- 1School of Health Sciences, Guangzhou Xinhua University, Guangzhou, Guangdong, China
- 2Department of Traditional Chinese Medicine, The Third Affiliated Hospital, Sun Yat-sen University, Guangzhou, Guangdong, China
- 3Department of Gynecology, The Second Clinical School of Guangzhou University of Chinese Medicine, The Second Affiliated Hospital of Guangzhou University of Chinese Medicine, Guangdong Provincial Hospital of Chinese Medicine, Guangdong Provincial Academy of Chinese Medical Sciences, Guangzhou University of Chinese Medicine, Guangzhou, Guangdong, China
- 4Department of Spleen, Stomach, and Liver and Gallbladder Diseases, The First Affiliated Hospital of Henan University of Chinese Medicine, Zhengzhou, Henan, China
- 5College of Animal Science and Technology, Yangtze University, Jingzhou, Hubei, China
- 6Research Center for Translational Medicine, The First Affiliated Hospital, Sun Yat-Sen University, Guangzhou, Guangdong, China
- 7Department of Physiology, School of Basic Medical Sciences, Guangzhou University of Chinese Medicine, Guangzhou, Guangdong, China
- 8Research Centre of Basic Integrative Medicine, School of Basic Medical Sciences, Guangzhou University of Chinese Medicine, Guangzhou, Guangdong, China
1 Introduction
Hydrogen gas (H2), the lightest gas in the universe, can act as an important antioxidant. Supplementation of exogenous H2 can improve many diseases. However, the physiological and pathological significance of endogenous H2 is not clear. In the recent issue of Cell, McCurry et al. reveal that endogenous H2 drives 21-dehydroxylation for transforming glucocorticoids to progestins, which may further influence female health, such as postpartum depression (1). We are very interested in this milestone study that reveals the physiological effects of endogenous H2. Based on the history, here, we will discuss the process of this important finding, the uncovered questions, the current status, and the future of endogenous H2 in female health.
2 The gut bacteria chemically modify glucocorticoids into progestins through 21-dehydroxylation
In 1969, Eriksson et al. reported that the feces from germ-free (GF) rats contain corticoids but no pregnanolones, while the feces from conventional rats contain pregnanolones but no corticoids (21-hydroxylated steroids), which suggested that gastrointestinal progestin formation is dependent on gut microbiome (2, 3). Similar to this, it has been reported that fecal slurries from rats and humans can convert glucocorticoids into progestins through reductive removal of a C21 hydroxyl group, a process called 21-dehydroxylation (4, 5). Therefore, this means that the presence of 21-dehydroxylation products in the gut might depend on the gut microbiome. However, it is unclear how gut bacteria chemically modify steroids and how the resulting metabolites affect the host health.
In 2024, Dr. Megan D. McCurry et al. had developed an ultrahigh-performance liquid chromatography (UHPLC)-based method to quantify glucocorticoids and progestins in the biological fluids and tissues (1). Similar to H. Eriksson’s reports (2, 3), total levels of tetrahydroprogesterones (THPs) were significantly reduced in the feces of GF female mice compared with specific-pathogen-free (SPF) female mice (1). Then, they further identify physiologically relevant substrates for 21-dehydroxlation through targeted corticoid profiling on human bile and found that the average concentrations of tetrahydrodeoxycorticosterone (THDOC), e.g., 3α5αTHDOC and 3α5βTHDOC, in the abundant biliary corticoids were higher than other components (1). This indicated that the gut bacteria are exposed to physiologically relevant concentrations of 3α5αTHDOC and 3α5βTHDOC. Because 3α5αTHDOC levels in bile are higher in pregnant women, and its 21-dehydroxylated products have potential biological activities in the context of pregnancy and women’s health (6–8), they next focused on 3α5αTHDOC and hypothesized that 3α5αTHDOC can be 21-dehydroxylated into 3α5αTHP.
