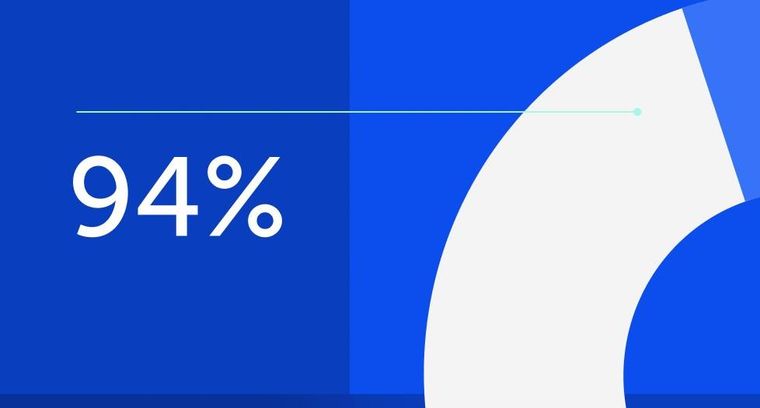
94% of researchers rate our articles as excellent or good
Learn more about the work of our research integrity team to safeguard the quality of each article we publish.
Find out more
ORIGINAL RESEARCH article
Front. Endocrinol., 16 April 2025
Sec. Reproduction
Volume 16 - 2025 | https://doi.org/10.3389/fendo.2025.1530692
This article is part of the Research TopicNervous System and Reproduction: A Highly Integrative PartnershipView all 6 articles
Introduction: The hypothalamus-pituitary-gonad axis is controlled by gonadotropins and by a direct neural pathway to the gonads. New evidence suggests the existence of neural connection from the hippocampus to the hypothalamus that can regulate its function. It could be a new control on the well-regulated hormonal and neural connection to the gonads and hence in reproduction. The objective of this study was to analyze the effects of independent lesion of the dorsal or ventral hippocampus in the female rat on the onset of puberty, follicular growth and serum concentration of sex steroid and gonadotropins.
Methods: Prepubertal female rats of the CII-ZV strain, 21 days old, were used. Ventral (VH-L) or dorsal (DH-L) hippocampus lesions by the administration of ibotenic acid were performed using stereotaxic surgery. Controls were sham-operated (VH-Sham and DH- Sham), a fifth group was used as absolute control. At 30 days of age all groups underwent novel object recognition tests (NORT).
Results: Data from memory using NORT showed a decrease both in short- and long-term memory in the animals in the VH- L and DH-L groups compared to their respective sham-operated controls and the absolute control group. Similarly, injured rats presented delayed vaginal opening and in first vaginal estrus, a decrease in the number of healthy ovarian follicles and an increase in follicular atresia. The ventral or dorsal hippocampus lesions also caused a significant decrease in the secretion of estradiol and progesterone, an increased plasma testosterone. Only DH-L group showed a significant decrease in serum FSH concentrations compared to their respective control groups.
Discussion: These results show for the first time that the hippocampus participates in a stimulatory manner, that could overcome the gonadotropic control by acting by a neural connection to the gonads giving a novel integrative mechanism between learning processes with neuroendocrine mechanism regulating the ovary function.
Reproduction is an essential process in life that guides biological evolution. It allows each individual to unconsciously compete to perpetuate itself in the next generation. The differential reproductive success of individuals drives the evolution of species (1). The hippocampus is one of the key brain structures involved in learning and memory, which are essential processes in the survival and evolution of species (2–4). Perception of information from outside and inside the organism activates sensory pathways that transport this information to the sensory cortex. The hippocampus receives and processes this information, particularly in the CA3 region (5).
The hippocampus has connections with almost all parts of the limbic system, including especially the amygdala, hippocampal gyrus, cingulate gyrus, hypothalamus and other areas closely related to the hypothalamus. Almost all types of sensory experience originate instantaneous activation in several parts of the hippocampus, which in turn distributes many output signals to the hypothalamus and other parts of the diencephalon via the fornix (6–8). This brain region is divided into dorsal (DH), intermediate (IH) and ventral (VH) hippocampi. While the DH is involved in cognitive functions and its dysfunction leads to amnesia, the HI only separates both regions (9–11). VH is responsible for emotional control, such as frustrations and regulates the activity of the hypothalamic-pituitary-adrenal (HPA) axis (12). It is particularly interesting to consider the role of this ventral portion and that it is strongly linked to circuits related to defensive behavior in the hypothalamus. A clear pattern of stronger projections of the monoaminergic systems to more ventral parts of the hippocampus is also apparent (11), which does not rule out its involvement in reproductive processes.
It is well known that reproduction is regulated by the hypothalamus-pituitary-gonads neuroendocrine axis (HPG). In addition to neuroendocrine regulation, a direct neural pathway has been identified linking the gonads to central nervous system structures, including the hypothalamus and amygdala, which play a critical role in the regulation of gonadal function (13–15). The ovaries receive neural input through noradrenergic, peptidergic, and cholinergic fibers (16–20). In return, they transmit sensory information to the central nervous system via immunoreactive fibers for substance P (SP) and calcitonin gene-related peptide (CGRP) (17–19, 21). Within the dorsal root ganglion, this information is processed by the activation of glutamatergic neurotransmission through both ionotropic and metabotropic receptors (22). In the female rat, denervation of these sensory fibers by capsaicin administration results in delayed puberty, decreased number of ovarian follicles and increased follicular atresia, these changes are related to lower secretion of serum estradiol concentrations (23, 24) and with a significant decrease in the activity of the noradrenergic and serotonergic systems of the anterior hypothalamus (24).
In rodents, sectioning of peripheral sensory nerves causes a decrease in both the number and activity of hippocampal CA1 and CA3 pyramidal neurons (25, 26) and a reconfiguration of neuronal connections between CA3 and CA1 (25). There is evidence that suggests the participation of the hippocampus in the regulation of gonadal functions. The hippocampus has been reported to send information to various hypothalamic regions, which control behavioral, endocrine, and autonomic responses (11). Through the lateral septum, hippocampal information is projected to the median hypothalamus, which is involved in regulating the expression of sexual behavior (27). In Huntington’s disease (HD), neurodegeneration of the hippocampus is observed, which is associated with cognitive dysfunctions, as well as gonadal dysfunction. Interestingly, various studies conducted in humans and animals with HD show changes in sexual behavior, degeneration of energetic GnRH neurons, gonadal atrophy, and alterations in the HPG axis (28, 29). Recently, changes in gene expression in the hippocampus during the estrous cycle have been shown to suggest that variations in sex steroid hormone secretion modify hippocampal GABAergic neural tone (30). Therefore, it allows us to suggest a bidirectional relationship between the hippocampus and the gonads. The objective of this study was to analyze the effects of independent lesion of the dorsal or ventral hippocampus in the female rat on the onset of puberty, follicular growth and serum concentration of sex steroid and gonadotropins.
Twenty-five female neonatal rats of the CII-ZV strain were used, the animals were kept in the “Claude Bernard” biotherium (University of Puebla) under conditions of constant light/dark cycle of 14/10 hrs. and were provided food and water ad libitum. All the procedures described in this study were approved by the Internal Committee for the Care and Use of Laboratory Animals (CICUAL) (100519168-UALVIEP-22/1) and adhered to the ethical framework for Care and Use of Laboratory Animals in accordance with NOM-062-ZOO-1999. Efforts were made to minimize the animals’ suffering and reduce the number of animals used. At 21 days of age, the rats were divided equally into five groups with n=5. Group 1 was lesioned on the ventral hippocampus (VH-L), group 2 lesioned in the dorsal hippocampus (DH-L). Two more groups in the same areas underwent a simulated operation (VH-Sham, DH-Sham) and finally a group of untouched animals (Control).
The VH-L and DH-L protocol was performed according to previous reports (31, 32). Briefly, considering the day of birth as postnatal day (PD) 0 at PD21, the female offspring (n=20) were anesthetized by ketamine-xylazine and fixed to a neonatal adapter (33) attached to a stereotaxic frame. Once the ventral and dorsal coordinates of the hippocampus were located (VH: anteroposterior: -5.2 mm, medial-lateral: +/- 5.0 mm, both from Bregma; and dorso-ventral: -6.5 mm from dura mater; DH: anteroposterior: -4.2 mm, medial-lateral: +/- 2.6 mm, both from Bregma; and dorso-ventral: -3.0 mm) 0.3 uL of ibotenic acid (IBO) (10 ug/uL; Sigma, St. Louis, MO) for animals with VH-L and DH-L (n=10) or the same vehicle volume (0,1 M phosphate-buffered saline [PBS], pH 7,4) for sham rats (n=10) were administered bilaterally during 3 minutes to ensure a proper administration, then the animals were sutured and administrated using a dose of the antibiotic enrofloxacin and the analgesic ketoprofen (0.20 ml/100 g live weight s.c.) and were left to recover under the indirect heat of a lamp to regain their temperature. For the intact group (n=5), the offspring were not disturbed until reaching puberty. The diagram representing the experimental protocol is shown in Figure 1A.
Figure 1. Experimental protocol. (A) At PD21 rats in the surgery group (continuous line) were administered PBS bilaterally into the ventral hippocampus to form the sham group, or IBO to form the VH-L and DH-L groups. The intact group (dashed line) was not disturbed until weaning (21 days post-surgery). At PD30–33 days post-surgery, motor activity and NORT were assessed. After reaching puberty, rats were administered an overdose of ketamine-xylazine to obtain samples. (B) NORT after lesion at 31 days of postnatal life (short- and long-term memory) of the CII-ZV strain with VH-L, DH-L, sham lesion and untouched animals’ group. The values indicate the recognition or discrimination index (IR) to objects at 0 h, 2h and 24 (h) The means ± SEM are presented with n=5 rats per group *p= 0.028, **p= 0.009, ***p< 0.001. (C) Motor activity after lesion at 30 days postnatal life of CII-ZV stain. Analysis of the total distance traveled of PD30 rats in open field motor activity post lesion with IBO or PBS. DH-L group traveled farther compared to the sham group and control group, (One-way ANOVA and Bonferroni for post hoc; *p = 0.019, **p= 0.002).
