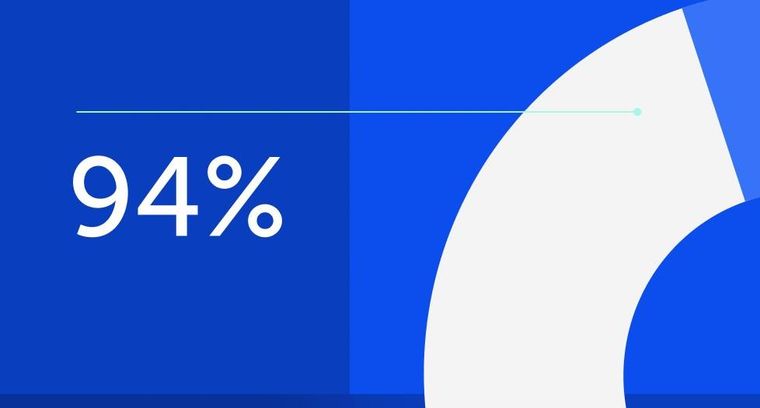
94% of researchers rate our articles as excellent or good
Learn more about the work of our research integrity team to safeguard the quality of each article we publish.
Find out more
ORIGINAL RESEARCH article
Front. Endocrinol., 07 April 2025
Sec. Cardiovascular Endocrinology
Volume 16 - 2025 | https://doi.org/10.3389/fendo.2025.1521415
Objective: Klotho is an anti-aging gene, and the α-klotho protein it encodes reportedly has cardiovascular protective effects. The atherogenic index of plasma (AIP) is a novel and comprehensive lipid index that correlates with atherosclerotic burden and is a critical risk factor for cardiovascular diseases. There are no studies examining the relationship between AIP and α-klotho; thus, we aimed to explore this potential association.
Methods: Data were extracted from the National Health and Nutrition Examination Survey 2007–2016 database, and the relationship between AIP and serum α-klotho levels was analyzed using weighted multivariate linear regression.
Results: After adjusting for risk factors, AIP showed a significant negative correlation with the logarithm of serum α-klotho levels in women. The trend analysis and smoothed curve fitting showed a nonlinear dose–response relationship. Threshold effect analysis showed a significant difference in the association between AIP and α-klotho before and after the AIP break point (0.434). Subgroup analyses demonstrated that the negative association of AIP with α-klotho was consistent across subgroups. However, the correlation between AIP and serum α-klotho in men was not significant.
Discussion: Our study provides new evidence for sex differences in the association between AIP and serum α-klotho levels.
Since Kuro et al. discovered the klotho gene in 1997 during a study on spontaneously hypertensive mice, numerous studies have investigated its relationship with health (1–3). As an anti-aging gene, klotho is strongly expressed in the epithelial cells of the renal distal tubules and the brain’s choroid plexus (4). It encodes a 135-kDa transmembrane protein, known as the klotho protein, also referred to as the α-klotho protein, which consists of 1,012 amino acids (5). The α-klotho protein exists in two molecular forms: membrane-bound and secreted. The membrane-bound form primarily promotes phosphate excretion in the kidneys and maintains calcium-phosphorus balance. In contrast, the secreted form acts as a hormone-like cytokine, participating in processes such as anti-inflammation, antioxidation, anti-apoptosis, anti-fibrosis, anti-tumor activity, ion channel regulation, and insulin resistance (1, 6, 7). Studies in both mice and humans have consistently demonstrated that low α-klotho expression correlates with premature aging phenotypes, including osteoporosis, atherosclerosis, cognitive deficits, and immune deficiencies. Conversely, overexpressing α-klotho can reverse the progression of these aging-related conditions and prolong lifespan (8–11). Therefore, understanding the factors associated with α-klotho protein levels is critical for health monitoring and prognostic assessment.