To verify their hypothesis, Megan D. McCurry cultured the feces of pregnant GF and SPF mice in the presence of 3α5αTHDOC and measured the levels of progestin, and found that a large amount of 3α5αTHP and 3β5αTHP were produced from pregnant SPF mouse fecal slurries, while fecal slurries from pregnant GF mice did not produce THPs (1). The culture pools with the human feces from healthy females and males 21-dehydroxylated 3α5αTHDOC, and moreover, the feces from pregnant human donors contained substantially higher levels of THPs than the feces from males and non-pregnant females. These indicate that both murine and human gut microbiome have the abilities to 21-dehydroxylate 3α5αTHDOC to produce progestins (1). However, why is there more 21-dehydroxylation product of 3α5αTHDOC in the context of pregnancy? One reason is that 3α5αTHDOC levels in bile are higher in pregnant women (7).
Dr. Megan D. McCurry therefore sought to isolate and characterize the 21-dehydroxylating species from human fecal microbial communities. In a culture pool isolated from a female donor, they found that Gordonibacter pamelaeae, a close relative of Eggerthella lenta, was the only bacterial species that function as active 21-dehydroxylators (1). Because the abundance of G. pamelaeae is low, they used an orthogonal assay and acquired the type strain of this bacterium, G. pamelaeae DSM 19378 (1). They found that G. pamelaeae DSM 19378 weakly 21-dehydroxylated 3α5αTHDOC to produce 3α5αTHP and 3β5αTHP, and this activity was significantly increased in co-culture with gut commensal Escherichia coli Nissle 1917 (EcN); however, EcN alone was unable to perform this transformation (1). They further tested the prevalence of 21-dehydroxylation activity in Eggerthellaceae family. In a 26-strain library of E. lenta and Gordonibacter species from the human gut, none of these strains performed 21-dehydroxylation in monoculture, and 12 strains produced varying levels of THP in co-culture with EcN (1). Thus, the members of the Eggerthellaceae family 21-dehydroxylate 3α5αTHDOC and indicate that this activity is induced by EcN (1).
3 The endogenous H2 drives 21-dehydroxylation for transforming 3α5αTHDOC into 3α5αTHP
What are the key mechanisms of induction of 21-dehydroxylation by E. coli? It has been reported that 21-dehydroxylation activity is inversely correlated with media redox potential (9). According to this, Dr. Megan D. McCurry first examined the hypothesis that E. coli lowers the redox potential and thus promoting 21-dehydroxylation. Their data showed that lower redox potential does not enable 21-dehydroxylation in E. lenta 14A (1). Second, they tested whether a 21-dehydroxylation-promoting factor was produced by EcN. The physical interactions between microbes can occur during cooperative metabolism; however, they found that contact between the two species is not required to induce 21-dehydroxylation (1). Third, they further tested whether EcN released extracellular inducing factors, and they found that EcN syringe-filtered supernatants but not vacuum-filtered supernatants induced 21-dehydroxylation in E. lenta (1). The basic difference between vacuum filtration and syringe filtration is that the dissolved gases are removed in the former. They therefore hypothesized that EcN may produce a gas that leads to 21-dehydroxylation in E. lenta. In healthy individuals, the colonic gases are composed of H2, carbon dioxide (CO2), methane (CH4), nitrogen (N2), and oxygen (O2), as well as several odiferous trace gases, and the former three are produced solely by colonic microbes (10). Thus, which gas or gases could be responsible for 21-dehydroxylation?
It has been reported that E. coli can produce H2 during its stationary phase of growth (11), which is when Dr. Megan D. McCurry started to observe substantial 21-dehydroxylation. Under anaerobic conditions, H2 can be used by microbes as an electron donor to drive sulfate reduction and methanogenesis (12). Moreover, bile acid oxidation by E. lenta can be inhibited by H2, and E. lenta performs reductive metabolic reactions under high H2 partial pressure (13). Therefore, they hypothesized that H2 might provide the reducing equivalents required for this highly reductive transformation.
