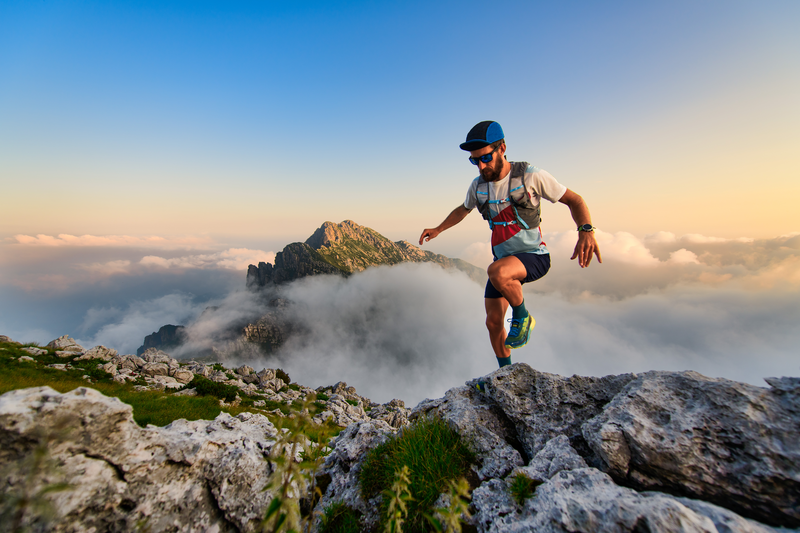
95% of researchers rate our articles as excellent or good
Learn more about the work of our research integrity team to safeguard the quality of each article we publish.
Find out more
ORIGINAL RESEARCH article
Front. Endocrinol. , 24 January 2025
Sec. Pediatric Endocrinology
Volume 15 - 2024 | https://doi.org/10.3389/fendo.2024.1496155
This article is part of the Research Topic Insights in Pediatric Endocrinology: 2024 View all 18 articles
Introduction: Studies assessing longitudinal changes in the prevalence of autoimmune thyroiditis (AIT) among the pediatric population are limited. During the COVID-19 era, several papers proposed a rise in AIT cases. Our study aimed to analyze the prevalence of thyroid autoimmunity (TA) over a 10-year period spanning pre-pandemic and pandemic years in a population who are regularly screened for thyroid disturbances.
Materials and methods: This single-center retrospective cohort study analyzed data from 1,361 children and young adults with type 1 diabetes (T1D) treated between 2013 and 2022 in Hungary’s largest pediatric endocrinology center. Results of anti-thyroid autoantibodies (anti-thyroid peroxidase/ATPO/and antithyroglobulin/ATG/), thyroid function tests (TFTs) and thyroid ultrasound examinations were obtained. Annual prevalence rates of TA and ultrasound-proven thyroiditis were calculated. Mean (± SD) follow-up period was 4.7 (± 2.8) years.
Results: The overall prevalence of TA among our T1D children was 22.8% ([20.3;25.5], 310 cases) with significantly more girls affected (p<0.001). From 2013 to 2022, TA prevalence rose from 15.9% to 20.6% (p=0.041). The increase was detected during the pre-pandemic years but not in the COVID-19 era. Ultrasound-confirmed thyroiditis was present in 80.0% of examined TA cases. Ultrasound positivity rate was stable during the study period. Among our children with TA, 28.5% exhibited clinically relevant thyroid-stimulating hormone (TSH) abnormalities (most commonly subclinical hypothyroidism) and/or were prescribed thyroid medication. Children with AIT had a significantly elevated risk of thyroid dysfunction compared to those with only thyroid autoantibody positivity (p<0.001).
Conclusion: Our results show a rise in the prevalence of thyroid autoimmunity among T1D children over the past decade, but our data do not support the assumed role of SARS-CoV-2 in the development of the disease.
During the coronavirus disease 2019 (COVID-19) pandemic era, numerous studies explored a potential connection between the Severe acute respiratory syndrome coronavirus 2 (SARS-CoV-2) and different autoimmune diseases. The majority of large clinical studies and meta-analyses reported an increase in the incidence or an acceleration in the progression of type 1 diabetes (T1D) during the pandemic and particularly, following SARS-CoV-2 infection (1–4). However, some studies presented opposing results or found that the rise during the pandemic was within the range of the expected incidence (5–7). Similarly, while several studies indicated a rise in thyroid autoimmunity (TA, presence of anti-thyroid autoantibodies) and autoimmune thyroiditis (AIT, positive autoantibodies with inflammation of the thyroid gland as proven by ultrasound) during the pandemic, evidence remains controversial (8–12). Two studies specifically investigated the prevalence of TA in newly diagnosed T1D children, however, they also reported contradictory findings (13, 14). Our research group has also examined the prevalence of thyroid disturbances among the pediatric population post-COVID-19 but we - like many other scientists - faced a major limitation: the scarcity of pre-pandemic control data (15).
Type 1 diabetes (T1D) is the most common childhood endocrine disease. It is well-established that individuals with T1D have an elevated risk of developing other autoimmune conditions, such as AIT. According to the literature, the prevalence of AIT in children with T1D ranges from 17 to 30%, highlighting the strong association between these two autoimmune disorders (16).
As pediatric patients with T1D are at higher risk of developing AIT [most commonly Hashimoto’s disease (17)], they undergo regular screening for TA based on the recommendation of the International Society for Pediatric and Adolescent Diabetes’ [ISPAD, most recently updated in 2022 (18)]. To better understand the impact of the COVID-19 pandemic, we aimed to collect and analyze longitudinal data on AIT and TA in our pediatric T1D population.