At prepuberal age, the behavior of the animals was evaluated. Locomotor activity was estimated at PD30. Then, from PD31 to PD33, the novel object recognition test (NORT) was performed.
An open field test was carried out for these ages (n = 5 per group), rats were kept thirty minutes before test in the work area with red lighting for their adaptation. This test began placing the animal on square 7 of a wooden box (60x60x60 cm) previously cleaned and divided into nine squares of 20x20 cm each for 10 minutes, the observer registered the number of squares visited considering as a visit when all four paws of the animal were inside the square (34–36) After the test, the animal was removed, and the corresponding cleaning was carried out to avoid influencing the conduct of the rodents by animal’s odor.
To evaluate the memory performance of rats, the NORT was employed as previously reported with some modifications (36–38). The test lasted three days and was assessed between 7:00–12:00 h, in a dark room with red light (30 lx) and isolated noise. The first day, the rats explored an open field box (60 x 60 x 60 cm) during 10 min (habituation stage). On the second and third day, the animals explored two objects located in the box for 6 min as described below. The second day, 24 hrs. after the habituation stage, the rats explored two objects that had the same characteristics (familiarization stage). 2 hrs. after the familiarization stage, an object was replaced by a novel one and the other object remained without change (short term retention stage). Finally, on the third day, 24 hrs. after the short-term retention stage, the novel object that had previously been changed was replaced by a new one and the other object remained unchanged. (long term retention stage). For each rat, the amount of exploration time with the new object was counted. The recognition index (IR) was determined with the following equation: IR = TN/(TK +TN). Where IR is the recognition index, TN is the time that each animal explores the new object and TK is the time that each animal explores the known object.
The vaginal smears were collected between 09:00 and 10:00 hrs. daily, after the vaginal opening. The smears were identified by microscopic examination of the cell types and were classified into 1 of 4 phases of the estrous cycle: proestrus, estrus, metestrus, or diestrus. The phases of the estrous cycle were identified according to the predominant cell type observed in the vaginal smears when examined with an optical microscope (39). Only rats that were shown to be in the estrus phase were sacrificed. Upon reaching puberty, the rats were anesthetized with an overdose of ketamine-xylazine (0.20 ml/100 g live weight i.p.) and perfused intracardially with 0.9% NaCl. Immediately at least 3 ml of blood was collected by cardiac puncture and the sample was centrifuged at 3000 rpm for 15 minutes to obtain the serum subsequent evaluations of estradiol, progesterone, testosterone, FSH and LH concentrations, as described below.
ELISA was used to measure estradiol (pg/ml), progesterone (ng/ml), testosterone (ng/ml), FSH (mlU/ml) and LH (mlU/ml) serum levels, using reagents and protocol supplied by MEXLAB (México). The absorbance of the reaction of all hormones was measured at a wavelength of 450 nm in a spectrophotometer. The intra- and inter assay percent variation coefficients for estradiol were 6.3 and 6.8, progesterone 5.3 and 9.68, testosterone 5.76 and 6.87, FSH 6.3 and 6.8 and for LH 6.18 and 8.13, respectively.
For the morphological parameters of the dendritic spines, the Golgi-Cox staining method was used with some modifications (40). These staining methods were performed as previously described (41–43). Once the animals were sacrificed the brains were removed from the skull, immersed in Golgi-Cox solution for 30 days in the dark. After that, 200 μm thick coronal sections from the regions of interest were obtained using a vibratome (Campden Instruments, Leicester, UK) and collected on gelatin-coated microscope slides and immersed in ammonium hydroxide (30 min), then in Kodak Rapid Fixer (30 min). Subsequently, the slides were rinsed in distilled water, dehydrated with sequential increasing ethanol concentration baths (50% for 1 min, 75% for 1 min, 90% for 1 min and 100% twice for 5 min), followed by rinsing in xylene solution (15 minutes). Finally, the slides were mounted with resinous microscopy medium.
The basal dendritic tree of the pyramidal neurons of the CA1, CA3, DG regions of the hippocampus was located, the stereotaxic atlas of (44) was used as a guide. For each rat, five dendritic segments were reproduced (a length 5.4 cm equivalent to 30 μm, of the most distal dendrite of the neurons in each hemisphere of the hippocampal areas using a lucid camera with a 1000x magnification (Leica DMLS microscope) to estimate the density of the dendritic spines On the same dendritic segments, the characterization of the spines according to their shape was performed as previously described with some modifications (41). For this purpose, using a 2x lens with a 2000x magnification (Leica DMLS microscope), one hundred spines were classified according to the shape of their head and neck into five groups: mushroom spines (prominent head with well identified neck), thin spines (elongated spines with head/neck of similar thickness), thick spines (wide spines with an indistinguishable neck), branched spines (spines with two heads), and unclassified spines (inconsistent with any of above criteria). A trained observer who was unaware of the experimental conditions performed the morphological and density classification of the spines.
The ovaries were fixed in Bouin solution, embedded in paraffin wax, serially sectioned at a thickness of 10 μm and stained with hematoxylin-eosin. All ovarian sections were analyzed with an optical microscope. (Primo Star microscope, ZEISS, Jena, Germany).
To determine the follicular growth, the number of follicles with presence (antral) or absence (preantral) of the follicular antrum was quantified. Those follicles that had a fluid filled space and several layers of granulosa cells were classified as antral follicles; those with only one layer of flattened pregranulosa cells and one of two layers of cubic granulosa cells were considered preantral follicles. For this evaluation, both healthy follicles and those showing signs of atresia were included for the analysis.
Follicular maturation is calculated by quantifying follicle types (45), considering the following parameters: Primordial: primary oocyte surrounded by a single layer of flattened pregranulosa cells. Primary: primary oocyte surrounded by a single layer of cubic granulosa cells. Secondary: primary oocyte surrounded by more than one layer of granulosa cells and with antral formation (fluid filled space). Tertiary: (also named and known as ovulatory follicle): late stage (or large) antral follicle (approximately 700 μm in diameter) where the primary oocyte is surrounded by an oophorus group, and the follicle will respond to the sudden increases in LH level.
The number of healthy and atretic follicles was quantified. Considering signs of follicular atresia: n nuclear pyknosis of granulosa cells, theca thickening, desquamation and oocyte fragmentation were analyzed.
All the data are presented as the mean ± standard error of the mean (SEM). A normality test was performed to verify that all sample distributions were normal and had equal variances. For the data analysis, a one-way ANOVA and two-way ANOVA were performed with the lesion and the testing phase as independent variables with a post-hoc uncorrected Bonferroni test for multiple comparisons using the GraphPad Prism 8 software (GraphPad Software, CA, USA). Values of P<0.05 were considered significant.
Behavioral tests in a novel environment were employed to assess short-term and long-term memory, as well as the effects of VH-L, DH-L, and sham lesions in rats corresponding to each region, along with an intact control group, through the novel object recognition task.
The behavioral testing of NORT in CII-ZV strain rats were performed after the lesion, at 31 days postnatal life with a recognition index (IR) that evaluates the time spent with a novel object versus a familiar object, a two-way ANOVA was performed with F (8, 60) =7.650, p<0.001 for the lesion phase followed by Turkey´s test p<0.05. It is observed that in the training phase the recognition indices of both groups are close to 0.5, this suggests that the groups do not present any preference for any of the objects. After two hours of the first exposure phase, a decrease in the recognition index of the experimental groups (VH-L, VH-Sham, DH-L, DH-Sham) can be observed in comparison with the control group, and lastly at 24 hours after the last exposure, a decrease in the recognition index is shown in the same experimental groups. On the other hand, the VH-L group presents a decreased recognition index for both short term and long-term memory phase compared to its VH-Sham control group (Figure 1B). This was performed using a two-way ANOVA: ***p< 0.001, **p= 0.009, *p= 0.028.
The effect of lesions on the ventral hippocampus (VH-L) and dorsal hippocampus (DH-L) were evaluated. The analysis shows that dorsal hippocampal (DH-L) lesioned rats have a higher motor activity F (4, 20) = 6.492, p =0.0016) compared to the untouched animals (Control) ** p = 0.0020 and their control group (DH-Sham) * p =0.0117, one-way ANOVA and Tukey’s test for multiple comparisons were performed (Figure 1C).
The registry of the ages of the vaginal opening and first estrus of injured animals at 21 days of age in dorsal or ventral hippocampus and a control group showed significant differences (Vaginal opening: F (4, 35) = 22.29 p<0.0001; First estrus: F (4, 35) = 24.97 p<0.0001.The ibotenic acid lesioning performed in the dorsal and ventral hippocampal regions in 21-day postnatal rats resulted in delayed age at vaginal opening (VH-L: 47.38 ± 0.77 vs VH-Sham: 44.63 ± 0.37 vs Control group: 39.50 ± 0.56; DH-L: 48.63 ± 1.28 vs DH-Sham: 44.13 ± 0.29 vs Control group: 39.50 ± 0.56); compared to rats with only mechanical injury and in animals without any damage (Figure 2A). Upon analyzing the age at first estrus, significant differences were presented in all experimental groups (VH-L: 47.63 ± 0.65 vs VH-Sham: 44.88 ± 0.39 vs Control group: 39.50 ± 0.56; DH-L: 49.50 ± 1.37 vs DH-Sham: 44.63 ± 0.26 vs Control group: 39.50 ± 0.56) (Figure 2B).