The atherogenic index of plasma (AIP) is a newly discovered biomarker associated with lipid metabolism (12). It reflects the balance between triglyceride (TG) and high-density lipoprotein cholesterol (HDL-C) levels in the blood and serves as a sensitive biomarker for atherosclerosis. Studies have shown that AIP is significantly correlated with cardiovascular events and can effectively predict cardiovascular disease risk (13, 14). Therefore, AIP not only holds high diagnostic value in clinical practice but also provides a useful reference for early prevention and intervention. Previously, low-density lipoprotein cholesterol (LDL-C) was considered the primary therapeutic target for dyslipidemia; however, some studies have shown that even when target LDL-C levels are achieved, patients still face a high residual cardiovascular risk (15, 16). Further studies found that small dense LDL-C (sdLDL-C) poses a more significant atherogenic risk than LDL-C (17). However, the complexity and high cost of sdLDL-C testing have limited its clinical application. Recently, it has been demonstrated that AIP can indirectly reflect LDL-C particle size, quantify the degree of lipid metabolism abnormalities, and outperform traditional lipid indices (18, 19). Therefore, studying AIP appears more relevant than focusing on LDL-C. Given the importance of AIP as a marker of atherosclerotic risk and the potential role of α-klotho in lipid metabolism and cardiovascular health, there may be an association between AIP and α-klotho levels. Studying this relationship could have significant clinical implications. In clinical practice, directly measuring plasma α-klotho levels require specialized equipment and techniques, which are complex and costly. In contrast, AIP is a routine, simple, and low-cost test that can be performed through conventional blood testing. If α-klotho levels could be estimated using AIP, it would provide a more convenient and economical screening method, particularly in resource-limited settings. To our knowledge, no studies have investigated the relationship between AIP and α-klotho. Therefore, we aimed to examine this relationship in middle-aged and older US populations using data from the National Health and Nutrition Examination Survey (NHANES) 2007–2016.
NHANES is a cross-sectional study that focuses on the health and nutritional status of the US population. It employs a complex multistage probability sampling method that includes face-to-face interviews, physical exams, and laboratory tests for data collection. The survey received ethical approval from the Ethics Review Board of the National Center for Health Statistics, and all participants provided written informed consent. Additional information is available on the NHANES website (www.cdc.gov/nchs/nhanes.htm).
This study included 50,588 participants from a nationally representative sample of NHANES from 2007 to 2016. We excluded 33,199 participants aged <40 or >79 years. Among the participants, 9,657 had missing AIP data, and 1,083 had missing α-klotho data. Ultimately, this study included 6,649 participants comprising 3,458 women and 3,191 men. Figure 1 shows the detailed screening process.
A professional technician collected participants’ fasting TG and HDL-C levels. AIP was calculated using the formula:
TG and HDL-C are expressed in mmol/L in the formula.
Due to the lack of α-klotho data for individuals younger than 40 and older than 79, only participants aged 40–79 were included in this study. Notably, all original serum α-klotho samples were frozen, stored at -80°C, and sent to the University of Washington for analysis between 2019 and 2020. α-Klotho concentrations were determined using a commercially available enzyme-linked immunosorbent assay kit manufactured by IBL (Fujioka, Japan). Each sample was analyzed twice, and the average values were calculated. Comprehensive details regarding the laboratory methods and quality assurance protocols are available on the official NHANES website.
On the basis of previous studies, we identified potential confounders for the relationship between AIP and serum α-klotho (20). These included age, race, education level, marital status, annual household income, physical activity, smoking behavior, drinking behavior, hypertension, diabetes, coronary heart disease, liver disease, kidney failure, and systemic immune-inflammatory index (SII). SII was calculated as follows:
Following NHANES recommendations, we categorized race as Mexican American, Other Hispanic, non-Hispanic White, non-Hispanic Black, and other. Educational level was categorized as “less than high school,” “completed high school,” and “more than high school.” Marital status was categorized as married/cohabiting or living alone. Annual household income was categorized as <$20,000 or ≥$20,000. Physical activity was categorized as inactive, moderate, vigorous, both moderate and vigorous. Smoking behavior was characterized by having smoked at least 100 cigarettes during one’s lifetime. Drinking behavior was assessed through two 24-hour dietary recalls, and participants were classified as drinkers if they reported consuming alcohol during at least one of the recalls. Diabetes was determined by meeting at least one of the following criteria: glycated hemoglobin level ≥ 6.5%, fasting blood glucose ≥ 7.0 mmol/L, self-reported physician diagnosis of diabetes, or self-reported use of insulin or glucose-lowering medications.