The primary purpose of our study was to determine the annual prevalence of TA and ultrasound-proven thyroiditis among pediatric patients with T1D treated in one of Central Europe’s largest pediatric endocrinology-diabetology centers over a 10-year period, covering both pre-pandemic (2013-2020) and pandemic (2021-2022) years. Additionally, we aimed to assess longitudinal trends in anti-thyroid peroxidase (ATPO) and antithyroglobulin (ATG) antibody titers along with thyroid function test (TFTs) results and data on thyroid medication during this period.
Our single-center retrospective cohort study was conducted at the Bókay Unit of the Pediatric Center, Semmelweis University, Budapest, Hungary. This center is responsible for the care of approximately 25% of all T1D children and adolescents in the country. Before exclusion, we obtained all patients’ data with diabetes mellitus (DM) who received insulin treatment and had at least one visit between 1st of January, 2013 and 31st of December, 2022 at the inpatient and/or outpatient Endocrinology and Diabetes Unit of our Center. Clinical data and laboratory results were obtained from Semmelweis University’s e-MedSolution software. Medical records were retrieved based on the BNO classification system, the Hungarian adaptation of the ICD-10 (The International Statistical Classification of Diseases and Related Health Problems, 10th Revision) classification released by the World Health Organization (https://icd.who.int/browse10/2019/en, accessed: 09.10.2024).
Data was completed and verified by four medical doctors and two medical students in agreement with our pre-specified research protocol. All arising questions were discussed in detail within the data extraction team.
Our study was conducted in accordance with the World Medical Association’s Declaration of Helsinki. Due to the retrospective nature of our study, ethical approval was not required.
Inclusion criteria: all patients between 0 to 21 years of age (hereafter referred to collectively as “children”), who had been treated with T1D at our center between 2013 and 2022, and had at least one simultaneous ATPO and ATG measurements during our study period. For the purposes of this study, we have chosen 21 years as the upper age limit, consistently with our clinical practice, where care is provided to individuals up to 21 years of age. Data on TA were obtained from both newly diagnosed and follow-up cases.
Exclusion criteria: patients over 21 years of age, children with diabetes forms other than T1D, and those who never underwent TA screening throughout the duration of our study.
We collected the following parameters: clinical data of children (sex, date of birth, T1D diagnosis date), visit dates, thyroid autoantibody (ATPO, ATG) and TFTs (thyroid-stimulating hormone/TSH/, free thyroxine/fT4/) results from all years when the patient was screened as well as thyroid ultrasound findings for antibody-positive patients. Mean ages and time since T1D diagnosis were calculated according to the mid-point of each calendar year (1st of July). Laboratory analysis were carried out at the Immunology Laboratory of Semmelweis University with assay methodology and normal ranges shown in Table 1. If a child had more than one laboratory examination of a parameter in a calendar year, we used the last data for our analysis (or the last one before they started taking thyroid medicines in case of TSH and fT4). TSH levels above 6 mU/L or below 0.1 mU/L, and/or results that were consistently abnormal in a patient, were considered clinically relevant. TA was defined by at least one positive ATPO and/or ATG laboratory result obtained during our study period. Assay methodology and ranges of normal values of autoantibodies had changed multiple times over the examined period, therefore we calculated both absolute and relative values. Thyroid ultrasounds were deemed positive for thyroiditis if they showed enlargement and/or inhomogeneity and/or hyperemia in the thyroid gland. AIT was diagnosed if at least one autoantibody was positive and thyroiditis was confirmed by ultrasound examination.
Screening frequency of children was primarily based on ISPAD’s guidelines (18), therefore patients without thyroid comorbidity did not go through autoantibody measurements each year. However, if a patient had presented a positive thyroid autoantibody result, they had been examined in all years afterwards. Due to the different screening frequencies between positive and negative children, prevalence data would be unrealistically high if not adjusted. Thus, in years when no laboratory examinations were carried out, we used the following protocol:
If the antibody titers were negative in both the preceding and subsequent years’, we considered the intermediate year to be negative as well. When both were positive, we recognized it as positive. If they differed, the intermediate year was administered as missing data.
We defined the pre-pandemic period as 2013 to 2020 and the pandemic period as 2021 and 2022. In the year of 2020, we did not expect a significant impact of the pandemic on our children, as a large Hungarian national representative study indicated that there were very few pediatric COVID-19 cases during the first pandemic wave (19).
Statistical analysis was carried out by a professional biostatistician. Each year, we calculated the prevalence using data from all children under care at that time. Consequently, the data for most children are included in our database for multiple years, corresponding to each year they were under care at our center. To calculate and compare prevalence data, and perform descriptive statistics, MedCalc Statistical Software version 22.023 was used (MedCalc Software bv, Ostend, Belgium; https://www.medcalc.org; 2020). Chi square comparison were conducted using StataCorp. (Stata Statistical Software: Release 18.5). The visualization was created using Microsoft Excel (Microsoft Corporation, 2016).
Between January 1, 2013 and December 31, 2022, a total of 1,667 patients with insulin-dependent DM were treated at our Clinic. We excluded 14 adults over 21 years old, 65 children who had another condition rather than T1D and 227 patients due to missing data and/or who were treated primarily at other centers. All in all, we included 1,361 T1D children’s data in our final analysis. Mean (± SD) follow-up period was 4.7 (± 2.8) years. The girl-to-boy ratio was 637:724 (46.8% vs 53.2%). Detailed annual data are provided in Table 2.