Methylene blue (MB) is well known to react with an equimolar amount of H2 in the presence of platinum (Pt) or palladium to produce colorless reduced MB (leucomethylene blue, leucoMB), as follows: MB blue + 2H+ + 2e- → leucoMB colorless (14). Thus, Dr. Megan D. McCurry used MB with colloidal Pt as H2 detection reagent based on the initial work by Tomoki Seo (14) and wildly used by our group (15) and others (16, 17). They found that E. lenta 14A grown under a H2 condition, rather than a N2 condition, performed 21-dehydroxylation. The H2 levels and 21-dehydroxylation activities of E. coli with hydrogenase mutations co-cultured with E. lenta 14A were more than four times lower than those of E. coli BW25113 co-cultured with E. lenta 14A (1). In contrast to this, the H2 levels and 21-dehydroxylation activities were not significantly influenced by disrupting a reductive pathway unrelated to H2 production through loss of cysJ, the NADH:flavin oxidoreductase for the sulfite reductase in E. coli, when co-cultured with E. lenta 14A (1). Thus, H2 produced by E. coli is a major driving force that promotes robust 21-dehydroxylation in E. lenta (Figure 1). Beyond EcN 1917, the Gram-positive strains Clostridium scindens VPI 12780 and C. perfringens ATCC 13124, and the Gram-negative strains Citrobacter rodentium ATCC 8090 and C. freundii ICC 168, which express hydrogenase or hydrogenase homologs, can also induce 21-dehydroxylation in E. lenta 14A (1).
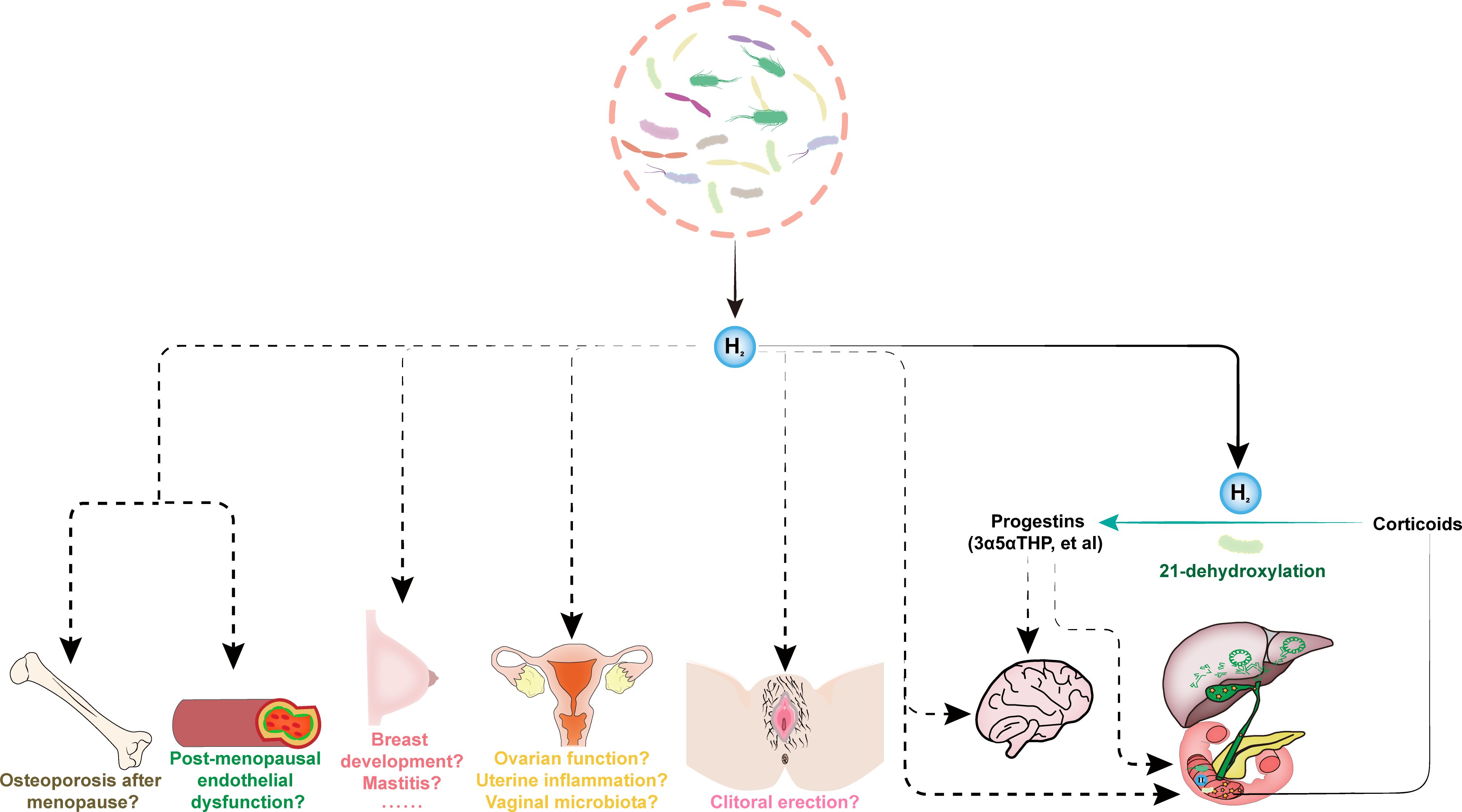
Figure 1. The endogenous H2 drives female sexual health. The gut microbiota-derived endogenous H2 in female subjects is necessary and sufficient to promote robust 21-dehydroxylation, which is a key step for converting abundant biliary corticoids into progestins, e.g., allopregnanolone (3α5αTHP). The feces from pregnant human donors contained substantially higher levels of THPs than feces from males and non-pregnant females. The low serum levels of 3α5αTHP are related to symptoms of depression in late pregnancy. Allopregnanolone (3α5αTHP), also known as brexanolone, is the first drug approved by the FDA to treat postpartum depression. 3α5αTHP, as a metabolite of gut microbiota, may also have essential impact on gut health. These are indirect effects of endogenous H2 dependent on the production of progestins, and endogenous H2 may also have direct effects independent on progestins, e.g., affecting intestinal and brain health, among others. The exogenous H2 emerged as a novel bioactive molecule involved in modulating sexual organ homeostasis and improving many reproductive diseases in animal models, including erectile dysfunction, polycystic ovary syndrome, chemotherapy-induced ovarian injury, uterine inflammation, mastitis and breast cancer, and postmenopausal osteoporosis. However, the effects of endogenous H2 on female health, such as post-menopausal endothelial dysfunction and osteoporosis, normal breast development and mastitis, ovarian function, uterine inflammation, vaginal microbiota, and clitoral erection, are unclear.
