami J. Sodium-glucose cotransporter-2 inhibition for heart failure with preserved ejection fraction and chronic kidney disease with or without type 2 diabetes mellitus: a narrative review. Cardiovasc Diabetol. (2023) 22:316. doi: 10.1186/s12933-023-02023-y
38. McDonagh TA, Metra M, Adamo M, Gardner RS, Baumbach A, Böhm M, et al. 2023 Focused Update of the 2021 ESC Guidelines for the diagnosis and treatment of acute and chronic heart failure. Eur Heart J. (2023) 44:3627–39. doi: 10.1093/eurheartj/ehad195
39. Ono K, Wada H, Satoh-Asahara N, Inoue H, Uehara K, Funada J, et al. Effects of metformin on left ventricular size and function in hypertensive patients with type 2 diabetes mellitus: results of a randomized, controlled, multicenter, phase IV trial. Am J Cardiovasc Drugs. (2020) 20:283–93. doi: 10.1007/s40256-019-00381-1
40. Campbell DJ, Somaratne JB, Prior DL, Yii M, Kenny JF, Newcomb AE, et al. Obesity is associated with lower coronary microvascular density. PloS One. (2013) 8:e81798. doi: 10.1371/journal.pone.0081798
41. Wu CK, Yang CY, Lin JW, Hsieh HJ, Chiu FC, Chen JJ, et al. The relationship among central obesity, systemic inflammation, and left ventricular diastolic dysfunction as determined by structural equation modeling. Obes (Silver Spring). (2012) 20:730–7. doi: 10.1038/oby.2011.30
42. Cai A, Liu L, Zhou D, Tang S, Tadic M, Schutte AE, et al. Obesity and risk of incident left ventricular hypertrophy in community-dwelling populations with hypertension: an observational study. J Am Heart Assoc. (2024) 13:e033521. doi: 10.1161/JAHA.123.033521
43. Alpert MA, Lavie CJ, Agrawal H, Aggarwal KB, Kumar SA. Obesity and heart failure: epidemiology, pathophysiology, clinical manifestations, and management. Trans research: J Lab Clin Med. (2014) 164:345–56. doi: 10.1016/j.trsl.2014.04.010
44. Zhang X, Li ZL, Eirin A, Ebrahimi B, Pawar AS, Zhu XY, et al. Cardiac metabolic alterations in hypertensive obese pigs. Hypertension (Dallas Tex: 1979). (2015) 66:430–6. doi: 10.1161/HYPERTENSIONAHA.115.05478
45. Alpert MA, Terry BE, Mulekar M, Cohen MV, Massey CV, Fan TM, et al. Cardiac morphology and left ventricular function in normotensive morbidly obese patients with and without congestive heart failure, and effect of weight loss. Am J Cardiol. (1997) 80:736–40. doi: 10.1016/S0002-9149(97)00505-5
46. Barbosa MM, Beleigoli AM, de Fatima Diniz M, Freire CV, Ribeiro AL, Nunes MC. Strain imaging in morbid obesity: insights into subclinical ventricular dysfunction. Clin Cardiol. (2011) 34:288–93. doi: 10.1002/clc.20907
47. Wong CY, O’Moore-Sullivan T, Leano R, Byrne N, Beller E, Marwick TH. Alterations of left ventricular myocardial characteristics associated with obesity. Circulation. (2004) 110:3081–7. doi: 10.1161/01.CIR.0000147184.13872.0F
48. Canepa M, Strait JB, Abramov D, Milaneschi Y, AlGhatrif M, Moni M, et al. Contribution of central adiposity to left ventricular diastolic function (from the Baltimore Longitudinal Study of Aging). Am J Cardiol. (2012) 109:1171–8. doi: 10.1016/j.amjcard.2011.11.054
49. Canepa M, Strait JB, Milaneschi Y, AlGhatrif M, Ramachandran R, Makrogiannis S, et al. The relationship between visceral adiposity and left ventricular diastolic function: results from the Baltimore Longitudinal Study of Aging. Nutrition metabolism Cardiovasc diseases: NMCD. (2013) 23:1263–70. doi: 10.1016/j.numecd.2013.04.003