The overall prevalence of thyroid autoimmunity among our T1D children was found to be 22.8% ([20.3;25.5], 310 children). Girls were significantly more affected. (TA group: 106 boys, 204 girls, non-TA group: 618 boys, 433 girls; p<0.001, RR: 1.60 [1.43;1.78]).
From 2013 to 2022, the prevalence of TA increased from 15.9% to 20.6% (p=0.041). We observed this increase during the pre-pandemic years (2013 to 2019) but not in the COVID-19 era. Whilst analyzing the yearly prevalence differences, we found no significant difference between any consecutive years. Annual prevalence rates with confidence intervals (CI) are shown in Figure 1.
Of the children with TA who were screened with ultrasound at least once (n=260), 208 (80.0%) showed signs of thyroiditis on ultrasound, representing 67.1% of all children with TA. This resulted in an overall AIT prevalence of 15.3% in our study group. Among the 208 children with ultrasound-proven thyroiditis, one was later diagnosed with papillary thyroid carcinoma, as was one other child who did not show signs of thyroiditis. Although we performed an increasing number of ultrasounds throughout the years, the positivity rate remained stable. Yearly ATPO, ATG and ultrasound positivity rates can be seen in Table 3.
Given the multiple changes in assay methodology of ATPO and ATG titers during our examined period, we calculated both absolute and relative values in case of both antibodies. Detailed results of autoantibody titers are provided in Supplementary Tables 1, 2.
We also analyzed the rate of thyroid hormone changes and data related to thyroid medication over this 10-year period in our children with TA. From the TFTs’ analysis, we excluded children who did not have at least one TSH result during the study period (n=8). For children who were prescribed thyroid medication, we included only data preceding the initiation of treatment. Therefore, children who started their medication before 2013 (the beginning of our study period) were also excluded (n=20). Among the included 282 children, 44 (15.6%) exhibited clinically relevant elevation in TSH levels, while eight (2.8%) showed TSH reduction during the study period. Increased fT4 levels were observed in four cases, while decreased fT4 levels were noted in two children. The annual results are presented in Table 4. Detailed description of TSH and fT4 values can be seen in Supplementary Table 3.
Among the 310 children with TA, 74 (23.9%) were treated for hypo- and/or hyperthyroidism. Of these, 63 were initially prescribed levothyroxine to manage subclinical or clinical hypothyroidism, while two required levothyroxine following a total thyroidectomy due to thyroid carcinoma. Additionally, nine children were originally treated with antithyroid (thyrostatic) medications (thiamazole or propylthiouracil). During our study period, three children required treatment with both types of medication. Two of them began with thiamazole and later transitioned to levothyroxine due to hypothyroidism, while one child started on levothyroxine and subsequently began taking thiamazole.
Altogether, out of the 302 children with TA who had at least one TSH result during the study period, 86 (28.5%) had clinically relevant TSH abnormalities and/or were prescribed thyroid medication.
We examined the connection between ultrasound positivity and thyroid function abnormalities among the children with at least one ultrasound and one TSH result (n=258). From this analysis, we excluded the two aforementioned children with papillary thyroid carcinoma, as they had started hormone replacement therapy following total thyroidectomy. The contingency table summarizing these data is presented as Table 5. Children with ultrasound-proven thyroiditis had a significantly elevated risk of having TSH abnormalities and/or being prescribed thyroid medication (p<0.001, RR: 6.24 [2.05;18.98]).
Table 5. Association between ultrasound positivity (thyroiditis) and thyroid function abnormalities.
In our retrospective cohort study involving 1,361 children and young adults with T1D, we found an increase in the prevalence of TA from 15.9% to 20.6% between 2013 and 2022. Interestingly, this increase was notable in the pre-COVID period (2013-2020) but not during the pandemic years (2021-2022). While the study’s primary aim was to explore the potential effect of the COVID-19 pandemic on the prevalence of TA among T1D children, our results revealed a previously underreported trend: the rise in TA prior to the pandemic.
Numerous data shows that the prevalence rates of autoimmune diseases are increasing (20–22). A population-based cohort study of 22 million individuals in the UK found the largest incidence rate increase in coeliac disease, Sjogren’s syndrome and Graves’ disease, while Hashimoto’s thyroiditis significantly decreased in incidence from 2000 to 2019 (23).
Studies involving the frequency of TA in pediatric T1D populations, mostly report on pooled prevalence data (17, 24). Cumulative incidence rate of TA was reported in children with T1D in a recent French study covering a similar study period like ours (2014 to 2020). They found an overall thyroid autoantibody frequency of 18% (25). The cumulative incidence of TA at 10 years of T1D was found to be 14% in a former study (26). However, longitudinal data on the trend of TA prevalence in the pediatric T1D population is scarce.
