- 1Department of Endocrinology and Metabolism, The Second Affiliated Hospital of Nanchang University, Nanchang, China
- 2The First Clinical Medicine School, Nanchang University, Nanchang, China
- 3The Second Clinical Medicine School, Nanchang University, Nanchang, China
Background: Osteocalcin has attracted attention for its potential role in diabetes management. However, there has been no bibliometric assessment of scientific progress in this field.
Methods: We analysed 1680 articles retrieved from the Web of Science Core Collection (WoSCC) between 1 January 1986 and 10 May 2024 using various online tools.
Result: These papers accumulated 42,714 citations,with an average of 25.43 citations per paper. Publication output increased sharply from 1991 onwards. The United States and China are at the forefront of this research area.
Discussion: The keywords were grouped into four clusters: ‘Differential and functional osteocalcin genes’, ‘Differential expression of osteocalcin genes in relation to diabetes mellitus’, ‘Role of osteocalcin in the assessment of osteoporosis and diabetes mellitus’, and ‘Indirect involvement of osteocalcin in metabolic processes’. Analysis using the VoS viewer suggests a shift in research focus towards the correlation between osteocalcin levels and diabetic complications, the clinical efficacy of therapeutic agents or vitamins in the treatment of osteoporosis in diabetic patients, and the mechanisms by which osteocalcin modulates insulin action. The proposed focus areas are “osteocalcin genes”, “insulin regulation and osteoporosis “, “different populations”, “diabetes-related complications” and “type 2 diabetes mellitus”,“effect of osteocalcin expression on insulin sensitivity as well as secretion”,“osteocalcin expression in different populations of diabetic patients and treatment-related studies”.
Introduction
Diabetes mellitus is a chronic metabolic disease characterised by a disorder of glucose metabolism resulting from the consistent presence of beta-cell dysfunction leading to insufficient insulin secretion, reduced insulin sensitivity, or both. In 2021, there were 529 million(95% uncertainty interval [UI] 500-564) people with diabetes globally, with an overall global age-standardised prevalence of diabetes of 6-1%(5-8-6-5), and a global age-standardised prevalence of diabetes of 6-1%(5-8 -6-5), representing a major public health challenge (1).
In addition to the most common secondary complications of diabetes including diabetic retinopathy, nephropathy, neuropathy, and cardiovascular disease (2), bone disorders are frequently diagnosed, and thus diabetes is classified as a bone-related disease. It is characterised by altered bone mineral density(BMD), abnormal bone metabolism and microarchitecture, and reduced bone strength (3).
Recent studies have suggested that osteocalcin may play an important role in regulating blood glucose levels and controlling the development of diabetes mellitus (4). Osteocalcin, also known as bone γ-carboxyglutamic acid(Gla) protein or BGP, is a 46-50 amino acid, 5.6 kDa secreted protein produced mainly by osteoblasts (5), while osteocalcin(OC) is the most abundant non-collagenous and osteoblastic bone secreted protein. It consists of carboxylated OC(cOC) and undercarboxylated OC(ucOC) forms (6).
OC, especially ucOC, can improve pancreatic function and metabolic status by targeting multiple tissues essential for glucose and lipid metabolism. In the pancreas, ucOC can directly promote β-cell proliferation and insulin production via GPRC6A (7, 8), and ucOC also indirectly promotes insulin production by increasing intestinal glucagon-like peptide-1 (GLP-1) levels and thereby promoting GPRC6A secretion (9). In insulin-targeted tissues, ucOC can increase glucose and fatty acid uptake (10), insulin sensitivity (11), nutrient utilisation and mitochondrial capacity, and reduces glycogen production in muscle and lipid synthesis in the liver (12, 13). Moreover, non-carboxylated osteocalcin ucOC is regulated by insulin and increases β-cell proliferation as well as insulin production and secretion, whereas skeletal muscle and adipose tissue respond to osteocalcin by increasing insulin sensitivity (14).
The study of the link between osteocalcin and diabetes suggests that we can control blood glucose by regulating osteocalcin levels.
A substantial body of evidence from numerous studies indicates that osteocalcin plays a role in regulating insulin secretion and in the prevention and treatment of diabetes. The numerous cross-sectional studies conducted provide a strong scientific basis and practical application for this finding.
In a comprehensive and systematic review of previous studies on osteocalcin, Meredith L. Zoch (14) and colleagues provide a new perspective on the role of osteocalcin in bone metabolism, reproduction, and cognition. They demonstrate that osteocalcin is a bone-derived factor that affects these processes through endocrine circuits in bone. This study challenges the conventional view of osteocalcin perception.
Naomi Dirckx (15) and others further reviewed and analysed the research in this field in relation to osteoblasts, reviewing the specific response of osteoblasts to metabolic hormones as well as the production of at least three endocrine factors affecting whole-body metabolism from an osteoblast perspective. Setor Kwadzo Kunutsor (16) et al. reviewed and meta-analysed serum total osteocalcin in relation to type 2 diabetes and intermediate metabolic phenotypes. Dong-Mei Liu et al. (17) outlined bone as another potential target for the treatment, prevention and prediction of diabetes. Yixuan Li (18) et al. focused on bone-derived hormones such as fibroblast growth factor 23 and osteocalcin, and summarised their new therapeutic roles in the regulation of diabetes and diabetic nephropathy. Monika Martiniakova (3) and others discussed the current role of osteocalcin in the management and treatment of diabetes mellitus, osteoporosis, osteomalacia, and inflammatory joint diseases from a preclinical as well as a clinical research perspective, and reviewed the latest disease research advances of osteocalcin.