Given the complex multistage probability sampling design of NHANES data, this study applied weighting using the sample weight calculation method recommended by NHANES, specifically the “WTSAF2YR” or “WTMEC2YR” weights variable, to enhance the reliability of the findings. Our objective was to explore sex differences in the relationship between AIP and α-klotho. Therefore, we compared baseline characteristics and performed multiple linear regressions separately for men and women. Furthermore, to address the severely skewed distribution of α-klotho data and reduce variability between variables, we log-transformed the a-klotho levels and expressed them as Ln(α-klotho) for analysis. In the baseline data, numerical variables with a normal or approximately normal distribution were presented as mean ± standard deviation; while those with a skewed distribution were reported as median (interquartile range). Categorical variables were expressed as frequency (percentage). Baseline characteristic comparisons based on AIP quartiles were conducted using a one-way analysis of variance or the Kruskal–Wallis test for continuous variables, and the chi-square test was used for categorical variables. Weighted multiple linear regression was used to test for independent associations between AIP and Ln(α-klotho) levels across three models. AIP was analyzed as both a continuous and categorical variable (quartiles). Model 1 did not adjust for any covariates. Model 2 adjusted for age, race, education level, marital status, and annual household income. Model 3 included adjustments for all covariates. In addition, to explore the potential nonlinear relationship between AIP and Ln(α-klotho) levels, smooth curve fitting and threshold effect analysis were conducted using EmpowerRCH software to determine the optimal break point. Two-piecewise regression models were applied to examine the relationship between AIP and Ln(α-klotho) levels on either side of the break point. Furthermore, subgroup analyses were performed to test the effects of different subgroups on the results and to explore potential heterogeneity among these subgroups.
The study included 6,649 participants (3,458 women and 3,191 men). Baseline characteristics of the study population, stratified by sex, are presented in Table 1. Given the close association between α-klotho and aging, we further stratified α-klotho levels by age groups. The results showed that α-klotho levels were 805.03 (664.03, 1002.90) pg/ml in the 40–59 age group and 786.73 (645.62, 959.74) pg/ml in the 60–79 age group, with a statistically significant difference (p = 0.001). The baseline characteristics of male and female participants, classified according to AIP quartiles (less than −0.25, −0.25 to −0.03, −0.03 to 0.19, and more than 0.19), are summarized in Tables 2, 3, respectively. Among female participants, there were significant differences in age, race, education level, annual household income, hypertension, diabetes, liver disease, kidney failure, physical activity, and smoking and drinking behaviors (p < 0.05). SII increased significantly with increasing AIP (p < 0.001), while Ln(α-klotho) decreased significantly with increasing AIP (p < 0.001). Similarly, among male participants, significant differences were observed in age, race, hypertension, diabetes, and smoking and drinking behaviors (p < 0.05). However, no significant differences were observed for SII and Ln(α-klotho) (p > 0.05).
Weighted multivariate linear regression were used to evaluate the correlation between AIP and Ln(α-klotho) in middle-aged and older women and men, respectively. In the women’s group, Models 1, 2, and 3 showed that AIP was significantly negatively correlated with Ln(α-klotho) (Model 1: β = -0.146, 95% confidence interval [CI] = -0.210, -0.082; Model 2: β = -0.127, 95% CI = -0.194, -0.060; Model 3: β = -0.129, 95% CI = -0.209, -0.048); the higher the AIP, the lower the Ln(α-klotho). After converting the continuous variables to categorical variables, in the Models 1 (Q3: β = -0.090, 95% CI = -0.138, -0.042; Q4: β = -0.133, 95% CI = -0.179, -0.087), 2 (Q3: β = -0.072, 95% CI = -0.123, -0.021; Q4: β = -0.118, 95% CI = -0.167, -0.070), and 3 (Q3: β = -0.071, 95% CI = -0.126, -0.016; Q4: β = -0.120, 95% CI = -0.178, -0.063) results, Ln(α-klotho) was significantly lower in participants with high AIP than in those with low AIP (Q1). The trend analysis was significant, and the adjusted trend was unchanged (p < 0.001) (Table 4). In the men’s group, the relationship between AIP and Ln(α-klotho) was not significant (p > 0.05) in any of the three models, irrespective of whether AIP was used as a continuous or categorical variable (Table 5).