It should be noted that 21-dehydroxylation was not reduced to undetectable levels in co-cultures with H2-deficient mutants, indicating that other factors produced by EcN may also contribute to this reaction (1). Their data also showed that there are low levels of H2 produced in the co-culture of E. lenta 14A and E. coli with hydrogenase mutations (1). Thus, where do they come from? Indeed, E. lenta also contains annotated but unconfirmed hydrogenases, and they found that the E. lenta 14A monoculture can produce levels of H2 comparable to those of the E. coli Nissle monoculture (1). In another independent experiment, Megan D. McCurry found that the E. lenta 14A monoculture and G. pamelaeae DSM 19743 monoculture produce the same levels of H2 (1). 3α5αTHP and 3β5αTHP were only detected in the G. pamelaeae DSM 19743 monoculture, but not in the E. lenta 14A monoculture (1). This indicated that G. pamelaeae DSM 19743 may contain or produce other factors, rather than H2, to induce 21-dehydroxylation. The synergistic H2 production and 21-dehydroxylation of co-culture by E. lenta 14A and EcN 1917, and by G. pamelaeae DSM 19743 and EcN 1917, were higher than the monoculture of E. lenta 14A (ND) or G. pamelaeae DSM 19743 (1). Therefore, they concluded that higher H2 produced in co-culture promoted 21-dehydroxylation activity.
Thus, which candidate genes could be involved in 21-dehydroxylation in E. lenta? They performed comparative genomics analysis on the genome sequences of producer and non-producer strains from the Actinobacteria strain library, and identified gene cluster Elen_2451–Elen_2454 as the candidate genes for 21-dehydroxylation (1). By co-culturing one of the top producer strains, E. lenta 14A, with EcN with or without tungstate, which is known to inhibit the oxidoreductase activity of Elen_2453, and by homologously and heterologously expressing the Elen_2451–2454 cluster, they confirmed that Elen_2451–2454 is responsible for 21-dehydroxylation in E. lenta (1). The feces from pregnant subjects are enriched in Elen_2451–2454 cluster-containing bacteria, and the concentrations of THPs positively correlated with the abundance of Elen_2451–2454 homologs (1). The feces from co-colonized female GF mice with producer strain E. lenta 14A and EcN contained significantly more THPs than female GF controls (1). Moreover, the co-colonized GF female mice with EcN and the cluster-containing strain E. lenta 14A, rather than the cluster-lacking strain E. lenta A2, produced higher levels of total THPs and 3α5βTHP in the feces (1). Elen_2451 is a formate dehydrogenase family accessory protein FdhD, Elen_2452 is 4Fe-4S ferredoxin iron-sulfur binding domain protein, Elen_2453 is molybdopterin oxidoreductase, and Elen_2454 is an SPFH domain band 7 family protein (1). However, we are still not clear about enzymatic mechanisms by which the above cluster regulate 21-dehydroxylation.
