- 1Department of Urology, Tianjin Children’s Hospital/Tianjin University Children’s Hospital, Tianjin, China
- 2Department of Pediatric Surgery, Qilu Hospital of Shandong University Dezhou Hospital (Dezhou People’s Hospital), Dezhou, Shandong, China
Objectives: Maternal exposure to environmental endocrine disruptors, such as phthalates, during pregnancy is a significant risk factor for the development of hypospadias. By consolidating existing research on the mechanisms by which phthalates induce hypospadias in rodent models, this systematic review aims to organize and analyze the discovered mechanisms and their potential connections.
Methods: The study involved all articles that explored the mechanisms of phthalate-induced hypospadias using rodent models. A comprehensive search of the PubMed and Web of Science databases was conducted using the terms “hypospadias” and “phthalates” before January 20, 2024. Then, two investigators screened for studies worthy of inclusion by setting inclusion and exclusion criteria.
Results: Of the initial 326 search results, 22 were included in the subsequent analysis. Based on the commonalities among different results, the mechanisms of phthalate-induced hypospadias could be categorized into the following five groups: sex steroids-related signaling pathways (n=10), epithelial-mesenchymal transition (n=6), autophagy (n=5), apoptosis (n=4) and angiogenesis (n=2). Among these, sex steroids-related signaling pathways might serve as a central regulator among all mechanisms, and reactive oxygen species (ROS) also played an important mediating role.
Conclusion: The systematic review indicates that phthalates may initially disrupt the balance of sex steroids-related pathways, leading to abnormally elevated levels of ROS and subsequently to other functional abnormalities, ultimately resulting in the development of hypospadias. All these findings will help to improve prevention strategies during pregnancy to reduce the adverse effects of phthalates on the offspring.
1 Introduction
Hypospadias ranks as one of the most common congenital malformations in the male genitourinary system, exhibiting a global incidence rate ranging from 0.6 to 34.2 cases per 10,000 live births (1). It is a male birth defect which is characterized by the ectopic urethral opening being displaced along the ventral side of the penis. Although surgical correction is possible, the procedure and subsequent post-operative complications undeniably present significant challenges for affected children. Therefore, elucidating the etiology of hypospadias has become an urgent scientific challenge, as it enables the development of potential preventive strategies. Current perspectives suggest that the pathogenesis of hypospadias is multifactorial, encompassing genetic factors, maternal influences, and environmental determinants (2). However, empirical genome-wide association studies (GWASs) on hypospadias in human cohorts indicate that detectable genetic mutations account for less than 10% of the observed cases (3, 4), suggesting that non-genetic factors may play a more significant role in the development of this congenital malformation. Consequently, the speculation that excessive maternal intake of environmental endocrine-disrupting chemicals (EDCs) leads to abnormal embryonic development of fetal genitalia has garnered increasing attention.
The development of male external genitalia is governed by a precise signaling pathway transduction within the genital tubercle (GT) of the embryo, guided by hormonal cues. This process orchestrates the orderly differentiation, migration, and proliferation of cells, resulting in the formation of a tubular urethra and a fully developed foreskin (5, 6). During the early hormone-independent stage of genital development, the urethral plate gradually forms along the embryonic midline. Subsequently, in the hormone-dependent phase, the influence of sex steroids-related pathways on external genitalia development becomes increasingly significant. A balanced signaling of androgens and estrogens jointly supports the normal masculinization process of the GT, leading to the elongation of the urethra, which ultimately encapsulates and forms the structures of the male external genitalia (7, 8). The WNT, FGF, BMP, and Hedgehog (HH) signaling pathways play crucial roles in the development and patterning of various tissues within the penis (9, 10). These pathways are interconnected and exhibit significant crosstalk within their genetic networks. Under the regulation of estrogens and androgens, they collaboratively facilitate signaling between the epithelium and mesenchyme, coordinating the spatiotemporal development of the external genitalia (11).
EDCs are a broad class of exogenous agents known to interfere with the endocrine system by imitating, blocking, or otherwise modifying hormonal signals, with a pronounced impact on sex steroids (12). Several studies have indicated that pregnant women’s exposure to high levels of EDCs may disrupt critical signaling pathways responsible for sexual differentiation in fetal development, potentially leading to genitourinary malformations, such as hypospadias (13, 14). Phthalates, serving as plasticizers, rank among the most frequently encountered EDCs in the daily lives of pregnant women due to their extensive use in a wide array of consumer and industrial products, including food packaging, toys, medical devices, and personal care items (15–17). These compounds can easily leach into the environment due to their non-covalent bond with plastics. Subsequently, when absorbed by pregnant women through pathways such as ingestion, inhalation, and dermal absorption, these substances can cross the placental barrier and ultimately impact fetal embryonic development (18, 19).
The positive correlation between prenatal exposure to phthalates and the incidence of hypospadias in offspring has been confirmed in epidemiological studies, highlighting the necessity for experimental investigation of the specific mechanisms by which phthalates induce hypospadias (20, 21). Given the considerable technical and ethical challenges associated with direct research on human penile embryonic development, researchers have predominantly relied on rodent models to investigate the etiology of hypospadias. This approach typically involves administering phthalates to pregnant rodents via oral gavage to induce hypospadias phenotypes in their offspring. While a substantial body of research has identified numerous potential pathogenic mechanisms triggered by phthalates, the findings are disparate and lack a cohesive framework. This divergence largely stems from variations in experimental designs and foci across studies, which complicates the task of drawing comprehensive conclusions.
The present systematic review aims to consolidate existing research on the mechanisms by which phthalates induce hypospadias in rodent models. By categorizing identified mechanisms and examining their interrelations, this review seeks to uncover underlying core mechanisms that may govern the wide array of signaling pathways implicated in phthalate-induced hypospadias, thereby offering new insights into potential preventive strategies for this congenital condition associated with environmental endocrine disruptors.
2 Methods
To systematically investigate the mechanisms of phthalate-induced hypospadias, we retrieved, screened, categorized and analyzed the data according to the Preferred Reporting Items for Systematic reviews and Meta-Analyses (PRISMA) statement (22).
2.1 Information search
Prior to undertaking the systematic review, both investigators first identified “phthalates” and “hypospadias” as search terms. A comprehensive search was conducted in PubMed and Web of Science databases on January 20, 2024 (The specific search strategy is detailed in Supplementary Table S1). This was not time-limited, but the search was restricted to English-language publications. Search results were independently evaluated by two investigators for relevance to the purpose of the study.
2.2 Inclusion and exclusion criteria
Experimental studies on the utilization of phthalates to create animal models of hypospadias for mechanism analysis were eligible for inclusion. The exclusion criteria were 1) narrative or systematic reviews and meta-analyses; 2) epidemiological research (e.g., case-control, prospective cohort studies); 3) reports or other non-experimental studies; 4) published abstracts without full text available or publications in languages other than English; 5) other animal studies not focused on hypospadias models. Three investigators individually screened all studies to confirm eligibility. Any disagreements were resolved through discussion to optimize the screening protocol and reach consensus.
2.3 Data extraction
Variables for study characteristics, methodology/design and outcomes were main elements of data integration. Study characteristics variables included first author, year of publication and study subject. The following data were extracted as methodology variables: phthalate selection and treatment, in vivo and/or in vitro experimental design. Outcome variables recorded the incidence and phenotype of hypospadias in the offspring, expression of relevant pathway components, alterations in biological function and key conclusions. Two investigators independently performed the data collection process described above, and if differential results were presented, they were validated and adjudicated by a third investigator.
3 Results
Our search ultimately yielded 326 preliminary results, of which 105 were duplicates. The eligibility criteria and the specific process for study selection are described in Figure 1. Afterward, we eliminated animal model studies without full text or those not in English, as well as certain types of publications (reviews, meta-analyses, epidemiological studies, and other non-experimental studies). Through full-text screening, 27 articles unrelated to the topic of our review were further excluded, resulting in 22 papers deemed qualified for integrated analysis.
3.1 Study characteristics
Table 1 summarizes the methodological designs for constructing hypospadias rodent models and the characteristics of the hypospadias offspring in the included studies. In the 22 studies reviewed, researchers used either di-n-butyl phthalate (DBP) or di-(2-ethylhexyl) phthalate (DEHP) for prenatal exposure. DBP was predominantly studied (N=16; all rat models), with 6 studies on DEHP (4 rat models and 2 mouse models). Among all rodent models, Sprague-Dawley (SD) rats were used in 20 studies, C57BL/6 mice were used in one study (23), and one study (24) did not specify the mouse strain. Due to the presence of specific urethral developmental windows, phthalate treatments were administered during days 10-19 of gestation in all studies, but the duration of exposure varied. In addition, the phthalate concentrations adopted in the experiments ranged from 100 mg/kg body weight (bw)/day to 1000 mg/kg bw/day. By comparing the incidence of hypospadias in the offspring, we could broadly see that higher phthalate concentrations were associated with greater induction success (23, 25–27). At the same time, there were also differences in the timing of offspring sample collection. After harvesting the offspring, 19 studies have directly or indirectly documented urethral developmental abnormalities in pups (23–41), which were important criteria for screening hypospadias fetuses, mainly characterized by an ectopic urethral meatus, and ventral foreskin deficiency with incomplete fusion in the middle of the skin folds.
To further investigate the underlying molecular mechanisms, in addition to in vivo experiments, 10 studies also combined in vitro experiments, including cell cultures [urethral epithelial cells (24, 34, 39–43), fibroblasts (35, 44), endothelial cells (28, 39, 41)] and isolated penis cultures (27). In selecting reagents for in vitro studies, to reflect the bioactive forms of phthalates in biological systems, the DEHP treatment group used its primary metabolite, mono-(2-ethylhexyl) phthalate (MEHP). However, in some studies, DBP rather than mono-butyl phthalate (MBP) was directly applied for cell induction (34, 39, 41–43).
3.2 Mechanisms of phthalate-induced hypospadias
Table 2 outlines the implications of DBP/DEHP on various signaling pathways and their terminal outcomes. After a comprehensive review of the included studies, based on the commonalities among different results, we categorized the mechanisms of phthalate-induced hypospadias into the following five groups: sex steroids-related signaling pathways, epithelial-mesenchymal transition (EMT), autophagy, apoptosis and angiogenesis.
3.2.1 Sex steroids-related pathways
Ten studies discussed alterations in sex steroids-related signaling pathways (Figure 2). The blocked androgen pathway, as evidenced by the inhibition of testosterone synthesis and impaired androgen receptor (AR) signaling, was observed in the nine included studies. Compared to normal offspring, hypospadias rats exhibited decreased serum testosterone levels and reduced AR expression in the GT. Although one study showed elevated testosterone levels, this was interpreted as a blockage in the conversion of testosterone to dihydrotestosterone (DHT) induced by DEHP (26). Four of these studies further histologically described testicular dysplasia in hypospadias male offspring, including testicular morphological abnormalities, disorganized structure of the seminiferous tubules, aberrant Leydig cell proliferation, and decreased germ cells in adulthood (25, 31, 32, 38). Moreover, three studies found that key enzymes involved in testosterone synthesis, represented by Cyp11a1, Cyp17a1, Hsd3b, Scarb1, Star, Hsd17b3 and Srd5α2, were significantly reduced at the genetic level in DBP- or DEHP-induced hypospadias male fetal rats (26, 31, 32). Among them, Shi et al. (26) indicated that the inhibition of Srd5α2 levels might be caused by DEHP-induced oxidative stress and the subsequent upregulation of MAPK10 and MAPK14. This oxidative stress effect was possibly related to the decreased activity of antioxidant enzymes and the increased expression of NOX1 and NOX4 following DEHP treatment. In addition to the observed decrease in AR expression, two studies investigated the inhibitory effect of DBP at different stages of AR signaling, respectively. Using molecular docking experiments, Zhou et al. (37) showed that DBP had a high affinity for AR and could competitively bind to the androgen receptor to block signal transduction. In the other study, comparing AR levels in the cytoplasm and nucleus, DBP was further found to disrupt AR nuclear translocation by inhibiting TGFB1I1 expression (41). The possible association between the lack of androgen signaling and certain ablated growth factors (Shh, Fgf10, Gli2, Gli3, Bmp4, Wnt5a, Hoxa13, Hoxd13, Fgf8, Fgfr2, etc.) were proposed in three studies (30–32). A limitation was that these studies only detected gene expression levels, in the absence of further experimental validation to confirm that the abnormal expression of these genes is indeed caused by disrupted androgen signaling pathway.