50. Baessler A, Lamounier-Zepter V, Fenk S, Strack C, Lahmann C, Loew T, et al. Adipocyte fatty acid-binding protein levels are associated with left ventricular diastolic dysfunction in morbidly obese subjects. Nutr Diabetes. (2014) 4:e106. doi: 10.1038/nutd.2014.3
51. Baessler A, Strack C, Rousseva E, Wagner F, Bruxmeier J, Schmiedel M, et al. Growth-differentiation factor-15 improves reclassification for the diagnosis of heart failure with normal ejection fraction in morbid obesity. Eur J Heart failure. (2014) 14:1240–8. doi: 10.1093/eurjhf/hfs116
52. Ravipati G, Aronow WS, Sidana J, Maguire GP, McClung JA, Belkin RN, et al. Association of reduced carbon monoxide diffusing capacity with moderate or severe left ventricular diastolic dysfunction in obese persons. Chest. (2005) 128:1620–2. doi: 10.1378/chest.128.3.1620
53. Jung MH, Ihm SH, Park SM, Jung HO, Hong KS, Baek SH, et al. Effects of sarcopenia, body mass indices, and sarcopenic obesity on diastolic function and exercise capacity in Koreans. Metabolism. (2019) 97:18–24. doi: 10.1016/j.metabol.2019.05.007
54. Yoo JH, Park SW, Jun JE, Jin SM, Hur KY, Lee MK, et al. Relationship between low skeletal muscle mass, sarcopenic obesity and left ventricular diastolic dysfunction in Korean adults. Diabetes Metab Res Rev. (2021) 37:e3363. doi: 10.1002/dmrr.v37.1
55. Grapsa J, Tan TC, Paschou SA, Kalogeropoulos AS, Shimony A, Kaier T, et al. The effect of bariatric surgery on echocardiographic indices: a review of the literature. Eur J Clin Invest. (2013) 43:1224–30. doi: 10.1111/eci.2013.43.issue-11
56. Zhou X, Ma L, Habibi J, Whaley-Connell A, Hayden MR, Tilmon RD, et al. Nebivolol improves diastolic dysfunction and myocardial remodeling through reductions in oxidative stress in the Zucker obese rat. Hypertension (Dallas Tex: 1979). (2010) 55:880–8. doi: 10.1161/HYPERTENSIONAHA.109.145136
57. Jalil JE, Gabrielli L, Ocaranza MP, MacNab P, Fernández R, Grassi B, et al. New mechanisms to prevent heart failure with preserved ejection fraction using glucagon-like peptide-1 receptor agonism (GLP-1 RA) in metabolic syndrome and in type 2 diabetes: A review. Int J Mol Sci. (2024) 25. doi: 10.3390/ijms25084407
58. Jia G, Habibi J, Aroor AR, Hill MA, DeMarco VG, Lee LE, et al. Enhanced endothelium epithelial sodium channel signaling prompts left ventricular diastolic dysfunction in obese female mice. Metabolism. (2018) 78:69–79. doi: 10.1016/j.metabol.2017.08.008
59. Reddy SS, Agarwal H, Barthwal MK. Cilostazol ameliorates heart failure with preserved ejection fraction and diastolic dysfunction in obese and non-obese hypertensive mice. J Mol Cell Cardiol. (2018) 123:46–57. doi: 10.1016/j.yjmcc.2018.08.017
60. Leggat J, Bidault G, Vidal-Puig A. Lipotoxicity: a driver of heart failure with preserved ejection fraction? Clin Sci (London England: 1979). (2021) 135:2265–83. doi: 10.1042/CS20210127
61. Watanabe S, Kumazaki S, Kusunoki K, Inoue T, Maeda Y, Usui S, et al. A high-fat and high-cholesterol diet induces cardiac fibrosis, vascular endothelial, and left ventricular diastolic dysfunction in SHRSP5/dmcr rats. J Atheroscler Thromb. (2018) 25:439–53. doi: 10.5551/jat.40956