Smooth curve fitting and threshold effect analysis were used to explore the relationship between AIP and Ln(α-klotho) in female participants. The findings revealed a negative nonlinear relationship (Figure 2). Threshold effect analysis showed a significant difference in the association of AIP with α-klotho before and after the AIP break point of 0.434. At this break point, the value of Ln(α-klotho) was 6.618. Before the break point, there was a significant effect of AIP on Ln(α-klotho) (p < 0.001). However, after the break point, the relationship between AIP and Ln(α-klotho) was not significant (p = 0.051) (Table 6).
Figure 2. Smooth curve fitting for AIP and serum Ln(α-klotho) in woman. Adjusted for age, race, education level, marital status, annual household income, diabetes, hypertension, CHD, liver disease, kidney failure, physical activity, smoking behavior, drinking behavior, and SII. AIP, atherogenic index of plasma; CHD, coronary heart disease; SII, systemic immune-inflammatory index.
Furthermore, to assess the consistency of the relationship between AIP and Ln(α-klotho) in different subgroups, we performed subgroup analyses and interaction tests in female participants. The findings indicated that AIP interacted with diabetes, smoking behavior, and drinking behavior (p for interaction < 0.05); however, the significant negative correlation between AIP and Ln(α-klotho) remained unchanged across subgroups (Figure 3). This indicates that the association between AIP and Ln(α-klotho) was consistent across various subgroups, suggesting our results’ reliability.
Figure 3. Subgroups analyses of the effect of AIP on Ln(α-klotho) in woman. AIP, atherogenic index of plasma.
Overall, this study included 6,649 participants, which comprised 3,458 women and 3,191 men. Univariate analysis showed that in female participants, Ln(α-klotho) decreased significantly with increasing AIP. However, in male participants, there was no significant difference in Ln(α-klotho) at different AIP levels. Furthermore, after adjusting for other covariates, weighted linear regression analyses of the three models consistently showed a negative correlation between AIP and Ln(α-klotho) in female participants, whereas the correlation was not significant in male participants. Subsequent smooth curve fitting analyses confirmed the negative nonlinear relationship between AIP and Ln(α-klotho) in female participants. However, threshold effect analyses confirmed the differential effect of AIP before and after the break point. Therefore, the results indicate a sex difference in the negative correlation between AIP and Ln(α-klotho); this negative correlation is significant in female participants, supporting its potential as a novel biomarker for evaluating serum α-klotho levels in women.
Current research has confirmed a decline in blood α-klotho levels with increasing age in healthy populations, with an even more pronounced reduction observed in individuals with kidney and endocrine/metabolic disorders (21). Moreover, age-related α-klotho deficiency is associated with medial calcification, intimal hyperplasia, endothelial dysfunction, arterial stiffness, hypertension, impaired angiogenesis, and vascular remodeling—hallmarks of early vascular aging (22). AIP serves as a crucial surrogate metabolic biomarker for atherosclerosis and cardiovascular disease (23). Currently, there is no direct evidence linking AIP to serum α-klotho; however, indirect evidence suggests such a connection. The two main components of AIP, TG and HDL-C, are involved in lipid metabolism. Previous studies have confirmed a potential link between lipid metabolism and aging. For instance, the aging process is associated with increased plasma lipoproteins and decreased plasma TG clearance due to decreased lipoprotein lipase activity (24). Furthermore, mean α-klotho levels were significantly lower in participants with elevated TG than in those with normal TG levels (25). In addition, klotho supplementation has been shown to reduce lipid accumulation and improved dyslipidemia in older adults (26). One study found that hypertriglyceridemia was independently associated with lower serum α-klotho levels; and this association was particularly pronounced in women (27). This finding aligns with our findings, where we observed a significant negative correlation between AIP, a novel indicator of lipid metabolism, and α-klotho levels, with this association present only in female participants.