Figure 2. Parameters of puberty. (A) Comparison of the age at vaginal opening and (B) first estrus in pubertal female rats with dorsal (DH-L) and ventral (VH-L) hippocampal lesions, sham lesions, and an untouched control group of the CII-ZV strain. The VH-L and DH-L groups exhibited a significant delay in puberty onset compared to the sham and control groups (One-way ANOVA followed by Tukey’s post hoc test; ***p < 0.0001, **p < 0.001, *p < 0.03).
When analyzing the follicular population, the results showed a decrease in the number of healthy follicles and an increase in atretic follicles in the experimental groups compared to the VH-Sham, DH-Sham, and the Control group (F (1.733, 5.198) = 120.3) (Figure 3A). Two-way ANOVA, Tukey’s test for multiple comparisons.
Figure 3. Effects of hippocampal lesion on the HPG axis. (A) Lesions induced in the ventral (VH-L) and dorsal (DH-L) hippocampus resulted in a decrease in healthy follicles and an increase in atretic follicles compared to the sham groups (*p = 0.03, ***p = 0.0007). (B) The VH-L and DH-L groups exhibited a significant reduction in preantral and antral follicles compared to the control group, while showing an increase relative to the sham groups. Data are presented as mean ± SEM, with n= 4 rats per group (two-way ANOVA followed by Tukey’s post hoc test; *p = 0.01, ***p < 0.0001). (C) VH-L and DH-L increase serum testosterone secretion relative to their control groups. (D) The lesions performed in ventral and dorsal hippocampus cause a decrease in serum estradiol release. (E) The serum progesterone release was significantly decreased in the ventral and dorsal hippocampal lesion groups. Statistics were performed by one way ANOVA test, Tukey’s test for multiple comparisons; *p = 0.0143, #p =0.0121, **p = 0.0020, ***p < 0.001. (F) VH-L and DH-L decrease serum FSH secretion. (G) Shows the decrease in serum LH concentration in animals with ventral and dorsal hippocampal lesion. (One-way ANOVA, Tukey’s test for multiple comparisons; *p = 0.0127, #p = 0.0399, **p = 0.0074, ***p =0.0003.
The classification of follicles in preantral and antral, shows that the ventral or dorsal hippocampal lesion causes a significant decrease in both types of follicles observed compared to the control group, two-way ANOVA, turkey tests for multiple comparisons: *p=0.01, ***p<0.0001 (Figure 3B).
The ibotenic acid lesion in ventral and dorsal hippocampal areas significantly increased serum testosterone concentration (Figure 3C), (F (4, 20) = 136.5, p=0.0001), p < 0.0001 vs. control, p < 0.0001 vs. sham lesion). Regarding serum estradiol concentrations, a decrease was observed (Figure 3D), (F(4, 20) = 52.71, p < 0.0001), p < 0.0001 VH-L, DH-L vs Control group, p=0.0413 VH L vs VH L Sham and p < 0.0001 DH-L vs DH-Sham) as for serum progesterone results a significant decrease was also observed (Figure 3E), (F(4, 20) = 27.85, p < 0.0001), p < 0.0001 VH-L, DH-L vs control group, p=0.0020 VH-L vs VH-Sham and p < 0.0001 DH-L vs DH-Sham.
The lesions performed in the ventral and dorsal hippocampal areas significantly decreased serum FSH concentration (Figure 3F), (F (4, 20) = 164.7, p <0.0001), p =0.0127 VH-L vs Control group, p =0.0074: DH-L vs Control group, p= 0.399 DH-L vs DH-Sham. Regarding serum LH concentrations, the lesions performed in the VH-L and DH-L groups caused a decrease in said hormone, compared to the control group. (Figure 3G), (F(4, 20) = 136.5, p < 0.0001), p = 0.0074 VH-L vs Control group, p <0.0003: DH-L vs Control group.
Rats with VH-L and DH-L had severe alterations in the dendritic spine density and dynamics in the region CA1, CA3, DG the hippocampus (Figures 4E, F, 5E, F). In the dorsal hippocampal area (Figure 4A), the ventral hippocampal lesion (VH-L) reduced the density of basilar dendritic spines of pyramidal and granular cells (F(2, 8) = 33.69, p=0.0001), p<0.0001 vs. control, p<0.0001 vs. sham lesion), in all three regions evaluated. Regarding the morphological classification of dendritic spines VH-L reduced the number of mushroom spines in the CA1 region (Figure 4B), (F(2, 12) = 184.2, p < 0.0001; p < 0.0001 vs. Control and Sham lesion) and thin spines (F(2, 12) = 8.960, p=0.0042; p=0.005 vs. Sham lesion), but increased the number of thick spines (F(2, 12) = 59.15, p<0.0001; p<0.0001 vs. control, p<0.001 vs. sham lesion). The VH-L had no effect on the number of bifurcated and unsorted spines of these neurons.
Figure 4. Structural neuroplasticity of the dorsal hippocampus of CII-ZV rats with VH-L. (A) VH-L decreases the number of spines in these neurons (CA1, CA3 and DG). (B-D) Morphological classification of dendritic spines. (B) number of spines in the CA1 region. VH-L reduces the number of mushrooms and thin spines and increases the number of thick spines. (C, D), Number of dendritic spines in CA3 and DG region, VH-L decreases the number of fungal spines and increases the number of thick spines (two-way ANOVA, Bonferroni test for multiple comparisons: *p = 0.033, **p = 0.005, ***p < 0.001. (E) Dendritic spine density and morphology. Representative photomicrographs of distal segments of the basal dendritic arbor of pyramidal neurons from CA1, CA3, and DG regions of the dorsal hippocampus from each of the groups, (n=5 per group; scale bar = 10 μm). (F) Representative photomicrographs of each type of spine, (scale bar=2 μm).
Figure 5. Structural neuroplasticity of the ventral hippocampus of CII-ZV rats with DH-L. (A) DH Lesion decreases the number of spines in these neurons (CA1, CA3 and DG). (B-D) Morphological classification of dendritic spines. (B) number of spines in the CA1 region. DH Lesion reduces the number of mushroom and thin spines and increases the number of thick spines. (C, D), Number of dendritic spines in CA3 and DG region, DH Lesion decreases the number of fungal spines and increases the number of thick spines percentages of these spine types (two-way ANOVA, Bonferroni test for multiple comparisons: *p= 0.02, ***p = 0.001. (E) Dendritic spine density and morphology. Representative photomicrographs of distal segments of the basal dendritic arbor of pyramidal neurons from CA1, CA3, and DG regions of the ventral hippocampus from each of the groups, (n=5 per group; scale bar = 10 μm). (F) Representative photomicrographs of each type of spine, (scale bar=2 μm).
Morphological classification of dendritic spines VH L reduced the number of fungal spines in the CA3 region (Figure 4C), (F(2, 12) = 51.61, p<0.0001; p<0.0001 vs. Control and Sham lesion) and of thin spines no significant differences were observed (F(2, 12) = 2.295, p=0.1432), but it did increase the number thick spines (F(2, 12) = 86.26, p < 0.0001; p <0.0001 vs. Control and Sham lesion). The VH-L also had no effect on the number of bifurcated and unsorted spines of these neurons.
Lastly, in the DG region there was also a decrease in mushroom like spines (Figure 4D) (F(2, 12) = 75.32, p <0.0001; p <0.0001 vs. Control and Sham lesion) and thin spines no significant differences were observed, but the number of thick spines increased (F(2, 12) = 8.196, p = 0.0057; p =0.0005 vs. control and p =0.001 sham lesion). The VH-L had no effect on the number of bifurcated and unsorted spines of these neurons.
In the ventral hippocampal area (Figure 5A), the dorsal hippocampal lesion (DH-L) reduced the density of basilar dendritic spines of pyramidal cells (F(2, 8) = 25.95, p=0.0003), p < 0.0001 vs. Control, p < 0.0001 vs. Sham lesion), in all three hippocampal regions evaluated. Regarding the morphological classification of dendritic spines the DH-L reduced the number of mushrooms spines in CA1 region (Figure 5B), (F(2, 12) = 139.2, p < 0.0001; p < 0.0001 vs. Control and Sham lesion) and thin spines (F(2, 12) = 13.40, p=0.0009; p = 0.001 vs. Sham lesion), but increased the number of thick spines (F(2, 12) = 59.6, p <0.0001; p <0.0001 vs. Control and vs. Sham lesion). DH-L had no effect on the number of bifurcated and unsorted spines of these neurons. The morphological classification of dendritic spines of the CA3 region (Figure 5C), a decrease of mushroom-like spines was observed (F(2, 12) = 61.78, p <0.0001; p < 0.0001 versus Control and Sham lesion) and thin spines (F(2, 12) = 10.63, p =0.0022; p = 0.0266 versus Sham lesion), but did increase the number of thick spines (F(2, 12) = 94.77, p < 0.0001; p <0.0001 versus control and sham lesion). DH-L also had no effect on the number of bifurcated and unsorted spines of these neurons.
Lastly, the morphological classification of dendritic spines of the DG region (Figure 5D), a decrease of mushroom-like spines was also observed in the (F(2, 12) = 48.18, p <0.0001; p < 0.0001 vs. control and sham lesion) and thin spines no significant differences were observed, but the number of thick spines increased (F(2, 12) = 61.16, p < 0.0001; p <0.0001 vs. Control and Sham lesion). DH-L had no effect on the number of bifurcated and unsorted spines of these neurons.
