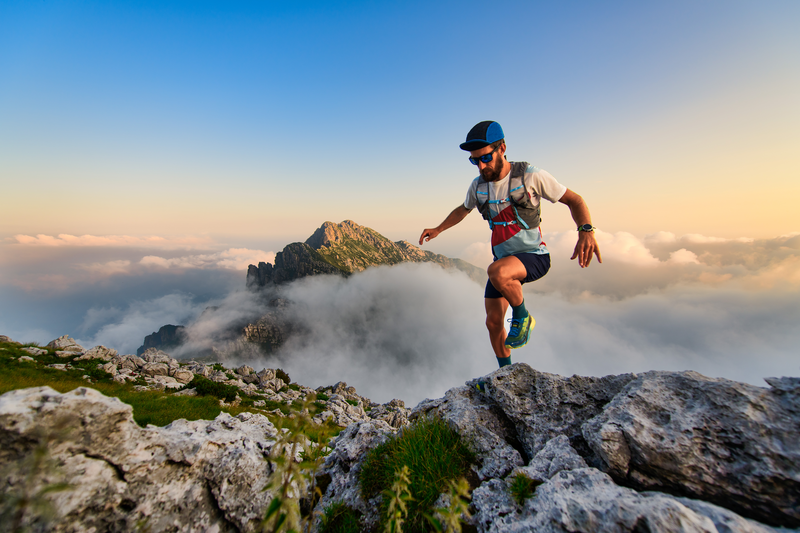
94% of researchers rate our articles as excellent or good
Learn more about the work of our research integrity team to safeguard the quality of each article we publish.
Find out more
REVIEW article
Front. Endocrinol.
Sec. Gut Endocrinology
Volume 16 - 2025 | doi: 10.3389/fendo.2025.1529703
The final, formatted version of the article will be published soon.
You have multiple emails registered with Frontiers:
Please enter your email address:
If you already have an account, please login
You don't have a Frontiers account ? You can register here
Polycystic ovary syndrome (PCOS) is a common endocrine and metabolic disorder affecting reproductive-age women, characterized primarily by hyperandrogenism, ovulatory dysfunction, and metabolic abnormalities. In recent years, the gut microbiota has garnered widespread attention for its potential role as a key regulator of host metabolism in the pathogenesis of PCOS. Studies have shown that PCOS patients exhibit dysbiosis in their gut microbiota, characterized by reduced microbial diversity, an imbalance in the ratio of Firmicutes to Bacteroidetes, changes in the abundance of specific taxa, and abnormal levels of metabolic products. These alterations may exacerbate metabolic dysfunction in PCOS through multiple mechanisms, including influencing host energy metabolism, disrupting lipid and bile acid metabolism, and inducing chronic inflammation. Addressing gut dysbiosis through the modulation of patients' microbiomes-such the use of, prebiotics, fecal microbiota transplantation, and optimizing diet lifestyle-may offer strategies for improving metabolic abnormalities and alleviating clinical symptoms in PCOS. Additionally, the gut microbiome promises as a potential marker, aiding in the precise diagnosis and personalized personalization of PCOS. Although our current understanding of by whichhow the gut microbiota influences PCOS is still limited, research is needed to explore the causal relationships and mechanisms involved, providing a more reliable theoretical basis for clinical. This review aims summarize the research progress on the relationship between gut microbiota and PCOS, and to suggest future directions to promote the development of prevention and treatment strategies for PCOS.
Keywords: Polycystic Ovary Syndrome, Gut Microbiota, Insulin Resistance, metabolic disorders, fecal microbiota transplantation
Received: 17 Nov 2024; Accepted: 27 Feb 2025.
Copyright: © 2025 Li, cheng and zhang. This is an open-access article distributed under the terms of the Creative Commons Attribution License (CC BY). The use, distribution or reproduction in other forums is permitted, provided the original author(s) or licensor are credited and that the original publication in this journal is cited, in accordance with accepted academic practice. No use, distribution or reproduction is permitted which does not comply with these terms.
* Correspondence:
tao zhang, Department of Stem Cells and Regenerative Medicine, Shenyang Key Laboratory of Stem Cell and Regenerative Medicine, China Medical University, shen yang, China
Disclaimer: All claims expressed in this article are solely those of the authors and do not necessarily represent those of their affiliated organizations, or those of the publisher, the editors and the reviewers. Any product that may be evaluated in this article or claim that may be made by its manufacturer is not guaranteed or endorsed by the publisher.
Research integrity at Frontiers
Learn more about the work of our research integrity team to safeguard the quality of each article we publish.