Therefore, based on our findings, we suggest that the following mechanisms may mediate the association between AIP and serum α-klotho. First, α-klotho can inhibit the inflammatory response, potentially through the inhibition of nuclear factor kappa B and Wnt activity (28, 29). Liu et al. demonstrated in an in vitro model of chronic kidney disease that α-klotho reduces cellular inflammatory responses and improves cellular lipid metabolism (30). In addition, serum α-klotho can inhibit insulin signaling through the insulin-like growth factor receptor 1→ phosphatidylinositol 3 kinase (PI3K) → protein kinase B (Akt) → mammalian target of rapamycin complex 1 → peroxisome proliferator-activated receptor α pathway. This mechanism regulates energy metabolism in the liver and adipose tissue, promoting lipid oxidation and reducing lipogenesis (31). Finally, abnormal lipid metabolism can disrupt endoplasmic reticulum homeostasis, leading to the generation of large amounts of reactive oxygen species (32). However, α-klotho can mitigate oxidative stress induced by abnormal lipid metabolism by activating the PI3K/Akt/endothelial nitric oxide synthase pathway, downregulating lectin-like oxidized LDL receptor 1 expression, and upregulating oxidative scavengers (superoxide dismutase and nitric oxide) (33). Overall, the role of α-klotho in lipid metabolism appears complex and warrants further investigation.
Currently, the mechanisms underlying sex differences in the relationship between AIP and α-klotho remain unclear. One possible explanation is that sex hormones play distinct roles in regulating lipid dynamics, leading to sex differences in lipid profiles (34), These differences may contribute to the observed sex-specific association between AIP and α-klotho. In addition, the androgen receptor (AR) in the renal distal tubule is functionally associated with α-klotho. Androgens may bind to the AR, potentially influencing klotho gene expression (35), thereby contributing to differences in α-klotho levels between men and women. However, the exact role of this interaction in health outcomes is complex and requires further investigation. Since AIP can serve as an indicator for pharmacological interventions, further studies on the potential association between AIP and α-klotho levels, as well as sex differences in this association, are necessary.This is the first study to examine the relationship between AIP and α-klotho, revealing that higher AIP is associated with lower serum α-klotho levels. In addition to its originality, a significant strength of our study is the large sample size from NHANES and the reliability of its data sources, which minimizes bias.
Despite our promising results, this study has some limitations. First, due to its cross-sectional design, we could not establish a causal relationship between AIP and serum α-klotho protein levels. Therefore, further prospective cohort studies are necessary to investigate this causality in the future. Second, our study was based on a non-institutional US population aged 40–79, limiting the generalizability of our findings to other populations. Additional studies in diverse populations are needed to confirm these findings. Thirdly, although we controlled for several confounders, we could not account for all potential confounding factors. Some of these factors may act as mediators rather than true confounders in the observed associations. Therefore, our results should be interpreted with caution. Finally, while AIP shows promise as a biomarker, our study does not fully justify its use over LDL-C. Residual effects after LDL-C treatment may arise from genetic variability and apolipoprotein differences, which contribute to population heterogeneity. AIP alone cannot address this variability, highlighting the need for further research to compare the advantages and limitations of AIP and LDL-C in different populations.
Our study provides new evidence for sex differences in the association between AIP and serum α-klotho levels. AIP and serum α-klotho levels were negatively correlated in female participants but not significantly correlated in male participants. Further prospective studies in diverse populations are necessary to validate our findings and assess causality more thoroughly.
Publicly available datasets were analyzed in this study. This data can be found here: www.cdc.gov/nchs/nhanes.htm.
