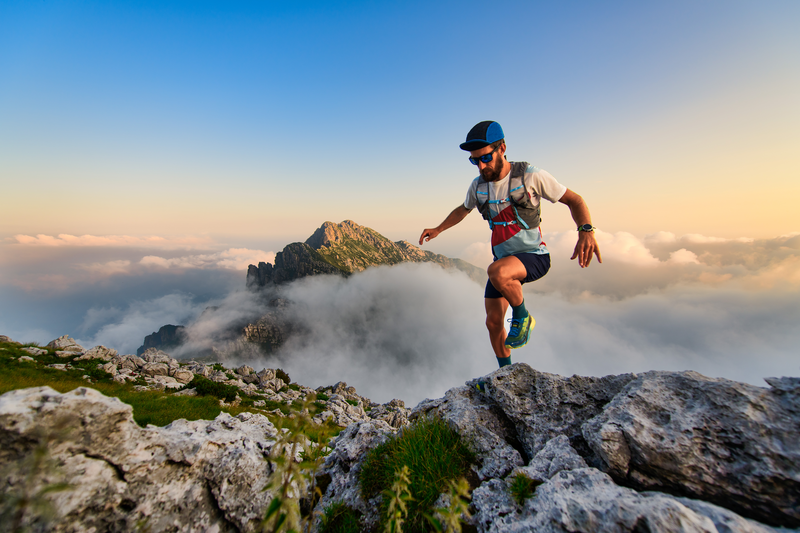
94% of researchers rate our articles as excellent or good
Learn more about the work of our research integrity team to safeguard the quality of each article we publish.
Find out more
ORIGINAL RESEARCH article
Front. Endocrinol.
Sec. Reproduction
Volume 16 - 2025 | doi: 10.3389/fendo.2025.1478124
The final, formatted version of the article will be published soon.
You have multiple emails registered with Frontiers:
Please enter your email address:
If you already have an account, please login
You don't have a Frontiers account ? You can register here
Background: Testosterone is a crucial hormone for male health, influencing metabolism, cardiovascular function, bone density, and cognitive abilities. Elevated non-HDL cholesterol to HDL cholesterol ratio (NHHR) has been implicated in lipid metabolism disorders, which may adversely affect testosterone levels. This study investigates the association between NHHR and testosterone levels in adult males, utilizing data from the National Health and Nutrition Examination Survey (NHANES).This cross-sectional study analyzed data from 2,859 adult males from the NHANES cycles 2011-2016. Total testosterone levels were measured using isotope dilution liquid chromatography-tandem mass spectrometry (ID-LC-MS/MS). NHHR was calculated and analyzed as both a continuous variable and in quartiles. Multivariable linear and logistic regression models, adjusted for demographic, biochemical, lifestyle factors, and medical comorbidities, were used to assess the relationship between NHHR and total testosterone levels and the risk of testosterone deficiency (TD).Results: Higher NHHR was significantly associated with lower total testosterone levels and increased risk of TD. In fully adjusted models, each unit increase in NHHR was associated with a decrease in total testosterone levels (β = -16.31, 95% CI: -26.58 to -6.04, P = 0.003) and an increased risk of TD (OR = 1.24, 95% CI: 1.07 to 1.44, P = 0.01). When NHHR was analyzed in quartiles, participants in the highest quartile (Q4) had significantly lower testosterone levels (β = -54.98, 95% CI: -86.21 to -23.74, P = 0.001) and a higher risk of TD (OR = 2.04, 95% CI: 1.20 to 3.49, P = 0.01) compared to those in the lowest quartile (Q1). Subgroup analyses confirmed these findings across different age groups, BMI categories, smoking status, and presence of comorbidities. Smooth curve fitting demonstrated a linear relationship among them.Our study is the first to identify a significant association between elevated NHHR and both reduced total testosterone levels and increased risk of TD in a large, representative sample of adult American males. These findings suggest that NHHR could serve as a valuable marker for early identification of individuals at risk for testosterone decline and TD, enabling timely and targeted clinical interventions.
Keywords: Testosterone, NHHR, Lipid Metabolism, Testosterone deficiency, NHANES
Received: 09 Aug 2024; Accepted: 28 Feb 2025.
Copyright: © 2025 Mei, Chen, Wang, Xu, Xu and Feng. This is an open-access article distributed under the terms of the Creative Commons Attribution License (CC BY). The use, distribution or reproduction in other forums is permitted, provided the original author(s) or licensor are credited and that the original publication in this journal is cited, in accordance with accepted academic practice. No use, distribution or reproduction is permitted which does not comply with these terms.
* Correspondence:
Xingliang Feng, The Third Affiliated Hospital of Soochow University, Changzhou, Jiangsu, China., Changzhou, China
Disclaimer: All claims expressed in this article are solely those of the authors and do not necessarily represent those of their affiliated organizations, or those of the publisher, the editors and the reviewers. Any product that may be evaluated in this article or claim that may be made by its manufacturer is not guaranteed or endorsed by the publisher.
Research integrity at Frontiers
Learn more about the work of our research integrity team to safeguard the quality of each article we publish.