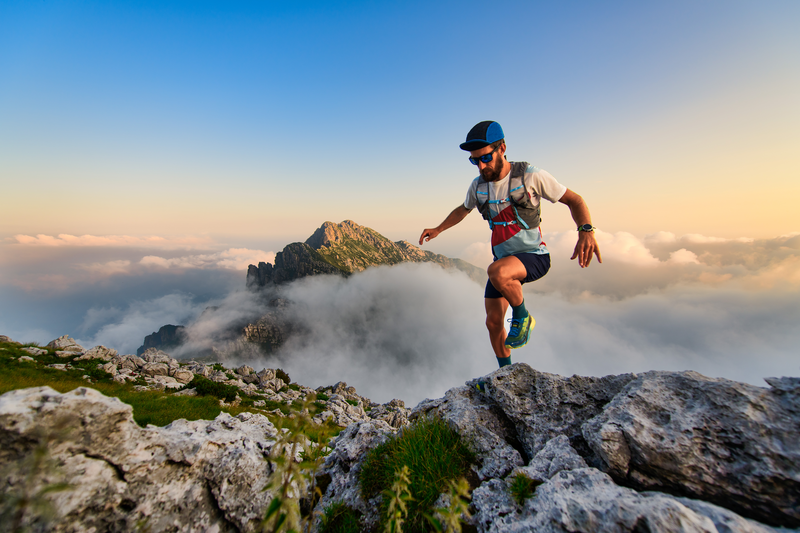
95% of researchers rate our articles as excellent or good
Learn more about the work of our research integrity team to safeguard the quality of each article we publish.
Find out more
REVIEW article
Front. Endocrinol. , 13 November 2024
Sec. Pediatric Endocrinology
Volume 15 - 2024 | https://doi.org/10.3389/fendo.2024.1505430
This article is part of the Research Topic Insights in Pediatric Endocrinology: 2024 View all 18 articles
Introduction: Type 1 diabetes (T1D) is a chronic condition marked by insulin deficiency and hyperglycemia, with an increasing global incidence, particularly among children. Despite improvements in diabetes management, individuals with T1D continue to experience higher rates of cardiovascular disease (CVD), the leading cause of mortality in this population. Traditional CVD risk factors such as dyslipidemia and poor glycemic control are insufficient to fully explain the elevated risk in T1D, prompting further investigation into additional factors. Emerging evidence suggests that metabolic dysfunction-associated steatotic liver disease (MASLD) plays a critical role in this heightened CVD risk.
Objective: This narrative review aims to explore the relationship between MASLD and CVD in individuals with T1D. The review focuses on the prevalence of MASLD, its contributing risk factors, and the potential impact of liver dysfunction on cardiovascular outcomes in this population.
Methods: A review of existing literature was conducted, focusing on observational studies, cohort studies, and meta-analyses that investigate the prevalence of MASLD in T1D populations and its association with CVD. The review also examines the physiological mechanisms linking MASLD and CVD, including insulin resistance, systemic inflammation, and hepatic dyslipidemia. Key studies were evaluated to identify patterns in MASLD prevalence based on diagnostic modalities and to assess the independent contribution of MASLD to cardiovascular risk in T1D patients.
Conclusion: MASLD is increasingly recognized as a significant contributor to CVD in individuals with T1D, particularly in those with shared risk factors like obesity and insulin resistance. Evidence suggests that MASLD exacerbates hepatic and systemic metabolic dysfunction, increasing CVD risk through mechanisms such as chronic inflammation and atherogenic lipid profiles. Routine liver health assessments and tailored management strategies targeting MASLD should be incorporated into clinical care for individuals with T1D to mitigate long-term cardiovascular complications.
Type 1 diabetes (T1D) is a common chronic metabolic disorder characterized by insulin deficiency and hyperglycemia, with an estimated worldwide prevalence of 95 per 100,000 people (1). Globally, the incidence of T1D is rising, particularly among children, although an estimated 25-50% of new diagnoses occur in adults (2–5). Managing T1D poses significant health challenges due to the chronic and highly demanding nature of the disease. Despite meticulous management, individuals with T1D remain at risk of developing both acute and long-term complications.
Recent advances in treatment, particularly hybrid closed-loop systems, have substantially improved quality of life and reduced the incidence of insulin-related acute complications such as severe hypoglycemia, hyperglycemia, and ketoacidosis (6). However, despite significant advances in diabetes management and recommendations of aggressive strategies to control cardiometabolic risk factors (i.e., lower blood pressure and cholesterol thresholds), individuals with T1D continue to experience higher rates of morbidity and mortality compared to the general population (7). Cardiovascular diseases (CVD) remain the leading cause of death among people with T1D. While traditional risk factors such as dyslipidemia, hypertension, and poor glycemic control play a role, they do not fully explain the increased risk of adverse CVD outcomes. This underscores the need for further research to identify additional contributing factors (8).
Recent studies suggest a link between metabolic dysfunction–associated steatotic liver disease (MASLD) and CVD In patients with T1D. In this narrative review, we will explore risk factors for CVD, the emerging role of the liver in cardiometabolic health, and management of MASLD and CVD risk in individuals with T1D. A deeper understanding of MASLD’s impact on CVD in T1D could lead to more accurate risk assessments and improved prevention strategies.
Cardiovascular disease is highly prevalent among individuals with T1D and is the leading cause of morbidity and mortality in this population (7). Coronary artery disease/atherosclerosis is the most common type of CVD (9). However, peripheral artery disease, cerebrovascular disease (stroke), heart failure, and cardiomyopathy also occur more frequently in those with T1D compared to the general population.
The increased burden of CVD in individuals with T1D has been consistently reported in observational studies across various cohorts. Earlier studies in individuals with juvenile diabetes (diagnosed before age 21 years) reported higher cumulative mortality rate (35 ± 5%) due to coronary artery disease compared to healthy subjects in the Framingham Heart Study (8% for men and 4% for women) by age 55 (10). Similarly, the Pittsburgh Epidemiology of Diabetes Complications study of childhood-onset type 1 diabetes showed a 19-fold increased relative risk of CVD mortality particularly in younger adults (< 45 years) compared to the age-matched background population (11). More recently, in a large nationwide cohort study in Denmark involving young people (ages 1–39), CVD mortality was found to be 11 times higher in individuals with T1D compared to age-matched individuals without diabetes (12).