4 Discussion: there is still a long way to go for endogenous H2 in female health
The ovarian hormone (progesterone and estrogen) levels increase over 100-fold during pregnancy, and consequently, neurosteroid allopregnanolone (also as brexanolone, 3α5αTHP) is also elevated in the brain (18, 19). The GABAAR expression in the brain is reduced by neurosteroids during pregnancy to prevent sedation (18–20). Upon parturition, hormones rapidly return to pre-pregnancy levels, but the expression of GABAAR may take time to recover (19). Therefore, a long-lasting mismatch between neurosteroid levels and GABAAR numbers may underlie postpartum depression (19). The serum levels of allopregnanolone were detectable postpartum and were significantly decreased in women with maternity “blues” (8). Brexanolone is a first Food and Drug Administration (FDA)-indicated drug for postpartum depression (21). The gut bacteria both consume and produce H2, and the remaining net H2 in a fecal culture from donor F2 was sufficient to complete 21-dehydroxylation in co-culture and produced THPs (1). The THPs, such as 3α5αTHP, 3α5βTHP, 3β5αTHP, and 3β5βTHP, are two orders of magnitude higher in feces from pregnant people in the third trimester compared with the feces of male and nonpregnant female subjects (1). However, we are unclear about the changes in levels of gastrointestinal-derived THPs in pregnant women with postpartum depression before and after delivery. We do not know whether progestins produced by E. lenta remain in the gastrointestinal tract or are absorbed into enterohepatic or systemic circulation (1). Thus, it is not clear whether gut-derived THPs can affect postpartum depression (Figure 1). Supplementation of exogenous H2 protected against depression in mice (22), and if THPs can be absorbed into enterohepatic or systemic circulation and have effects on depression, it is difficult to distinguish the observed effects of exogenous H2 on depression that are caused directly by H2 or indirectly by produced THPs or by the synergistic effects of H2 and THPs (Figure 1).
Sex entails cutting-edge science but is bathed in mystery, and it is a fundamental pleasure and quality-of-life issue (23). H2 has emerged as a novel bioactive molecule involved in modulating sexual organ homeostasis (23). Supplementation of exogenous H2 can improve erectile dysfunction in a model of diabetic rats (24) and alleviate polycystic ovary syndrome (25), chemotherapy-induced ovarian injury (26), uterine inflammation (27), mastitis (28) and breast cancer (29, 30), and postmenopausal osteoporosis (31). It also has the effect of vasodilation, and attenuates chronic intermittent hypoxia-induced hypertension in rats (32, 33). However, the effects of endogenous H2 on female health, such as post-menopausal endothelial dysfunction, osteoporosis after menopause, normal breast development and mastitis, ovarian function, uterine inflammation, vaginal microbiota, and clitoral erection are unclear (Figure 1). To answer the effects of endogenous H2 on female health, we should confirm biological distributions of endogenous H2 (and its related metabolites, such as THPs) produced by microbiota, and analyze the changes of endogenous H2 (and its related metabolites, such as THPs) and microbiota in blood and feces and organs/tissues before and after disease (or compare between normal individuals and patients). The animal models should be used to answer the causal relationship between endogenous H2 and female health. Moreover, the strategies to reduce endogenous H2 and increase endogenous H2 should be employed. Reducing endogenous H2 is typically achieved by the systematic use of antibiotics; however, the effects of antibiotics are broad and not specific, and both beneficial and harmful bacteria will be affected; therefore, this indiscriminate treatment cannot accurately answer the effects of endogenous H2. After killing gut microbial community via antibiotics, it seems that supplementing with indicated strains can provide a relatively accurate answer to the physiological functions of indicated bacteria (34). We should note that it is not possible to have only one type of bacteria in the body, and the indicated bacteria can interact with one or more other bacteria or the host cells in multiple dimensions, thereby producing a range of physiological effects.