High prevalence of autoimmune thyroid diseases (AITDs) has been linked to various genetic, epigenetic, and environmental factors, though evidence on their specific effects remains inconsistent. Altered DNA methylation patterns, which regulate gene expression without altering the DNA sequence, may play a role in AITD development (27). Some studies have identified a relationship between vitamin D deficiency or insufficiency and AITDs (28, 29), while others found no such connection (30, 31). Additionally, insufficient selenium intake has been implicated in Hashimoto’s disease occurrence (32). Higher iodine consumption has also been associated with an increased presence of anti-thyroid antibodies (33, 34). Stress-related disorders significantly elevated the risk of subsequent AITD, with a hazard ratio of 1.49 (1.42–1.56) observed in a large Swedish cohort study (35). However, the prospective Amsterdam AITD Cohort study found no link between stress exposure and de novo appearance of ATPO (36). Furthermore, an increasing number of studies are investigating the role of endocrine-disrupting chemicals (EDCs) in the onset and severity of AITDs, though research in this area remains limited (37). Among infectious agents, besides SARS-CoV-2, Epstein–Barr virus, Parvovirus B19, Human Herpesvirus 6A and Helicobacter pylori have all been suggested as contributors to AITD development (38–41).
Our hypothesis was that due to the high pediatric SARS-CoV-2 infection rate, we would observe an even higher TA prevalence among T1D children during the pandemic period. Our result do not support this initial hypothesis and it should be added, that even if we would have found an increasing prevalence during the pandemic period, our study design would only allow us to describe an association but not to state a cause-effect relationship. With the analysis of the pre-pandemic and pandemic era, we only had the opportunity to investigate the hypothesized change of the cumulative effect of numerous possible influencing factors (impact of SARS-CoV-2 and COVID-19 vaccines, delays in diagnosis making, changes in numerous habits such as eating, taking supplements, physical activity, decrease of other viral infections due to the restrictive measures and also other aspects which are not related to the pandemic and were discussed earlier).
To the best of our knowledge, only a few studies compared TA prevalence among T1D children between pre-pandemic and pandemic era with only one reporting annual data as well (14). Our results are in line with a small Turkish study, which found no significant difference in the frequency of concurrent thyroid autoantibodies (neither in ATPO nor in ATG) in newly diagnosed T1D children during the first pandemic year (February 2020 - January 2021) in comparison to the previous three years (February 2017 - January 2020) (13). In contrast, Al-Abdulrazzaq et al. published opposing results from Kuwait: children diagnosed with T1D during the COVID-19 pandemic (February 2020 - December 2022) had double the odds of testing positive for thyroid antibodies compared to those diagnosed earlier (January 2017 - February 2020). Additionally, according to their results, children with a positive history of COVID-19 were more likely to present with thyroid antibodies. In contrast to our finding of an increasing trend during the pre-pandemic years, they documented a stable prevalence between 2017 and 2019 (14).
Several studies reported an increased rate of TA during and after COVID-19 in adult, non-T1D populations. Increased prevalence of latent TA (ATPO positivity) was described in patients hospitalized for COVID-19 compared to healthy, pre-pandemic controls (8). Rossini et al. described a doubled prevalence of autoimmune thyroid disease (increased ATPO) in COVID-19 survivor adults compared to age and sex-matched controls (15.7% vs 7.7%) (9). An observational study from Spain documented a doubled diagnosis rate of Graves’ disease in 2021, compared to data from 2017-2020 (10). In contrast, Lui et al. found only incidental TA positivity among adults recovering from COVID-19 (11). Moreover, a retrospective cohort study of pregnant women showed no significant difference in the incidence of new-onset thyroid dysfunction or autoimmunity between those who were SARS-CoV-2 seropositive and those who were seronegative postpartum (12). In a recent comprehensive review on the topic, the authors concluded that although SARS-CoV-2 can affect the thyroid gland and possibly cause subacute thyroiditis or TA, according to follow-up studies, survivors of COVID-19 showed no substantial long-term thyroid sequelae (42).
This issue is much less discussed in the pediatric literature than in adult studies. According to a recent narrative review focusing on the impact of the COVID-19 pandemic on thyroid diseases in the general pediatric population, there is currently no conclusive evidence linking SARS-CoV-2 with an increased incidence of TA in children. Additionally, they highlighted the paucity of data on the youth (43). A retrospective analysis by Shidid et al. observed no significant differences in the percentage of abnormal TSH results between the pre-pandemic (January 2017–October 2019) and pandemic periods (March 2020–October 2021). They focused on thyroid dysfunction without reporting on the frequency of TA (44). Consistently, a retrospective observational study from a tertiary pediatric endocrine center in the United Kingdom found no substantial changes in the presentation of thyroid dysfunction (hypo- and hyperthyroidism) in children before and after the pandemic. McCowan et al. also highlighted that the COVID-19 pandemic had no significant effect on fT4 or ATPO in hypothyroid patients (45). It is evident that there is a need for more pediatric-specific research.
Recent studies suggest that COVID-19-related thyroid dysfunction may occur through multiple mechanisms. SARS-CoV-2 can potentially disrupt thyroid function either by directly damaging thyroid cells via angiotensin-converting enzyme 2 (ACE-2) receptors or through indirect effects, such as triggering or exacerbating autoimmune processes (46–48). The virus may trigger autoimmune responses through various mechanisms, including molecular mimicry of SARS-CoV-2 proteins, bystander activation, epitope spreading during tissue damage, disruption of immunoprivileged barriers, polyclonal lymphocyte activation, and pathological activation of antigen-presenting cells via superantigens (48).
Our results support the findings of numerous previous large studies reporting female predominance among T1D children with TA (14, 17, 21, 24, 26). Additionally, older age and longer diabetes duration might increase the risk of developing TA (14, 24). In our study, the mean of these parameters remained stable during our study period so these factors did not bias our results.