Among adults with T1D, the prevalence of CVD is seven times higher than in healthy controls and typically arises a decade earlier, resulting in a shortened life expectancy by an average of 10 years (7, 13). This was further supported by a Swedish cohort study, which demonstrated that the age at T1D diagnosis is an important determinant of CVD risk. Individuals diagnosed between ages 0–10 had a fivefold higher risk of developing CVD compared to those diagnosed between ages 26–30 (14). Additionally, early-onset T1D was associated with a greater loss of life expectancy compared to late-onset T1D, with CVD-related mortality being higher among women than men (14).
Although clinically manifest CVD is rare before adulthood, early signs – such as increased arterial intima-media thickness (IMT), arterial stiffness, and decreased pulse wave velocity (PWV) – can be detected during childhood. These findings are not surprising, as CVD risk factors are prevalent among youth with T1D. In a cohort study involving multi-ethnic children with T1D in the UK, up to 60% of patients were found to have at least one traditional CVD risk factor (15). Several studies, including SEARCH-CVD (an ancillary study to SEARCH for Diabetes in Youth) in the US, have shown increased carotid intima-media thickness (cIMT) in youth with T1D compared to healthy controls (16). A systematic review and meta-analysis by Giannopoulou et al., which compiled data from 23 studies (n=2,860 T1D and n=1,861 control subjects), found higher cIMT and PWV in children with T1D than in matched controls (17).
The increased prevalence of CVD in individuals with diabetes is attributed to a combination of modifiable and non-modifiable factors, further complicated by social determinants of health and individual-level differences in behaviors (18). Prolonged hyperglycemia, dyslipidemia, hypertension, smoking, obesity, and presence of microvascular complications (i.e., nephropathy) are regarded as traditional risk factors for adverse CVD outcomes (19). While improvements in these risk factors are associated with reductions in cardiovascular events and decreased mortality, even sustained improvements do not fully mitigate adverse CVD outcomes. This suggests the role of additional unexplored risk factors in CVD morbidity and mortality in T1D (20). Recently, emerging roles of insulin resistance (IR), visceral adiposity, chronic inflammation, increased oxidative stress, epigenetic modification, and cardiac autoimmunity have been recognized in the pathogenesis of CVD in diabetes (21, 22).
Metabolic dysfunction–associated steatotic liver disease (MASLD), formerly known as nonalcoholic fatty liver disease or NAFLD, is the most common etiology of chronic liver disease in children and adults (23, 24). The MASLD spectrum ranges from simple hepatosteatosis (HS) to metabolic dysfunction–associated steatohepatitis (MASH), cirrhosis, and eventually liver failure. MASLD is considered the hepatic manifestation of metabolic syndrome and is strongly associated with obesity, insulin resistance, dyslipidemia, hypertension, and increased waist circumference. It is estimated that up to 10% of all children in the United States have some form of steatotic liver disease, with a prevalence of up to 50% among girls with obesity and polycystic ovarian syndrome (PCOS) (23, 25). MASH-related cirrhosis is one of the leading causes of liver transplantation (25). Despite the well-established relationship between MASLD and T2D, the association between MASLD and T1D has been largely unexplored.
Rates of obesity among individuals with T1D are increasing in parallel with global population trends in both children and adults, with more than a third of patients with T1D estimated to have overweight or obesity (26, 27). Excess weight gain may also occur over time due to intensive insulin therapy, inadequate exercise, and excess food intake, often in defense against hypoglycemia. Despite a substantial proportion of T1D subjects sharing similar phenotypes to those with T2D, such as obesity, insulin resistance, dyslipidemia, chronic hyperglycemia, and systemic hyperinsulinemia, routine screening for MASLD in T1D is not the standard of care in clinical practice. However, ADA’s 2024 standards of care guidelines urge the providers to consider screening for fibrosis in people with T1D in the presence of additional risk factors for MASLD such as obesity, incidental hepatic steatosis on imaging, or elevated plasma aminotransferases.
Epidemiological studies assessing the prevalence of MASLD in youth with T1D are scarce. Furthermore, results from the available studies are not readily comparable due to differences in the diagnostic modalities and study populations (Table 1). In a meta-analysis assessing MASLD prevalence in T1D, comprising 20 studies in children and adults, De Vries and colleagues (28) found a pooled prevalence of 7.9% (95% CI: 2.6-15.5%) among children with T1D (n=202 in 3 studies), compared to 22.0% (95% CI: 13.9-31.2%) among adults with T1D (n=3699 in 17 studies).
MASLD prevalence has consistently been reported as higher in studies utilizing ultrasound as the diagnostic tool (29–33). This may be partly due to ultrasound’s inability to distinguish glycogenopathy and fatty infiltration in liver images. For example, Regnell et al. (34) found no cases of MASLD using hepatic fat fraction MRI for diagnosis in a small set of children (n=22) with T1D. Similarly, Kummer et al. (35) reported only one potential MASLD case in a cohort of 93 children using a combination of ultrasound, transient elastography (FibroScan®), and acoustic radiation force imaging. However, these studies had very few or no subjects with obesity, limiting their generalizability given the rising prevalence of obesity among children with T1D. In contrast, a study using MRI as the diagnostic tool linked MASLD to obesity (36). Likewise, in our previous work, we demonstrated that pediatric patients with T1D and obesity (n=15) shared similar clinical, laboratory, and imaging findings (FibroScan®) compared to patients with obesity without T1D (n=28), supporting the notion that obesity is the major determinant of MASLD (37). In a cross-sectional study, West et al. (38) demonstrated that elevated serum alanine aminotransferase (ALT) levels were more common in T1D patients (n=517, majority adults) than in the general population, with a prevalence of 9.5% (95%CI: 7.1-12.3%).