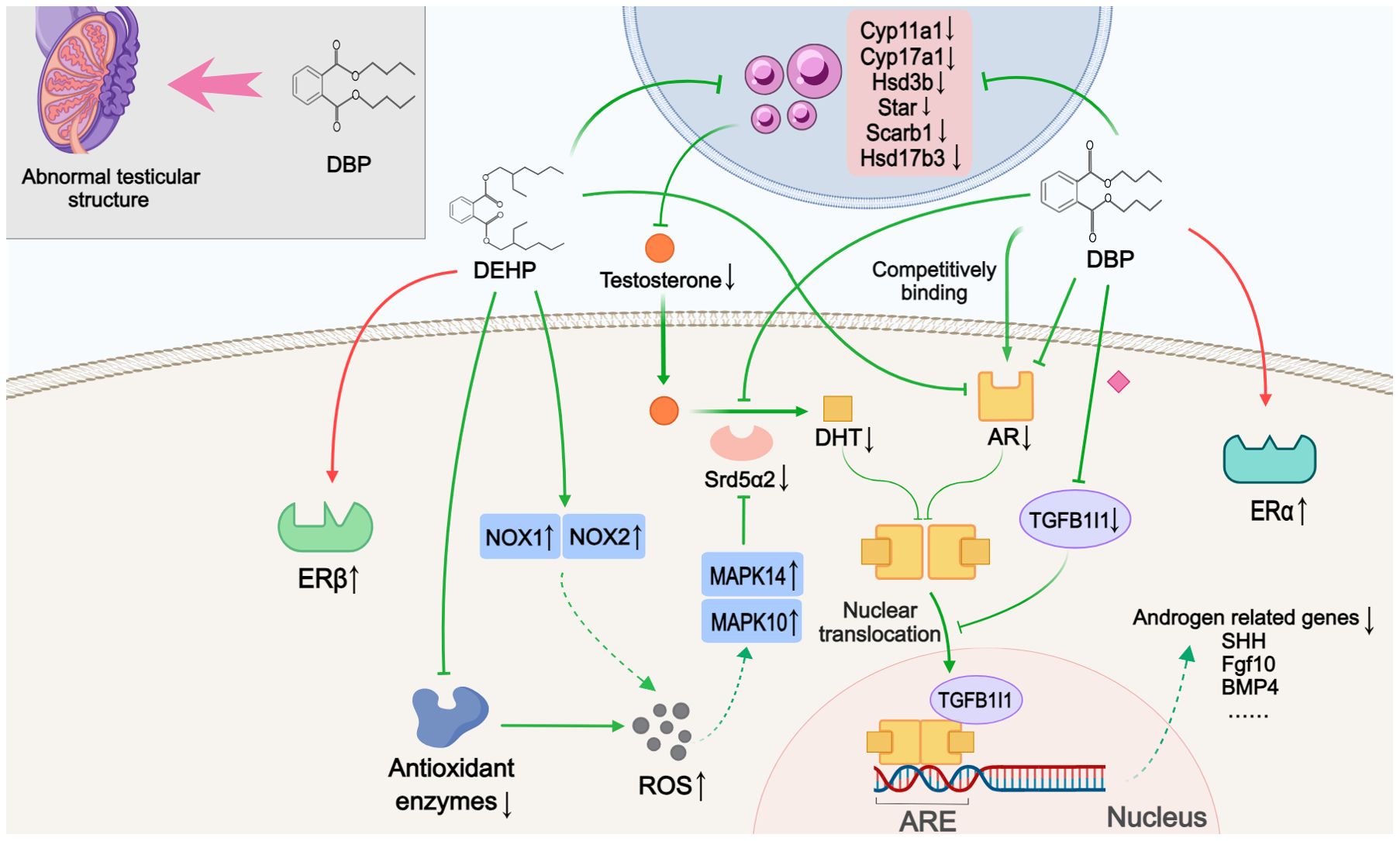
Figure 2. Mechanisms by which phthalates affected sex steroids-related pathways. During the development of offspring, DBP caused abnormal testicular structure. DBP and DEHP hindered testosterone synthesis and androgen signaling through various mechanisms, such as downregulating key enzymes involved in androgen synthesis, antagonizing AR, and reducing TGFB1I1 expression to inhibit nuclear translocation. This impaired androgen pathway may subsequently inhibit the expression of androgen-related genes. Simultaneously, DEHP and DBP also activated estrogen signaling by promoting the expression of ERβ and ERα, respectively. ARE: Androgen response element. (Solid arrows represent mechanisms that have been demonstrated in the included studies). Dashed arrows indicate that mechanisms were proposed but were not experimentally validated in the included studies. Diamond-shaped icons represent the mechanisms that have been validated in vitro experiment in the included studies).
Three studies were categorized as related to estrogenic effects. Interestingly, the data suggested a discrepancy in the selectivity of DBP and DEHP for estrogen receptors (ERs). One study showed that DBP had high affinity values for ERα protein and could bind to and activate the ERα protein (37). Yet another study indicated that in the DEHP-treated groups, with increasing concentrations, DEHP did not seem to have a significant effect on the expression of ERα, but rather promoted the expression of ERβ. In the third study, high expression of the proto-oncogene c-Fos, which was thought to be activated by estrogen signaling, was detected in the GT of hypospadias rats as well as in the human foreskin (36).
3.2.2 EMT
The six included studies demonstrated that phthalates influence EMT through various mechanisms (Figure 3A). Alterations in the expression of epithelial markers (e.g., E-cadherin, ZO-1, occludin) and mesenchymal markers (e.g., N-cadherin, α-SMA, vimentin) were used to evaluate EMT levels. Four studies observed a decreased level of EMT during urethral development in hypospadias offspring. Zhou et al. (27) showed that DEHP treatment inhibited the TGF-β/Smad signaling pathway, contributing to a decline in EMT. In a study by Zhu et al., overexpression of PFN2 was found to promote EMT in urethral epithelial cells through activation of the Smad/TGF-β1 pathway, suggesting that the significantly downregulated PFN2 in hypospadias may inhibit EMT by suppressing the expression of Smad2/3 and TGF-β1 (40). Moreover, a previous study indicated that decreased AR expression and increased ERα expression were potentially associated with decreased EMT (37). One study also found that the oxidative stress effect resulting from the combination of elevated reactive oxygen species (ROS) levels and reduced antioxidant enzyme genes expression after DBP exposure increased IP3R expression and intracellular calcium concentration in urethral epithelial cells, thereby inhibiting EMT (43). However, two other studies reported the contrary results. In a further study of autophagy, Zhao et al. (34) found that DBP-induced autophagy due to impaired androgen signaling could have activated EMT via the autophagy-lysosomal pathway. With the addition of autophagy inhibitors, EMT levels in urethral epithelial cells were also suppressed. Unlike single cell line cultures, another study verified cellular interactions by co-culturing human endothelial and urothelial cells. DBP exposure of vascular endothelium increased NAP-2 secretion via activation of the RhoA/Rock pathway and promotion of ROS production. This vascular endothelial-derived NAP-2 contributed to EMT of urothelial cells by upregulating TGF-β expression (39). Comparing the methodological differences in the above studies, we found that inconsistencies in sampling times resulted in hypospadias pups exhibiting decreased levels of EMT on GD19 or PND1, enhanced EMT on PND7, and again diminished EMT on PND10 (Figure 3B). There were four additional studies in relation to the expression of TGF-β1 (23, 24, 28, 38). The issue was that these studies lacked valid evidence to confirm their association with EMT, and therefore, we have not included them in our analysis.
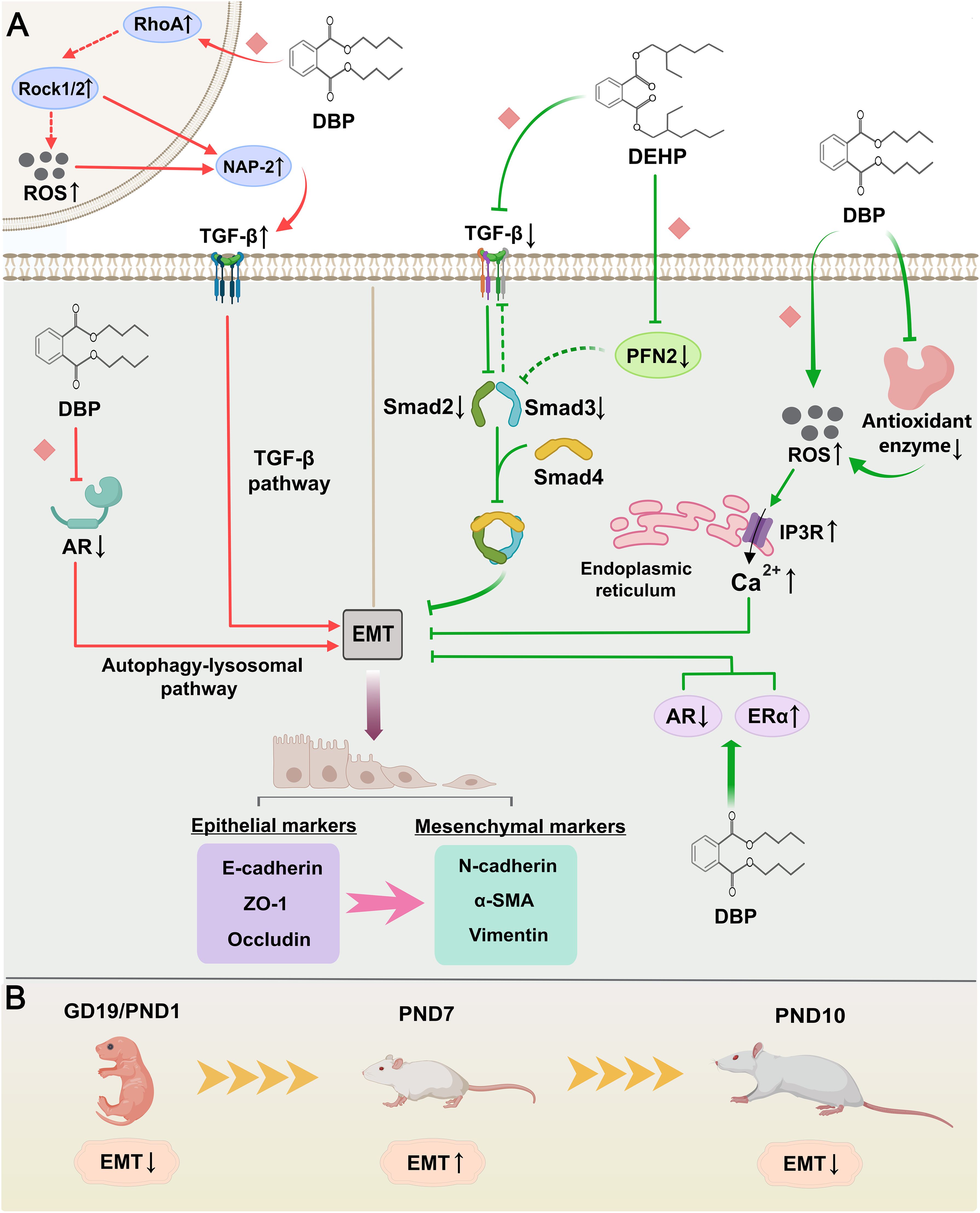
Figure 3. Mechanisms by which phthalates affect epithelial-mesenchymal transition (EMT). (A) The green arrows indicate that both DEHP and DBP have inhibitory effects on EMT. DEHP could reduce the expression of the TGF-β/Smad pathway by directly inhibiting or downregulating PFN2, thereby suppressing EMT. Simultaneously, DBP also inhibited EMT not only by upregulating IP3R and intracellular calcium levels, but also by affecting the expression of AR and ERα. The red arrows show that DBP has a promoting effect on EMT. DBP increased TGF-β expression by promoting NAP-2 secretion in the vascular endothelium, which in turn enhanced EMT in urethral epithelial cells. Furthermore, DBP could induce autophagy by impairing androgen signaling and enhance the EMT process through the autophagy-lysosome pathway. (B) Changes in EMT levels in phthalate-treated neonatal rats at different sampling times. (Solid arrows represent mechanisms that have been demonstrated in the included studies). Dashed arrows indicate that mechanisms were proposed but were not experimentally validated in the included studies. Diamond-shaped icons represent the mechanisms that have been validated in vitro experiment in the included studies).