Though the ISPAD protocol does not require ultrasound examinations for diagnosis and monitoring TA, we are proud that the number of performed ultrasound examinations grew remarkably during our study period and we could cover the vast majority of children among our TA population by 2022. It should be noted, that the positivity rate of ultrasound examinations was stable throughout the whole study period. We find this diagnostic tool inevitable in the early detection of malignancies.
Furthermore, we aimed to examine the differences between AIT and TA. While AIT specifically refers to an autoimmune condition in which the thyroid gland shows evidence of inflammation, TA is a broader term that encompasses the presence of thyroid-specific autoantibodies without necessarily having inflammation detectable by ultrasound. We found that children with inflammation of the thyroid gland (AIT) had a significantly greater likelihood of thyroid function abnormalities and subsequent thyroid medication use compared to those with only thyroid-specific autoantibodies and negative ultrasound results (TA). However, it is noteworthy that three children exhibited TSH alterations despite having no signs of thyroiditis on their ultrasound examinations.
The main strength of our study is that it covers ten years of annual data on more than 1,300 children allowing us to assess the prevalence of TA longitudinally. The decade-long study period enables for a comprehensive assessment of trends in TA over time. By analyzing TA prevalence on a year-by-year basis, the study provides a more nuanced understanding of the trends compared to pooled data analysis. It has the ability to reflect on the increasing trend during a long-term period, while with the comparison of pre-pandemic and pandemic groups, one might assume that the difference between the pooled prevalences could be attributed to the impact of the pandemic. The inclusion of both pre-pandemic and pandemic years adds an important dimension to the understanding of the trends before and during the COVID-19 pandemic. Additionally, given that our center is responsible for the care of about 25% of all T1D children in Hungary and is one of the largest in Central Europe, we believe that our findings are representative of this region.
As this was a retrospective study, we encountered instances of incomplete or missing data in certain cases. Possible reasons for these were missed annual check-ups by some patients, variations in protocols among specialists, overlooked screenings or insufficient blood sample volumes. Furthermore, the documentation regarding medication may have been inconsistent. Moreover, the laboratory test method used for the measurement of ATPO and ATG changed multiple times during our study period, thus we needed to present relative values of ATPO and ATG besides absolute ones. Unfortunately, as to our experiences, neither of them is ideal for precise comparison, nevertheless we indicated both results. Finally, it should be noted, that autoimmune diseases may develop months or years after an infection, so our study (ending in 2022) might not capture the long-term effects of the pandemic.
In our retrospective cohort study, we found an increase in the prevalence of thyroid autoimmunity among children with type 1 diabetes from 2013 to 2022. Unexpectedly, this growth was remarkable during the studied pre-pandemic period and it just stopped during the COVID-19 pandemic years. Therefore, our results among T1D children do not support the assumed role of SARS-CoV-2 in the development of thyroid autoimmunity.
The raw data supporting the conclusions of this article will be made available by the authors, without undue reservation.
The requirement of ethical approval was waived by Prof. András Arató, Pediatric Center, Semmelweis University for the studies involving humans. Due to the retrospective nature of our study, ethical approval was not required. The studies were conducted in accordance with the local legislation and institutional requirements. The ethics committee/institutional review board also waived the requirement of written informed consent for participation from the participants or the participants’ legal guardians/next of kin because Due to the retrospective nature of our study and unidentifiable patients, written informed consent was not required.
VH: Conceptualization, Data curation, Formal analysis, Funding acquisition, Investigation, Methodology, Project administration, Resources, Software, Supervision, Validation, Visualization, Writing – original draft, Writing – review & editing. EM: Conceptualization, Investigation, Methodology, Project administration, Writing – original draft, Writing – review & editing. DC: Data curation, Formal analysis, Investigation, Project administration, Visualization, Writing – original draft, Writing – review & editing. LT: Data curation, Formal analysis, Investigation, Visualization, Writing – original draft, Writing – review & editing. JT: Conceptualization, Data curation, Formal analysis, Software, Supervision, Validation, Visualization, Writing – original draft, Writing – review & editing. RG: Conceptualization, Data curation, Formal analysis, Investigation, Writing – original draft, Writing – review & editing. FK: Data curation, Investigation, Project administration, Visualization, Writing – original draft, Writing – review & editing. AL: Data curation, Formal analysis, Investigation, Methodology, Supervision, Writing – original draft, Writing – review & editing. AK: Conceptualization, Investigation, Supervision, Writing – original draft, Writing – review & editing. PT: Conceptualization, Data curation, Formal analysis, Funding acquisition, Investigation, Methodology, Project administration, Resources, Software, Supervision, Validation, Visualization, Writing – original draft, Writing – review & editing.
The author(s) declare financial support was received for the research, authorship, and/or publication of this article. This work was supported by the EKÖP-2024-144 New National Excellence Program of the Ministry of Culture and Innovation from the source of the National Research, Development and Innovation Fund. The sponsor had no role in the study design, data collection, analysis, interpretation, writing of the report and decision to submit the paper for publication.
We would like to acknowledge the help of our colleagues: László Madácsy, MD, DSc; László Derzbach, MD, PhD; Erzsébet Hrapka, MD; Eszter Világos, MD; Dániel Sándor Veres, MD, PhD.
The authors declare that the research was conducted in the absence of any commercial or financial relationships that could be construed as a potential conflict of interest.