More recently, Koutny et al., using serum ALT levels as a proxy for MASLD, examined the longitudinal data from 32,325 children (age 2-17 years) with T1D in the Diabetes-Patienten-Verlaufsdokumentation study (39). They reported that children with poorly controlled T1D (i.e., HbA1c > 11%) had 2.54 (95% CI: 2.10-3.10) times the odds of having elevated liver enzymes after adjusting for sex, age, diabetes duration, and overweight status. Their findings suggest that the association between T1D and MASLD is independent of weight status.
Estimates of MASLD burden among T1D patients vary widely, depending on the population studied and diagnostic modality used, leaving the true prevalence and impact of MASLD in T1D remain unclear (40). Moreover, there is a paucity of pediatric studies in the T1D population utilizing histology (i.e., liver biopsy) as the diagnostic tool. As a result, the prognostic importance of elevated liver enzymes or abnormal imaging findings remains unknown. In a more recent meta-analysis, Ciardullo and Perseghin reported a 5% prevalence for increased liver stiffness, a surrogate marker for fibrosis, in adult subjects with T1D (41).
The “two-hit” model and its extension, the “multiple parallel hits” hypothesis, offer a detailed understanding of the pathogenesis of MASLD and its systemic repercussions. According to these frameworks, chronic inflammation in MASLD emerges from a combination of various stressors acting simultaneously, such as insulin resistance and lipotoxicity, particularly in genetically susceptible individuals (42, 43). Excessive liver fat accumulation in MASLD results from an imbalance between lipid accumulation and utilization. This imbalance is driven by peripheral and hepatic IR, which promotes increased hepatic uptake of free fatty acids and enhanced triglyceride synthesis. When the liver’s ability to oxidize fatty acids or export triglycerides as very-low-density lipoproteins cannot keep pace with fat accumulation, hepatosteatosis occurs.
In T1D, several factors contribute to MASLD, mirroring those seen in obesity and T2D. These factors include poor glycemic control, obesity, IR, dyslipidemia, lipoprotein abnormalities, poor dietary habits, impaired systemic to portal insulin gradient, and altered gut microbiome in genetically susceptible individuals (44, 45).
In a cross-sectional study of young adults with T1D (n=659, mean age 37 ± 13 years, mean diabetes duration 20 ± 12 years), Della Pepa et al. (46) reported an association between elevated HbA1c and MASLD. In their cohort, patients with HbA1c > 7.6% had significantly higher liver indices—the fatty liver index (FLI) and hepatic steatosis index, two commonly used non-invasive composite scoring systems to determine risk for MASLD with higher scores predicting MASLD. This association was independent of obesity status. A more recent cross-sectional study examined the association of continuous glucose meter-derived outcomes with MASLD in adults with T1D (n=302, median age 49 [34-61] years, median diabetes duration 29 [17-38] years).The study found independent associations between MASLD (ultrasound diagnosed) and time in range (55% ± 16%, p=0.028), time above range (p=0.007), and time below range (p=0.036) (47). These studies indicate that overall glycemic control and blood glucose variability are linked to MASLD.
As mentioned above, individuals with T1D often have obesity rates comparable to their age-matched peers at diagnosis and are at risk for further weight gain due to systemic insulin therapy, frequent snacking to manage hypoglycemia, and reduced physical activity (48–50). The interplay between hyperglycemia, hyperinsulinemia, and hyperglucagonemia exacerbates IR. Elevated glucose levels enhance hepatic glucose uptake through glucose transporter 2 (GLUT 2) and stimulate genes encoding sterol regulatory element-binding proteins (SREBP) and carbohydrate-responsive element-binding protein (ChREBP), two transcription factors upregulating hepatic de novo lipogenesis (51). Additionally, systemic hyperinsulinemia and reduced hepatic insulin clearance—potentially due to decreased expression of carcinoembryonic antigen-related cell adhesion molecule 1 (CEACAM1) because of hepatic IR—further promote hepatic lipogenesis (52).
These mechanisms collectively contribute to the development of MASLD in T1D patients. Moreover, steatotic or inflamed liver releases pro-inflammatory and pro-coagulant factors mediating vascular endothelial damage (53).
The link between MASLD and adverse CVD outcomes is well-documented in subjects with obesity and T2D (54). Examining the nationwide health screening database of more than 9.5 million adults (age 40-64 years) in South Korea, Lee et al. (55) estimated a hazard ratio of 1.43 (95% CI: 1.41-1.45) for CVD events – such as myocardial infarction, ischemic stroke, heart failure, or CVD-related death – in individuals with MASLD compared to a reference group with no fatty liver disease (unadjusted for diabetes or other potential mediators). Similarly, Vaz et al. (56) analyzed the data obtained longitudinally from adults in Australia (25,469 person-years follow-up) and found that subjects with MASLD had a 1.5 times (95% CI: 1.11-2.06) the hazard ratio of adverse CVD outcomes, including non-fatal myocardial infarction, cerebrovascular accidents, and CVD-related deaths, after adjusting for demographic covariates and known cardiometabolic risk factors, including diabetes. Both studies used fatty liver index, a biomarker panel for MASLD diagnosis.
The relationship between MASLD and CVD is hypothesized to be bidirectional such that MASLD is not only a marker of CVD but also a contributor to its pathogenesis. Hepatosteatosis might contribute to hepatic and systemic IR, and atherogenic dyslipidemia (57). Steatosis triggers inflammatory pathways and cytokine release, leading to liver fibrosis and systemic inflammation. Studies highlight the predictive role highly sensitive C-reactive protein as a marker of low-grade systemic inflammation in adverse CVD outcomes in patients with MASLD (58, 59). However, due to overlapping risk factors for the development of CVD and MASLD, it remains challenging to assess a causal relationship (Table 2).