62. van der Meer RW, Hammer S, Smit JW, Frolich M, Bax JJ, Diamant M, et al. Short-term caloric restriction induces accumulation of myocardial triglycerides and decreases left ventricular diastolic function in healthy subjects. Diabetes. (2007) 56:2849–53. doi: 10.2337/db07-0768
63. Hammer S, van der Meer RW, Lamb HJ, de Boer HH, Bax JJ, de Roos A, et al. Short-term flexibility of myocardial triglycerides and diastolic function in patients with type 2 diabetes mellitus. Am J Physiol Endocrinol Metab. (2008) 295:E714–8. doi: 10.1152/ajpendo.90413.2008
64. Di Salvo G, D’Aiello AF, Castaldi B, Fadel B, Limongelli G, D’Andrea A, et al. Early left ventricular abnormalities in children with heterozygous familial hypercholesterolemia. J Am Soc Echocardiography: Off Publ Am Soc Echocardiography. (2012) 25:1075–82. doi: 10.1016/j.echo.2012.07.002
65. Aung N, Sanghvi MM, Piechnik SK, Neubauer S, Munroe PB, Petersen SE. The effect of blood lipids on the left ventricle: A mendelian randomization study. J Am Coll Cardiol. (2020) 76:2477–88. doi: 10.1016/j.jacc.2020.09.583
66. Talini E, Di Bello V, Bianchi C, Palagi C, Delle Donne MG, Penno G, et al. Early impairment of left ventricular function in hypercholesterolemia and its reversibility after short term treatment with rosuvastatin A preliminary echocardiographic study. Atherosclerosis. (2008) 197:346–54. doi: 10.1248/bpb.b15-00126
67. Morimoto T, Katanasaka Y, Sunagawa Y, Hirano S, Miyazaki Y, Funamoto M, et al. Effects of statins on left ventricular diastolic function in patients with dyslipidemia and diastolic dysfunction (Stat-LVDF study). Biol Pharm bulletin. (2015) 38:1404–9. doi: 10.1248/bpb.b15-00126
68. Merlet N, Busseuil D, Mihalache-Avram T, Mecteau M, Shi Y, Nachar W, et al. HDL mimetic peptide CER-522 treatment regresses left ventricular diastolic dysfunction in cholesterol-fed rabbits. Int J Cardiol. (2016) 215:364–71. doi: 10.1016/j.ijcard.2016.04.029
69. Parvanescu T, Vitel A, Sporea I, Mare R, Buz B, Bordejevic DA, et al. Significant association between left ventricular diastolic dysfunction, left atrial performance and liver stiffness in patients with metabolic syndrome and non-alcoholic fatty liver disease. Diabetes Metab syndrome obesity: Targets Ther. (2021) 14:1535–45. doi: 10.2147/DMSO.S300450
70. Chung JW, Seo DI, Park Y, So WY. Echocardiography evaluation of left ventricular diastolic function in elderly women with metabolic syndrome. Open Med (Wars). (2019) 14:633–8. doi: 10.1515/med-2019-0073
71. Jorgensen PG, Jensen MT, Biering-Sorensen T, Mogelvang R, Fritz-Hansen T, Vilsboll T, et al. Burden of uncontrolled metabolic risk factors and left ventricular structure and function in patients with type 2 diabetes mellitus. J Am Heart Assoc. (2018) 7:e008856. doi: 10.1161/JAHA.118.008856
72. Burroughs Pena M, Swett K, Schneiderman N, Spevack DM, Ponce SG, Talavera GA, et al. Cardiac structure and function with and without metabolic syndrome: the Echocardiographic Study of Latinos (Echo-SOL). BMJ Open Diabetes Res Care. (2018) 6:e000484. doi: 10.1136/bmjdrc-2017-000484