The studies involving humans were approved by Ethics Review Board of the National Center for Health Statistics. The studies were conducted in accordance with the local legislation and institutional requirements. The participants provided their written informed consent to participate in this study.
CZ: Conceptualization, Data curation, Formal Analysis, Methodology, Visualization, Writing – original draft, Writing – review & editing. ZS: Data curation, Formal Analysis, Writing – review & editing. YL: Conceptualization, Supervision, Writing – review & editing.
The author(s) declare that no financial support was received for the research and/or publication of this article.
We express our gratitude to the CDC/NCHS for supplying NHANES data from 2007 to 2016. We would also like to thank the director of the Department of Cardiology of the Central Hospital of Baotou.
The authors declare that the research was conducted in the absence of any commercial or financial relationships that could be construed as a potential conflict of interest.
The author(s) declare that no Generative AI was used in the creation of this manuscript.
All claims expressed in this article are solely those of the authors and do not necessarily represent those of their affiliated organizations, or those of the publisher, the editors and the reviewers. Any product that may be evaluated in this article, or claim that may be made by its manufacturer, is not guaranteed or endorsed by the publisher.
1. Kuro-O M. The Klotho proteins in health and disease. Nat Rev Nephrol. (2019) 15:27–44. doi: 10.1038/s41581-018-0078-3
2. Xu Y, Sun Z. Molecular basis of Klotho: from gene to function in aging. Endocr Rev. (2015) 36:174–93. doi: 10.1210/er.2013-1079
3. Chen K, Wang S, Sun QW, Zhang B, Ullah M, Sun Z. Klotho deficiency causes heart aging via impairing the Nrf2-GR pathway. Circ Res. (2021) 128:492–507. doi: 10.1161/CIRCRESAHA.120.317348
4. Kato Y, Arakawa E, Kinoshita S, Shirai A, Furuya A, Yamano K, et al. Establishment of the anti-Klotho monoclonal antibodies and detection of Klotho protein in kidneys. Biochem Biophys Res Commun. (2000) 267:597–602. doi: 10.1006/bbrc.1999.2009
5. Razzaque MS. The FGF23-Klotho axis: endocrine regulation of phosphate homeostasis. Nat Rev Endocrinol. (2009) 5:611–9. doi: 10.1038/nrendo.2009.196
6. Yamamoto M, Clark JD, Pastor JV, Gurnani P, Nandi A, Kurosu H, et al. Regulation of oxidative stress by the anti-aging hormone klotho. J Biol Chem. (2005) 280:38029–34. doi: 10.1074/jbc.M509039200
7. Yuan Q, Ren Q, Li L, Tan H, Lu M, Tian Y, et al. A Klotho-derived peptide protects against kidney fibrosis by targeting TGF-β signaling. Nat Commun. (2022) 13:438. doi: 10.1038/s41467-022-28096-z
8. Kuro-o M, Matsumura Y, Aizawa H, Kawaguchi H, Suga T, Utsugi T, et al. Mutation of the mouse klotho gene leads to a syndrome resembling ageing. Nature. (1997) 390:45–51. doi: 10.1038/36285
9. Chuang MH, Wang HW, Huang YT, Jiang MY. Association between soluble α-klotho and mortality risk in middle-aged and older adults. Front Endocrinol (Lausanne). (2023) 14:1246590. doi: 10.3389/fendo.2023.1246590
10. Wu Y, Lei S, Li D, Li Z, Zhang Y, Guo Y. Relationship of Klotho with cognition and dementia: Results from the NHANES 2011-2014 and Mendelian randomization study. Transl Psychiatry. (2023) 13:337. doi: 10.1038/s41398-023-02632-x