Emerging evidence suggests a connection between MASLD and CVD in adolescents and young adults. A Swedish nationwide cohort study (60) found that children and young adults under 25 years (n=699) with biopsy-confirmed MASLD had significantly higher rates of incident CVD events compared to matched population controls. Specifically, they reported adjusted hazard ratios (aHR) of 2.33 (95% CI: 1.43 to 3.78) for ischemic heart disease, 3.07 (95% CI: 1.62 to 5.83) for congestive heart disease, and 3.16 (95% CI: 1.49 to 6.68) for arrhythmia after a median follow-up of 16.6 years. Notably, the incident rates were higher in the advanced liver disease subgroup, suggesting a possible role of the liver in the progression of CVD. Additionally, a recent systematic review and meta-analysis (61) that examined the data from 4 large cohorts (10,668,189 participants) corroborated these findings, demonstrating a strong link between MASLD in young adults and children and the risk of CVD, with a HR of 1.63 (95% CI: 1.46–1.82, p<0.001).
Data on CVD and MASLD in T1D population is scarce. Major barriers to directly assessing this relationship, particularly in children and young adults, include the natural course of MASLD and CVD (i.e., long asymptomatic phases), the lack of non-invasive diagnostic tools to stage MASLD, the reliance on composite scores rather than clinical outcomes in CVD assessment, and the presence of confounding factors that often co-exist with both conditions such as dyslipidemia, hypertension, visceral adiposity, and obesity.
Despite these challenges, a few studies have shown that MASLD is independently associated with adverse cardiovascular outcomes (62) and microvascular complications (63, 64) in individuals with T1D. In an observational study of 286 adults with T1D (median diabetes duration 17 [10-30] years), Mantovani et al. (62) assessed baseline MASLD status via ultrasound and followed the patients for a mean of 5.3 ± 2.1 years for the occurrence of incident CVD events. After adjusting for demographic and clinical covariates, the incidence of composite CVD events was found to be 6.73 times (95% CI: 1.2–38.1) higher in those with MASLD than those without MASLD, highlighting the importance of non-traditional risk factors for adverse CVD outcomes. The same research group replicated their findings on two other cohorts, demonstrated an independent relationship between MASLD and CVD, even after adjusting for age, sex, body mass index, glycemic control (i.e., HbA1c), diabetes duration, plasma lipids, albuminuria, smoking status, and family history of CVD (65, 66). It is important to note that the presence of fatty liver was assessed using ultrasound, and the prevalence of MASLD varied between 44 and 52% in these studies, which is significantly higher than most other prevalence studies (28).
Cross-sectional studies from two different research groups reported similar findings. Zhang et al. (67) assessed the relationship between MASLD and cIMT in adult T1D patients. Of the 722 patients (mean age 46 ± 13 years, diabetes duration 7.5 ± 4.2 years), 15.9% had MASLD by ultrasound. This subgroup had greater cIMT (0.81 ± 0.25 vs. 0.69 ± 0.18 mm; p<0.001) than non-MASLD patients even after adjusting for potential confounders. Moreover, MASLD was independently associated with cIMT in a linear regression model (standardized β, 0.151, p < 0.001). Similarly, Serra-Planas et al. (68) examined the prevalence of MASLD and its relationship to CVD risk factors in 100 adult T1D patients (mean age 39.4 ± 7.8 years, diabetes duration 21.7 ± 8.6 years. They reported a lower MASLD (only 12% of the cohort) by ultrasound. Those with MASLD had a greater cIMT than those without NAFLD (0.65 ± 0.17 vs 0.55 ± 0.14 mm; p=0.029), but the groups did not differ regarding carotid artery calcification score or the presence of carotid plaque—two other markers of CVD.
In a recently published observational prospective study with a median follow-up of 11 years, Garofolo et al. (69) examined the association between MASLD (assessed by FLI), all-cause mortality, and first cardiovascular events in 774 young adult T1D patients (mean age 30.3 ± 11.1 years, diabetes duration 18.5 ± 11.6 years, HbA1c 7.8 ± 1.2%). They showed an increased incident of CVD events in those with elevated FLI, even after adjusting for clinical and biochemical factors considered important in Steno Type 1 and EURODIAB Risk Engines for determining CVD risk.
Traditionally, CVD risk assessment in T1D has focused on factors like glycemic control, blood pressure, lipid levels, and prevention and treatment of diabetic kidney disease. Although the aforementioned studies show an independent association between MASLD and CVD in T1D population, evidence providing a causal relationship is lacking. Given the accumulating data suggesting that MASLD is an independent CVD risk factor, the liver health assessments should be included in risk estimation and individualized treatment.
In an ongoing clinical trial in adults at the University Hospital, Antwerp, Belgium (NCT04664036), the researchers are examining the utility of non-invasive diagnostic tools for assessing MASLD and their correlation with microvascular and macrovascular complications of diabetes in a prospective manner. The findings of study are expected to shed light on liver’s role in the development of CVD in individuals with T1D. Detecting and managing MASLD could potentially reduce the risk of developing cardiovascular complications, the leading cause of morbidity and mortality in individuals with T1D.
In conclusion, the complex interplay between MASLD and CVD in T1D patients underscores the importance of early detection and comprehensive management of MASLD. Despite advancements in T1D therapies, including continuous glucose monitors and hybrid closed-loop systems, individuals with T1D continue to face an elevated risk of CVD, which is not fully explained by traditional factors such as dyslipidemia, hypertension, and poor glycemic control. Emerging evidence now points to MASLD as a significant contributor to cardiovascular risk, even in the T1D population. MASLD, once primarily linked to T2D, is increasingly recognized in T1D due to overlapping risk factors such as obesity, insulin resistance, and chronic inflammation. This bidirectional relationship between MASLD and CVD, characterized by hepatic insulin resistance, atherogenic dyslipidemia, and systemic inflammation, suggests that MASLD may be both a marker and a mediator of CVD risk in T1D.