73. Crendal E, Walther G, Vinet A, Dutheil F, Naughton G, Lesourd B, et al. Myocardial deformation and twist mechanics in adults with metabolic syndrome: impact of cumulative metabolic burden. Obes (Silver Spring). (2013) 21:E679–86. doi: 10.1002/oby.v21.12
74. de las Fuentes L, Brown AL, Mathews SJ, Waggoner AD, Soto PF, Gropler RJ, et al. Metabolic syndrome is associated with abnormal left ventricular diastolic function independent of left ventricular mass. Eur Heart J. (2007) 28:553–9. doi: 10.1093/eurheartj/ehl526
75. Zhou D, Ye Z, Nie Z, Chen C, Luo S, Yan M, et al. Positive additive interaction effects of age, sex, obesity, and metabolic syndrome on left ventricular dysfunction. J Diabetes. (2024) 16:e13478. doi: 10.1111/1753-0407.13478
76. Burger PM, Koudstaal S, Dorresteijn JAN, Savarese G, van der Meer MG, de Borst GJ, et al. Metabolic syndrome and risk of incident heart failure in non-diabetic patients with established cardiovascular disease. Int J Cardiol. (2023) 379:66–75. doi: 10.1016/j.ijcard.2023.03.024
77. Kosmala W, Przewlocka-Kosmala M, Wojnalowicz A, Mysiak A, Marwick TH. Integrated backscatter as a fibrosis marker in the metabolic syndrome: association with biochemical evidence of fibrosis and left ventricular dysfunction. Eur Heart J Cardiovasc Imaging. (2012) 13:459–67. doi: 10.1093/ejechocard/jer291
78. Iribarren C, Karter AJ, Go AS, Ferrara A, Liu JY, Sidney S, et al. Glycemic control and heart failure among adult patients with diabetes. Circulation. (2001) 103:2668–73. doi: 10.1161/01.cir.103.22.2668
79. Wang YC, Liang CS, Gopal DM, Ayalon N, Donohue C, Santhanakrishnan R, et al. Preclinical systolic and diastolic dysfunctions in metabolically healthy and unhealthy obese individuals. Circ Heart Fail. (2015) 8:897–904. doi: 10.1161/CIRCHEARTFAILURE.114.002026
80. Grandi AM, Maresca AM, Giudici E, Laurita E, Marchesi C, Solbiati F, et al. Metabolic syndrome and morphofunctional characteristics of the left ventricle in clinically hypertensive nondiabetic subjects. Am J hypertension. (2006) 19:199–205. doi: 10.1016/j.amjhyper.2005.07.024
81. Aksoy S, Durmus G, Ozcan S, Toprak E, Gurkan U, Oz D, et al. Is left ventricular diastolic dysfunction independent from presence of hypertension in metabolic syndrome? An echocardiographic study. J Cardiol. (2014) 64:194–8. doi: 10.1016/j.jjcc.2014.01.002
82. Widya RL, de Mutsert R, den Heijer M, le Cessie S, Rosendaal FR, Jukema JW, et al. Association between hepatic triglyceride content and left ventricular diastolic function in a population-based cohort: the Netherlands epidemiology of obesity study. Radiology. (2016) 279:443–50. doi: 10.1148/radiol.2015150035
83. Leclerc J, Arsenault M, Despres JP, Brassard P, Gaudreault V, Bergeron J, et al. Determinants of improvement in left ventricular diastolic function following a 1-year lifestyle modification program in abdominally obese men with features of the metabolic syndrome. Metab syndrome related Disord. (2016) 14:483–91. doi: 10.1089/met.2016.0021
84. Kosmala W, Przewlocka-Kosmala M, Szczepanik-Osadnik H, Mysiak A, O’Moore-Sullivan T, Marwick TH. A randomized study of the beneficial effects of aldosterone antagonism on LV function, structure, and fibrosis markers in metabolic syndrome. JACC Cardiovasc imaging. (2011) 4:1239–49. doi: 10.1016/j.jcmg.2011.08.014