3.2.3 Autophagy
Five studies reported various pathways through which phthalates affect autophagy (Figure 4). Among all included studies, DBP was used to construct rat models of hypospadias, and the LC3II/LC3I ratio or polyubiquitin-binding protein p62 were considered crucial indicators for assessing autophagy levels. Three studies documented increased autophagy levels in GTs of hypospadias fetal male rats following maternal exposure to DBP. Using electron microscopy to observe and compare GTs in hypospadias and normal offspring rats, Li et al. (33) found that autophagosomes were only detected in the hypospadias group. Meanwhile, some direct or indirect inhibitory proteins of the autophagy pathway (e.g., p-Akt, p-mTOR and p-S6) were also significantly decreased in the GT of hypospadias fetuses, suggesting that DBP might enhance autophagy by inhibiting the PI3K/Akt/mTOR pathway (33). Another study established a stable AR-transfected urethral epithelial cell line and found that increased AR expression was associated with decreased levels of autophagy compared to the non-transfected group (34). This indicated that DBP-induced impaired androgen signaling also contributed to the promotion of autophagy. In addition, Zhao et al. (44) demonstrated that maternal exposure to DBP activated the PERK/eIF2α pathway, leading to increased autophagy in hypospadias offspring. In transfection experiments of GT fibroblasts in vitro, PERK silencing was found to downregulate the phosphorylation of eIF2α and the expression of ATF4, thereby inhibiting autophagy, which inversely confirmed the intrinsic correlation between the PERK/eIF2α pathway and DBP-induced autophagy. The remaining two studies examined the effects of DBP on autophagy levels in cell lines, rather than on the entire GT. DBP exposure has been reported to promote cellular autophagy via inhibition of hedgehog signaling in urethral epithelial cells through upregulation of HhIP expression (42). DBP-induced oxidative stress, through increased HhIP mRNA and protein expression, was also observed to be involved in this regulatory process. However, Feng et al. (35) presented a different view, suggesting that overexpression of NONRATT008453.2 in GT fibroblasts from hypospadias male rat fetuses had an inhibitory effect on cellular autophagy. Although this lncRNA was detected to be highly expressed in GTs of hypospadias rats, direct evidence of autophagy inhibition in GTs was still lacking.
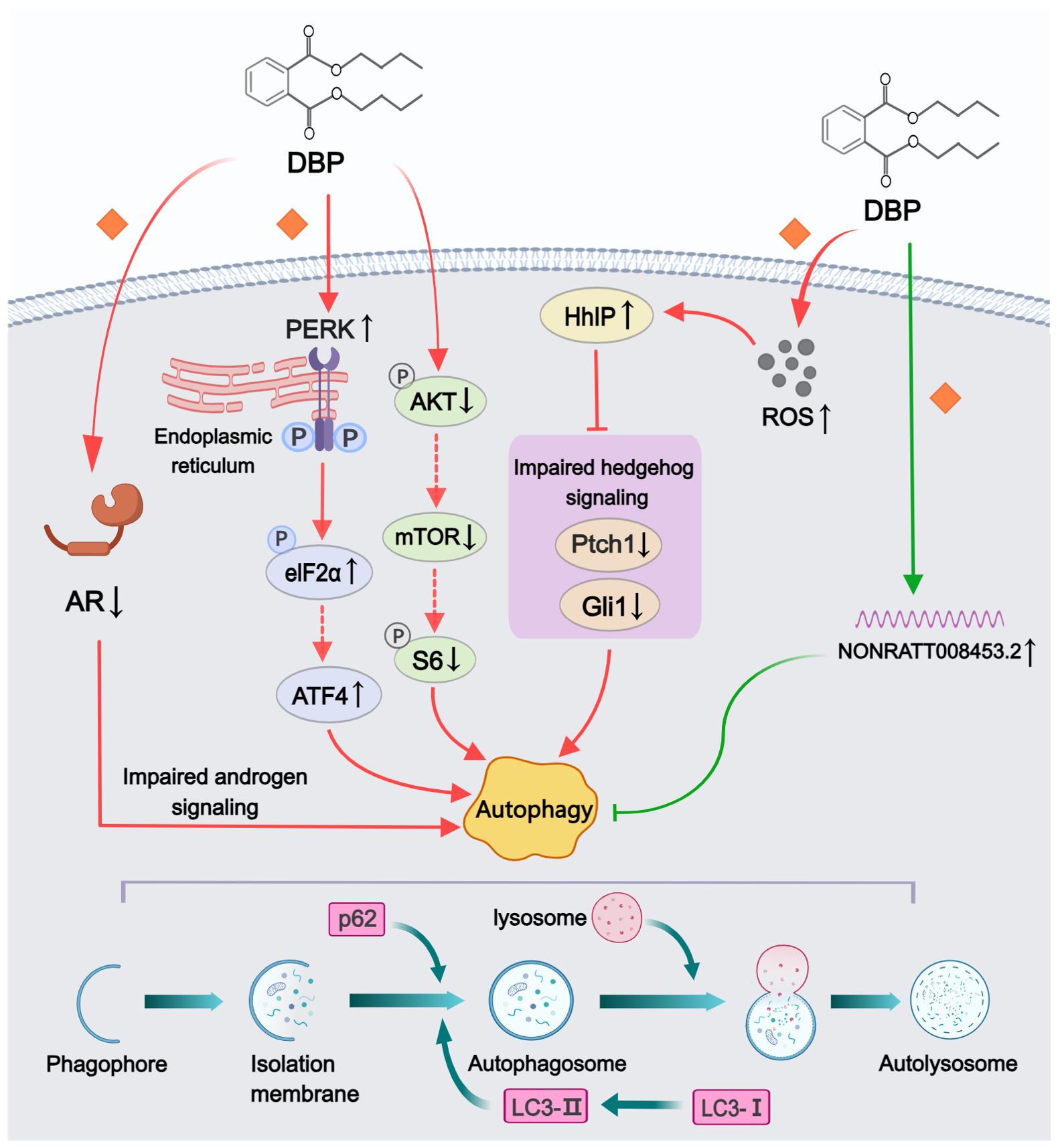
Figure 4. Mechanisms by which phthalates affected autophagy. DBP could enhance autophagy through various pathways, including disrupting androgen signaling, inhibiting the PI3K/Akt/mTOR signaling pathway, impairing the hedgehog signaling pathway, and activating the PERK/eIF2α signaling pathway. However, the elevated levels of NONRATT008453.2 following DBP treatment exhibited autophagy-inhibiting effects. (Solid arrows represent mechanisms that have been demonstrated in the included studies). Dashed arrows indicate that mechanisms were proposed but were not experimentally validated in the included studies. Diamond-shaped icons represent the mechanisms that have been validated in vitro experiment in the included studies).
3.2.4 Apoptosis
Four studies investigated the regulation of phthalates on cell apoptosis (Figure 5). Terminal deoxynucleotidyl transferase dUTP nick end labelling (TUNEL) assay or annexin V-fluorescein isothiocyanate (FITC)/PI double staining were widely adopted to detect cell apoptosis index. In a study on autophagy, Li et al. (33) found inhibition of apoptosis in hypospadias male fetuses, indicating that DBP-induced autophagy may resist apoptosis. The other three studies examined the pathways impacting apoptosis in different tissues or cells. One study analyzed the differential levels of autophagy and apoptosis in GT fibroblasts, suggesting that DBP-induced activation of the PERK/eIF2α signaling pathway and phosphorylation of eIF2α could attenuate apoptosis (44). In addition, Tian et al. (24) demonstrated through in vitro transfection experiments that following DEHP treatment, the overexpression of miR-494 and the reduced expression of its target gene Nedd4L can inhibit apoptosis in urethral epithelial cells. Conversely in another study, increased levels of apoptosis were observed in corpus cavernosum of ten-week-old DBP-induced hypospadias offspring rats (38). The Akt/Bax/caspase-3 pathway was implicated in regulating apoptosis levels, with increased caspase-3 protein levels and Bax/Bcl-2 ratios, alongside decreased pAkt/Akt ratios, detected in the hypospadias group.
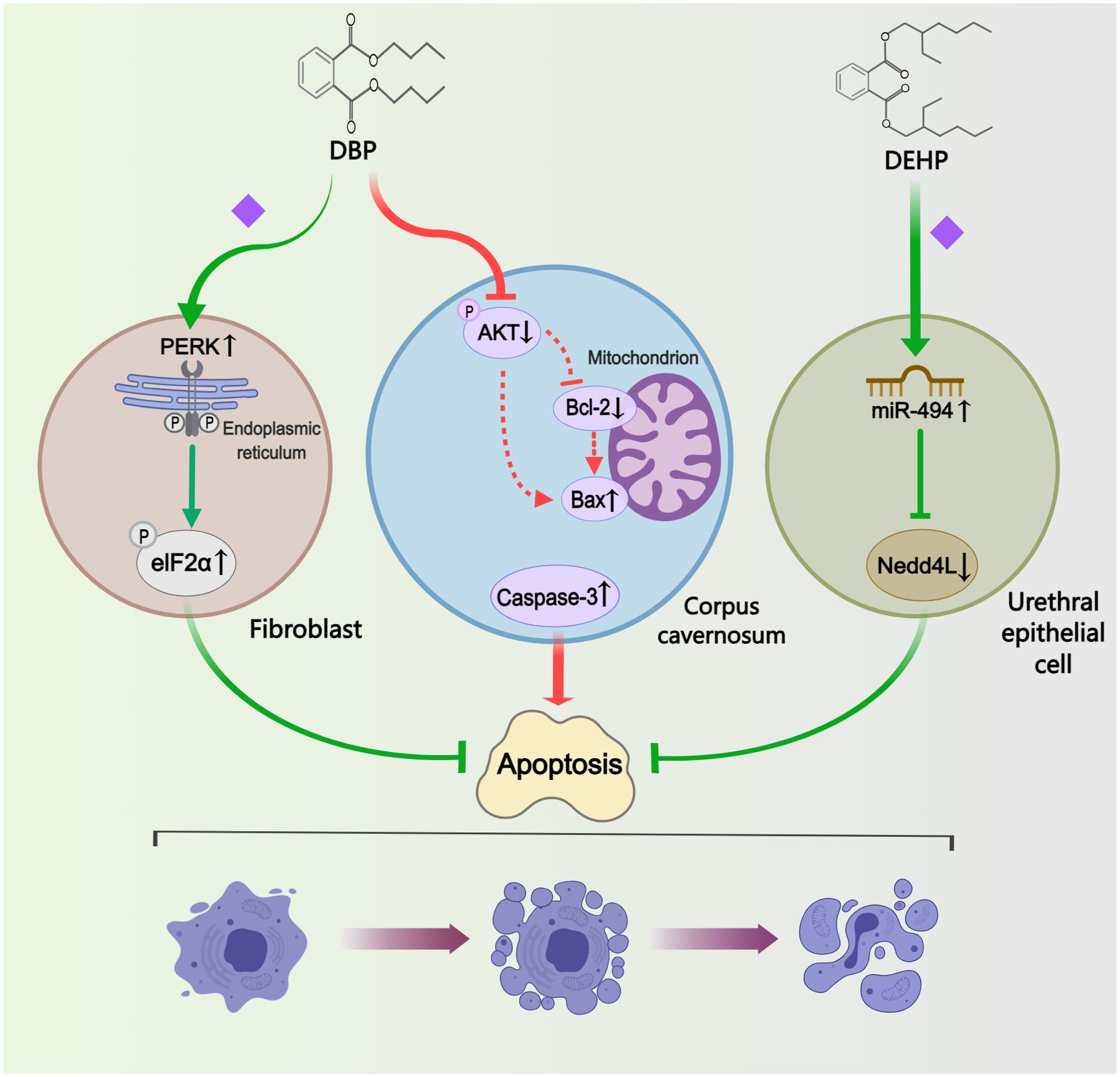
Figure 5. Mechanisms by which phthalates affected apoptosis. In different cells or tissues, the regulation of apoptosis exhibits variability: DBP inhibited fibroblast apoptosis by activating the PERK/eIF2α pathway; DBP enhanced apoptosis in the corpus cavernosum by modulating the Akt/Bax/caspase-3 pathway; and DEHP reduced apoptosis in the urethral epithelial cell by upregulating miR-494. (Solid arrows represent mechanisms that have been demonstrated in the included studies). Dashed arrows indicate that mechanisms were proposed but were not experimentally validated in the included studies. Diamond-shaped icons represent the mechanisms that have been validated in vitro experiment in the included studies).
3.2.5 Angiogenesis
Two studies were considered relevant for angiogenesis (Figure 6). Key criteria for evaluating angiogenic potential included tube-forming capacity and endothelial function. One study showed that low expression of TGFB1I1 was involved in DBP-induced aberrant angiogenesis in hypospadias fetal rats (41). By assessing tube formation and endothelial migration in vitro, Wu et al. (41) further found that overexpressed TGFB1I1 could mitigate the angiogenic inhibitory effect of DBP exposure. The other study observed only a significant decrease in the expression of eNOS and nNOS in corpus cavernosum of hypospadias rats upon maternal exposure to DBP (38). The author thought the subsequent inhibition of the NOS/cGMP pathway might be associated with depressed angiogenesis and endothelial dysfunction in corpus cavernosum. Additionally, this study suggested that reduced testosterone levels might also be partly responsible for the decreased expression of eNOS and nNOS. However, this study lacked direct assays of angiogenic function.