Considering available and proposed treatment options, managing MASLD in T1D patients requires a multifaceted approach. Lifestyle interventions, including weight loss, exercise, and dietary modifications, remain central to MASLD management, particularly in individuals with concurrent obesity. Pharmacological therapies targeting the liver are also being explored. Current treatments under investigation for MASLD in T2D, such as GLP-1 receptor agonists (70) and SGLT-2 inhibitors (71), have shown promise in improving liver steatosis and reducing CVD risk and may hold potential for T1D patients. These agents not only improve glycemic control but also promote weight loss, reduce inflammation, and improve lipid profiles—key factors in managing MASLD. Additionally, new therapies targeting fibrosis, such as FGF21 analogs, are in clinical trials and may offer benefits in preventing progression from simple steatosis to more advanced liver disease (72). While no specific treatments have been validated for MASLD in T1D, ongoing research in T2D could inform future therapeutic strategies. Early liver health assessments and tailored interventions in T1D patients could significantly reduce the burden of CVD, emphasizing the importance of integrated cardiometabolic care for improving long-term outcomes.
ET: Funding acquisition, Conceptualization, Data curation, Writing – original draft. BKV: Conceptualization, Writing – review & editing. BM: Writing – review & editing. IL: Conceptualization, Supervision, Writing – review & editing. RM: Conceptualization, Data curation, Funding acquisition, Methodology, Writing – review & editing.
The author(s) declare financial support was received for the research, authorship, and/or publication of this article. This project is supported by UPMC Children's Hospital Foundation Cochrane-Weber Grant (CHP0016748) and UPMC Children's Scholar Grant (#04-20543).
The authors declare that the research was conducted in the absence of any commercial or financial relationships that could be construed as a potential conflict of interest.
The author(s) declared that they were an editorial board member of Frontiers, at the time of submission. This had no impact on the peer review process and the final decision.
All claims expressed in this article are solely those of the authors and do not necessarily represent those of their affiliated organizations, or those of the publisher, the editors and the reviewers. Any product that may be evaluated in this article, or claim that may be made by its manufacturer, is not guaranteed or endorsed by the publisher.
1. Mobasseri M, Shirmohammadi M, Amiri T, Vahed N, Hosseini Fard H, Ghojazadeh M. Prevalence and incidence of type 1 diabetes in the world: a systematic review and meta-analysis. Health Promot Perspect. (2020) 10:98–115. doi: 10.34172/hpp.2020.18
2. Gong B, Yang W, Xing Y, Lai Y, Shan Z. Global, regional, and national burden of type 1 diabetes in adolescents and young adults. Pediatr Res. (2024). doi: 10.1038/s41390-024-03107-5
3. Rogers MAM, Kim C, Banerjee T, Lee JM. Fluctuations in the incidence of type 1 diabetes in the United States from 2001 to 2015: a longitudinal study. BMC Med. (2017) 15:199. doi: 10.1186/s12916-017-0958-6
4. Thunander M, Petersson C, Jonzon K, Fornander J, Ossiansson B, Torn C, et al. Incidence of type 1 and type 2 diabetes in adults and children in Kronoberg, Sweden. Diabetes Res Clin Pract. (2008) 82:247–55. doi: 10.1016/j.diabres.2008.07.022
5. Weng J, Zhou Z, Guo L, Zhu D, Ji L, Luo X, et al. Incidence of type 1 diabetes in China, 2010-13: population based study. BMJ. (2018) 360:j5295. doi: 10.1136/bmj.j5295
6. Templer S. Closed-loop insulin delivery systems: past, present, and future directions. Front Endocrinol (Lausanne). (2022) 13:919942. doi: 10.3389/fendo.2022.919942
7. Secrest AM, Washington RE, Orchard TJ, Cowie CC, Casagrande SS, Menke A, et al. Mortality in type 1 diabetes. In: Cowie CC, Casagrande SS, Menke A, Cissell MA, Eberhardt MS, Meigs JB, editors. Diabetes in America, 3rd ed. Bethesda (MD (2018).
8. Snell-Bergeon JK, Nadeau K. Cardiovascular disease risk in young people with type 1 diabetes. J Cardiovasc Transl Res. (2012) 5:446–62. doi: 10.1007/s12265-012-9363-x
9. Colom C, Rull A, Sanchez-Quesada JL, Perez A. Cardiovascular disease in type 1 diabetes mellitus: epidemiology and management of cardiovascular risk. J Clin Med. (2021) 10. doi: 10.3390/jcm10081798
10. Krolewski AS, Kosinski EJ, Warram JH, Leland OS, Busick EJ, Asmal AC, et al. Magnitude and determinants of coronary artery disease in juvenile-onset, insulin-dependent diabetes mellitus. Am J Cardiol. (1987) 59:750–5. doi: 10.1016/0002-9149(87)91086-1
11. Miller RG, Mahajan HD, Costacou T, Sekikawa A, Anderson SJ, Orchard TJ. A contemporary estimate of total mortality and cardiovascular disease risk in young adults with type 1 diabetes: the Pittsburgh epidemiology of diabetes complications study. Diabetes Care. (2016) 39:2296–303. doi: 10.2337/dc16-1162
12. Svane J, Lynge TH, Pedersen-Bjergaard U, Jespersen T, Gislason GH, Risgaard B, et al. Cause-specific mortality in children and young adults with diabetes mellitus: A Danish nationwide cohort study. Eur J Prev Cardiol. (2021) 28:159–65. doi: 10.1177/2047487319836550
13. Arffman M, Hakkarainen P, Keskimaki I, Oksanen T, Sund R. Long-term and recent trends in survival and life expectancy for people with type 1 diabetes in Finland. Diabetes Res Clin Pract. (2023) 198:110580. doi: 10.1016/j.diabres.2023.110580
14. Rawshani A, Sattar N, Franzen S, Rawshani A, Hattersley AT, Svensson AM, et al. Excess mortality and cardiovascular disease in young adults with type 1 diabetes in relation to age at onset: a nationwide, register-based cohort study. Lancet. (2018) 392:477–86. doi: 10.1016/S0140-6736(18)31506-X
15. Jones S, Khanolkar AR, Gevers E, Stephenson T, Amin R. Cardiovascular risk factors from diagnosis in children with type 1 diabetes mellitus: a longitudinal cohort study. BMJ Open Diabetes Res Care. (2019) 7:e000625. doi: 10.1136/bmjdrc-2018-000625
16. Urbina EM, Dabelea D, D’Agostino RB Jr., Shah AS, Dolan LM, Hamman RF, et al. Effect of type 1 diabetes on carotid structure and function in adolescents and young adults: the SEARCH CVD study. Diabetes Care. (2013) 36:2597–9. doi: 10.2337/dc12-2024
17. Giannopoulou EZ, Doundoulakis I, Antza C, Christoforidis A, Haidich AB, Kotsis V, et al. Subclinical arterial damage in children and adolescents with type 1 diabetes: A systematic review and meta-analysis. Pediatr Diabetes. (2019) 20:668–77. doi: 10.1111/pedi.12874
18. Shah AS, Wilson DP. Cardiovascular risk reduction in youth with diabetes- opportunities and challenges. In: Feingold KR, Anawalt B, Blackman MR, Boyce A, Chrousos G, Corpas E, editors. Endotext. South Dartmouth (MA (2000).
