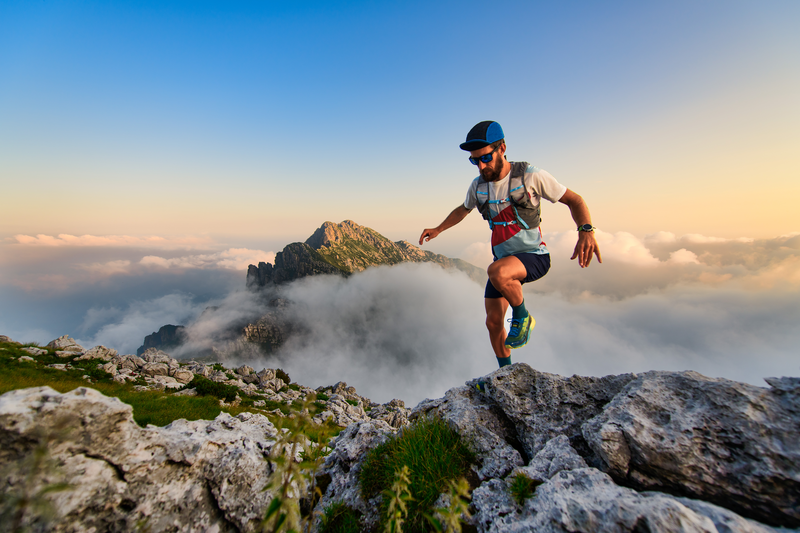
94% of researchers rate our articles as excellent or good
Learn more about the work of our research integrity team to safeguard the quality of each article we publish.
Find out more
ORIGINAL RESEARCH article
Front. Endocrinol.
Sec. Renal Endocrinology
Volume 16 - 2025 | doi: 10.3389/fendo.2025.1405003
This article is part of the Research Topic Endocrine Imbalances of Mineral Ions and Vitamins in Chronic Disease Pathogenesis View all 15 articles
The final, formatted version of the article will be published soon.
You have multiple emails registered with Frontiers:
Please enter your email address:
If you already have an account, please login
You don't have a Frontiers account ? You can register here
AbstractPurpose: The controversial nature of the association between α-Klotho and mortality risk in the general population warrants further investigation. This study aims to examine the correlation between circulating α-Klotho levels and the risk of all-cause mortality.Methods: A sample size of 13,748 individuals from the NAHNES 2005-2016 cycles was included in this study. The effect of different α-Klotho levels (divided into quartiles) on survival was assessed using Kaplan-Meier (KM) curves. Cox proportional hazards models were used to analyse the linear relationship between log α-Klotho and the risk of all-cause mortality. Restricted cubic spline Cox proportional hazards regression model was used to analyse the non-linear relationship between log α-Klotho and risk of all-cause mortality. Threshold effect analysis was performed to determine the most favourable inflection point for log α-Klotho. Stratification and sensitivity analyses were performed to assess the robustness of the results.Results: A total of 1,569 deaths were reported during the median follow-up period of 5.33 years (2.83-7.83 years). Among the log α-Klotho quartile groups, quartile 1 had the highest mortality rate compared to quartiles 2, 3, and 4. Multifactorial Cox regression analysis revealed a weak association between log α-Klotho and a 44% reduction in the risk of all-cause mortality (p=0.0473). We also found a U-shaped non-linear association between log α-Klotho and risk of all-cause mortality, with an optimal inflection point identified at 2.89 pg/mL. The stability of the U-shaped association between log α-Klotho and mortality risk was observed in various stratification and sensitivity analyses.Conclusion: This study identified a U-shaped association between circulating α-Klotho levels and risk of all-cause mortality, with a notable inflection point at 2.89 pg/mL. Further investigation is warranted to fully elucidate the potential mechanisms underlying the association between α-Klotho and risk of all-cause mortality in the broader population.
Keywords: α-klotho, All-cause mortality, NHANES, U-shaped relationship, Klotho
Received: 22 Mar 2024; Accepted: 21 Feb 2025.
Copyright: © 2025 Song, Li and Fang. This is an open-access article distributed under the terms of the Creative Commons Attribution License (CC BY). The use, distribution or reproduction in other forums is permitted, provided the original author(s) or licensor are credited and that the original publication in this journal is cited, in accordance with accepted academic practice. No use, distribution or reproduction is permitted which does not comply with these terms.
* Correspondence:
Jianling Song, Second Clinical Medical College, Nanchang University, Nanchang, China
Disclaimer: All claims expressed in this article are solely those of the authors and do not necessarily represent those of their affiliated organizations, or those of the publisher, the editors and the reviewers. Any product that may be evaluated in this article or claim that may be made by its manufacturer is not guaranteed or endorsed by the publisher.
Research integrity at Frontiers
Learn more about the work of our research integrity team to safeguard the quality of each article we publish.