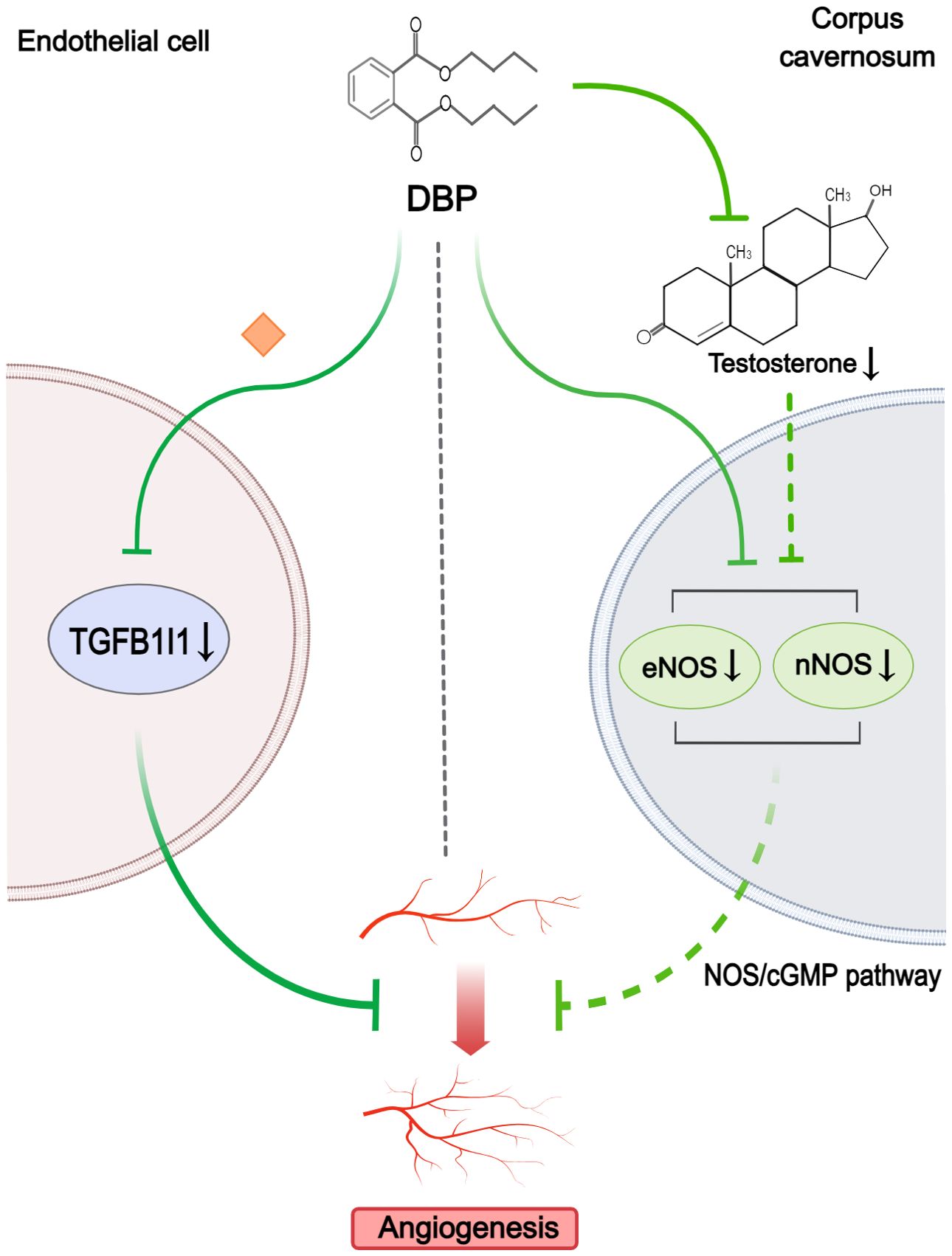
Figure 6. Mechanisms by which phthalates affected angiogenesis. DBP could inhibit angiogenesis by reducing TGFB1I1 expression in endothelial cells. Simultaneously, in the corpus cavernosum, DBP downregulated eNOS and nNOS, with low testosterone levels partly contributing to this reduction, resulting in impaired NOS/cGMP signaling and subsequent angiogenesis disruption. (Solid arrows represent mechanisms that have been demonstrated in the included studies). Dashed arrows indicate that mechanisms were proposed but were not experimentally validated in the included studies. Diamond-shaped icons represent the mechanisms that have been validated in vitro experiment in the included studies).
3.2.6 Interactions among the identified mechanisms
Several studies included in our review have investigated multiple mechanisms, as outlined in the preceding sections. We synthesized the findings from these studies to explore potential interactions among the identified mechanisms. For exploring the correlation between sex steroid signals and EMT, Zhou et al. (37) suggested that the AR downregulation and ERα upregulation induced by DBP may lead to EMT inhibition during urethral development. Observations in hypospadias fetal rats revealed that inhibition of AR expression resulted in an increase in autophagy (34). Notably, low levels of testosterone were associated with the attenuation of the NOS/cGMP pathway, implicating this pathway as one of the means by which androgen signaling influences angiogenesis (38). Considering above results, sex steroids-related signaling pathways might serve as a central regulator among all mechanisms, given its capacity to modulate the remaining ones (Supplementary Figure S1).
3.2.7 The mediating role of oxidative stress
Among the included studies, four mentioned that exposure to phthalates during pregnancy promoted the production of ROS in hypospadias fetal rats, and that this increase was dose-dependent (26, 39, 42, 43). And we further notably found that oxidative stress played a crucial role in regulating several identified mechanisms (Supplementary Figure S2). For the sex steroids-related pathways, DEHP-induced high levels of ROS interfered with androgen signaling by inhibiting Srd5α2 activity (26). Additionally, oxidative stress has been shown to increase autophagy in urothelial epithelial cells via the ROS-HhIP-Gli-autophagy pathway (42). Moreover, two studies elucidated the opposite effects of oxidative stress on EMT in fetal rats of different developmental stages. In fetal rats on PND1, DBP-induced oxidative stress might inhibit EMT by increasing IP3R expression and intracellular calcium levels in urethral epithelial cells (43). Another study found that oxidative stress in the vascular endothelium after DBP treatment upregulated NAP-2 secretion and subsequently enhanced EMT in urothelial cells on PND7 (39). Consequently, rather than categorizing ROS as a distinct mechanism, we considered it as a significant mediator in the phthalate-induced hypospadias.
4 Discussion
Phthalates, extensively used as plasticizers in everyday life, are one of the most prominent EDCs known to impact male urogenital system embryonic development and even adult reproductive health (45). Prenatal exposure to high doses of plasticizers has been identified as a potential risk factor for the development of hypospadias. Numerous animal experimental studies have found direct correlations between phthalates and hypospadias, however, the identified genes or signaling pathways were inconsistent and lacked a holistic overview. Therefore, this review systematically organized existing animal studies on phthalate-induced hypospadias, aiming to construct a more comprehensive understanding of this area. We included a total of 22 studies, all utilizing rodent models (rat or mouse) to establish hypospadias through the application of phthalates. Based on the objectives and the functions of the signaling pathways identified in each study, we have categorized the mechanisms of phthalate-induced hypospadias that have been validated to date into five groups: sex steroids-related signaling pathways, EMT, autophagy, apoptosis, and angiogenesis.
Hypospadias arises from the disruption of sexual differentiation during embryonic development, a process in which sex steroids, especially androgens, play a critical mediating role (46). The presence or absence of androgens during this period determines the ultimate outcome of ambisexual GT development (6). Phthalates are considered to have estrogen-like or anti-androgenic properties due to their ability to mimic estrogen effects in various tissues, including the reproductive system (13). Many studies investigating their pathogenic role in hypospadias have also focused on this aspect. The transmission of androgen signals can be understood as mainly composed of two parts: androgen synthesis and AR pathway. For the first part, testosterone is the major human androgen produced by Leydig cells in the testes (47), however, current research has discovered that phthalates can induce testicular histological structural disturbances, such as disorganized structure of the seminiferous tubules, and abnormal Leydig cell proliferation (31, 32). Additionally, phthalates also downregulate genes involved in androgen synthesis, such as Cyp11a1 and Cyp17a1, and inhibit Srd5a2, which converts testosterone to DHT, a more potent AR ligand (25, 26). The chaotic testicular structure and the suppression of androgen synthase gene expression, both induced by phthalates, collectively contribute to the inhibition of androgen synthesis. For the second part, upon binding and activation by testosterone or DHT, the AR undergoes nuclear translocation. Subsequently, it binds to androgen response elements located on the target DNA, thereby regulating the transcription of specific genes (48). AR expression was found to be markedly suppressed following exposure to phthalates (26, 30). Furthermore, Wu et al. (41) discovered that DBP could alter AR nuclear translocation via inhibiting TGFB1I1 expression. Beyond directly impacting the AR, DBP demonstrated a high affinity for AR proteins, enabling them to bind competitively to AR and exert inhibitory effects (37). In summary, phthalates can impact both androgen synthesis and AR pathway, leading to a reduction in androgen levels and efficacy. The disruption of androgen signaling ultimately led to diminished expression of androgen-related genes like Shh, Fgf10, and their downstream factors (Gli2, Gli3, Bmp4, Wnt5a, Hoxa13, Hoxd13, Fgfr2) (32). It is noteworthy that these genes are key components of the signaling pathways previously mentioned as critical for normal urethral development, and the aberrant expression of certain genes among these has been clinically confirmed to be closely associated with the incidence and severity of hypospadias (49, 50). Moreover, this aberrant sex steroids-related pathway may also suppress the expression of other important genes involved in urethral development. Comparative studies of foreskin tissue from patients with hypospadias and normal children have revealed significant downregulation of Rab25 and Mafb expression in the hypospadias group (51, 52). These genes are closely linked to androgen signal transduction, and their expression is suppressed when androgen signaling is impaired or absent. Phthalates may affect the expression of these genes by inhibiting normal androgen signaling. Contrary to the previous belief that androgens play a dominant role in the embryonic development of male genitalia, current research posits that the coordinated action between androgens and estrogens is key to penile development (53). Phthalates like DBP have been shown to increased ERα protein levels in the GT of hypospadias rats and could also bind to and activate ERα proteins (37). For DEHP, although it appeared to have no effect on the expression of ERα, but instead induced the expression of ERβ (26). To conclude, phthalates may simultaneously disrupt the androgen signaling pathway and anomalously stimulate the estrogen signaling pathway. This dual action can cause an imbalance between these pathways, ultimately culminating in the development of hypospadias.
EMT is an important biological process that allows polarized epithelial cells to lose their typical epithelial characteristics and acquire mesenchymal phenotypes (54). It is indispensable in the development and differentiation of embryonic tissues and organs (55). A critical step in the development of the male urethra in humans is the bilateral fusion of the urethral plates, which subsequently generates the urethral seam and forms a normal tubular urethra (56, 57). EMT, along with apoptosis and cell migration, has been considered as a pivotal cellular process essential for this developmental stage (58). This hypothesis was initially verified by a study detecting several epithelial-mesenchymal co-expression markers associated with EMT in the urethral seam (59). While the promotion of EMT by phthalate exposure has been mentioned in fibrosis (60) and tumor metastasis (61), the potential relationship between the two in hypospadias has not yet been fully elucidated. Among the various EMT regulators, TGF-β has attracted much attention as a major inducer (62, 63). In rat models, gestational exposure to DEHP has been reported to reduce the occurrence of EMT by inhibiting the TGF-β/Smad pathway, resulting in hypospadias (27). Zhu et al. in their research on urinary extracellular vesicles further discovered that the downregulation of TGF-β1 by DEHP may be mediated by PFN2 (40). DBP has also been found to inhibit the EMT process in the GT of hypospadias rats, with ROS and Ca2+ potentially playing significant roles in this effect (43). However, two studies observed an enhancement of the EMT process induced by DBP in the GT and urethral epithelial cells of hypospadias rats (34, 39). To explain these seemingly contradictory results, we compared the experimental details of studies focusing on the impact of phthalates on the EMT process in hypospadias. We found that variations in the timing of sample collection could be one contributing factor to the discrepancies observed across different studies. In summary, in studies involving prenatal fetuses or neonatal rats, the EMT process was inhibited by phthalates. By postnatal day seven, phthalates appear to promote EMT, whereas by postnatal day ten, they exhibit an inhibitory effect again. We propose that a plausible explanation for this phenomenon may be attributable to the unique characteristics of penile development in rats. Unlike the human penis, which completes its development prenatally, the rat penis continues to undergo morphological changes after birth (64). Hence, the EMT process in the penile region may continue to undergo dynamic changes after birth in rats, leading to varying results at different sampling times. This suggests that the regulation of EMT by phthalates may not be unidirectional; in other words, it can antagonize the normal rhythmic progression of EMT during development. To elucidate the regulatory mechanisms of phthalates on EMT in penile development, more in-depth explorations are needed, such as conducting longitudinal studies.