Although there are many challenges in H2 medicine, especially endogenous H2, Megan D. McCurry’s study provides a new paradigm for future research on H2 medicine. Based on the expression profile of hydrogenase, we should investigate the physical and chemical essences of production and utilization of H2 by human microorganisms and the physical and chemical essences of utilization of H2 by the host cells and, thus, confirm the significance of endogenous H2 for human homeostasis and pathogenesis of diseases.
Author contributions
YZ: Conceptualization, Funding acquisition, Supervision, Writing – review & editing. SY: Writing – original draft. JZ: Conceptualization, Writing – review & editing. LX: Visualization, Writing – review & editing. YG: Writing – review & editing. CF: Writing – review & editing. SZ: Writing – review & editing. HY: Writing – review & editing. HL: Supervision, Writing – review & editing.
Funding
The author(s) declare financial support was received for the research, authorship, and/or publication of this article. This work was supported by the Natural Science Foundation of Guangdong Province (2023A1515012828), the Traditional Chinese Medicine (TCM) Project from TCM Bureau of Guangdong Province (20231103 and 20241081), the National Natural Science Foundation of China (81900376), the Special Project for Key Fields of Guangdong Provincial Ordinary Colleges and Universities (2024ZDZX2042), the Undergraduate Teaching Project of Guangzhou Xinhua University (Cultivation of Practical and Innovative Abilities) (B6602010310), the Guangdong Province Key Discipline Construction Project of TCM (20220104), and the National Famous TCM Inheritance Studio Construction Project (140000020162).
Acknowledgments
We apologize to the colleagues for not citing their articles of relevance to this work due to citation limitations.
Conflict of interest
The authors declare that the research was conducted in the absence of any commercial or financial relationships that could be construed as a potential conflict of interest.
Generative AI statement
The author(s) declare that no Generative AI was used in the creation of this manuscript.
Publisher’s note
All claims expressed in this article are solely those of the authors and do not necessarily represent those of their affiliated organizations, or those of the publisher, the editors and the reviewers. Any product that may be evaluated in this article, or claim that may be made by its manufacturer, is not guaranteed or endorsed by the publisher.
References
1. McCurry MD, D’Agostino GD, Walsh JT, Bisanz JE, Zalosnik I, Dong X, et al. Gut bacteria convert glucocorticoids into progestins in the presence of hydrogen gas. Cell. (2024) 187:2952–68.e2913. doi: 10.1016/j.cell.2024.05.005
2. Eriksson H, Gustafsson JA, Sjövall J. Steroids in germfree and conventional rats. 21-dehydroxylation by intestinal microorganisms. Eur J Biochem. (1969) 9:550–4. doi: 10.1111/j.1432-1033.1969.tb00644.x
3. Eriksson H, Gustafsson JA, Sjövall J. Steroids in germfree and conventional rats. Free steroids in faeces from conventional rats. Eur J Biochem. (1969) 9:286–90. doi: 10.1111/j.1432-1033.1969.tb00607.x
4. Morris DJ, Brem AS. Role of gut metabolism of adrenal corticosteroids and hypertension: clues gut-cleansing antibiotics give us. Physiol Genomics. (2019) 51:83–9. doi: 10.1152/physiolgenomics.00115.2018
5. Morris DJ, Ridlon JM. Glucocorticoids and gut bacteria: “The GALF Hypothesis” in the metagenomic era. Steroids. (2017) 125:1–13. doi: 10.1016/j.steroids.2017.06.002