In the present study, the VH-lesioned animals presented a significant decrease in the short- and long-term memory retention index compared to the VH-Sham and Control groups. The decrease in serum estrogen concentration in these animals may explain their results in the NORT test, since estrogens are a determining factor in the memory and learning process (46, 47). In ovariectomized female mice with global cerebral ischemia present a decrease in estrogen receptor proteins and aromatase expression in the hippocampus that affect their ability to discriminate tasks, such as learning and memory (48). Estrogen receptors Erα and ERβ are critical for memory preservation after cerebral ischemia in adult female rodents (49). Interestingly, analysis of locomotor activity showed increased hyperactivity in DH-injured animals compared with DH-Sham and Control groups. Locomotor activity to novel environment allows assessment of dopaminergic tone in the NAcc indirectly (32). Therefore, increased locomotor activity suggests hyperactivity of the mesolimbic pathway in DH-lesioned rats, altering the connectivity of the corticolimbic system, as well as mediating in part the effects of biologically significant stimuli on learning (50).
The observed decrease in short- and long-term memory in the Sham groups compared to the Control group may be attributed to alterations in the afferent and efferent projection fields of the hippocampus. These alterations could impact structures such as the septum, amygdala, cortex, and nucleus accumbens, likely as a consequence of the surgical procedure used to access the ventral or dorsal hippocampus (51, 52). Therefore, the differences observed between the hippocampal lesion groups and their respective Sham groups in the novel object recognition test (NORT) are more likely associated with the specific effects of the ibotenic acid-induced lesion (52, 53). Furthermore, the observed reduction in dendritic spine density in the Sham groups, along with an even more pronounced reduction in the hippocampal lesion groups, further supports this hypothesis.
In groups with ventral or dorsal hippocampal lesions induced by ibotenic acid, it is likely that mechanisms mediated by proinflammatory substances, which increase the activity of glial cells such as astrocytes and microglia. This increased glial activity is primarily driven by the activation of microglial mGluR5 receptors (54). Notably, this pathway may also trigger anti-inflammatory mechanisms that enhance cognitive function, as observed in both the prefrontal cortex and hippocampus (55).
Our results showed that the lesion performed in the VH or DH caused a delay in the age of vaginal opening and first vaginal estrus compared to animals with sham lesion and the control group. Interestingly, these animals also showed a significant decrease in the density of dendritic spines of CA1, CA3 and DG hippocampal pyramidal neurons compared to their respective control groups. The lesion in VH or DH probably blocked important sensory pathways that activate neuroendocrine mechanisms that regulate follicular growth and maturation, secretion of sex steroid hormones and gonadotropins, and thus fertility. It is transcendental that hippocampal lesions not only affected short- and long-term memory, but also provoked changes in reproductive parameters, in the concentration of steroid and gonadotropic hormones, in ovarian morphology and in the modulation of neuronal plasticity. Some studies provide evidence that allows us to consider the possibility that the hippocampus maintains bidirectional communication with the gonads (28, 29). It remains unclear whether this pathway is direct or mediated indirectly through the hypothalamus, amygdala, and cortical pathways (56–60). We have shown that sensory denervation causes a delay in puberty and in the age of first vaginal estrus (23, 24, 61). Puberty is an important period for the development of cognitive and signal integration functions and determines hippocampal function in adulthood (62). Estrogens stimulate the neuroendocrine mechanisms that initiate puberty (63, 64). Endocrine-disrupting compounds with estrogenic activity can delay puberty and exert detrimental effects on neurogenesis in the dentate gyrus, potentially leading to impaired learning and memory (65). There are few data evidencing the role of hippocampal involvement in the onset of puberty; however, estrogens may be a key factor as they regulate synaptic plasticity in the hippocampus of female rodents (66–69). In the ovariectomized rat, estrogen administration increases the density of dendritic spines in CA1 pyramidal cells of the hippocampus (68). Estrogen-induced plastic changes in CA1, CA3, and DG pyramidal neurons could contribute to the activation of neuroendocrine mechanisms that regulate the onset of puberty.
There is a bidirectional relationship between the hypothalamic-pituitary-gonadal (HPG) axis and the hippocampus, which, along with other structures of the limbic system, exhibits a high density of gonadotropin-releasing hormone (GnRH) receptors (70). Activation of these receptors in hippocampal pyramidal neurons within the CA1 and CA3 regions has been shown to enhance long-term neuronal excitability (71, 72).
It is possible that lesions in the ventral or dorsal hippocampus resulted in increased GABAergic tone and a reduction in excitatory inputs to the hypothalamus, which may have inhibited the secretion of GnRH (64). This effect, in conjunction with decreased estrogen secretion, could have contributed to the delayed onset of puberty. The impact may also be evident at the time of first vaginal estrus, as animals with hippocampal lesions exhibit reduced gonadotropin secretion. Supporting this hypothesis, during ovarian senescence, the loss of negative feedback from estrogen and inhibin leads to elevated GnRH concentrations, resulting in increased follicle-stimulating hormone (FSH) and luteinizing hormone (LH) levels (73). Notably, hippocampal pyramidal neurons, which are particularly vulnerable to Alzheimer’s disease, express LH receptors (74), and their activation has been associated with biochemical and cellular processes linked to neurodegeneration (72). Further research is required to determine whether the hippocampus can influence the pulsatility of GnRH secretion in the hypothalamus across different stages of life, beyond its established role during senescence.
Our results support the idea of a bidirectional connection between the hippocampus and the ovaries. This connection is advantageous for both components, the ovaries require neural and neuroendocrine signals, mediated in part by the hippocampus, for the control of follicular growth and the secretion of sex steroid hormones, in particular estrogens. These have receptors in the hippocampus to modulate its neuronal plasticity, the establishment of connections, neuronal survival and control of GABAergic tone (75–77). In support of the above, it has been demonstrated that in women, early bilateral salpingo-oophorectomy (BSO) causes a decrease in connectivity between both hippocampi and between the anterior and posterior part of the left hippocampus, decreasing associative memory (78). Women with BSO have an increased risk of developing Alzheimer’s disease and accelerated cognitive decline (79, 80). Sensory information sent from the ovaries to the CNS stimulates follicular growth in a stimulatory manner (23, 61). Any sensory information received by the hippocampus is processed by the entorhinal cortex, enters the hippocampus through two pathways: the trisynaptic loop from the dentate gyrus CA3 and CA1 and then back to the entorhinal cortex and the temporoammonic pathway, from the entorhinal cortex directly to CA1 (81). The CA1 region then sends axonal projections to the subiculum. Afferents from the subiculum travel through the corticohypothalamic tract, passing through the paraventricular nucleus (PVN) and the suprachiasmatic nucleus before terminating in the dorsomedial hypothalamus and mammillary bodies. Additionally, cells in the PVN project directly to the ventral subiculum and send some projections to hippocampal area CA1 (58). The hippocampus would translate excitatory inputs into inhibitory outputs, as well as encode a specific pattern to generate signals that regulate endocrine secretions (76). In this work, the ventral or dorsal lesion of the hippocampus could have modified this bidirectional communication, which would explain the increase in folliculogenesis and failures in steroidogenesis. This response has been seen in other studies that have induced local or peripheral denervation. The increase in follicles that we observed was mostly atretic, is understandable because the signals controlling the neural and neuroendocrine mechanisms that regulate hormone secretion are affected, for example, as is a lower secretion of progesterone and estradiol. Several studies on the involvement of ovarian nerves in controlling the secretion of sex steroid hormones (23, 24, 61, 82–84) and gonadotropins (61, 85, 86) support this hypothesis.
The significant reduction in the number of ovarian follicles and the lower secretion of sex steroid hormones observed in the sham animals compared to the control group reinforce the idea that the surgical procedure used to access the ventral or dorsal hippocampus affects afferent and efferent pathways. These pathways not only contribute to memory and learning processes (87, 88) but also play a role in the regulation of ovarian functions, including follicular growth and hormone secretion.
The increase in serum testosterone concentrations observed in the hippocampal lesion groups would also explain this increase in atretic follicles (89–93) as well as the total increase in follicles as an inducing effect of androgens on the onset of follicular growth (90, 93, 94). It would also account for the increased locomotion observed in DH-lesioned animals by an increase in hippocampal excitability, as has been shown in the hyperandrogenism model with the female PCOS rat (95). The decrease in serum FSH concentrations observed in the hippocampal lesion groups would be another factor contributing to the increase in atretic follicles in hippocampal lesion animals (92, 96, 97). This diminished response in ovarian functions, gonadotropin secretion and alterations in neural communication between the hippocampus and ovaries would explain a greater difficulty for females with hippocampal lesion, more dorsal than ventral, to become pregnant.
The decrease of estrogens in the group of animals with hippocampal lesion would explain the decrease in the density of dendritic spines, especially of the mushroom type. Estrogens are known to regulate reproductive behavior, neurodevelopment, plasticity and hippocampal function (68, 98). Woolley and collaborators (69) demonstrated the effect of estradiol and progesterone in ovariectomized rats on the density of dendritic spines in CA1 pyramidal cells of the hippocampus of female rats, where they observed an increase in dendritic density after twenty-four hours following the administration of estradiol, On the other hand, they showed that progesterone has a biphasic effect on spine density, i.e., progesterone administration after estradiol causes an initial increase, but then causes a much sharper decrease.