All claims expressed in this article are solely those of the authors and do not necessarily represent those of their affiliated organizations, or those of the publisher, the editors and the reviewers. Any product that may be evaluated in this article, or claim that may be made by its manufacturer, is not guaranteed or endorsed by the publisher.
The Supplementary Material for this article can be found online at: https://www.frontiersin.org/articles/10.3389/fendo.2024.1496155/full#supplementary-material
1. Barrett CE, Koyama AK, Alvarez P, Chow W, Lundeen EA, Perrine CG, et al. Risk for newly diagnosed diabetes >30 days after SARS-CoV-2 infection among persons aged <18 years - United States, March 1, 2020-June 28, 2021. MMWR Morb Mortal Wkly Rep. (2022) 71:59–65. doi: 10.15585/mmwr.mm7102e2
2. Zhang T, Mei Q, Zhang Z, Walline JH, Liu Y, Zhu H, et al. Risk for newly diagnosed diabetes after COVID-19: a systematic review and meta-analysis. BMC Med. (2022) 20:444. doi: 10.1186/s12916-022-02656-y
3. Wise J. Covid-19: Progression to clinical type 1 diabetes accelerated after infection, study suggests. BMJ. (2024) 386:q1557. doi: 10.1136/bmj.q1557
4. D’Souza D, Empringham J, Pechlivanoglou P, Uleryk EM, Cohen E, Shulman R. Incidence of diabetes in children and adolescents during the COVID-19 pandemic: A systematic review and meta-analysis. JAMA Netw Open. (2023) 6:e2321281. doi: 10.1001/jamanetworkopen.2023.21281
5. Rathmann W, Kuss O, Kostev K. Incidence of newly diagnosed diabetes after Covid-19. Diabetologia. (2022) 65:949–54. doi: 10.1007/s00125-022-05670-0
6. Reschke F, Lanzinger S, Herczeg V, Prahalad P, Schiaffini R, Mul D, et al. The COVID-19 pandemic affects seasonality, with increasing cases of new-onset type 1 diabetes in children, from the worldwide SWEET registry. Diabetes Care. (2022) 45:2594–601. doi: 10.2337/dc22-0278
7. Shulman R, Cohen E, Stukel TA, Diong C, Guttmann A. Examination of trends in diabetes incidence among children during the COVID-19 pandemic in Ontario, Canada, from March 2020 to September 2021. JAMA Netw Open. (2022) 5:e2223394. doi: 10.1001/jamanetworkopen.2022.23394
8. Anaya JM, Monsalve DM, Rojas M, Rodríguez Y, Montoya-García N, Mancera-Navarro LM, et al. Latent rheumatic, thyroid and phospholipid autoimmunity in hospitalized patients with COVID-19. J Transl Autoimmun. (2021) 4:100091. doi: 10.1016/j.jtauto.2021.100091
9. Rossini A, Cassibba S, Perticone F, Benatti SV, Venturelli S, Carioli G, et al. Increased prevalence of autoimmune thyroid disease after COVID-19: A single-center, prospective study. Front Endocrinol (Lausanne). (2023) 14:1126683. doi: 10.3389/fendo.2023.1126683
10. Barajas Galindo DE, Ramos Bachiller B, González Roza L, García Ruiz de Morales JM, Sánchez Lasheras F, González Arnáiz E, et al. Increased incidence of Graves’ disease during the SARS-CoV2 pandemic. Clin Endocrinol (Oxf). (2023) 98:730–7. doi: 10.1111/cen.14860
11. Lui DTW, Tsoi KH, Lee CH, Cheung CYY, Fong CHY, Lee ACH, et al. A prospective follow-up on thyroid function, thyroid autoimmunity and long COVID among 250 COVID-19 survivors. Endocrine. (2023) 80:380–91. doi: 10.1007/s12020-022-03281-8
12. Goyal A, Gupta Y, Kalaivani M, Bhatla N, Tandon N. Impact of SARS-CoV-2 on progression of glycemic and cardiometabolic variables and changes in insulin indices: A longitudinal study. Diabetes Ther. (2021) 12:3011–23. doi: 10.1007/s13300-021-01158-z
13. Kaya G, Cimbek EA, Yeşilbaş O, Bostan YE, Karagüzel G. A long-term comparison of presenting characteristics of children with newly diagnosed type 1 diabetes before and during the COVID-19 pandemic. J Clin Res Pediatr Endocrinol. (2022) 14:267–74. doi: 10.4274/jcrpe.galenos.2022.2021-10-2
14. Al-Abdulrazzaq D, Albatineh AN, Khalifa D, Alrefae A, Al-Awadhi E, Alkandari A, et al. Prevalence and factors associated with thyroid autoimmunity among children newly diagnosed with type 1 diabetes before and during the COVID-19 pandemic: Evidence from Kuwait. Diabetes Metab Res Rev. (2024) 40:e3824. doi: 10.1002/dmrr.3824
15. Herczeg V, Garai R, Takács J, Kovács F, Luczay A, Hrapka E, et al. Thyroid disturbances after COVID-19 and the effect of vaccination in children: a prospective tri-center registry analysis. Eur J Pediatrics. (2023) 182:4443–55. doi: 10.1007/s00431-023-05097-8
16. American Diabetes Association Professional Practice Committee. 14. Children and adolescents: standards of medical care in diabetes-2022. Diabetes Care. (2022) 45:208–31. doi: 10.2337/dc22-S014