85. Evaristi MF, Poirier B, Chenede X, Lefebvre AM, Roccon A, Gillot F, et al. A G-protein-biased S1P1 agonist, SAR247799, improved LVH and diastolic function in a rat model of metabolic syndrome. PloS One. (2022) 17:e0257929. doi: 10.1371/journal.pone.0257929
86. Park SH, Farooq MA, Gaertner S, Bruckert C, Qureshi AW, Lee HH, et al. Empagliflozin improved systolic blood pressure, endothelial dysfunction and heart remodeling in the metabolic syndrome ZSF1 rat. Cardiovasc Diabetol. (2020) 19:19. doi: 10.1186/s12933-020-00997-7
87. Cuijpers I, Papageorgiou AP, Carai P, Herwig M, Mugge A, Klein T, et al. Linagliptin prevents left ventricular stiffening by reducing titin cleavage and hypophosphorylation. J Cell Mol Med. (2021) 25:729–41. doi: 10.1111/jcmm.16122
88. Takatsu M, Nakashima C, Takahashi K, Murase T, Hattori T, Ito H, et al. Calorie restriction attenuates cardiac remodeling and diastolic dysfunction in a rat model of metabolic syndrome. Hypertension (Dallas Tex: 1979). (2013) 62:957–65. doi: 10.1161/HYPERTENSIONAHA.113.02093
89. Hermida N, Markl A, Hamelet J, Van Assche T, Vanderper A, Herijgers P, et al. HMGCoA reductase inhibition reverses myocardial fibrosis and diastolic dysfunction through AMP-activated protein kinase activation in a mouse model of metabolic syndrome. Cardiovasc Res. (2013) 99:44–54. doi: 10.1093/cvr/cvt070
90. Ho KK, Pinsky JL, Kannel WB, Levy D. The epidemiology of heart failure: the Framingham Study. J Am Coll Cardiol. (1993) 22:6a–13a. doi: 10.1016/0735-1097(93)90455-A
91. Ayalon N, Gopal DM, Mooney DM, Simonetti JS, Grossman JR, Dwivedi A, et al. Preclinical left ventricular diastolic dysfunction in metabolic syndrome. Am J Cardiol. (2014) 114:838–42. doi: 10.1016/j.amjcard.2014.06.013
92. Hwang Y-C, Jee JH, Kang M, Rhee E-J, Sung J, Lee M-K. Metabolic syndrome and insulin resistance are associated with abnormal left ventricular diastolic function and structure independent of blood pressure and fasting plasma glucose level. Int J Cardiol. (2012) 159:107–11. doi: 10.1016/j.ijcard.2011.02.039
Keywords: metabolic syndrome, left ventricular diastolic dysfunction, heart failure with preserved ejective fraction, hypertension, diabetes mellitus
Citation: Zhou D, Lin S, Liu Z, Yuan J, Ren H, Tan H, Guo Y and Jiang X (2025) Metabolic syndrome, left ventricular diastolic dysfunction and heart failure with preserved ejective fraction. Front. Endocrinol. 16:1544908. doi: 10.3389/fendo.2025.1544908
Received: 13 December 2024; Accepted: 07 March 2025;
Published: 14 April 2025.
Edited by:
Paola Di Pietro, University of Salerno, ItalyReviewed by:
Kaijian Hou, Shantou University, ChinaChangjiang Yu, Harbin Medical University Cancer Hospital, China
Copyright © 2025 Zhou, Lin, Liu, Yuan, Ren, Tan, Guo and Jiang. This is an open-access article distributed under the terms of the Creative Commons Attribution License (CC BY). The use, distribution or reproduction in other forums is permitted, provided the original author(s) and the copyright owner(s) are credited and that the original publication in this journal is cited, in accordance with accepted academic practice. No use, distribution or reproduction is permitted which does not comply with these terms.
*Correspondence: Xin Jiang, amlhbmd4aW5zekAxNjMuY29t