These results are similar to those previously found by several groups (99–101) who suggest natural fluctuations in dendritic spine density and estrogen receptor expression during the estrous cycle. It cannot be ruled out that the changes observed in the dendritic morphology of CA1, CA3 and DG pyramidal neurons in animals with lesions in VH and DH are associated with the effect of an interruption in communication in the ovary-hypothalamus-hippocampus neural pathway that stimulated a delay in puberty and the aforementioned changes in follicular growth. It is possible that the lesion in the ventral or dorsal hippocampus may have caused intact hippocampal areas to have less excitatory sensory stimulus from the ovaries. In support of this, the plasticity of dendritic spines may be modified by several factors, such as actin that is regulated by Rho family GTPases and AMPA receptors that promote dendritic spine formation by increasing Ca2+ levels in hippocampal neurons. Estrogen-regulated NMDA receptors have also been associated with an increase in the size and density of dendritic spines of hippocampal pyramidal neurons (102–105). In addition to changes in dendritic morphology potentially induced by decreased estradiol levels, these alterations may also result from reduced levels of neurotrophic factors, such as brain-derived neurotrophic factor (BDNF) and inducible nerve growth factor B (NGFB), which are observed in animals with hippocampal injury (106–108). These factors may contribute to functional alterations in the hippocampus and its neural circuits, affecting communication with other central nervous system structures (109, 110). This supports our hypothesis that hippocampal lesions lead to disruptions in bidirectional communication between the hippocampus and ovaries.
Kolb’s group (111) suggest that changes in neuronal morphology may be completely independent. Hippocampal damage may have important implications not only for the function of the hippocampus itself such as memory and learning, but also for understanding the behavior of the individual. In addition, the involvement and influence of ovarian hormones may have particularly important implications for differential synaptic plasticity at different times of the menstrual cycle. Estrogens are known to promote some spines to change from a thin filopodia-like structure to spines with larger, well-defined, mushroom-like necks and heads (112–114). Consistent with the increase in thin and stubby spines observed and a decrease in mushroom-like spines and the decrease in serum estradiol concentrations observed in the VH-L and DH-L groups in our study.
The existence of a hippocampus-pituitary-gonads axis has been proposed, whose function would be to ensure hippocampal plasticity in the adult brain through the regulation of steroid secretion of ovarian and neural origin (114–116). According to our results, the neuroendocrine mechanisms that regulate reproduction and cognitive processes are mutually dependent, and it would be necessary to keep this in mind from now on for a holistic analysis of reproductive and cognitive problems. Probably, the existence of a Hippocampus-Hypothalamus-Hypothalamus-Pituitary-Gonads (H-HPG) axis would have to be considered.
In summary, these results show, for the first time, that the hippocampus participates in a stimulatory manner in the regulation of ovarian function, through a neural connection with the gonads. This leads to an integrative pathway between learning processes and the neuroendocrine mechanisms that regulate reproduction. Further studies are required to anatomically delineate the neural pathway between the ovaries and the hippocampus.
The raw data supporting the conclusions of this article will be made available by the authors, without undue reservation.
The animal study was approved by Internal Committee for the Care and Use of Laboratory Animals (CICUAL) and adhered to the ethical framework for Care and Use of Laboratory Animals in accordance with NOM-060-ZOO1999. The study was conducted in accordance with the local legislation and institutional requirements.
EC: Conceptualization, Data curation, Formal Analysis, Funding acquisition, Investigation, Methodology, Project administration, Resources, Software, Supervision, Validation, Visualization, Writing – original draft, Writing – review & editing. UQ: Conceptualization, Data curation, Formal Analysis, Funding acquisition, Investigation, Methodology, Project administration, Resources, Software, Supervision, Validation, Visualization, Writing – original draft, Writing – review & editing. LM: Conceptualization, Data curation, Formal Analysis, Methodology, Software, Validation, Writing – original draft. RR: Conceptualization, Data curation, Formal Analysis, Methodology, Validation, Writing – original draft. GF: Conceptualization, Data curation, Formal Analysis, Funding acquisition, Methodology, Software, Validation, Writing – original draft.
The author(s) declare that financial support was received for the research and/or publication of this article. The authors declare that they have received financial support for the research, authorship and/or publication of this article. The authors acknowledge financial support from VIEP (BUAP), CONAHCYT grant (No. 798636), CONCYTEP and FES Zaragoza, UNAM.
We want to thank Dr. Francisco Ramos Collazo for his help with the animal care. The authors wish to thanks Dr. Rubén Antonio Vazquez-Roque for helpful comments and suggestions. Thanks to Professor Mayra Karina Feria for editing the English language text.
The authors declare that the present study was conducted in the absence of any commercial or financial relationships that could be construed as a potential conflict of interest.
The author(s) declare that no Generative AI was used in the creation of this manuscript.
All claims expressed in this article are solely those of the authors and do not necessarily represent those of their affiliated organizations, or those of the publisher, the editors and the reviewers. Any product that may be evaluated in this article, or claim that may be made by its manufacturer, is not guaranteed or endorsed by the publisher.
1. Ellison PT. En tierra fértil. Historia natural de la reproducción humana. México: Fondo de Cultura Económica (2006).
2. Pine DS, Wise SP, Murray EA. Evolution, emotion, and episodic engagement. Am J Psychiatry. (2021) 178:701–14. doi: 10.1176/appi.ajp.2020.20081187
3. Fleischer AW, Frick KM. New perspectives on sex differences in learning and memory. Trends Endocrinol metabolism: TEM. (2023) 34:526–38. doi: 10.1016/j.tem.2023.06.003
4. Zacks O, Jablonka E. The evolutionary origins of the Global Neuronal Workspace in vertebrates. Neurosci consciousness. (2023) 2):niad020. doi: 10.1093/nc/niad020
5. Behrendt RP. Hippocampus and consciousness. Rev Neurosci. (2013) 24:239–66. doi: 10.1515/revneuro-2012-0088
6. Guyton T, Gilson AJ. Clinical use of the vertex view in brain scanning. J nuclear medicine: Off publication Soc Nuclear Med. (1967) 8:384–5.
7. Herman JP, Figueiredo H, Mueller NK, Ulrich-Lai Y, Ostrander MM, Choi DC, et al. Central mechanisms of stress integration: hierarchical circuitry controlling hypothalamo-pituitary-adrenocortical responsiveness. Front neuroendocrinol. (2003) 24:151–80. doi: 10.1016/j.yfrne.2003.07.001
8. Herman JP, Ostrander MM, Mueller NK, Figueiredo H. Limbic system mechanisms of stress regulation: hypothalamo-pituitary-adrenocortical axis. Prog Neuropsychopharmacol Biol Psychiatry. (2005) 29:1201–13. doi: 10.1016/j.pnpbp.2005.08.006
9. Dedovic K, Duchesne A, Andrews J, Engert V, Pruessner JC. The brain and the stress axis: the neural correlates of cortisol regulation in response to stress. NeuroImage. (2009) 47:864–71. doi: 10.1016/j.neuroimage.2009.05.074
10. Fanselow MS, Dong HW. Are the dorsal and ventral hippocampus functionally distinct structures? Neuron. (2010) 65:7–19. doi: 10.1016/j.neuron.2009.11.031
11. Strange BA, Witter MP, Lein ES, Moser EI. Functional organization of the hippocampal longitudinal axis. Nat Rev Neurosci. (2014) 15:655–69. doi: 10.1038/nrn3785
12. Flores G, Morales-Medina JC, Diaz A. Neuronal and brain morphological changes in animal models of schizophrenia. Behav Brain Res. (2016) 301:190–203. doi: 10.1016/j.bbr.2015.12.034
13. Gerendai I, Banczerowski P, Halász B. Functional significance of the innervation of the gonads. Endocrine. (2005) 28:309–18. doi: 10.1385/ENDO:28:3:309
