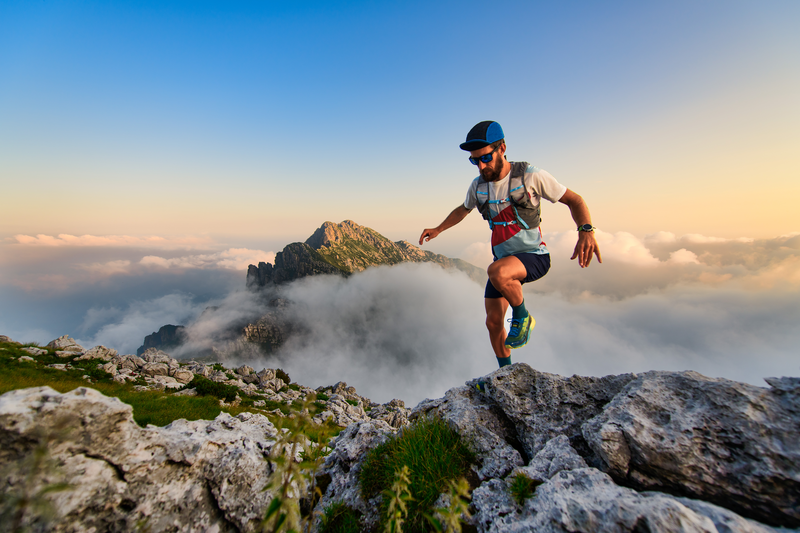
94% of researchers rate our articles as excellent or good
Learn more about the work of our research integrity team to safeguard the quality of each article we publish.
Find out more
ORIGINAL RESEARCH article
Front. Endocrinol.
Sec. Thyroid Endocrinology
Volume 16 - 2025 | doi: 10.3389/fendo.2025.1433534
The final, formatted version of the article will be published soon.
You have multiple emails registered with Frontiers:
Please enter your email address:
If you already have an account, please login
You don't have a Frontiers account ? You can register here
Pregnant women with subclinical hypothyroidism or clinical hypothyroidism often exhibit lipid metabolism disorders and are correlated with adverse pregnant outcomes. It was suggested that isolated positive thyroid peroxidase antibody (TPOAb) served as a risk factor for adverse outcomes. However, little was known about the lipid metabolism profile in pregnant women with isolated positive TPOAb.The purpose of this prospective observational study was to investigate lipid profile changes by using untargeted liquid chromatography-mass spectrometry (LC-MS) and to explore their associations with clinical parameters. A total of 90 pregnant women in the first trimester were enrolled in the analysis: 46 were TPOAb-positive euthyroid women, and 44 were healthy controls. A total of 1238 lipid molecules were identified. These molecules belonging to 46 lipid classes and 5 lipid categories (fatty acyl (FA), glycerophospholipid (GP), glycerolipid (GL), sphingolipid (SP), and sterol lipid (ST)). A total of 202 differential lipid molecules were screened between the two groups. KEGG pathway enrichment analysis revealed that the differentially expressed lipids participate in multiple pathways, such as Thermogenesis, Regulation of lipolysis, PD-L1 expression and PD-1 checkpoint pathway in cancer, Neurotrophin signaling pathway, Natural killer cell mediated cytotoxicity, NF-kappa B signaling pathway, Insulin resistance, Glycerophospholipid metabolism, Choline metabolism in cancer, Adipocytokine signaling pathway, Fat digestion and absorption, AGE-RAGE signaling pathway in diabetic complications, Diabetic cardiomyopathy, the Th1/Th2/Th17 cell differential pathway.
Keywords: Thyroid peroxidase antibody, Pregnancy, Lipids, Metabolism, Thyroid function
Received: 16 May 2024; Accepted: 04 Mar 2025.
Copyright: © 2025 Chen, Qin, Qingyao, Wu, Zang, Cong, Shen and Chen. This is an open-access article distributed under the terms of the Creative Commons Attribution License (CC BY). The use, distribution or reproduction in other forums is permitted, provided the original author(s) or licensor are credited and that the original publication in this journal is cited, in accordance with accepted academic practice. No use, distribution or reproduction is permitted which does not comply with these terms.
* Correspondence:
Lei Chen, Suzhou Municipal Hospital, Suzhou, China
Disclaimer: All claims expressed in this article are solely those of the authors and do not necessarily represent those of their affiliated organizations, or those of the publisher, the editors and the reviewers. Any product that may be evaluated in this article or claim that may be made by its manufacturer is not guaranteed or endorsed by the publisher.
Research integrity at Frontiers
Learn more about the work of our research integrity team to safeguard the quality of each article we publish.