11. Jiang J, Liu Q, Mao Y, Wang N, Lin W, Li L, et al. Klotho reduces the risk of osteoporosis in postmenopausal women: a cross-sectional study of the National Health and Nutrition Examination Survey (NHANES). BMC Endocr Disord. (2023) 23:151. doi: 10.1186/s12902-023-01380-9
12. Alifu J, Xiang L, Zhang W, Qi P, Chen H, Liu L, et al. Association between the atherogenic index of plasma and adverse long-term prognosis in patients diagnosed with chronic coronary syndrome. Cardiovasc Diabetol. (2023) 22:255. doi: 10.1186/s12933-023-01989-z
13. Fernández-Macías JC, Ochoa-Martínez AC, Varela-Silva JA, Pérez-Maldonado IN. Atherogenic index of plasma: novel predictive biomarker for cardiovascular illnesses. Arch Med Res. (2019) 50:285–94. doi: 10.1016/j.arcmed.2019.08.009
14. Lioy B, Webb RJ, Amirabdollahian F. The association between the atherogenic index of plasma and cardiometabolic risk factors: a review. Healthcare (Basel). (2023) 11:966. doi: 10.3390/healthcare11070966
15. Sekimoto T, Koba S, Mori H, Sakai R, Arai T, Yokota Y, et al. Small dense low-density lipoprotein cholesterol: a residual risk for rapid progression of non-culprit coronary lesion in patients with acute coronary syndrome. J Atheroscler Thromb. (2021) 28:1161–74. doi: 10.5551/jat.60152
16. Hoogeveen RC, Ballantyne CM. Residual cardiovascular risk at low LDL: remnants, lipoprotein(a), and inflammation. Clin Chem. (2021) 67:143–53. doi: 10.1093/clinchem/hvaa252
17. Weintraub WS, Arbab-Zadeh A. Should we adjust low-density lipoprotein cholesterol management to the severity of coronary artery disease? JACC Cardiovasc Imaging. (2020) 13:1973–5. doi: 10.1016/j.jcmg.2020.04.007
18. Dobiásová M, Frohlich J. The plasma parameter log (TG/HDL-C) as an atherogenic index: correlation with lipoprotein particle size and esterification rate in apoB-lipoprotein-depleted plasma (FER(HDL)). Clin Biochem. (2001) 34:583–8. doi: 10.1016/s0009-9120(01)00263-6
19. Zhu X, Yu L, Zhou H, Ma Q, Zhou X, Lei T, et al. Atherogenic index of plasma is a novel and better biomarker associated with obesity: a population-based cross-sectional study in China. Lipids Health Dis. (2018) 17:37. doi: 10.1186/s12944-018-0686-8
20. Yan S, Luo W, Lei L, Zhang Q, Xiu J. Association between serum Klotho concentration and hyperlipidemia in adults: a cross-sectional study from NHANES 2007-2016. Front Endocrinol (Lausanne). (2023) 14:1280873. doi: 10.3389/fendo.2023.1280873
21. Abraham CR, Li A. Aging-suppressor Klotho: prospects in diagnostics and therapeutics. Ageing Res Rev. (2022) 82:101766. doi: 10.1016/j.arr.2022.101766
22. Iurciuc S, Cimpean AM, Mitu F, Heredea R, Iurciuc M. Vascular aging and subclinical atherosclerosis: why such a “never ending” and challenging story in cardiology? Clin Interv Aging. (2017) 12:1339–45. doi: 10.2147/CIA.S141265
23. Huang Q, Liu Z, Wei M, Huang Q, Feng J, Liu Z, et al. The atherogenic index of plasma and carotid atherosclerosis in a community population: a population-based cohort study in China. Cardiovasc Diabetol. (2023) 22:125. doi: 10.1186/s12933-023-01839-y
24. Spitler KM, Davies BSJ. Aging and plasma triglyceride metabolism. J Lipid Res. (2020) 61:1161–7. doi: 10.1194/jlr.R120000922
25. Orces CH. The association between metabolic syndrome and the anti-aging humoral factor klotho in middle-aged and older adults. Diabetes Metab Syndr. (2022) 16:102522. doi: 10.1016/j.dsx.2022.102522
26. Liu W, Chen X, Wu M, Li L, Liu J, Shi J, et al. Recombinant Klotho protein enhances cholesterol efflux of THP-1 macrophage-derived foam cells via suppressing Wnt/β-catenin signaling pathway. BMC Cardiovasc Disord. (2020) 20:120. doi: 10.1186/s12872-020-01400-9