17. Hughes JW, Riddlesworth TD, DiMeglio LA, Miller KM, Rickels MR, McGill JB. Autoimmune diseases in children and adults with type 1 diabetes from the T1D exchange clinic registry. J Clin Endocrinol Metab. (2016) 101:4931–7. doi: 10.1210/jc.2016-2478
18. Fröhlich-Reiterer E, Elbarbary NS, Simmons K, Buckingham B, Humayun KN, Johannsen J, et al. ISPAD Clinical Practice Consensus Guidelines 2022: Other complications and associated conditions in children and adolescents with type 1 diabetes. Pediatr Diabetes. (2022) 23:1451–67. doi: 10.1111/pedi.13445
19. Merkely B, Szabó AJ, Kosztin A, Berényi E, Sebestyén A, Lengyel C, et al. Novel coronavirus epidemic in the Hungarian population, a cross-sectional nationwide survey to support the exit policy in Hungary. Geroscience. (2020) 42:1063–74. doi: 10.1007/s11357-020-00226-9
20. Miller FW. The increasing prevalence of autoimmunity and autoimmune diseases: an urgent call to action for improved understanding, diagnosis, treatment, and prevention. Curr Opin Immunol. (2023) 80:102266. doi: 10.1016/j.coi.2022.102266
21. McLeod DS, Cooper DS. The incidence and prevalence of thyroid autoimmunity. Endocrine. (2012) 42:252–65. doi: 10.1007/s12020-012-9703-2
22. Norris JM, Johnson RK, Stene LC. Type 1 diabetes-early life origins and changing epidemiology. Lancet Diabetes Endocrinol. (2020) 8:226–38. doi: 10.1016/s2213-8587(19)30412-7
23. Conrad N, Misra S, Verbakel JY, Verbeke G, Molenberghs G, Taylor PN, et al. Incidence, prevalence, and co-occurrence of autoimmune disorders over time and by age, sex, and socioeconomic status: a population-based cohort study of 22 million individuals in the UK. Lancet. (2023) 401:1878–90. doi: 10.1016/S0140-6736(23)00457-9
24. Warncke K, Fröhlich-Reiterer EE, Thon A, Hofer SE, Wiemann D, Holl RW. Polyendocrinopathy in children, adolescents, and young adults with type 1 diabetes: a multicenter analysis of 28,671 patients from the German/Austrian DPV-Wiss database. Diabetes Care. (2010) 33:2010–2. doi: 10.2337/dc10-0404
25. Burbaud M, Renard E, Jellimann S, Luc A, Di Patrizio M, Remen T, et al. Additional autoimmune diseases associated with type 1 diabetes in children and adolescents: A French single-center study from 2014 to 2021. Arch Pédiatrie. (2022) 29:381–7. doi: 10.1016/j.arcped.2022.03.002
26. Kordonouri O, Hartmann R, Deiss D, Wilms M, Grüters-Kieslich A. Natural course of autoimmune thyroiditis in type 1 diabetes: association with gender, age, diabetes duration, and puberty. Arch Dis Child. (2005) 90:411–4. doi: 10.1136/adc.2004.056424
27. Lafontaine N, Wilson SG, Walsh JP. DNA methylation in autoimmune thyroid disease. J Clin Endocrinol Metab. (2022) 108:604–13. doi: 10.1210/clinem/dgac664
28. Ma J, Wu D, Li C, Fan C, Chao N, Liu J, et al. Lower serum 25-hydroxyvitamin D level is associated with 3 types of autoimmune thyroid diseases. Medicine. (2015) 94:1–7. doi: 10.1097/MD.0000000000001639
29. Çamurdan OM, Döğer E, Bideci A, Çelik N, Cinaz P. Vitamin D status in children with Hashimoto thyroiditis. J Pediatr Endocrinol Metab. (2012) 25:467–70. doi: 10.1515/jpem-2012-0021
30. Effraimidis G, Badenhoop K, Tijssen JGP, Wiersinga WM. Vitamin D deficiency is not associated with early stages of thyroid autoimmunity. Eur J Endocrinol. (2012) 167:43–8. doi: 10.1530/EJE-12-0048
31. Botelho IMB, Moura Neto A, Silva CA, Tambascia MA, Alegre SM, Zantut-Wittmann DE. Vitamin D in Hashimoto’s thyroiditis and its relationship with thyroid function and inflammatory status. Endocrine J. (2018) 65:1029–37. doi: 10.1507/endocrj.EJ18-0166
32. Wu Q, Wang Y, Chen P, Wei J, Lv H, Wang S, et al. Increased incidence of Hashimoto thyroiditis in selenium deficiency: A prospective 6-year cohort study. J Clin Endocrinol Metab. (2022) 107:e3603–e11. doi: 10.1210/clinem/dgac410
33. Pedersen IB, Knudsen N, Carlé A, Vejbjerg P, Jørgensen T, Perrild H, et al. A cautious iodization programme bringing iodine intake to a low recommended level is associated with an increase in the prevalence of thyroid autoantibodies in the population. Clin Endocrinol. (2011) 75:120–6. doi: 10.1111/j.1365-2265.2011.04008.x
34. Aghini Lombardi F, Fiore E, Tonacchera M, Antonangeli L, Rago T, Frigeri M, et al. The effect of voluntary iodine prophylaxis in a small rural community: the Pescopagano survey 15 years later. J Clin Endocrinol Metab. (2013) 98:1031–9. doi: 10.1210/jc.2012-2960
35. Song H, Fang F, Tomasson G, Arnberg FK, Mataix-Cols D, Fernández de la Cruz L, et al. Association of stress-related disorders with subsequent autoimmune disease. JAMA. (2018) 319:2388–400. doi: 10.1001/jama.2018.7028