14. Gerendai I, Halász B. Central nervous system structures connected with the endocrine glands. findings obtained with the viral transneuronal tracing technique. Exp Clin Endocrinol diabetes: Off journal German Soc Endocrinol [and] German Diabetes Assoc. (2000) 108:389–95. doi: 10.1055/s-2000-8134
15. Gerendai I, Tóth IE, Boldogköi Z, Medveczky I, Halász B. CNS structures presumably involved in vagal control of ovarian function. J autonomic nervous system. (2000) 80:40–5. doi: 10.1016/s0165-1838(00)00071-0
16. Klein CM, Burden HW. Anatomical localization of afferent and postganglionic sympathetic neurons innervating the rat ovary. Neurosci Lett. (1988) 85:217–22. doi: 10.1016/0304-3940(88)90354-0
17. Papka RE, Cotton JP, Traurig HH. Comparative distribution of neuropeptide tyrosine-, vasoactive intestinal polypeptide-, substance P-immunoreactive, acetylcholinesterase-positive and noradrenergic nerves in the reproductive tract of the female rat. Cell Tissue Res. (1985) 242:475–90. doi: 10.1007/BF00225412
18. Dees WL, Ahmed CE, Ojeda SR. Substance P- and vasoactive intestinal peptide-containing fibers reach the ovary by independent routes. Endocrinology. (1986) 119:638–41. doi: 10.1210/endo-119-2-638
19. Calka J, McDonald JK, Ojeda SR. The innervation of the immature rat ovary by calcitonin gene-related peptide. Biol Reprod. (1988) 39:1215–23. doi: 10.1095/biolreprod39.5.1215
20. Mayerhofer A, Dissen GA, Costa ME, Ojeda SR. A role for neurotransmitters in early follicular development: induction of functional follicle-stimulating hormone receptors in newly formed follicles of the rat ovary. Endocrinology. (1997) 138:3320–9. doi: 10.1210/endo.138.8.5335
21. Ojeda SR, Costa ME, Katz KH, Hersh LB. Evidence for the existence of substance P in the prepubertal rat ovary. I. Biochem physiologic Stud Biol Reprod. (1985) 33:286–95. doi: 10.1095/biolreprod33.2.286
22. Fernández-Montoya J, Avendaño C, Negredo P. The glutamatergic system in primary somatosensory neurons and its involvement in sensory input-dependent plasticity. Int J Mol Sci. (2017) 19:69. doi: 10.3390/ijms19010069
23. Morán C, Morales L, Razo RS, Apolonio J, Quiróz U, Chavira R, et al. Effects of sensorial denervation induced by capsaicin injection at birth or on day three of life, on puberty, induced ovulation and pregnancy. Life Sci. (2003) 73:2113–25. doi: 10.1016/s0024-3205(03)00598-8
24. Quiróz U, Morales-Ledesma L, Morán C, Trujillo A, Domínguez R. Lack of sensorial innervation in the newborn female rats affects the activity of hypothalamic monoaminergic system and steroid hormone secretion during puberty. Endocrine. (2014) 46:309–17. doi: 10.1007/s12020-013-0055-3
25. Milshtein-Parush H, Frere S, Regev L, Lahav C, Benbenishty A, Ben-Eliyahu S, et al. Sensory deprivation triggers synaptic and intrinsic plasticity in the hippocampus. Cereb Cortex. (2017) 27:3457–70. doi: 10.1093/cercor/bhx084
26. Li F, Zhang YY, Jing XM, Yan CH, Shen XM. The influence of forepaw palmar sensorimotor deprivation on learning and memory in young rats. Neurosci Res. (2009) 63:17–23. doi: 10.1016/j.neures.2008.09.008
27. Risold PY, Swanson LW. Structural evidence for functional domains in the rat hippocampus. Science. (1996) 272:1484–6. doi: 10.1126/science.272.5267.1484
28. Du X, Pang TY, Mo C, Renoir T, Wright DJ, Hannan AJ. The influence of the HPG axis on stress response and depressive-like behaviour in a transgenic mouse model of Huntington's disease. Exp Neurol. (2015) 263:63–71. doi: 10.1016/j.expneurol.2014.09.009
29. Selvaraj K, Manickam N, Kumaran E, Thangadurai K, Elumalai G, Sekar A, et al. Deterioration of neuroregenerative plasticity in association with testicular atrophy and dysregulation of the hypothalamic-pituitary-gonadal (HPG) axis in Huntington's disease: A putative role of the huntingtin gene in steroidogenesis. J Steroid Biochem Mol Biol. (2020) 197:105526. doi: 10.1016/j.jsbmb.2019.105526
30. Wijesena HR, Nonneman DJ, Keel BN, Lents CA. Gene expression in the amygdala and hippocampus of cyclic and acyclic gilts. J Anim Sci. (2022) 100:skab372. doi: 10.1093/jas/skab372
31. Lipska BK, Jaskiw GE, Weinberger DR. Postpubertal emergence of hyperresponsiveness to stress and to amphetamine after neonatal excitotoxic hippocampal damage: a potential animal model of schizophrenia. Neuropsychopharmacol: Off Publ Am Coll Neuropsychopharmacol. (1993) 9:67–75. doi: 10.1038/npp.1993.44
32. Flores G, Barbeau D, Quirion R, Srivastava LK. Decreased binding of dopamine D3 receptors in limbic subregions after neonatal bilateral lesion of rat hippocampus. J neurosci: Off J Soc Neurosci. (1996) 16:2020–6. doi: 10.1523/JNEUROSCI.16-06-02020.1996
33. Sierra A, Camacho-Abrego I, Escamilla C, Negrete-Diaz JV, Rodriguez-Sosa L, Flores G. Plataforma economica para cirugia estereotaxica en rata neonata [Economical body platform for neonatal rats stereotaxic surgery. Rev neurología. (2009) 48:141–6. doi: 10.33588/rn.4803.2008571
34. Muñoz-Arenas G, Pulido G, Treviño S, Vázquez-Roque R, Flores G, Moran C, et al. Effects of metformin on recognition memory and hippocampal neuroplasticity in rats with metabolic syndrome. Synapse. (2020) 74:e22153. doi: 10.1002/syn.22153
35. Aguilar-Hernández L, Vázquez-Hernández AJ, de-Lima-Mar DF, Vázquez-Roque RA, Tendilla-Beltrán H, Flores G. Memory and dendritic spines loss, and dynamic dendritic spines changes are age-dependent in the rat. J Chem Neuroanat. (2020) 110:101858. doi: 10.1016/j.jchemneu.2020.101858
36. Diaz A, Treviño S, Vázquez-Roque R, Venegas B, Espinosa B, Flores G, et al. The aminoestrogen prolame increases recognition memory and hippocampal neuronal spine density in aged mice. Synapse. (2017) 71:e21987. doi: 10.1002/syn.21987
37. Hernández-Hernández EM, Caporal Hernandez K, Vázquez-Roque RA, Díaz A, de la Cruz F, Florán B, et al. The neuropeptide-12 improves recognition memory and neuronal plasticity of the limbic system in old rats. Synapse. (2018) 72:e22036. doi: 10.1002/syn.22036
38. Vidal B, Vázquez-Roque RA, Gnecco D, Enríquez RG, Floran B, Díaz A, et al. Curcuma treatment prevents cognitive deficit and alteration of neuronal morphology in the limbic system of aging rats. Synapse. (2017) 71:e21952. doi: 10.1002/syn.21952
39. Cora MC, Kooistra L, Travlos G. Vaginal cytology of the laboratory rat and mouse: review and criteria for the staging of the estrous cycle using stained vaginal smears. Toxicologic Pathol. (2015) 43:776–93. doi: 10.1177/0192623315570339
40. Gibb R, Kolb B. A method for vibratome sectioning of Golgi-Cox stained whole rat brain. J Neurosci Methods. (1998) 79:1–4. doi: 10.1016/s0165-0270(97)00163-5
41. Flores G, Alquicer G, Silva-Gómez AB, Zaldivar G, Stewart J, Quirion R, et al. Alterations in dendritic morphology of prefrontal cortical and nucleus accumbens neurons in post-pubertal rats after neonatal excitotoxic lesions of the ventral hippocampus. Neuroscience. (2005) 133:463–70. doi: 10.1016/j.neuroscience.2005.02.021
42. Flores-Vivaldo YM, Camacho-Abrego I, Picazo O, Flores G. Pregnancies alters spine number in cortical and subcortical limbic brain regions of old rats. Synapse. (2019) 73:e22100. doi: 10.1002/syn.22100
43. Pinzón-Parra C, Vidal-Jiménez B, Camacho-Abrego I, Flores-Gómez AA, Rodríguez-Moreno A, Flores G. Juvenile stress causes reduced locomotor behavior and dendritic spine density in the prefrontal cortex and basolateral amygdala in Sprague-Dawley rats. Synapse. (2019) 73:e22066. doi: 10.1002/syn.22066
45. Picut CA, Dixon D, Simons ML, Stump DG, Parker GA, Remick AK. Postnatal ovary development in the rat: morphologic study and correlation of morphology to neuroendocrine parameters. Toxicologic Pathol. (2015) 43:343–53. doi: 10.1177/0192623314544380
46. Dohanich G, Korol D, Shors T. Steroids, learning and memory. In: Pfaff DW, Arnold AP, Etgen AM, Fahrbach SE, Rubin RT, editors. Hormones, brain and behavior, 2nd ed. Amsterdam, Boston, USA: Elsevier Academic Press (2009). p. 539–76. doi: 10.1016/B978-008088783-8.00015-2
47. Zurkovsky L, Brown SL, Korol DL. Estrogen modulates place learning through estrogen receptors in the hippocampus. Neurobiol Learn Memory. (2006) 86:336–43. doi: 10.1016/j.nlm.2006.07.008
48. Ma Y, Liu M, Yang L, Zhang L, Guo H, Qin P, et al. Loss of estrogen efficacy against hippocampus damage in long-term OVX mice is related to the reduction of hippocampus local estrogen production and estrogen receptor degradation. Mol Neurobiol. (2020) 57:3540–51. doi: 10.1007/s12035-020-01960-z
49. Zafer D, Aycan N, Ozaydin B, Kemanli P, Ferrazzano P, Levine JE, et al. Sex differences in Hippocampal Memory and Learning following Neonatal Brain Injury: Is There a Role for Estrogen Receptor-α? Neuroendocrinology. (2019) 109:249–56. doi: 10.1159/000499661