Autophagy is the primary intracellular degradation and recycling system that breaks down and recycles aged, damaged, or excess organelles, proteins, and other intracellular components through the lysosomal system (65). During biological growth, autophagy is considered a crucial dynamic metabolic process for maintaining normal cell differentiation and development (66). However, insufficient or excessive autophagy can disrupt intracellular homeostasis, potentially leading to cell death (67). Consequently, abnormal autophagic activity during penile embryonic development may contribute to the formation of hypospadias. Evidence supporting this included the observation of increased autophagosomes and autophagosome-forming proteins (LC3-I and LC3-II) in the GT of rats with DBP-induced hypospadias (33). Li et al. (33) discovered that DBP enhanced autophagy by inhibiting the PI3K/Akt/mTOR signaling pathway. Beyond this classic pathway, Hedgehog pathway has also been implicated in abnormally activated autophagy in hypospadias rats (42). In addition, endoplasmic reticulum stress, a protective cellular stress response, was involved in the regulation of autophagy (68). Activation of endoplasmic reticulum stress induced by DBP has been proved to enhance autophagy through phosphorylation of eIF2α (44). However, NONRATT008453.2, a long non-coding RNA inversely associated with cellular autophagy, was found to be overexpressed under DBP induction in GT fibroblasts from hypospadias rats (35). We propose that a possible explanation is that this overexpression may represent a negative feedback regulatory mechanism by which the organism responds to DBP-induced autophagy in GTs.
Apoptosis is a process of programmed cell death, actively triggered by the cell itself through appropriate means, and it plays a crucial role in maintaining cellular homeostasis in the organism (69). Early research suggested that apoptosis was involved in the morphogenesis and development of GTs, and that abnormal apoptotic function could cause developmental disorders such as hypospadias (70–72). Consistently, measurement of GTs in phthalate-treated hypospadias rats using the TUNEL assay showed a significant decrease in the number of apoptotic cells (33, 44). However, we observed interesting differences in the regulation of apoptosis across various penile tissues induced by phthalates. In urethral epithelial cells, Tian et al. (24) found that miR-494, overexpressed in neonatal hypospadias rats, promoted proliferation and inhibited apoptosis. Upregulation of the PERK/eIF2α signaling pathway has also been reported to attenuate the apoptotic activity of GT fibroblasts in male hypospadias fetal rats (44). Contrary to these findings, Zhou et al. (38) demonstrated that activation of the Akt/Bax/caspase-3 pathway increased apoptosis in corpus cavernosum of 10-week-old hypospadias rats. Furthermore, they confirmed that this is one of the significant causes of erectile dysfunction in rats with hypospadias. Integrating these results, we propose that the regulation of apoptosis by phthalates exhibits tissue-specificity during penile development, and that apoptotic changes varying across different tissues collectively contribute to penile morphological abnormalities and functional impairments. This complex mechanism suggests that merely restoring or inhibiting apoptosis may not adequately counteract the effects of phthalates on penile development.
Angiogenesis is the development of new vascular networks from existing vessels, and its role in embryonic development is increasingly emphasized (73). Both molecular and epidemiological studies have indicated that the penile tissues in children with hypospadias exhibit characteristics of vascular dysfunction (74). In cases of hypospadias, there is abnormal development of the arterial vessels in the foreskin, including the loss of major axial vessels (75). Moreover, penile dartos vessels in hypospadias are distinguished from the normal condition by the presence of small-sized axial ventrolateral vessels and the possible absence of median superior vessels (76). It has been demonstrated that the expression of TGFB1I1 and other pro-angiogenic growth factors was significantly reduced in the GT of DBP-induced hypospadias rats, ultimately resulting in impaired angiogenic capacity (41). Additionally, decreased expression of eNOS and nNOS was observed in DBP-induced hypospadias rats (38). As nitric oxide synthases, eNOS and nNOS exert important vasodilatory effects in normal vascular physiology (77). Meanwhile, endothelial dysfunction was also thought to result from the reduction in eNOS and nNOS (78). The most direct consequence of impaired angiogenesis is the limitation of gas exchange and nutrient supply. However, the specific link between these adverse outcomes and the incidence of hypospadias remains under-researched. We believe that future investigations into this area will enhance our understanding of the relationship between vascular dysfunction and hypospadias.
In conducting this systematic review, we have further analyzed multiple mechanisms examined in the included studies. This approach has revealed a significant insight: the five mechanisms identified as potential contributors to the phthalate-induced hypospadias are not isolated from each other, instead, there are intriguing interconnections between them. Of particular interest is the sex steroids-related pathways, as they have the capacity to impact other mechanisms (Figure 7). Impaired androgen signaling not only induced enhanced autophagy but also inhibited angiogenesis (34, 38, 41). Furthermore, AR downregulation and ERα upregulation might collectively cause a decrease in EMT (37). This effect could stem from disrupted sex steroids-related pathways impacting the expression of certain genes, such as Rab25, which, as previously mentioned, is closely associated with hypospadias and can regulate EMT through integrin trafficking (79, 80). Although no direct relationship between sex steroids and apoptosis was found in the studies included in this paper, the decline in androgen levels has been shown to promote apoptosis in prostate tissue (81). Additionally, there exists a complex interaction between autophagy and apoptosis. Several studies have found that DBP-induced autophagy can inhibit apoptotic activity to some extent (33, 44). The possible reason for this lied in the pro-survival mechanism of autophagy, which may alleviate endoplasmic reticulum load and resist apoptosis in normal cells (82, 83). On the other hand, over-activated autophagy has also been suggested to play a cytotoxic role in conjunction with apoptosis (84). Therefore, abnormal sex steroids levels may also potentially influence the level of apoptosis during penile development through autophagy. In summary, while phthalates pose a threat to multiple functions during penile development, the sex steroids-related pathways appear to be central, potentially acting as the primary regulatory factor for other functional alterations.
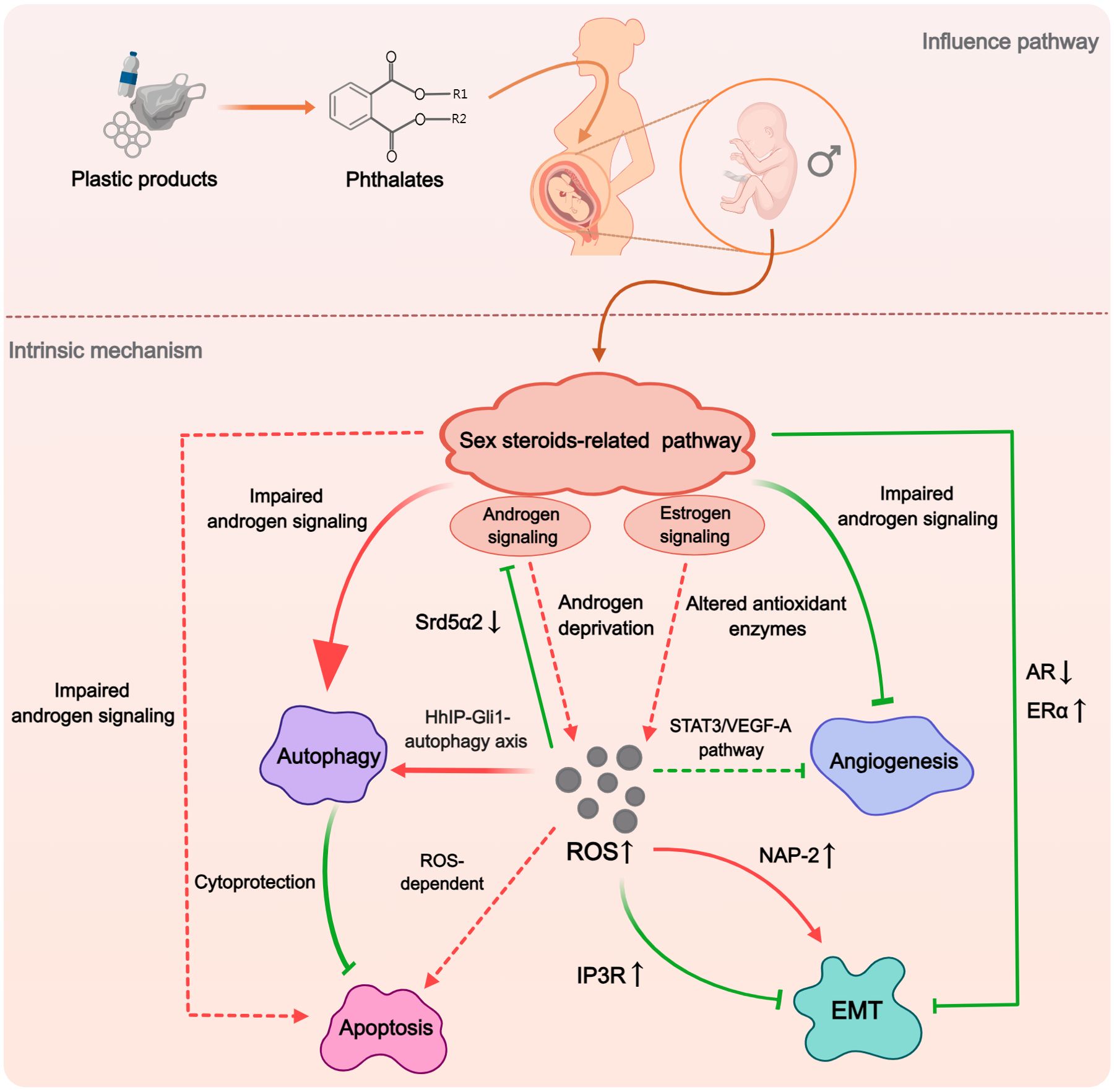
Figure 7. The hypothesized macro-mechanism by which phthalates induce hypospadias. Phthalates would initially impact the sex steroid pathway, which plays the dominant role. The impaired androgen signaling and enhanced estrogen signaling could influence the expression and activity of autophagy, apoptosis, angiogenesis, and EMT. ROS may act as an important mediator in this process, with abnormally elevated ROS participating in the regulation of various mechanisms through different pathways. (Solid arrows represent mechanisms that have been elucidated in the included study). Dashed arrows indicate mechanisms cited from related studies that were not included in this systematic review).
ROS is a generic term for a class of unstable oxygen-containing chemicals associated with oxygen metabolism (85). They play a complex role in organisms, not only causing oxidative damage to cells or tissues, but also acting as signaling molecules (86). The ability of phthalates to increase ROS production and inhibit antioxidant enzyme activity has been demonstrated in numerous experiments (87, 88). Through literature review, we found that ROS may serve as a critical mediator in the phthalate-induced hypospadias (Figure 7). In the studies included in this review, ROS has been closely associated with impaired androgen signaling pathways, upregulation of autophagy, and disruption of the EMT process (26, 39, 42, 43). Although no studies have specifically explored the association between ROS and either apoptosis or angiogenesis in phthalate-induced hypospadias, oxidative stress has been demonstrated to induce cellular apoptosis through both mitochondria-dependent and mitochondria-independent pathways across various cell types (89). Regarding angiogenesis, low concentrations of ROS in cells were considered as promoting factors for angiogenesis, however, high levels of ROS in mitochondria could inhibit the STAT3/VEGF-A pathway to reduce angiogenesis (90, 91). Interestingly, the sex steroids-related pathways may not only serve as the initiating factor for other mechanisms, as previously hypothesized, but can also be a crucial route by which phthalates increase ROS production. On the one hand, in a study of cardiomyocytes, testosterone deficiency was found to induce oxidative stress (92). On the other hand, estrogen signaling appeared to exert pro-oxidant effects, with ER potentially serving as the upstream signal that promotes ROS generation (93, 94).
Based on our findings, we propose a plausible macroscopic mechanism by which phthalates induce hypospadias. When phthalates enter the fetal body during the period of sexual differentiation, they would exert estrogen-like properties. They initially activate the estrogen signaling pathway while inhibiting the androgen signaling pathway. This alteration in sex steroids-related pathways stimulates the production of ROS in the GT. Elevated ROS levels further suppress the androgen signaling pathway during genital development and promote cellular autophagy. The impact on cellular apoptosis is tissue-specific; in epithelial tissues, increased autophagy exerts a cytoprotective effect inhibiting apoptosis, whereas in corpus spongiosum tissues, the pro-apoptotic effects of ROS may predominate, leading to increased cell death. Meanwhile, ROS also activates signaling pathways that antagonize the normal process of EMT and inhibit angiogenesis during penile development. This multifunctional abnormality during penile development, triggered by sex steroids-related pathways with ROS acting as the mediator, ultimately leads to the occurrence of hypospadias. We propose that this comprehensive perspective offers novel insights into the prevention of hypospadias induced by phthalates. Firstly, the most critical aspect is to restore the balance in the sex steroids levels disrupted by phthalates, as alterations in sex steroids-related pathways are the primary initiators of other functional abnormalities associated with hypospadias. Designing intervention strategies focused on the estrogen-like properties of phthalates is likely the most fundamental approach. Simultaneously, another potentially more feasible key strategy involves reducing the production of ROS induced by phthalates, since elevated ROS levels are crucial mediators of various functional abnormalities caused by phthalates. It is noteworthy that maternal intake of certain antioxidants, such as folic acid and vitamins, during pregnancy has been shown in retrospective studies and animal research to reduce the incidence of hypospadias (95, 96). However, larger-scale clinical studies are still needed to substantiate the preventive effects of antioxidants on hypospadias.