19. Donaghue K, Jeanne Wong SL. Traditional cardiovascular risk factors in adolescents with type 1 diabetes mellitus. Curr Diabetes Rev. (2017) 13:533–43. doi: 10.2174/1573399813666170124095113
20. Rawshani A, Rawshani A, Franzen S, Eliasson B, Svensson AM, Miftaraj M, et al. Mortality and cardiovascular disease in type 1 and type 2 diabetes. N Engl J Med. (2017) 376:1407–18. doi: 10.1056/NEJMoa1608664
21. Poznyak A, Grechko AV, Poggio P, Myasoedova VA, Alfieri V, Orekhov AN. The diabetes mellitus-atherosclerosis connection: the role of lipid and glucose metabolism and chronic inflammation. Int J Mol Sci. (2020) 21. doi: 10.3390/ijms21051835
22. Sousa GR, Pober D, Galderisi A, Lv H, Yu L, Pereira AC, et al. Glycemic control, cardiac autoimmunity, and long-term risk of cardiovascular disease in type 1 diabetes mellitus. Circulation. (2019) 139:730–43. doi: 10.1161/CIRCULATIONAHA.118.036068
23. Vos MB, Abrams SH, Barlow SE, Caprio S, Daniels SR, Kohli R, et al. NASPGHAN clinical practice guideline for the diagnosis and treatment of nonalcoholic fatty liver disease in children: recommendations from the expert committee on NAFLD (ECON) and the North American society of pediatric gastroenterology, hepatology and nutrition (NASPGHAN). J Pediatr Gastroenterol Nutr. (2017) 64:319–34. doi: 10.1097/MPG.0000000000001482
24. Younossi ZM, Koenig AB, Abdelatif D, Fazel Y, Henry L, Wymer M. Global epidemiology of nonalcoholic fatty liver disease-Meta-analytic assessment of prevalence, incidence, and outcomes. Hepatology. (2016) 64:73–84. doi: 10.1002/hep.28431
25. Andrisse S, Garcia-Reyes Y, Pyle L, Kelsey MM, Nadeau KJ, Cree-Green M. Racial and ethnic differences in metabolic disease in adolescents with obesity and polycystic ovary syndrome. J Endocr Soc. (2021) 5:bvab008. doi: 10.1210/jendso/bvab008
26. Corbin KD, Driscoll KA, Pratley RE, Smith SR, Maahs DM, Mayer-Davis EJ, et al. Obesity in type 1 diabetes: pathophysiology, clinical impact, and mechanisms. Endocr Rev. (2018) 39:629–63. doi: 10.1210/er.2017-00191
27. Libman IM, Pietropaolo M, Arslanian SA, LaPorte RE, Becker DJ. Changing prevalence of overweight children and adolescents at onset of insulin-treated diabetes. Diabetes Care. (2003) 26:2871–5. doi: 10.2337/diacare.26.10.2871
28. de Vries M, Westerink J, Kaasjager K, de Valk HW. Prevalence of nonalcoholic fatty liver disease (NAFLD) in patients with type 1 diabetes mellitus: A systematic review and meta-analysis. J Clin Endocrinol Metab. (2020) 105:3842–53. doi: 10.1210/clinem/dgaa575
29. Al-Hussaini AA, Sulaiman NM, Alzahrani MD, Alenizi AS, Khan M. Prevalence of hepatopathy in type 1 diabetic children. BMC Pediatr. (2012) 12:160. doi: 10.1186/1471-2431-12-160
30. El-Karaksy HM, Anwar G, Esmat G, Mansour S, Sabry M, Helmy H, et al. Prevalence of hepatic abnormalities in a cohort of Egyptian children with type 1 diabetes mellitus. Pediatr Diabetes. (2010) 11:462–70. doi: 10.1111/j.1399-5448.2009.00627.x
31. Elkabbany ZA, Elbarbary NS, Ismail EA, Mohamed NA, Ragab D, Abdel Alem S, et al. Transient elastography as a noninvasive assessment tool for hepatopathies of different etiology in pediatric type 1 diabetes mellitus. J Diabetes Complications. (2017) 31:186–94. doi: 10.1016/j.jdiacomp.2016.09.009
32. Aydin F, Gerenli N, Dursun F, Atasoy TO, Kalin S, Kirmizibekmez H. Hepatopathies in children and adolescents with type 1 diabetes. J Pediatr Endocrinol Metab. (2019) 32:121–6. doi: 10.1515/jpem-2018-0255
33. Abdallah HR, Youness ER, Bedeir MM, Abouelnaga MW, Ezzat WM, Elhosary Y, et al. Clinical and diagnostic characteristics of non-alcoholic fatty liver disease among Egyptian children and adolescents with type1 diabetes. Diabetol Metab Syndr. (2023) 15:52. doi: 10.1186/s13098-023-01029-6
34. Regnell SE, Peterson P, Trinh L, Broberg P, Leander P, Lernmark A, et al. Magnetic resonance imaging reveals altered distribution of hepatic fat in children with type 1 diabetes compared to controls. Metabolism. (2015) 64:872–8. doi: 10.1016/j.metabol.2015.04.002
35. Kummer S, Klee D, Kircheis G, Friedt M, Schaper J, Haussinger D, et al. Screening for non-alcoholic fatty liver disease in children and adolescents with type 1 diabetes mellitus: a cross-sectional analysis. Eur J Pediatr. (2017) 176:529–36. doi: 10.1007/s00431-017-2876-1
36. Sae-Wong J, Chaopathomkul B, Phewplung T, Chaijitraruch N, Sahakitrungruang T. The prevalence of nonalcoholic fatty liver disease and its risk factors in children and young adults with type 1 diabetes mellitus. J Pediatr. (2021) 230:32–7 e1. doi: 10.1016/j.jpeds.2020.10.043
37. Tas E, Bai S, Mak D, Diaz EC, Dranoff JA. Obesity, but not glycemic control, predicts liver steatosis in children with type 1 diabetes. J Diabetes Complications. (2022) 36:108341. doi: 10.1016/j.jdiacomp.2022.108341