50. Beninger RJ. The role of dopamine in locomotor activity and learning. Brain Res. (1983) 287:173–96. doi: 10.1016/0165-0173(83)90038-3
51. Wood GK, Lipska BK, Weinberger DR. Behavioral changes in rats with early ventral hippocampal damage vary with age at damage. Brain Res Dev Brain Res. (1997) 101:17–25. doi: 10.1016/s0165-3806(97)00050-3
52. Marenco S, Weinberger DR. The neurodevelopmental hypothesis of schizophrenia: following a trail of evidence from cradle to grave. Dev Psychopathol. (2000) 12:501–27. doi: 10.1017/s0954579400003138
53. Vázquez-Roque RA, Solis O, Camacho-Abrego I, Rodríguez-Moreno A, deL Cruz F, Zamudio S, et al. Dendritic morphology of neurons in prefrontal cortex and ventral hippocampus of rats with neonatal amygdala lesion. Synapse (New York N.Y.). (2012) 66:373–82. doi: 10.1002/syn.21517
54. Drouin-Ouellet J, Brownell AL, Saint-Pierre M, Fasano C, Emond V, Trudeau LE, et al. Neuroinflammation is associated with changes in glial mGluR5 expression and the development of neonatal excitotoxic lesions. Glia. (2011) 59:188–99. doi: 10.1002/glia.21086
55. Dogra S, Conn PJ. Metabotropic glutamate receptors as emerging targets for the treatment of schizophrenia. Mol Pharmacol. (2022) 101:275–85. doi: 10.1124/molpharm.121.000460
56. Phelps EA. Human emotion and memory: interactions of the amygdala and hippocampal complex. Curr Opin Neurobiol. (2004) 14:198–202. doi: 10.1016/j.conb.2004.03.015
57. Rocha MI, Mestriner RG, Hermel EE, Xavier LL, Rasia-Filho AA, Achaval M. Neuronal somatic volume of posteroventral medial amygdala cells from males and across the estrous cycle of female rats. Neurosci Lett. (2007) 420:110–5. doi: 10.1016/j.neulet.2007.04.043
58. Stranahan AM, Lee K, Mattson MP. Contributions of impaired hippocampal plasticity and neurodegeneration to age-related deficits in hormonal pulsatility. Ageing Res Rev. (2008) 7:164–76. doi: 10.1016/j.arr.2007.12.004
59. Beissner F, Preibisch C, Schweizer-Arau A, Popovici RM, Meissner K. Psychotherapy with somatosensory stimulation for endometriosis-associated pain: the role of the anterior hippocampus. Biol Psychiatry. (2018) 84:734–42. doi: 10.1016/j.biopsych.2017.01.006
60. Yang Y, Wang JZ. From structure to behavior in basolateral amygdala-hippocampus circuits. Front Neural circuits. (2017) 11:86. doi: 10.3389/fncir.2017.00086
61. Morán C, Morales L, Quiróz U, Domínguez R. Effects of unilateral or bilateral superior ovarian nerve section in infantile rats on follicular growth. J Endocrinol. (2000) 166:205–11. doi: 10.1677/joe.0.1660205
62. Yildirim M, Mapp OM, Janssen WG, Yin W, Morrison JH, Gore AC. Postpubertal decrease in hippocampal dendritic spines of female rats. Exp Neurol. (2008) 210:339–48. doi: 10.1016/j.expneurol.2007.11.003
63. Xu J, Liao L, Ning G, Yoshida-Komiya H, Deng C, O'Malley BW. The steroid receptor coactivator SRC-3 (p/CIP/RAC3/AIB1/ACTR/TRAM-1) is required for normal growth, puberty, female reproductive function, and mammary gland development. Proc Natl Acad Sci United States America. (2000) 97:6379–84. doi: 10.1073/pnas.120166297
64. Sultan C, Gaspari L, Maimoun L, Kalfa N, Paris F. Disorders of puberty. Best Pract Res Clin obstetrics gynaecol. (2018) 48:62–89. doi: 10.1016/j.bpobgyn.2017.11.004
65. Bourguignon JP, Franssen D, Gérard A, Janssen S, Pinson A, Naveau E, et al. Early neuroendocrine disruption in hypothalamus and hippocampus: developmental effects including female sexual maturation and implications for endocrine disrupting chemical screening. J neuroendocrinol. (2013) 25:079–1087. doi: 10.1111/jne.12107
66. Woolley CS, Gould E, Frankfurt M, McEwen BS. Naturally occurring fluctuation in dendritic spine density on adult hippocampal pyramidal neurons. J neurosci: Off J Soc Neurosci. (1990) 10:4035–9. doi: 10.1523/JNEUROSCI.10-12-04035.1990
67. Woolley CS, McEwen BS. Estradiol mediates fluctuation in hippocampal synapse density during the estrous cycle in the adult rat. J neurosci: Off J Soc Neurosci. (1992) 12:2549–54. doi: 10.1523/JNEUROSCI.12-07-02549.1992
68. Woolley CS, McEwen BS. Roles of estradiol and progesterone in regulation of hippocampal dendritic spine density during the estrous cycle in the rat. J Comp Neurol. (1993) 336:293–306. doi: 10.1002/cne.903360210
69. Woolley CS, Weiland NG, McEwen BS, Schwartzkroin PA. Estradiol increases the sensitivity of hippocampal CA1 pyramidal cells to NMDA receptor-mediated synaptic input: correlation with dendritic spine density. J neurosci: Off J Soc Neurosci. (1997) 17:1848–59. doi: 10.1523/JNEUROSCI.17-05-01848.1997
70. Leblanc P, Crumeyrolle M, Latouche J, Jordan D, Fillion G, L'Heritier A, et al. Characterization and distribution of receptors for gonadotropin-releasing hormone in the rat hippocampus. Neuroendocrinology. (1988) 48:482–8. doi: 10.1159/000125053
71. Lu F, Yang JM, Wu JN, Chen YC, Kao YH, Tung CS, et al. Activation of gonadotropin-releasing hormone receptors produces neuronal excitation in the rat hippocampus. Chin J Physiol. (1999) 42:67–71.
72. Meethal SV, Smith MA, Bowen RL, Atwood CS. The gonadotropin connection in Alzheimer's disease. Endocrine. (2005) 26:317–26. doi: 10.1385/ENDO:26:3:317
73. Kermath BA, Gore AC. Neuroendocrine control of the transition to reproductive senescence: lessons learned from the female rodent model. Neuroendocrinology. (2012) 96:1–12. doi: 10.1159/000335994
74. Lei ZM, Rao CV, Kornyei JL, Licht P, Hiatt ES. Novel expression of human chorionic gonadotropin/luteinizing hormone receptor gene in brain. Endocrinology. (1993) 132:2262–70. doi: 10.1210/endo.132.5.8477671
75. Barker JM, Galea LA. Repeated estradiol administration alters different aspects of neurogenesis and cell death in the hippocampus of female, but not male, rats. Neuroscience. (2008) 152:888–902. doi: 10.1016/j.neuroscience.2007.10.071
76. Lathe R. Hormones and the hippocampus. J Endocrinol. (2001) 169:205–31. doi: 10.1677/joe.0.1690205
77. Galea LA, Wainwright SR, Roes MM, Duarte-Guterman P, Chow C, Hamson DK. Sex, hormones and neurogenesis in the hippocampus: hormonal modulation of neurogenesis and potential functional implications. J neuroendocrinol. (2013) 25:1039–61. doi: 10.1111/jne.12070
78. Brown A, Gervais NJ, Rieck J, Almey A, Gravelsins L, Reuben R, et al. Women's brain health: midlife ovarian removal affects associative memory. Mol Neurobiol. (2023) 60:6145–59. doi: 10.1007/s12035-023-03424-6
79. Rocca WA, Bower JH, Maraganore DM, Ahlskog JE, Grossardt BR, de Andrade M, et al. Increased risk of cognitive impairment or dementia in women who underwent oophorectomy before menopause. Neurology. (2007) 69:1074–83. doi: 10.1212/01.wnl.0000276984.19542.e6
80. Bove R, Secor E, Chibnik LB, Barnes LL, Schneider JA, Bennett DA, et al. Age at surgical menopause influences cognitive decline and Alzheimer pathology in older women. Neurology. (2014) 82:222–9. doi: 10.1212/WNL.0000000000000033
81. Amaral DG, Witter MP. The three-dimensional organization of the hippocampal formation: a review of anatomical data. Neuroscience. (1989) 31:571–91. doi: 10.1016/0306-4522(89)90424-7
82. Morales-Ledesma L, Vieyra E, Ramírez DA, Trujillo A, Chavira R, Cárdenas M, et al. Effects on steroid hormones secretion resulting from the acute stimulation of sectioning the superior ovarian nerve to pre-pubertal rats. Reprod Biol endocrinol: RB&E. (2012) 10:88. doi: 10.1186/1477-7827-10-88
83. Uchida S, Kagitani F. Autonomic nervous regulation of ovarian function by noxious somatic afferent stimulation. J Physiol sciences: JPS. (2015) 65:1–9. doi: 10.1007/s12576-014-0324-9
84. Uchida S, Kagitani F. Neural mechanisms involved in the noxious physical stress-induced inhibition of ovarian estradiol secretion. Anatomical record (Hoboken N.J.: 2007). (2019) 302:904–11. doi: 10.1002/ar.24078
85. Lara HE, McDonald JK, Ahmed CE, Ojeda SR. Guanethidine-mediated destruction of ovarian sympathetic nerves disrupts ovarian development and function in rats. Endocrinology. (1990) 127:2199–209. doi: 10.1210/endo-127-5-2199
86. Albuquerque-Araújo WI, Rosa-e-Silva AA, Antunes-Rodrigues J, Favaretto AL. Is ovarian adrenergic innervation essential to gonadal function in adult rats? Arch Physiol Biochem. (1995) 103:109–13. doi: 10.3109/13813459509007572
87. Daenen EW, Wolterink G, Gerrits MA, Van Ree JM. Amygdala or ventral hippocampal lesions at two early stages of life differentially affect open field behaviour later in life; an animal model of neurodevelopmental psychopathological disorders. Behav Brain Res. (2002) 131:67–78. doi: 10.1016/s0166-4328(01)00350-3
88. Leuner B, Gould E. Structural plasticity and hippocampal function. Annu Rev Psychol. (2010) 61:111–C3. doi: 10.1146/annurev.psych.093008.100359