27. Lee J, Kim D, Lee HJ, Choi JY, Min JY, Min KB. Association between serum klotho levels and cardiovascular disease risk factors in older adults. BMC Cardiovasc Disord. (2022) 22:442. doi: 10.1186/s12872-022-02885-2
28. Maekawa Y, Ishikawa K, Yasuda O, Oguro R, Hanasaki H, Kida I, et al. Klotho suppresses TNF-alpha-induced expression of adhesion molecules in the endothelium and attenuates NF-kappaB activation. Endocrine. (2009) 35:341–6. doi: 10.1007/s12020-009-9181-3
29. Zhou L, Li Y, Zhou D, Tan RJ, Liu Y. Loss of Klotho contributes to kidney injury by derepression of Wnt/β-catenin signaling. J Am Soc Nephrol. (2013) 24:771–85. doi: 10.1681/ASN.2012080865
30. Liu J, Wang H, Liu Q, Long S, Wu Y, Wang N, et al. Klotho exerts protection in chronic kidney disease associated with regulating inflammatory response and lipid metabolism. Cell Biosci. (2024) 14:46. doi: 10.1186/s13578-024-01226-4
31. Gu H, Jiang W, You N, Huang X, Li Y, Peng X, et al. Soluble klotho improves hepatic glucose and lipid homeostasis in type 2 diabetes. Mol Ther Methods Clin Dev. (2020) 18:811–23. doi: 10.1016/j.omtm.2020.08.002
32. Mo JS, Choi D, Han YR, Kim N, Jeong HS. Morin has protective potential against ER stress induced apoptosis in renal proximal tubular HK-2 cells. BioMed Pharmacother. (2019) 112:108659. doi: 10.1016/j.biopha.2019.108659
33. Yao Y, Wang Y, Zhang Y, Liu C. Klotho ameliorates oxidized low density lipoprotein (ox-LDL)-induced oxidative stress via regulating LOX-1 and PI3K/Akt/eNOS pathways. Lipids Health Dis. (2017) 16:77. doi: 10.1186/s12944-017-0447-0
34. Wang X, Magkos F, Mittendorfer B. Sex differences in lipid and lipoprotein metabolism: it’s not just about sex hormones. J Clin Endocrinol Metab. (2011) 96:885–93. doi: 10.1210/jc.2010-2061
Keywords: atherogenic index of plasma, klotho, sex differences, NHANES, lipid
Citation: Zuo C, Shen Z-D and Lu Y (2025) Sex differences between atherogenic index of plasma and α-klotho levels in middle-aged and older adults: NHANES 2007–2016. Front. Endocrinol. 16:1521415. doi: 10.3389/fendo.2025.1521415
Received: 01 November 2024; Accepted: 20 March 2025;
Published: 07 April 2025.
Edited by:
Vikrant Rai, Western University of Health Sciences, United StatesReviewed by:
Antonio Chiariello, University of Bologna, ItalyCopyright © 2025 Zuo, Shen and Lu. This is an open-access article distributed under the terms of the Creative Commons Attribution License (CC BY). The use, distribution or reproduction in other forums is permitted, provided the original author(s) and the copyright owner(s) are credited and that the original publication in this journal is cited, in accordance with accepted academic practice. No use, distribution or reproduction is permitted which does not comply with these terms.
*Correspondence: Yaojun Lu, THV5YW9qdW45OTVAMTI2LmNvbQ==
Disclaimer: All claims expressed in this article are solely those of the authors and do not necessarily represent those of their affiliated organizations, or those of the publisher, the editors and the reviewers. Any product that may be evaluated in this article or claim that may be made by its manufacturer is not guaranteed or endorsed by the publisher.
Research integrity at Frontiers
Learn more about the work of our research integrity team to safeguard the quality of each article we publish.