Secondly, based on the findings of our literature review, we recommend that future animal experiments on phthalate-induced hypospadias should place greater emphasis on the timing of sample collection. On the one hand, studies have demonstrated that the effects of phthalates on EMT during penile development vary depending on the timing of tissue sampling. This difference might be attributed to the continued postnatal development of the penis in rats, but it also implies that the influence of phthalates may be time-specific. On the other hand, we discovered that phthalates may regulate apoptosis in cells from different penile tissues with tissue specificity. If sampling is done during embryonic development, the various penile tissues in rats have not yet fully developed and are difficult to distinguish, making it easy to overlook tissue specificity in experimental results. However, if samples are collected after birth, although penile tissues have matured, since embryonic development has already concluded, we may only detect the biological abnormalities caused by phthalates, missing potential early signaling pathway changes. In summary, different sampling times each have their advantages and disadvantages. Therefore, incorporating multiple sampling points into a single study for a temporal analysis may yield significant new insights into the mechanisms by which phthalates lead to hypospadias.
Hypospadias is also considered a phenotype of Disorders of Sexual Development (DSD). DSD encompasses a range of conditions characterized by abnormal sexual differentiation, which in males, can also present as cryptorchidism and micropenis (97). Our further investigation of the existing literature reveals a significant positive correlation between phthalate exposure and the incidence of cryptorchidism, likely due to disruptions in estrogen and androgen signaling pathways (37). Additionally, ROS play a critical role; studies indicate that DEHP can cause abnormal testicular development by altering oxidative balance and modulating SIRT1/PGC1α levels (98). This testicular toxicity can be mitigated by antioxidants such as vanillic acid and vitamin C (99). Thus, the reproductive toxicity of phthalates may not be limited to the induction of hypospadias but may also exert an inductive effect on other manifestations of DSD. Research on phthalates’ role in causing micropenis is relatively scarce, which could serve as a potential direction for future studies.
5 Limitations
It is necessary to point out here the limitations of this review caused by the characteristics of the included studies. Firstly, although the keyword “phthalates” was used in the literature search, only two types of phthalates, DEHP and DBP, were employed in all the studies. Thus, the applicability of these findings to other types of phthalates requires further experimental validation. Secondly, since current in vivo experiments utilize rodent models, caution is needed when applying the conclusions of this article to explain the mechanisms by which phthalates induce hypospadias in humans, considering the species-specific differences in embryonic development.
Finally, this review has discussed only the mechanisms by which individual phthalates induce hypospadias; however, in real-world conditions, humans are often exposed to multiple EDCs (100). Other EDCs may also disrupt the pathways mentioned here, interfering with normal cellular activities. For example, finasteride can downregulate apoptosis levels (101), and vinclozolin can unbalance sex steroids-related pathways (102, 103). Therefore, concurrent exposure to phthalates and various other EDCs during pregnancy may further amplify the disruptions of the mechanisms discussed in this review, potentially increasing the risk of hypospadias, although additional research is needed to substantiate this.
6 Conclusion
This systematic review dissects the intricate mechanisms underlying phthalate-induced hypospadias revealed through rodent model studies, organizing them into five distinct categories: sex steroids-related pathways, EMT, autophagy, apoptosis, and angiogenesis. We find that phthalates may initially disrupt the balance of sex steroids-related pathways, leading to abnormally elevated levels of ROS. This escalation in ROS subsequently precipitates other functional abnormalities, ultimately resulting in the development of hypospadias. Our findings suggest that interventions such as antioxidant supplementation during pregnancy may mitigate the teratogenic effects of phthalates. Future research should expand on the types of phthalates studied and conduct longitudinal studies to enhance our understanding for phthalate-induced hypospadias.
Data availability statement
The original contributions presented in the study are included in the article/Supplementary Material. Further inquiries can be directed to the corresponding author.
Author contributions
YZ: Conceptualization, Formal analysis, Validation, Writing – original draft. JW: Data curation, Formal analysis, Validation, Writing – original draft. HY: Supervision, Writing – original draft. YG: Conceptualization, Supervision, Writing – review & editing.
Funding
The author(s) declare financial support was received for the research, authorship, and/or publication of this article. This study was supported by the Tianjin Key Medical Discipline (Specialty)Construction Project (no. TJYXZDXK-040A).
Conflict of interest
The authors declare that the research was conducted in the absence of any commercial or financial relationships that could be construed as a potential conflict of interest.
Publisher’s note
All claims expressed in this article are solely those of the authors and do not necessarily represent those of their affiliated organizations, or those of the publisher, the editors and the reviewers. Any product that may be evaluated in this article, or claim that may be made by its manufacturer, is not guaranteed or endorsed by the publisher.
Supplementary material
The Supplementary Material for this article can be found online at: https://www.frontiersin.org/articles/10.3389/fendo.2024.1490011/full#supplementary-material
Glossary
EDCs: Endocrine disrupting chemicals
DSD: Disorders of Sex Development
DEHP: Di-(2-ethylhexyl) phthalate
MEHP: Mono-(2-ethylhexyl) phthalate
DBP: Di-n-butyl phthalate
MBP: Mono-butyl phthalate
GT: Genital tubercle
BW: Body Weight
GD: Gestational day
PND: Postnatal day
PUECs: Primary urethral epithelial cells
HUVECs: Human umbilical vein endothelial cells
LncRNA: Long Non-Coding RNA
ROS: Reactive oxygen species
EMT: Epithelial-mesenchymal transition
α-SMA: Alpha-Smooth Muscle Actin
ZO-1: Zonula Occludens-1
AR: Androgen receptor
ER: Estrogen Receptor
DHT: Dihydrotestosterone
Shh: Sonic hedgehog
Ptch1: Patched 1
Bmp4/7: Bone morphogenetic protein 4/7
Fgf8/10: Fibroblast growth factor 8/10
Fgfr2: Fibroblast growth factor receptor 2
Srd5a2: Steroid 5 Alpha-Reductase 2
Hsd17b3: Hydroxysteroid 17-Beta Dehydrogenase 3
Hsd3b: Hydroxysteroid 3-Beta Dehydrogenase
Scarb1: Scavenger Receptor Class B Member 1
Star: Steroidogenic Acute Regulatory Protein
ATF4: Activating Transcription Factor 4
PFN2: Profilin 2
IP3R: Inositol 1,4,5-Trisphosphate Receptor
c-Fos: Cellular Proto-Oncogene Fos
FITC: Fluorescein isothiocyanate
TUNEL: Terminal deoxynucleotidyl transferase dUTP nick end labelling
LC3-I/II: Microtubule-Associated Protein 1 Light Chain 3 Alpha/Beta
miR-494: MicroRNA-494
Nedd4L: NEDD4 Like E3 Ubiquitin Protein Ligase
HhIP: Hedgehog Interacting Protein
Gli1/2/3: Glioma-Associated Oncogene Family Zinc Finger 1/2/3
Bcl-2: B-Cell Lymphoma 2
Bax: Bcl-2-Associated X Protein
Caspase-3: Cysteine-aspartic Acid Protease 3
eNOS: Endothelial Nitric Oxide Synthase
nNOS: Neuronal Nitric Oxide Synthase
cGMP: Cyclic Guanosine Monophosphate
RhoA: Ras Homolog Family Member A
ROCK 1/2: Rho-Associated Coiled-Coil Containing Protein Kinase 1/2
NAP-2: Neutrophil-Activating Protein 2
NOX 1/4: NADPH Oxidase 1/4
MAPK10/14: Mitogen-Activated Protein Kinase 10/14
GSK-3β: Glycogen Synthase Kinase 3 Beta
NFκB: Nuclear Factor Kappa B
Wnt5a: Wingless-Type MMTV Integration Site Family, Member 5A
Hoxa13: Homeobox A13
Hoxd13: Homeobox D13
Rab25: Ras-Related Protein Rab-25
Mafb: MAF BZIP Transcription Factor B
TGF-βrIII: Transforming growth factor beta receptor III
TGF-β1: Transforming growth factor beta 1
TGFB1I1: Transforming Growth Factor Beta 1 Induced Transcript 1
Smad2/3: Mothers Against Decapentaplegic Homolog 2/3
Cyp11a1: Cytochrome P450 Family 11 Subfamily A Member 1
Cyp17a1: Cytochrome P450 Family 17 Subfamily A Member 1
PI3K: Phosphoinositide 3-Kinase
STAT3: Signal Transducer and Activator of Transcription 3
VEGF-A: Vascular Endothelial Growth Factor A
p-Akt: Phosphorylated Protein Kinase B
p-S6: Phosphorylated Ribosomal Protein S6
p-mTOR: Phosphorylated Mechanistic Target of Rapamycin
p-eIF2α: Phosphorylated Eukaryotic Initiation Factor 2 Alpha
p-PERK: Phosphorylated Protein Kinase R-like Endoplasmic Reticulum Kinase
References
1. Diamond DA, Chan IHY, Holland AJA, Kurtz MP, Nelson C, Estrada CR, et al. Advances in paediatric urology. Lancet Lond Engl. (2017) 390:1061–71. doi: 10.1016/S0140-6736(17)32282-1
2. Van Der Horst HJR, De Wall LL. Hypospadias, all there is to know. Eur J Pediatr. (2017) 176:435–41. doi: 10.1007/s00431-017-2864-5
3. Geller F, Feenstra B, Carstensen L, Pers TH, van Rooij IALM, Körberg IB, et al. Genome-wide association analyses identify variants in developmental genes associated with hypospadias. Nat Genet. (2014) 46:957–63. doi: 10.1038/ng.3063
4. van der Zanden LFM, van Rooij IALM, Feitz WFJ, Knight J, Donders ART, Renkema KY, et al. Common variants in DGKK are strongly associated with risk of hypospadias. Nat Genet. (2011) 43:48–50. doi: 10.1038/ng.721
5. Yamada G, Satoh Y, Baskin LS, Cunha GR. Cellular and molecular mechanisms of development of the external genitalia. Differ Res Biol Divers. (2003) 71:445–60. doi: 10.1046/j.1432-0436.2003.7108001.x
6. Cunha GR, Baskin LS. Development of the external genitalia. Differ Res Biol Divers. (2020) 112:7–9. doi: 10.1016/j.diff.2019.10.008
7. Baskin L, Shen J, Sinclair A, Cao M, Liu X, Liu G, et al. Development of the human penis and clitoris. Differ Res Biol Divers. (2018) 103:74–85. doi: 10.1016/j.diff.2018.08.001
8. Govers LC, Phillips TR, Mattiske DM, Rashoo N, Black JR, Sinclair A, et al. A critical role for estrogen signaling in penis development. FASEB J Off Publ Fed Am Soc Exp Biol. (2019) 33:10383–92. doi: 10.1096/fj.201802586RR
9. Chen Y, Yu H, Pask AJ, Fujiyama A, Suzuki Y, Sugano S, et al. Hormone-responsive genes in the SHH and WNT/β-catenin signaling pathways influence urethral closure and phallus growth. Biol Reprod. (2018) 99:806–816. doi: 10.1093/biolre/ioy117