6. Cadeddu R, Bäckström T, Floris G, Nordkild P, Segerdahl M, Bortolato M. Isoallopregnanolone reduces tic-like behaviours in the D1CT-7 mouse model of Tourette syndrome. J Neuroendocrinol. (2020) 32:e12754. doi: 10.1111/jne.12754
7. Laatikainen T, Karjalainen O. Excretion of conjugates of neutral steroids in human bile during late pregnancy. Acta Endocrinol (Copenh). (1972) 69:775–88. doi: 10.1530/acta.0.0690775
8. Nappi RE, Petraglia F, Luisi S, Polatti F, Farina C, Genazzani AR. Serum allopregnanolone in women with postpartum “blues. Obstet Gynecol. (2001) 97:77–80. doi: 10.1016/s0029-7844(00)01112-1
9. Noecker C, Sanchez J, Bisanz JE, Escalante V, Alexander M, Trepka K, et al. Systems biology elucidates the distinctive metabolic niche filled by the human gut microbe Eggerthella lenta. PloS Biol. (2023) 21:e3002125. doi: 10.1371/journal.pbio.3002125
10. Carbonero F, Benefiel AC, Gaskins HR. Contributions of the microbial hydrogen economy to colonic homeostasis. Nature reviews. Gastroenterol Hepatol. (2012) 9:504–18. doi: 10.1038/nrgastro.2012.85
11. McDowall JS, Murphy BJ, Haumann M, Palmer T, Armstrong FA, Sargent F. Bacterial formate hydrogenlyase complex. Proc Natl Acad Sci USA. (2014) 111:E3948–3956. doi: 10.1073/pnas.1407927111
12. Wolf PG, Biswas A, Morales SE, Greening C, Gaskins HR. H2 metabolism is widespread and diverse among human colonic microbes. Gut Microbes. (2016) 7:235–45. doi: 10.1080/19490976.2016.1182288
13. Harris SC, Devendran S, Méndez-García C, Mythen SM, Wright CL, Fields CJ, et al. Bile acid oxidation by Eggerthella lenta strains C592 and DSM 2243(T). Gut Microbes. (2018) 9:523–39. doi: 10.1080/19490976.2018.1458180
14. Seo T, Kurokawa R, Sato B. A convenient method for determining the concentration of hydrogen in water: use of methylene blue with colloidal platinum. Med Gas Res. (2012) 2:1. doi: 10.1186/2045-9912-2-1
15. Zhang Y, Long Z, Xu J, Tan S, Zhang N, Li A, et al. Hydrogen inhibits isoproterenol−induced autophagy in cardiomyocytes in vitro and in vivo. Mol Med Rep. (2017) 16:8253–8. doi: 10.3892/mmr.2017.7601
16. Liang B, Shi L, Du D, Li H, Yi N, Xi Y, et al. Hydrogen-rich water ameliorates metabolic disorder via modifying gut microbiota in impaired fasting glucose patients: A randomized controlled study. Antioxidants (Basel). (2023) 12:1245. doi: 10.3390/antiox12061245
17. Song Y, Zhang R, Qin H, Xu W, Sun J, Jiang J, et al. Micromotor-enabled active hydrogen and tobramycin delivery for synergistic sepsis therapy. Adv Sci (Weinh). (2023) 10:e2303759. doi: 10.1002/advs.202303759
18. Maguire J, Mody I. GABA(A)R plasticity during pregnancy: relevance to postpartum depression. Neuron. (2008) 59:207–13. doi: 10.1016/j.neuron.2008.06.019
19. Mody I. GABA(A)R modulator for postpartum depression. Cell. (2019) 176:1. doi: 10.1016/j.cell.2018.12.016
20. Nemeroff CB. Understanding the pathophysiology of postpartum depression: implications for the development of novel treatments. Neuron. (2008) 59:185–6. doi: 10.1016/j.neuron.2008.07.015
21. Wisner KL, Stika CS, Ciolino JD. The first food and drug administration-indicated drug for postpartum depression-brexanolone. JAMA Psychiatry. (2019) 76:1001–2. doi: 10.1001/jamapsychiatry.2019.1546
22. Zhang Y, Su WJ, Chen Y, Wu TY, Gong H, Shen XL, et al. Effects of hydrogen-rich water on depressive-like behavior in mice. Sci Rep. (2016) 6:23742. doi: 10.1038/srep23742
23. Zhang Y, Liu H, Xu J, Zheng S, Zhou L. Hydrogen gas: A novel type of antioxidant in modulating sexual organs homeostasis. Oxid Med Cell Longev. (2021) 2021:8844346. doi: 10.1155/2021/8844346