38. West J, Brousil J, Gazis A, Jackson L, Mansell P, Bennett A, et al. Elevated serum alanine transaminase in patients with type 1 or type 2 diabetes mellitus. QJM. (2006) 99:871–6. doi: 10.1093/qjmed/hcl116
39. Koutny F, Wiemann D, Eckert A, Meyhofer S, Fritsch M, Pappa A, et al. Poorly controlled pediatric type 1 diabetes mellitus is a risk factor for metabolic dysfunction associated steatotic liver disease (MASLD): An observational study. J Pediatr Gastroenterol Nutr. (2024) 78:1027–37. doi: 10.1002/jpn3.12194
40. Mertens J, Van Gaal LF, Francque SM, De Block C. NAFLD in type 1 diabetes: overrated or underappreciated? Ther Adv Endocrinol Metab. (2021) 12:20420188211055557. doi: 10.1177/20420188211055557
41. Ciardullo S, Perseghin G. Prevalence of elevated liver stiffness in patients with type 1 and type 2 diabetes: A systematic review and meta-analysis. Diabetes Res Clin Pract. (2022) 190:109981. doi: 10.1016/j.diabres.2022.109981
42. Day CP, James OF. Steatohepatitis: a tale of two “hits”? Gastroenterology. (1998) 114(4):842–5. doi: 10.1016/s0016-5085(98)70599-2
43. Tilg H, Moschen AR. Evolution of inflammation in nonalcoholic fatty liver disease: the multiple parallel hits hypothesis. Hepatology. (2010) 52:1836–46. doi: 10.1002/hep.24001
44. Memaj P, Jornayvaz FR. Non-alcoholic fatty liver disease in type 1 diabetes: Prevalence and pathophysiology. Front Endocrinol (Lausanne). (2022) 13:1031633. doi: 10.3389/fendo.2022.1031633
45. Schwarzler J, Grabherr F, Grander C, Adolph TE, Tilg H. The pathophysiology of MASLD: an immunometabolic perspective. Expert Rev Clin Immunol. (2024) 20:375–86. doi: 10.1080/1744666X.2023.2294046
46. Della Pepa G, Lupoli R, Masulli M, Boccia R, De Angelis R, GianFrancesco S, et al. Blood glucose control and metabolic dysfunction-associated steatotic liver disease in people with type 1 diabetes. J Endocrinol Invest. (2024) 47:2371–8. doi: 10.1007/s40618-024-02333-2
47. Aernouts C, Belde SPW, Lambrechts J, Mertens J, Ledeganck KJ, Francque SM, et al. Metabolic dysfunction-associated steatotic liver disease is associated with worse time in ranges in type 1 diabetes. Diabetes Obes Metab. (2024) 26:3781–90. doi: 10.1111/dom.15723
48. Priya G, Kalra S. A review of insulin resistance in type 1 diabetes: is there a place for adjunctive metformin? Diabetes Ther. (2018) 9:349–61. doi: 10.1007/s13300-017-0333-9
49. Calcaterra V, De Silvestri A, Schneider L, Acunzo M, Vittoni V, Meraviglia G, et al. Acanthosis nigricans in children and adolescents with type 1 diabetes or obesity: the potential interplay role between insulin resistance and excess weight. Children (Basel). (2021) 8. doi: 10.3390/children8080710
50. Nadeau KJ, Regensteiner JG, Bauer TA, Brown MS, Dorosz JL, Hull A, et al. Insulin resistance in adolescents with type 1 diabetes and its relationship to cardiovascular function. J Clin Endocrinol Metab. (2010) 95:513–21. doi: 10.1210/jc.2009-1756
51. Xiao X, Luo Y, Peng D. Updated understanding of the crosstalk between glucose/insulin and cholesterol metabolism. Front Cardiovasc Med. (2022) 9:879355. doi: 10.3389/fcvm.2022.879355
52. Najjar SM, Perdomo G. Hepatic insulin clearance: mechanism and physiology. Physiol (Bethesda). (2019) 34:198–215. doi: 10.1152/physiol.00048.2018
53. Stefan N, Schick F, Birkenfeld AL, Haring HU, White MF. The role of hepatokines in NAFLD. Cell Metab. (2023) 35:236–52. doi: 10.1016/j.cmet.2023.01.006
54. Duell PB, Welty FK, Miller M, Chait A, Hammond G, Ahmad Z, et al. Nonalcoholic fatty liver disease and cardiovascular risk: A scientific statement from the American heart association. Arterioscler Thromb Vasc Biol. (2022) 42:e168–e85. doi: 10.1161/ATV.0000000000000153
55. Lee H, Lee YH, Kim SU, Kim HC. Metabolic dysfunction-associated fatty liver disease and incident cardiovascular disease risk: A nationwide cohort study. Clin Gastroenterol Hepatol. (2021) 19:2138–47 e10. doi: 10.1016/j.cgh.2020.12.022
56. Vaz K, Kemp W, Majeed A, Lubel J, Magliano DJ, Glenister KM, et al. NAFLD and MAFLD independently increase the risk of major adverse cardiovascular events (MACE): a 20-year longitudinal follow-up study from regional Australia. Hepatol Int. (2024) 18:1135–43. doi: 10.1007/s12072-024-10706-1
57. Stefan N, Haring HU, Cusi K. Non-alcoholic fatty liver disease: causes, diagnosis, cardiometabolic consequences, and treatment strategies. Lancet Diabetes Endocrinol. (2019) 7:313–24. doi: 10.1016/S2213-8587(18)30154-2
58. Huang J, Wang M, Wu Y, Kumar R, Lin S. Serum high-sensitive C-reactive protein is a simple indicator for all-cause among individuals with MAFLD. Front Physiol. (2022) 13:1012887. doi: 10.3389/fphys.2022.1012887
59. Ridker PM, Bhatt DL, Pradhan AD, Glynn RJ, MacFadyen JG, Nissen SE, et al. Inflammation and cholesterol as predictors of cardiovascular events among patients receiving statin therapy: a collaborative analysis of three randomised trials. Lancet. (2023) 401:1293–301. doi: 10.1016/S0140-6736(23)00215-5