10. Harada M, Omori A, Nakahara C, Nakagata N, Akita K, Yamada G. Tissue-specific roles of FGF signaling in external genitalia development. Dev Dyn Off Publ Am Assoc Anat. (2015) 244:759–73. doi: 10.1002/dvdy.24277
11. Tarulli GA, Cripps SM, Pask AJ, Renfree MB. Spatiotemporal map of key signaling factors during early penis development. Dev Dyn Off Publ Am Assoc Anat. (2022) 251:609–24. doi: 10.1002/dvdy.433
12. Yilmaz B, Terekeci H, Sandal S, Kelestimur F. Endocrine disrupting chemicals: exposure, effects on human health, mechanism of action, models for testing and strategies for prevention. Rev Endocr Metab Disord. (2020) 21:127–47. doi: 10.1007/s11154-019-09521-z
13. Thacharodi A, Hassan S, Acharya G, Vithlani A, Hoang Le Q, Pugazhendhi A. Endocrine disrupting chemicals and their effects on the reproductive health in men. Environ Res. (2023) 236:116825. doi: 10.1016/j.envres.2023.116825
14. Antony S, Antony S, Rebello S, George S, Biju DT, RR, et al. Bioremediation of endocrine disrupting chemicals- advancements and challenges. Environ Res. (2022) 213:113509. doi: 10.1016/j.envres.2022.113509
15. Mariana M, Feiteiro J, Verde I, Cairrao E. The effects of phthalates in the cardiovascular and reproductive systems: A review. Environ Int. (2016) 94:758–76. doi: 10.1016/j.envint.2016.07.004
16. Trasande L, Sargis RM. Endocrine-disrupting chemicals: mainstream recognition of health effects and implications for the practicing internist. J Intern Med. (2024) 295:259–74. doi: 10.1111/joim.13748
17. Tuan Tran H, Lin C, Bui X-T, Ky Nguyen M, Dan Thanh Cao N, Mukhtar H, et al. Phthalates in the environment: characteristics, fate and transport, and advanced wastewater treatment technologies. Bioresour Technol. (2022) 344:126249. doi: 10.1016/j.biortech.2021.126249
18. Suzuki Y, Yoshinaga J, Mizumoto Y, Serizawa S, Shiraishi H. Foetal exposure to phthalate esters and anogenital distance in male newborns. Int J Androl. (2012) 35:236–44. doi: 10.1111/j.1365-2605.2011.01190.x
19. Daniel S, Balalian AA, Whyatt RM, Liu X, Rauh V, Herbstman J, et al. Perinatal phthalates exposure decreases fine-motor functions in 11-year-old girls: results from weighted quantile sum regression. Environ Int. (2020) 136:105424. doi: 10.1016/j.envint.2019.105424
20. Kalfa N, Paris F, Philibert P, Orsini M, Broussous S, Fauconnet-Servant N, et al. Is hypospadias associated with prenatal exposure to endocrine disruptors? A french collaborative controlled study of a cohort of 300 consecutive children without genetic defect. Eur Urol. (2015) 68:1023–30. doi: 10.1016/j.eururo.2015.05.008
21. Das D, Dutta HK, Borbora D, Brahma RC, Das JM. Assessing the relationship between hypospadias risk and parental occupational exposure to potential endocrine-disrupting chemicals. Occup Environ Med. (2023) 80:93–6. doi: 10.1136/oemed-2022-108594
22. Page MJ, McKenzie JE, Bossuyt PM, Boutron I, Hoffmann TC, Mulrow CD, et al. The PRISMA 2020 statement: an updated guideline for reporting systematic reviews. Syst Rev. (2021) 10:89. doi: 10.1186/s13643-021-01626-4
23. Liu X, He D-W, Zhang D-Y, Lin T, Wei G-H. Di(2-ethylhexyl) phthalate (DEHP) increases transforming growth factor-β1 expression in fetal mouse genital tubercles. J Toxicol Environ Health A. (2008) 71:1289–94. doi: 10.1080/15287390802114915
24. Tian R-H, Guo K-M, Han G-H, Bai Y. Downregulation of microRNA-494 inhibits the TGF-β1/smads signaling pathway and prevents the development of hypospadias through upregulating nedd4L. Exp Mol Pathol. (2020) 115:104452. doi: 10.1016/j.yexmp.2020.104452
25. Kim TS, Jung KK, Kim SS, Kang IH, Baek JH, Nam H-S, et al. Effects of in utero exposure to DI(n -butyl) phthalate on development of male reproductive tracts in sprague-dawley rats. J Toxicol Environ Health A. (2010) 73:1544–59. doi: 10.1080/15287394.2010.511579
26. Shi B, He E, Chang K, Xu G, Meng Q, Xu H, et al. Genistein prevents the production of hypospadias induced by di-(2-ethylhexyl) phthalate through androgen signaling and antioxidant response in rats. J Hazard Mater. (2024) 466:133537. doi: 10.1016/j.jhazmat.2024.133537
27. Zhou Y, Huang F, Liu Y, Li D, Zhou Y, Shen L, et al. TGF-β1 relieves epithelial–mesenchymal transition reduction in hypospadias induced by DEHP in rats. Pediatr Res. (2020) 87:639–46. doi: 10.1038/s41390-019-0622-2
28. Zhu Y-J, Jiang J-T, Ma L, Zhang J, Hong Y, Liao K, et al. Molecular and toxicologic research in newborn hypospadiac male rats following in utero exposure to di-n-butyl phthalate (DBP). Toxicology. (2009) 260:120–5. doi: 10.1016/j.tox.2009.03.017
29. Zhang L-F, Qin C, Wei Y-F, Wang Y, Chang J-K, Mi Y-Y, et al. Differential expression of the wnt/β-catenin pathway in the genital tubercle (GT) of fetal male rat following maternal exposure to di-n-butyl phthalate (DBP). Syst Biol Reprod Med. (2011) 57:244–50. doi: 10.3109/19396368.2011.577509
30. Liu S-B, Ma Z, Sun W-L, Sun X-W, Hong Y, Ma L, et al. The role of androgen-induced growth factor (FGF8) on genital tubercle development in a hypospadiac male rat model of prenatal exposure to di-n-butyl phthalate. Toxicology. (2012) 293:53–8. doi: 10.1016/j.tox.2011.12.010
31. Jiang J-T, Zhong C, Zhu Y-P, Xu D-L, Wood K, Sun W, et al. Prenatal exposure to di-n-butyl phthalate (DBP) differentially alters androgen cascade in undeformed versus hypospadiac male rat offspring. Reprod Toxicol. (2016) 61:75–81. doi: 10.1016/j.reprotox.2016.02.016
32. Zhu Y-P, Li E-H, Sun W-L, Xu D-L, Liu Z-H, Zhao W, et al. Maternal exposure to di-n-butyl phthalate (DBP) induces combined anorectal and urogenital malformations in male rat offspring. Reprod Toxicol. (2016) 61:169–76. doi: 10.1016/j.reprotox.2016.04.007
33. Li X, Li J, Zhang Y, Zhou Y. Di-n-butyl phthalate induced hypospadias relates to autophagy in genital tubercle via the PI3K/akt/mTOR pathway. J Occup Health. (2017) 59:8–16. doi: 10.1539/joh.16-0089-OA
34. Zhao S, Li D, Bei X-Y, Zhu Y-P, Sun W-L, Shen C, et al. Maternal exposure to di-n-butyl phthalate (DBP) promotes epithelial-mesenchymal transition via regulation of autophagy in uroepithelial cell. Toxicology. (2018) 406–407:114–22. doi: 10.1016/j.tox.2018.07.013
35. Feng X, Huang E, Gao Y, Zhang Y, Zhou Y. The effects of NONRATT008453.2 on autophagy in genital tubercle fibroblasts of rats with hypospadias induced by dibutyl phthalate. Birth Defects Res. (2021) 113:399–408. doi: 10.1002/bdr2.1863
36. Xiang H, Wang S, Kong X, Yu Y, Shen L, Long C, et al. C-fos is upregulated in the genital tubercle of DEHP-induced hypospadiac rats and the prepuce of patients with hypospadias. Syst Biol Reprod Med. (2021) 67:193–200. doi: 10.1080/19396368.2020.1862356
37. Zhou X, Zhang X, Zhou X, Abulimiti G, Wang Y, Zhang Q, et al. Identification of endocrine-disrupting chemicals targeting the genes and pathways of genital anomalies in males. Ecotoxicol Environ Saf. (2022) 247:114241. doi: 10.1016/j.ecoenv.2022.114241
38. Zhou X, Wang S, Zhou R, Zhang T, Wang Y, Zhang Q, et al. Erectile dysfunction in hypospadiac male adult rats induced by maternal exposure to di-n-butyl phthalate. Toxicology. (2022) 475:153227. doi: 10.1016/j.tox.2022.153227
39. Hua S, Shi F, Xie Z, Wu L, Dai M, Zhang Y, et al. Di-n-butyl phthalate induces oversecretion of vascular endothelium-derived NAP-2 and promotes epithelial-mesenchymal transition of urothelial cells in newborn hypospadias rats. Ecotoxicol Environ Saf. (2023) 256:114892. doi: 10.1016/j.ecoenv.2023.114892
40. Zhu S, Tang X, Zhang J, Hu J, Gao X, Li D, et al. Urinary extracellular vesicles prevent di-(2-ethylhexyl) phthalate-induced hypospadias by facilitating epithelial-mesenchymal transition via PFN2 delivery. Cell Biol Toxicol. (2023) 39:2569–86. doi: 10.1007/s10565-023-09838-1
41. Wu L, Shi F, Zhang Y, Xu X, Xie Z, Hua S, et al. Maternal exposure to dibutyl phthalate (DBP) impairs angiogenesis and AR signalling pathway through suppression of TGFB1I1 in hypospadias offspring. Ecotoxicol Environ Saf. (2024) 270:115941. doi: 10.1016/j.ecoenv.2024.115941
42. Zhao S, Pan L, Chen M, Zhu Y-P, Han B-M, Xia S-J, et al. Di-n-butyl phthalate induced autophagy of uroepithelial cells via inhibition of hedgehog signaling in newborn male hypospadias rats. Toxicology. (2019) 428:152300. doi: 10.1016/j.tox.2019.152300
43. Zhu Y-P, Zhao W, Sun W-L, Zhao S, Chen M, Pan L, et al. Di-n-Butyl Phthalate (DBP) Reduces Epithelial-Mesenchymal Transition via IP3R in Hypospadias during Maternal Exposure. Ecotoxicol Environ Saf. (2020) 192:110201. doi: 10.1016/j.ecoenv.2020.110201
44. Zhao Z, Liu G, Fu W. Role of PERK-eIF2α Signaling pathway in fetal male rats with hypospadias induced by di-n-butyl phthalate. Kaohsiung J Med Sci. (2018) 34:487–93. doi: 10.1016/j.kjms.2018.04.002
45. Foster WG, Evans JA, Little J, Arbour L, Moore A, Sauve R, et al. Human exposure to environmental contaminants and congenital anomalies: A critical review. Crit Rev Toxicol. (2017) 47:59–84. doi: 10.1080/10408444.2016.1211090
46. Cunha GR, Liu G, Sinclair A, Cao M, Glickman S, Cooke PS, et al. Androgen-independent events in penile development in humans and animals. Differ Res Biol Divers. (2020) 111:98–114. doi: 10.1016/j.diff.2019.07.005
47. Ghayee HK, Auchus RJ. Clinical implications of androgen synthesis via 5alpha-reduced precursors. Endocr Dev. (2008) 13:55–66. doi: 10.1159/000134780
48. Ipulan-Colet LA. Sexual dimorphism through androgen signaling; from external genitalia to muscles. Front Endocrinol. (2022) 13:940229. doi: 10.3389/fendo.2022.940229
49. Saraç M, Canpolat Ş, Önalan Etem E, Tektemur A, Tartar T, Bakal U, et al. The role of sonic hedgehog homologue signal pathway in hypospadias aetiology. J Pediatr Urol. (2021) 17:630.e1–7. doi: 10.1016/j.jpurol.2021.06.025
50. Beleza-Meireles A, Lundberg F, Lagerstedt K, Zhou X, Omrani D, Frisén L, et al. FGFR2, FGF8, FGF10 and BMP7 as candidate genes for hypospadias. Eur J Hum Genet. (2007) 15:405–10. doi: 10.1038/sj.ejhg.5201777
51. Kong X, Luo J, Xiang H, Wang S, Shen L, Long C, et al. Expression of mafb is down-regulated in the foreskin of children with hypospadias. J Pediatr Urol. (2021) 17:70.e1–6. doi: 10.1016/j.jpurol.2020.10.006
52. Zhang Q, Zhang Z, He X, Liu Z, Shen L, Long C, et al. Expression of rab25 is down-regulated in the foreskin of children with hypospadias. J Pediatr Urol. (2023) 19:581.e1–6. doi: 10.1016/j.jpurol.2023.05.006
53. Baskin L, Sinclair A, Derpinghaus A, Cao M, Li Y, Overland M, et al. Estrogens and development of the mouse and human external genitalia. Differ Res Biol Divers. (2021) 118:82–106. doi: 10.1016/j.diff.2020.09.004
54. Kalluri R, Weinberg RA. The basics of epithelial-mesenchymal transition. J Clin Invest. (2009) 119:1420–8. doi: 10.1172/JCI39104
55. Thiery JP, Acloque H, Huang RYJ, Nieto MA. Epithelial-mesenchymal transitions in development and disease. Cell. (2009) 139:871–90. doi: 10.1016/j.cell.2009.11.007