89. Li JR, Shen T, Wang YL, Wei QW, Shi FX. Expression of matrix metalloproteinases and ovarian morphological changes in androgenized cyclic female Guinea pigs. Tissue Cell. (2016) 48:72–80. doi: 10.1016/j.tice.2015.10.003
90. Chou CH, Chen MJ. The effect of steroid hormones on ovarian follicle development. Vitamins hormones. (2018) 107:155–75. doi: 10.1016/bs.vh.2018.01.013
91. Puttabyatappa M, Guo X, Dou J, Dumesic D, Bakulski KM, Padmanabhan V. Developmental programming: sheep granulosa and theca cell-specific transcriptional regulation by prenatal testosterone. Endocrinology. (2020) 161:bqaa094. doi: 10.1210/endocr/bqaa094
92. Casarini L, Paradiso E, Lazzaretti C, D'Alessandro S, Roy N, Mascolo E, et al. Regulation of antral follicular growth by an interplay between gonadotropins and their receptors. J assisted Reprod Genet. (2022) 39:893–904. doi: 10.1007/s10815-022-02456-6
93. Garzia E, Galiano V, Guarnaccia L, Marfia G, Murru G, Guermandi E, et al. Basal serum level of Δ4-androstenedione reflects the ovaries' ability to respond to stimulation in IVF cycles: setting up a new reliable index of both ovarian reserve and response. J assisted Reprod Genet. (2022) 39:1917–26. doi: 10.1007/s10815-022-02546-5
94. Oduwole OO, Huhtaniemi IT, Misrahi M. The roles of luteinizing hormone, follicle-stimulating hormone and testosterone in spermatogenesis and folliculogenesis revisited. Int J Mol Sci. (2021) 22:12735. doi: 10.3390/ijms222312735
95. Zhang J, Lai W, Tang Y, Gao J, Zhou X, Chen L. Hyperandrogenism decreases seizure threshold in a rat model of polycystic ovary syndrome. Neuroendocrinology. (2024) 114:1005–17. doi: 10.1159/000540523
96. Chu YL, Xu YR, Yang WX, Sun Y. The role of FSH and TGF-β superfamily in follicle atresia. Aging. (2018) 10:305–21. doi: 10.18632/aging.101391
97. Hirshfield AN. Development of follicles in the mammalian ovary. Int Rev cytol. (1991) 124:43–101. doi: 10.1016/s0074-7696(08)61524-7
98. Hösli E, Hösli L. Cellular localization of estrogen receptors on neurones in various regions of cultured rat CNS: coexistence with cholinergic and galanin receptors. Int J Dev neurosci: Off J Int Soc Dev Neurosci. (1999) 17:317–30. doi: 10.1016/s0736-5748(99)00038-6
99. Gould E, Woolley CS, Frankfurt M, McEwen BS. Gonadal steroids regulate dendritic spine density in hippocampal pyramidal cells in adulthood. J neurosci: Off J Soc Neurosci. (1990) 10:1286–91. doi: 10.1523/JNEUROSCI.10-04-01286.1990
100. Brusco J, Wittmann R, de Azevedo MS, Lucion AB, Franci CR, Giovenardi M, et al. Plasma hormonal profiles and dendritic spine density and morphology in the hippocampal CA1 stratum radiatum, evidenced by light microscopy, of virgin and postpartum female rats. Neurosci Lett. (2008) 438:346–50. doi: 10.1016/j.neulet.2008.04.063
101. Chen JR, Yan YT, Wang TJ, Chen LJ, Wang YJ, Tseng GF. Gonadal hormones modulate the dendritic spine densities of primary cortical pyramidal neurons in adult female rat. Cereb Cortex (New York N.Y.: 1991). (2009) 19:2719–27. doi: 10.1093/cercor/bhp048
102. Ethell IM, Pasquale EB. Molecular mechanisms of dendritic spine development and remodeling. Prog Neurobiol. (2005) 75:161–205. doi: 10.1016/j.pneurobio.2005.02.003
103. Sekino Y, Kojima N, Shirao T. Role of actin cytoskeleton in dendritic spine morphogenesis. Neurochem Int. (2007) 51:92–104. doi: 10.1016/j.neuint.2007.04.029
104. Bellot A, Guivernau B, Tajes M, Bosch-Morató M, Valls-Comamala V, Muñoz FJ. The structure and function of actin cytoskeleton in mature glutamatergic dendritic spines. Brain Res. (2014) 1573:1–16. doi: 10.1016/j.brainres.2014.05.024
105. Joensuu M, Lanoue V, Hotulainen P. Dendritic spine actin cytoskeleton in autism spectrum disorder. Prog Neuropsychopharmacol Biol Psychiatry. (2018) 84:362–81. doi: 10.1016/j.pnpbp.2017.08.023
106. Lipska BK, Khaing ZZ, Weickert CS, Weinberger DR. BDNF mRNA expression in rat hippocampus and prefrontal cortex: effects of neonatal ventral hippocampal damage and antipsychotic drugs. Eur J Neurosci. (2001) 14:135–44. doi: 10.1046/j.1460-9568.2001.01633.x
107. Molteni R, Lipska BK, Weinberger DR, Racagni G, Riva MA. Developmental and stress-related changes of neurotrophic factor gene expression in an animal model of schizophrenia. Mol Psychiatry. (2001) 6:285–92. doi: 10.1038/sj.mp.4000865
108. Bhardwaj SK, Beaudry G, Quirion R, Levesque D, Srivastava LK. Neonatal ventral hippocampus lesion leads to reductions in nerve growth factor inducible-B mRNA in the prefrontal cortex and increased amphetamine response in the nucleus accumbens and dorsal striatum. Neuroscience. (2003) 122:669–76. doi: 10.1016/j.neuroscience.2003.08.016
109. Thierry AM, Gioanni Y, Dégénétais E, Glowinski J. Hippocampo-prefrontal cortex pathway: anatomical and electrophysiological characteristics. Hippocampus. (2000) 10:411–9. doi: 10.1002/1098-1063(2000)10:4<411::AID-HIPO7>3.0.CO;2-A
110. Vázquez-Roque RA, Ramos B, Tecuatl C, Juárez I, Adame A, de la Cruz F, et al. Chronic administration of the neurotrophic agent cerebrolysin ameliorates the behavioral and morphological changes induced by neonatal ventral hippocampus lesion in a rat model of schizophrenia. J Neurosci Res. (2012) 90:288–306. doi: 10.1002/jnr.22753
111. Kolb B, Forgie M, Gibb R, Gorny G, Rowntree S. Age, experience and the changing brain. Neurosci biobehavioral Rev. (1998) 22:143–59. doi: 10.1016/s0149-7634(97)00008-0
112. Choi JM, Romeo RD, Brake WG, Bethea CL, Rosenwaks Z, McEwen BS. Estradiol increases pre- and post-synaptic proteins in the CA1 region of the hippocampus in female rhesus macaques (Macaca mulatta). Endocrinology. (2003) 144:4734–8. doi: 10.1210/en.2003-0216
113. Li C, Brake WG, Romeo RD, Dunlop JC, Gordon M, Buzescu R, et al. Estrogen alters hippocampal dendritic spine shape and enhances synaptic protein immunoreactivity and spatial memory in female mice. Proc Natl Acad Sci United States America. (2004) 101:2185–90. doi: 10.1073/pnas.0307313101
114. Prange-Kiel J, Rune GM. Direct and indirect effects of estrogen on rat hippocampus. Neuroscience. (2006) 138:765–72. doi: 10.1016/j.neuroscience.2005.05.061
115. Meethal SV, Liu T, Chan HW, Ginsburg E, Wilson AC, Gray DN, et al. Identification of a regulatory loop for the synthesis of neurosteroids: a steroidogenic acute regulatory protein-dependent mechanism involving hypothalamic-pituitary-gonadal axis receptors. J Neurochem. (2009) 110:1014–27. doi: 10.1111/j.1471-4159.2009.06192.x
116. Kandasamy M, Radhakrishnan RK, Poornimai Abirami GP, Roshan SA, Yesudhas A, Balamuthu K, et al. Possible existence of the hypothalamic-pituitary-hippocampal (HPH) axis: A reciprocal relationship between hippocampal specific neuroestradiol synthesis and neuroblastosis in ageing brains with special reference to menopause and neurocognitive disorders. Neurochem Res. (2019) 44:1781–95. doi: 10.1007/s11064-019-02833-1
Keywords: hippocampus, NORT, ovary, puberty, follicular growth, steroid hormones, gonadotropins
Citation: Castillo EdM, Quiróz U, Milflores L, Reyes R and Flores G (2025) Lesion of the ventral or dorsal hippocampus in the rat delays puberty, follicular growth and secretion of sex steroid hormones. Front. Endocrinol. 16:1530692. doi: 10.3389/fendo.2025.1530692
Received: 19 November 2024; Accepted: 31 March 2025;
Published: 16 April 2025.
Edited by:
Hernan E. Lara, University of Chile, ChileReviewed by:
Gonzalo Cruz, Universidad de Valparaiso, ChileCopyright © 2025 Castillo, Quiróz, Milflores, Reyes and Flores. This is an open-access article distributed under the terms of the Creative Commons Attribution License (CC BY). The use, distribution or reproduction in other forums is permitted, provided the original author(s) and the copyright owner(s) are credited and that the original publication in this journal is cited, in accordance with accepted academic practice. No use, distribution or reproduction is permitted which does not comply with these terms.
*Correspondence: Ubaldo Quiróz, dXF1aXJvekB5YWhvby5jb20ubXg=
†These authors have contributed equally to this work and share first authorship
Disclaimer: All claims expressed in this article are solely those of the authors and do not necessarily represent those of their affiliated organizations, or those of the publisher, the editors and the reviewers. Any product that may be evaluated in this article or claim that may be made by its manufacturer is not guaranteed or endorsed by the publisher.
Research integrity at Frontiers
Learn more about the work of our research integrity team to safeguard the quality of each article we publish.