60. Simon TG, Roelstraete B, Alkhouri N, Hagstrom H, Sundstrom J, Ludvigsson JF. Cardiovascular disease risk in paediatric and young adult non-alcoholic fatty liver disease. Gut. (2023) 72:573–80. doi: 10.1136/gutjnl-2022-328105
61. Liao YL, Zhu GY, Chang C. Non-alcoholic fatty liver disease increases the risk of cardiovascular disease in young adults and children: a systematic review and meta-analysis of cohort studies. Front Cardiovasc Med. (2023) 10:1291438. doi: 10.3389/fcvm.2023.1291438
62. Mantovani A, Mingolla L, Rigolon R, Pichiri I, Cavalieri V, Zoppini G, et al. Nonalcoholic fatty liver disease is independently associated with an increased incidence of cardiovascular disease in adult patients with type 1 diabetes. Int J Cardiol. (2016) 225:387–91. doi: 10.1016/j.ijcard.2016.10.040
63. Popa SG, Simion AM, Soare M, Arcomita D. Insulin resistance and hepatic steatosis in type 1 diabetes mellitus and their association with diabetic chronic complications. Minerva Endocrinol (Torino). (2023) 48:27–34. doi: 10.23736/S2724-6507.20.03290-3
64. Targher G, Mantovani A, Pichiri I, Mingolla L, Cavalieri V, Mantovani W, et al. Nonalcoholic fatty liver disease is independently associated with an increased incidence of chronic kidney disease in patients with type 1 diabetes. Diabetes Care. (2014) 37:1729–36. doi: 10.2337/dc13-2704
65. Targher G, Bertolini L, Padovani R, Rodella S, Zoppini G, Pichiri I, et al. Prevalence of non-alcoholic fatty liver disease and its association with cardiovascular disease in patients with type 1 diabetes. J Hepatol. (2010) 53:713–8. doi: 10.1016/j.jhep.2010.04.030
66. Targher G, Pichiri I, Zoppini G, Trombetta M, Bonora E. Increased prevalence of cardiovascular disease in Type 1 diabetic patients with non-alcoholic fatty liver disease. J Endocrinol Invest. (2012) 35:535–40. doi: 10.3275/7875
67. Zhang L, Guo K, Lu J, Zhao F, Yu H, Han J, et al. Nonalcoholic fatty liver disease is associated with increased carotid intima-media thickness in type 1 diabetic patients. Sci Rep. (2016) 6:26805. doi: 10.1038/srep26805
68. Serra-Planas E, Aguilera E, Castro L, Rodriguez R, Salinas I, Lucas A, et al. Low prevalence of non-alcoholic fatty liver disease in patients with type 1 diabetes is associated with decreased subclinical cardiovascular disease. J Diabetes. (2017) 9(12):1065–72. doi: 10.1111/1753-0407.12539
69. Garofolo M, Lucchesi D, Giambalvo M, Aragona M, Bertolotto A, Campi F, et al. Fatty liver index is an independent risk factor for all-cause mortality and major cardiovascular events in type 1 diabetes: an 11-year observational study. Cardiovasc Diabetol. (2024) 23:85. doi: 10.1186/s12933-024-02171-9
70. Nevola R, Epifani R, Imbriani S, Tortorella G, Aprea C, Galiero R, et al. GLP-1 receptor agonists in non-alcoholic fatty liver disease: current evidence and future perspectives. Int J Mol Sci. (2023) 24. doi: 10.3390/ijms24021703
71. Ala M. SGLT2 inhibition for cardiovascular diseases, chronic kidney disease, and NAFLD. Endocrinology. (2021) 162. doi: 10.1210/endocr/bqab157
Keywords: hepatosteatosis, cardiovascular disease, insulin resistance, inflammation, type 1 diabetes
Citation: Tas E, Vu B-MK, Mendizabal B, Libman I and Muzumdar R (2024) Relationship between liver and cardiometabolic health in type 1 diabetes. Front. Endocrinol. 15:1505430. doi: 10.3389/fendo.2024.1505430
Received: 02 October 2024; Accepted: 22 October 2024;
Published: 13 November 2024.
Edited by:
Sally Radovick, Rutgers, The State University of New Jersey, United StatesReviewed by:
Angela Zanfardino, University of Campania Luigi Vanvitelli, ItalyCopyright © 2024 Tas, Vu, Mendizabal, Libman and Muzumdar. This is an open-access article distributed under the terms of the Creative Commons Attribution License (CC BY). The use, distribution or reproduction in other forums is permitted, provided the original author(s) and the copyright owner(s) are credited and that the original publication in this journal is cited, in accordance with accepted academic practice. No use, distribution or reproduction is permitted which does not comply with these terms.
*Correspondence: Radhika Muzumdar, UmFkaGlrYS5tdXp1bWRhckBjaHAuZWR1
Disclaimer: All claims expressed in this article are solely those of the authors and do not necessarily represent those of their affiliated organizations, or those of the publisher, the editors and the reviewers. Any product that may be evaluated in this article or claim that may be made by its manufacturer is not guaranteed or endorsed by the publisher.
Research integrity at Frontiers
Learn more about the work of our research integrity team to safeguard the quality of each article we publish.