56. Kurzrock EA, Baskin LS, Cunha GR. Ontogeny of the male urethra: theory of endodermal differentiation. Differ Res Biol Divers. (1999) 64:115–22. doi: 10.1046/j.1432-0436.1999.6420115.x
57. Baskin LS, Lee YT, Cunha GR. Neuroanatomical ontogeny of the human fetal penis. Br J Urol. (1997) 79:628–40. doi: 10.1046/j.1464-410x.1997.00119.x
58. Baskin L, Erol A, Jegatheesan P, Li Y, Liu W, Cunha G. Urethral seam formation and hypospadias. Cell Tissue Res. (2001) 305:379–87. doi: 10.1007/s004410000345
59. Zhou Y, Liu X, Huang F, Liu Y, Cao X, Shen L, et al. Epithelial–mesenchymal transformation and apoptosis in rat urethra development. Pediatr Res. (2017) 82:1073–9. doi: 10.1038/pr.2017.185
60. Chen M, Wu G-B, Hua S, Zheng L, Fan Q, Luo M. Dibutyl phthalate (DBP) promotes epithelial-mesenchymal transition (EMT) to aggravate liver fibrosis into cirrhosis and portal hypertension (PHT) via ROS/TGF-β1/snail-1 signalling pathway in adult rats. Ecotoxicol Environ Saf. (2024) 274:116124. doi: 10.1016/j.ecoenv.2024.116124
61. Shih M-S, Suk F-M, Chiu W-C, Lee C-Y, Hsu F-Y, Liao Y-J. Long-term di-(2-ethylhexyl) phthalate exposure reduces sorafenib treatment efficacy by enhancing mesenchymal transition in hepatocellular carcinoma. Ecotoxicol Environ Saf. (2024) 273:116161. doi: 10.1016/j.ecoenv.2024.116161
62. Zhang J, van der Zon G, Ma J, Mei H, Cabukusta B, Agaser CC, et al. ST3GAL5-catalyzed gangliosides inhibit TGF-β-induced epithelial-mesenchymal transition via TβRI degradation. EMBO J. (2023) 42:e110553. doi: 10.15252/embj.2021110553
63. Xu J, Lamouille S, Derynck R. TGF-β-induced epithelial to mesenchymal transition. Cell Res. (2009) 19:156–72. doi: 10.1038/cr.2009.5
64. Cunha GR, Sinclair A, Risbridger G, Hutson J, Baskin LS. Current understanding of hypospadias: relevance of animal models. Nat Rev Urol. (2015) 12:271–80. doi: 10.1038/nrurol.2015.57
65. Parzych KR, Klionsky DJ. An overview of autophagy: morphology, mechanism, and regulation. Antioxid Redox Signal. (2014) 20:460–73. doi: 10.1089/ars.2013.5371
66. Mizushima N, Levine B. Autophagy in mammalian development and differentiation. Nat Cell Biol. (2010) 12:823–30. doi: 10.1038/ncb0910-823
67. Li L, Tan J, Miao Y, Lei P, Zhang Q. ROS and autophagy: interactions and molecular regulatory mechanisms. Cell Mol Neurobiol. (2015) 35:615–21. doi: 10.1007/s10571-015-0166-x
68. Bhardwaj M, Leli NM, Koumenis C, Amaravadi RK. Regulation of autophagy by canonical and non-canonical ER stress responses. Semin Cancer Biol. (2020) 66:116–28. doi: 10.1016/j.semcancer.2019.11.007
69. D’Arcy MS. Cell death: a review of the major forms of apoptosis, necrosis and autophagy. Cell Biol Int. (2019) 43:582–92. doi: 10.1002/cbin.11137
70. Haanen C, Vermes I. Apoptosis: programmed cell death in fetal development. Eur J Obstet Gynecol Reprod Biol. (1996) 64:129–33. doi: 10.1016/0301-2115(95)02261-9
71. Morgan EA, Nguyen SB, Scott V, Stadler HS. Loss of bmp7 and fgf8 signaling in hoxa13-mutant mice causes hypospadia. Development. (2003) 130:3095–109. doi: 10.1242/dev.00530
72. Suzuki K, Bachiller D, Chen YP, Kamikawa M, Ogi H, Haraguchi R, et al. Regulation of outgrowth and apoptosis for the terminal appendage: external genitalia: development by concerted actions of BMP signaling. Development. (2003) 130:6209–20. doi: 10.1242/dev.00846
73. Le Noble F, Kupatt C. Interdependence of angiogenesis and arteriogenesis in development and disease. Int J Mol Sci. (2022) 23:3879. doi: 10.3390/ijms23073879
74. Lucas-Herald AK, Montezano AC, Alves-Lopes R, Haddow L, Alimussina M, O’Toole S, et al. Vascular dysfunction and increased cardiovascular risk in hypospadias. Eur Heart J. (2022) 43:1832–45. doi: 10.1093/eurheartj/ehac112
75. Perovic SV, Radojicic ZI. Vascularization of the hypospadiac prepuce and its impact on hypospadias repair. J Urol. (2003) 169:1098–101. doi: 10.1097/01.ju.0000052820.35946.99
76. Gupta A, Sunil K, Singh GP, Kureel S. Study of variations in axial pattern vessels of penile dartos in hypospadias and implied surgical significance. Urology. (2020) 146:201–6. doi: 10.1016/j.urology.2020.08.016
77. Capettini LSA, Cortes SF, Lemos VS. Relative Contribution of eNOS and nNOS to Endothelium-Dependent Vasodilation in the Mouse Aorta. Eur J Pharmacol. (2010) 643:260–6. doi: 10.1016/j.ejphar.2010.06.066
78. Forstermann U, Sessa WC. Nitric oxide synthases: regulation and function. Eur Heart J. (2012) 33:829–37. doi: 10.1093/eurheartj/ehr304
79. Stenmark H. Rab GTPases as coordinators of vesicle traffic. Nat Rev Mol Cell Biol. (2009) 10:513–25. doi: 10.1038/nrm2728
80. Yin C, Mou Q, Pan X, Zhang G, Li H, Sun Y. MiR-577 suppresses epithelial-mesenchymal transition and metastasis of breast cancer by targeting rab25. Thorac Cancer. (2018) 9:472–9. doi: 10.1111/1759-7714.12612
81. Joseph DB, Henry GH, Malewska A, Reese JC, Mauck RJ, Gahan JC, et al. 5-alpha reductase inhibitors induce a prostate luminal to club cell transition in human benign prostatic hyperplasia. J Pathol. (2022) 256:427–41. doi: 10.1002/path.5857
82. Li M, Yang X, Wang H, Xu E, Xi Z. Inhibition of androgen induces autophagy in benign prostate epithelial cells. Int J Urol. (2014) 21:195–9. doi: 10.1111/iju.12210
83. Gordy C, He Y-W. The crosstalk between autophagy and apoptosis: where does this lead? Protein Cell. (2012) 3:17–27. doi: 10.1007/s13238-011-1127-x
84. Sun Y, Shen J, Zeng L, Yang D, Shao S, Wang J, et al. Role of autophagy in di-2-ethylhexyl phthalate (DEHP)-induced apoptosis in mouse leydig cells. Environ pollut Barking Essex 1987. (2018) 243:563–72. doi: 10.1016/j.envpol.2018.08.089
85. Yang S, Lian G. ROS and diseases: role in metabolism and energy supply. Mol Cell Biochem. (2020) 467:1–12. doi: 10.1007/s11010-019-03667-9
86. D’Autréaux B, Toledano MB. ROS as signalling molecules: mechanisms that generate specificity in ROS homeostasis. Nat Rev Mol Cell Biol. (2007) 8:813–24. doi: 10.1038/nrm2256
87. Cui Y, Li B, Du J, Huo S, Song M, Shao B, et al. Dibutyl phthalate causes MC3T3-E1 cell damage by increasing ROS to promote the PINK1/parkin-mediated mitophagy. Environ Toxicol. (2022) 37:2341–53. doi: 10.1002/tox.23600
88. Huang Y, Wu C, Ye Y, Zeng J, Zhu J, Li Y, et al. The increase of ROS caused by the interference of DEHP with JNK/P38/P53 pathway as the reason for hepatotoxicity. Int J Environ Res Public Health. (2019) 16:356. doi: 10.3390/ijerph16030356
89. Sinha K, Das J, Pal PB, Sil PC. Oxidative stress: the mitochondria-dependent and mitochondria-independent pathways of apoptosis. Arch Toxicol. (2013) 87:1157–80. doi: 10.1007/s00204-013-1034-4
90. Wang Z, Yang J, Qi J, Jin Y, Tong L. Activation of NADPH/ROS pathway contributes to angiogenesis through JNK signaling in brain endothelial cells. Microvasc Res. (2020) 131:104012. doi: 10.1016/j.mvr.2020.104012
91. Hu C, Wu Z, Huang Z, Hao X, Wang S, Deng J, et al. Nox2 impairs VEGF-A-induced angiogenesis in placenta via mitochondrial ROS-STAT3 pathway. Redox Biol. (2021) 45:102051. doi: 10.1016/j.redox.2021.102051
92. Zhang L, Wu S, Ruan Y, Hong L, Xing X, Lai W. Testosterone suppresses oxidative stress via androgen receptor-independent pathway in murine cardiomyocytes. Mol Med Rep. (2011) 4:1183–8. doi: 10.3892/mmr.2011.539
93. Fan P, Griffith OL, Agboke FA, Anur P, Zou X, McDaniel RE, et al. c-src modulates estrogen-induced stress and apoptosis in estrogen-deprived breast cancer cells. Cancer Res. (2013) 73:4510–20. doi: 10.1158/0008-5472.CAN-12-4152
94. Mobley JA, Brueggemeier RW. Estrogen receptor-mediated regulation of oxidative stress and DNA damage in breast cancer. Carcinogenesis. (2004) 25:3–9. doi: 10.1093/carcin/bgg175
95. Mavrogenis S, Urban R, Czeizel AE, Ács N. Possible preventive effect of high doses of folic acid for isolated hypospadias: A national population-based case-control study. Am J Med Genet A. (2014) 164A:3108–14. doi: 10.1002/ajmg.a.36781
96. Adrien N, Orta OR, Nestoridi E, Carmichael SL, Yazdy MM, National Birth Defects Prevention Study. Early pregnancy vitamin D status and risk of select congenital anomalies in the national birth defects prevention study. Birth Defects Res. (2023) 115:290–301. doi: 10.1002/bdr2.2101
97. Sandberg DE, Gardner M. Differences/disorders of sex development: medical conditions at the intersection of sex and gender. Annu Rev Clin Psychol. (2022) 18:201–31. doi: 10.1146/annurev-clinpsy-081219-101412
98. Li Q, Zhu Q, Tian F, Li J, Shi L, Yu Y, et al. In utero di-(2-ethylhexyl) phthalate-induced testicular dysgenesis syndrome in male newborn rats is rescued by taxifolin through reducing oxidative stress. Toxicol Appl Pharmacol. (2022) 456:116262. doi: 10.1016/j.taap.2022.116262
99. Ogunlade B, Gbotolorun SC, Adedotun OA, Iteire K, Adejayi J. Vanillic acid and vitamin C attenuated di-2-ethylhexyl phthalate-induced testicular toxicity in adult male rats. Reprod Fertil. (2022) 3:220–30. doi: 10.1530/RAF-22-0045
100. Mattiske DM, Pask AJ. Endocrine disrupting chemicals in the pathogenesis of hypospadias; developmental and toxicological perspectives. Curr Res Toxicol. (2021) 2:179–91. doi: 10.1016/j.crtox.2021.03.004
101. An N, Peng J, He G, Fan X, Li F, Chen H. Involvement of activation of mitogen-activated protein kinase (MAPK)/extracellular signal-regulated kinase (ERK) signaling pathway in proliferation of urethral plate fibroblasts in finasteride-induced rat hypospadias. Med Sci Monit Int Med J Exp Clin Res. (2018) 24:8984–92. doi: 10.12659/MSM.911271
102. Yu H, Yang J, Zhang Y, Fu H, Yan Z, Zhu Y. Vinclozolin-Induced Mouse Penile Malformation and “Small Testis” via miR132, miR195a Together with the Hippo Signaling Pathway. Toxicology. (2021) 460:152842. doi: 10.1016/j.tox.2021.152842
Keywords: phthalates, hypospadias, molecular mechanisms, sex steroids, ROS
Citation: Zhang Y, Wang J, Yang H and Guan Y (2024) The potential mechanisms underlying phthalate-induced hypospadias: a systematic review of rodent model studies. Front. Endocrinol. 15:1490011. doi: 10.3389/fendo.2024.1490011
Received: 02 September 2024; Accepted: 18 November 2024;
Published: 04 December 2024.
Edited by:
Lawrence Merle Nelson, Mary Elizabeth Conover Foundation, Inc., United StatesReviewed by:
Yifan Hong, Chongqing Medical University, ChinaAlka Gupta, University of California, Santa Cruz, United States
Copyright © 2024 Zhang, Wang, Yang and Guan. This is an open-access article distributed under the terms of the Creative Commons Attribution License (CC BY). The use, distribution or reproduction in other forums is permitted, provided the original author(s) and the copyright owner(s) are credited and that the original publication in this journal is cited, in accordance with accepted academic practice. No use, distribution or reproduction is permitted which does not comply with these terms.
*Correspondence: Yong Guan, Z3Vhbnlvbmd5aXNoZW5nQDE2My5jb20=
†These authors have contributed equally to this work and share first authorship