36. Effraimidis G, Tijssen JGP, Brosschot JF, Wiersinga WM. Involvement of stress in the pathogenesis of autoimmune thyroid disease: A prospective study. Psychoneuroendocrinology. (2012) 37:1191–8. doi: 10.1016/j.psyneuen.2011.12.009
37. Pitto L, Gorini F, Bianchi F, Guzzolino E. New insights into mechanisms of endocrine-disrupting chemicals in thyroid diseases: the epigenetic way. Int J Environ Res Public Health. (2020) 17:1–18. doi: 10.3390/ijerph17217787
38. Assaad SN, Meheissen MA, Elsayed ET, Alnakhal SN, Salem TM. Study of Epstein–Barr virus serological profile in Egyptian patients with Hashimoto’s thyroiditis: A case-control study. J Clin Trans Endocrinol. (2020) 20:100222. doi: 10.1016/j.jcte.2020.100222
39. Heidari Z, Jami M. Parvovirus B19 infection is associated with autoimmune thyroid disease in adults. Int J Endocrinol Metab. (2021) 19:e115592. doi: 10.5812/ijem.115592
40. Seyyedi N, Dehbidi GR, Karimi M, Asgari A, Esmaeili B, Zare F, et al. Human herpesvirus 6A active infection in patients with autoimmune Hashimoto’s thyroiditis. Braz J Infect Diseases. (2019) 23:435–40. doi: 10.1016/j.bjid.2019.10.004
41. Figura N, Di Cairano G, Moretti E, Iacoponi F, Santucci A, Bernardini G, et al. Helicobacter pylori infection and autoimmune thyroid diseases: the role of virulent strains. Antibiotics (Basel). (2019) 9:1–13. doi: 10.3390/antibiotics9010012
42. Lui DTW, Lee CH, Woo YC, Hung IFN, Lam KSL. Thyroid dysfunction in COVID-19. Nat Rev Endocrinol. (2024) 20:336–48. doi: 10.1038/s41574-023-00946-w
43. d’Aniello F, Amodeo ME, Grossi A, Ubertini G. Thyroiditis and COVID-19: focus on pediatric age. A narrative review. J Endocrinol Invest. (2024) 47:1633–40. doi: 10.1007/s40618-024-02331-4
44. Shidid S, Kohlhoff S, Smith-Norowitz TA. Thyroid stimulating hormone levels in children before and during the coronavirus disease-19 pandemic. Health Sci Rep. (2022) 5:e579. doi: 10.1002/hsr2.579
45. McCowan R, Wild E, Lucas-Herald AK, McNeilly J, Mason A, Wong SC, et al. The effect of COVID-19 on the presentation of thyroid disease in children. Front Endocrinol (Lausanne). (2022) 13:1014533. doi: 10.3389/fendo.2022.1014533
46. Murugan AK, Alzahrani AS. SARS-CoV-2: emerging role in the pathogenesis of various thyroid diseases. J Inflammation Res. (2021) 14:6191–221. doi: 10.2147/jir.S332705
47. Vahabi M, Ghazanfari T, Sepehrnia S. Molecular mimicry, hyperactive immune system, and SARS-COV-2 are three prerequisites of the autoimmune disease triangle following COVID-19 infection. Int Immunopharmacol. (2022) 112:109183. doi: 10.1016/j.intimp.2022.109183
Keywords: type 1 diabetes mellitus, COVID-19, epidemiology, Hashimoto disease, pediatric endocrinology, post-acute COVID-19 syndrome, SARS-CoV-2, autoimmune thyroiditis
Citation: Herczeg V, Muzslay E, Czipó D, Terkovics L, Takács J, Garai R, Kovács F, Luczay A, Körner A and Tóth-Heyn P (2025) Increasing prevalence of thyroid autoimmunity in childhood type 1 diabetes in the pre-COVID but not during the COVID era. Front. Endocrinol. 15:1496155. doi: 10.3389/fendo.2024.1496155
Received: 13 September 2024; Accepted: 31 December 2024;
Published: 24 January 2025.
Edited by:
Sally Radovick, The State University of New Jersey, United StatesReviewed by:
Vera M. Zdravkovic, University Children’s Hospital, SerbiaCopyright © 2025 Herczeg, Muzslay, Czipó, Terkovics, Takács, Garai, Kovács, Luczay, Körner and Tóth-Heyn. This is an open-access article distributed under the terms of the Creative Commons Attribution License (CC BY). The use, distribution or reproduction in other forums is permitted, provided the original author(s) and the copyright owner(s) are credited and that the original publication in this journal is cited, in accordance with accepted academic practice. No use, distribution or reproduction is permitted which does not comply with these terms.
*Correspondence: Péter Tóth-Heyn, dG90aC1oZXluLnBldGVyQHNlbW1lbHdlaXMuaHU=
Disclaimer: All claims expressed in this article are solely those of the authors and do not necessarily represent those of their affiliated organizations, or those of the publisher, the editors and the reviewers. Any product that may be evaluated in this article or claim that may be made by its manufacturer is not guaranteed or endorsed by the publisher.
Research integrity at Frontiers
Learn more about the work of our research integrity team to safeguard the quality of each article we publish.