24. Fan M, Xu X, He X, Chen L, Qian L, Liu J, et al. Protective effects of hydrogen-rich saline against erectile dysfunction in a streptozotocin induced diabetic rat model. J Urol. (2013) 190:350–6. doi: 10.1016/j.juro.2012.12.001
25. Makav M, Kuru M, Aras Ş Y, Sarı EK, Bulut M, Alwazeer D. The effect of hydrogen-rich water on letrozole-induced polycystic ovary syndrome in rats. Reprod BioMed Online. (2023) 47:103332. doi: 10.1016/j.rbmo.2023.103332
26. Meng X, Chen H, Wang G, Yu Y, Xie K. Hydrogen-rich saline attenuates chemotherapy-induced ovarian injury via regulation of oxidative stress. Exp Ther Med. (2015) 10:2277–82. doi: 10.3892/etm.2015.2787
27. Nakano T, Kotani T, Imai K, Iitani Y, Ushida T, Tsuda H, et al. Effect of molecular hydrogen on uterine inflammation during preterm labour. BioMed Rep. (2018) 8:454–60. doi: 10.3892/br.2018.1082
28. Geng N, Gao X, Wang X, Cui S, Wang J, Liu Y, et al. Hydrogen helps to ameliorate Staphylococcus aureus-induced mastitis in mice. Int Immunopharmacol. (2022) 109:108940. doi: 10.1016/j.intimp.2022.108940
29. Frajese GV, Benvenuto M, Mattera R, Giampaoli S, Ambrosin E, Bernardini R, et al. Electrochemically reduced water delays mammary tumors growth in mice and inhibits breast cancer cells survival in vitro. Evid Based Complement Alternat Med. (2018) 2018:4753507. doi: 10.1155/2018/4753507
30. Zhang C, Zheng DW, Li CX, Zou MZ, Yu WY, Liu MD, et al. Hydrogen gas improves photothermal therapy of tumor and restrains the relapse of distant dormant tumor. Biomaterials. (2019) 223:119472. doi: 10.1016/j.biomaterials.2019.119472
31. Wu G, Pan L, Sun J, Chen G, Wang S. Hydrogen gas protects against ovariectomy-induced osteoporosis by inhibiting NF-kappaB activation. Menopause. (2019) 26:785–92. doi: 10.1097/GME.0000000000001310
32. Guan P, Lin XM, Yang SC, Guo YJ, Li WY, Zhao YS, et al. Hydrogen gas reduces chronic intermittent hypoxia-induced hypertension by inhibiting sympathetic nerve activity and increasing vasodilator responses via the antioxidation. J Cell Biochem. (2019) 120:3998–4008. doi: 10.1002/jcb.v120.3
33. Zheng H, Yu YS. Chronic hydrogen-rich saline treatment attenuates vascular dysfunction in spontaneous hypertensive rats. Biochem Pharmacol. (2012) 83:1269–77. doi: 10.1016/j.bcp.2012.01.031
Keywords: hydrogen gas, 21-dehydroxylation, women health, glucocorticoids, progestins
Citation: Yang S, Zhang J, Xu L, Guan Y, Fang C, Zheng S, Yang H, Liu H and Zhang Y (2025) The endogenous hydrogen gas (H2) drives women’s health: a comment on “Gut bacteria convert glucocorticoids into progestins in the presence of hydrogen gas”. Front. Endocrinol. 15:1504814. doi: 10.3389/fendo.2024.1504814
Received: 01 October 2024; Accepted: 30 December 2024;
Published: 05 February 2025.
Edited by:
Jing Xu, Liberty University, United StatesReviewed by:
Tsuyoshi Baba, Sapporo Medical University, JapanCopyright © 2025 Yang, Zhang, Xu, Guan, Fang, Zheng, Yang, Liu and Zhang. This is an open-access article distributed under the terms of the Creative Commons Attribution License (CC BY). The use, distribution or reproduction in other forums is permitted, provided the original author(s) and the copyright owner(s) are credited and that the original publication in this journal is cited, in accordance with accepted academic practice. No use, distribution or reproduction is permitted which does not comply with these terms.
*Correspondence: Yaxing Zhang, emhhbmd5YXhpbmdAZ3p1Y20uZWR1LmNu; Haimei Liu, bGhtZWk5OUBnenVjbS5lZHUuY24=
†These authors have contributed equally to this work
‡Lead Contact