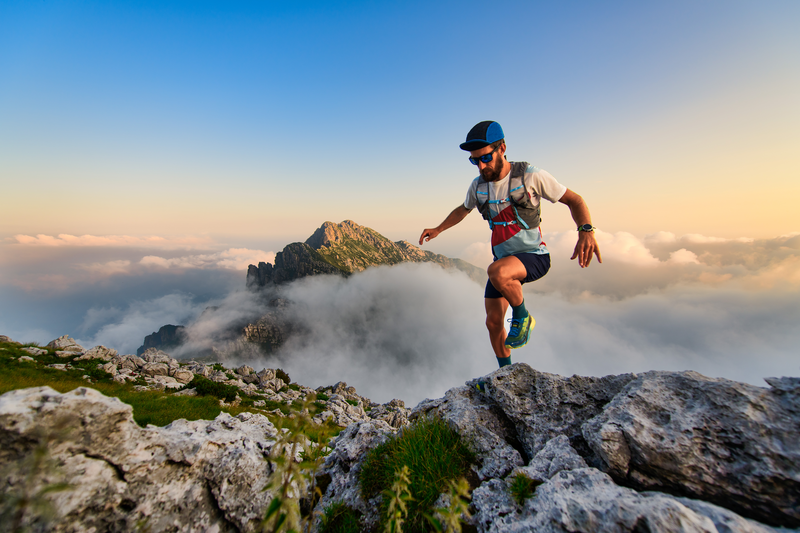
94% of researchers rate our articles as excellent or good
Learn more about the work of our research integrity team to safeguard the quality of each article we publish.
Find out more
ORIGINAL RESEARCH article
Front. Endocrinol.
Sec. Thyroid Endocrinology
Volume 16 - 2025 | doi: 10.3389/fendo.2025.1503144
The final, formatted version of the article will be published soon.
You have multiple emails registered with Frontiers:
Please enter your email address:
If you already have an account, please login
You don't have a Frontiers account ? You can register here
This study assesses the global burden of thyroid cancer (TC) among adolescents and young adults (AYA) aged 15-39 from 1990 to 2021, with projections to 2050. Using data from the Global Burden of Disease (GBD) 2021 database, we analysed the incidence, prevalence, mortality, and disability-adjusted life years (DALYs) across 204 countries. Thyroid cancer incidence has risen significantly, with new cases increasing from 19,268 in 1990 to 48,203 in 2021. Females continue to experience a higher disease burden than males, with an incidence rate of 2.38 per 100,000 in 2021, compared to 0.88 in males. Regional disparities also exist, with the highest burden observed in the Middle East and North Africa, where incidence rates reached 2.49 per 100,000 in 2021.The ARIMA models project that by 2050, the global prevalence of TC will reach 103.62 per million, with an incidence rate of 11.41 per 100,000. Mortality is expected to rise slightly, while the DALYs burden is projected to increase to 34.41 per million. Females, particularly in lower-SDI regions, are predicted to bear a disproportionate share of the disease burden. While high-SDI regions are forecasted to experience a decline in incidence and prevalence, lower-SDI regions will face increasing challenges due to limited healthcare infrastructure and delayed diagnoses.This study underscores the growing public health burden of thyroid cancer, particularly among AYA populations. Effective strategies for early diagnosis, improved treatment, and equitable resource distribution are critical to addressing the rising incidence, especially in vulnerable regions.
Keywords: thyroid cancer, AYA population, GBD, ARIMA prediction, burden of disease
Received: 28 Sep 2024; Accepted: 10 Mar 2025.
Copyright: © 2025 Jia, Wang, Liu and Li. This is an open-access article distributed under the terms of the Creative Commons Attribution License (CC BY). The use, distribution or reproduction in other forums is permitted, provided the original author(s) or licensor are credited and that the original publication in this journal is cited, in accordance with accepted academic practice. No use, distribution or reproduction is permitted which does not comply with these terms.
* Correspondence:
Shen Wang, School of Public Health, China Medical University, Shenyang, China
Huilin Li, Department of Endocrinology, Shenzhen Traditional Chinese Medicine Hospital, Shenzhen, 518033, China
Disclaimer: All claims expressed in this article are solely those of the authors and do not necessarily represent those of their affiliated organizations, or those of the publisher, the editors and the reviewers. Any product that may be evaluated in this article or claim that may be made by its manufacturer is not guaranteed or endorsed by the publisher.
Research integrity at Frontiers
Learn more about the work of our research integrity team to safeguard the quality of each article we publish.