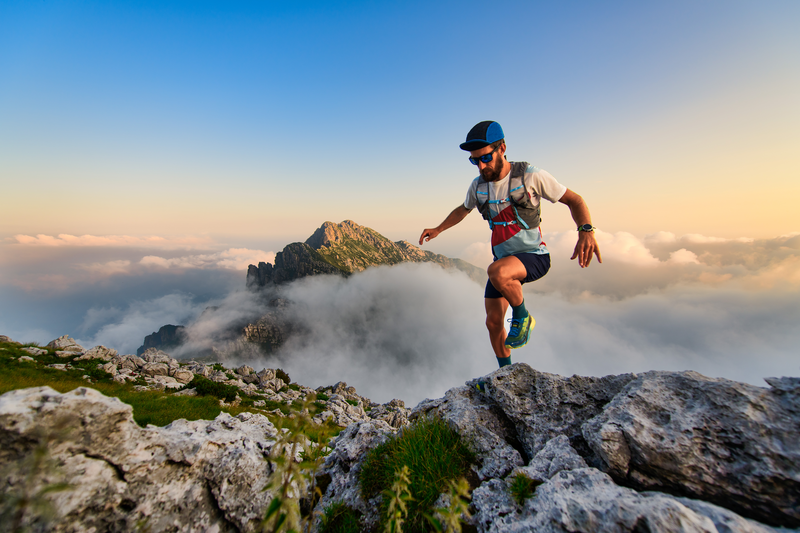
94% of researchers rate our articles as excellent or good
Learn more about the work of our research integrity team to safeguard the quality of each article we publish.
Find out more
REVIEW article
Front. Endocrinol.
Sec. Clinical Diabetes
Volume 16 - 2025 | doi: 10.3389/fendo.2025.1393883
This article is part of the Research Topic Women in Clinical Diabetes View all 15 articles
The final, formatted version of the article will be published soon.
You have multiple emails registered with Frontiers:
Please enter your email address:
If you already have an account, please login
You don't have a Frontiers account ? You can register here
Gestational diabetes mellitus (GDM) is a common pregnancy complication closely associated with maternal oxidative and antioxidant imbalance, known as oxidative stress. Environmental and dietary exposure plays an important role in inducing oxidative stress during pregnancy. This review aims to provide an in-depth analysis of the role of oxidative stress induced by environmental and dietary exposure in GDM while incorporating current research frontiers. Environmental pollution, smoking, excessive nutrition, and unhealthy eating habits such as a high-fat diet and vitamin deficiency, may contribute to the generation and accumulation of reactive oxygen species (ROS), leading to oxidative stress. Within the pathway of oxidative stress in GDM, the production and clearance mechanisms of ROS play a pivotal role. Relevant studies have demonstrated that ROS production is closely linked to insulin resistance, adipose tissue accumulation, inflammation, and other pathological processes.Antioxidant substances like vitamins C and E or glutathione can mitigate oxidative stress damage on pregnant women and fetuses by scavenging ROS. Currently, there remain several cutting-edge issues regarding the involvement of the oxidative stress pathway in GDM pathogenesis as well as its relationship with environmental and dietary factors, for instance: how to reduce maternal oxidative stress levels through dietary adjustments or lifestyle modifications; how antioxidant substances can be utilized for intervention treatment; and accurate assessment methods for maternal oxidative stress status along with its association with GDM risk. In conclusion, environmental and dietary factors exert significant influence on GDM pathogenesis while highlighting increasing attention toward understanding the role played by the oxidative stress pathway within this context. In-depth research endeavors within this field are anticipated to offer novel insights into prevention strategies as well as therapeutic approaches for GDM.
Keywords: gestational diabetes mellitus, Oxidative Stress, Reactive Oxygen Species, Treatment, Environmental Exposure, Dietary exposure
Received: 29 Feb 2024; Accepted: 27 Feb 2025.
Copyright: © 2025 Sun, Shen, Fang, Huang, Lai, Hu and Zheng. This is an open-access article distributed under the terms of the Creative Commons Attribution License (CC BY). The use, distribution or reproduction in other forums is permitted, provided the original author(s) or licensor are credited and that the original publication in this journal is cited, in accordance with accepted academic practice. No use, distribution or reproduction is permitted which does not comply with these terms.
* Correspondence:
Yanjun Hu, Wenzhou People’s Hospital, Wenzhou, 325000, Zhejiang Province, China
Jianqiong Zheng, Wenzhou People’s Hospital, Wenzhou, 325000, Zhejiang Province, China
Disclaimer: All claims expressed in this article are solely those of the authors and do not necessarily represent those of their affiliated organizations, or those of the publisher, the editors and the reviewers. Any product that may be evaluated in this article or claim that may be made by its manufacturer is not guaranteed or endorsed by the publisher.
Research integrity at Frontiers
Learn more about the work of our research integrity team to safeguard the quality of each article we publish.