Although the above review articles provide insights and value for understanding some specific aspects of the research on the relationship between diabetes and osteocalcin, they fail to consider the skeleton as a whole as an endocrine organ. Instead, they focus on osteocalcin as part of the narrative, examining only the mechanism of osteocalcin or the treatment of diabetes. Furthermore, these reviews do not analyse osteocalcin and diabetes in a systematic manner. They also fail to provide insights into the range of topics covered in the research area, as well as the composition and geographical distribution of influential journals and distinguished authors and institutions in this scientific field, and its history and latest trends. Furthermore, the articles in question do not provide insights into the range of topics covered by the research area, nor do they offer an overview of the scientific field in terms of influential journals, the composition of distinguished authors and institutions, or the geographical distribution. They also fail to provide information on the history and recent trends of the field. This is not a deficiency of the literature itself, but rather a consequence of the choice of focus, coverage, and research methods and tools employed in these review articles.
Bibliometrics is a comprehensive scientific mapping and analysis tool that enables the analysis and processing of vast quantities of literature information, thereby enhancing the efficiency of scientific research (19). It is employed to illustrate relationships, such as citations, collaborations, and co-occurrences, within the literature. It is also used to construct various types of knowledge graphs and to explore the critical paths, research hotspots, and cutting-edge directions of the evolution of a discipline or field. The visualisation method of co-citation analysis in bibliometrics assists in the interpretation of data and defines a relationship between two articles if they are cited by one or more other articles at the same time. Co-citation analysis allows for the assessment of the relative importance placed by researchers on the cited literature.
However, to the best of our knowledge, there are no previous bibliometric studies that have reported an association between osteocalcin and diabetes.
It was therefore our intention to address this knowledge gap. In this study, the bibliometric software Bibliometrix and the visualisation tool VOSviewer were employed to analyse the literature pertaining to osteocalcin and diabetes and to map scientific knowledge.
Materials and methods
Literature sources and search strategy
After obtaining relevant title keywords from PubMed and supplementing them with web subject terms, we initiated an exhaustive online bibliographic search via WoS in the following format:
All articles from October 1986 to May 2024 were searched in the Web of Science Core Collection (WoSCC) database using the search formula (ALL = (Osteocalcin)) AND ALL = (diabetes or diabetes mellitus).
A meticulous examination of the extant literature yielded 1979 potential results, which were subjected to a rigorous screening process. This yielded the registration of 1680 papers, as illustrated in
Figure 1-Inclusion and exclusion criteria for diabetes in osteocalcin studies.
Data collection and statistics
The raw data downloaded from WoSCC was initially imported into Microsoft Excel 2019 for preliminary collation. Subsequently, two researchers (FZG and WJ) conducted independent verification assessments. In the event of discrepancies, the assessment was reassessed by a third party and immediately triangulated. Finally, the bibliometric parameters were extracted, namely the number of papers and citation frequency.
The statistical methods employed included importing the collated data into the bibliometric online analysis platform (https://bibliometric.com/) for statistical analysis of the total number.
A cluster analysis of keywords was conducted using VoS viewer, based on their occurrence in titles and abstracts (19). Furthermore, the frequency and interconnections of different keywords were described by the colour, size and connecting lines of the circles (20).
Result
Annual scientific production
As illustrated in Figure 2- Line graph of the number of articles published over time., the earliest pertinent article was published in 1986. Between 1986 and 1990, the field is still in its infancy, with no more than five articles published each year. In 1991, there was a notable increase in the number of articles published, which can be attributed to the advancement of molecular biology techniques, such as the study of the osteocalcin gene sequence (21). This resulted in a scientific output that was greater than that of the previous five years combined. The period from 1991 to 2013 demonstrates a consistent upward trajectory, punctuated by fluctuations. The greatest increase in articles occurred in 2013, due to the proposal that “research was needed to define the role of osteocalcin and its carboxylated or under-carboxylated forms in the regulation of human glucose metabolism” (22). Consequently, as a consequence of the evolution of research themes, the annual scientific production of articles shows a decreasing trend, with two notable declines occurring in 2019 and 2023. In 2019, the majority of studies were related to osteoporosis, a complication of diabetes mellitus. The data from 2023 and 2024 are not relevant to the research topic due to the timing of the entry.
Average number of citations per year
A total of 81,674 citations have been made of the 1,680 articles selected from the Web of Science (WoS) database, with an average citation frequency of 48.62 per article. As illustrated in Figure 3- A line graph showing the total citation volume per article over time., the peak of the average total citations per article was observed in 2007, with 120.76 citations per article as of 19 May 2024. This was followed by 2006, with 115.63 citations per article. Further analysis of the graph of the average number of citations per article per year in Table 1 Since 1986, the number of articles and citation counts in this field(MeanTCperArt means the mean number of total citations per article. N means the total number of articles in that year. MeanTCperYear means the mean number of MeanTCperArt per article) reveals that 2007 was the most prolific year, with an average of 6.71 citations per article per year. This was followed by 2006 (6.09), 2009 (5.24), and 2005 (5.21). 2010 ranked fifth, with an average of 4.83 citations per article per year and a total of 4,190 citations. In 2010, the literature was ranked fifth, with an average of 4.83 citations per article per year and a total of 4,199 citations, which placed it second only to the 4,468 citations in 2007. A detailed examination of these three types of data reveals the intricate nature of the influence of scholarly outputs and underscores the significance of a comprehensive assessment of the impact of the scholarly literature.
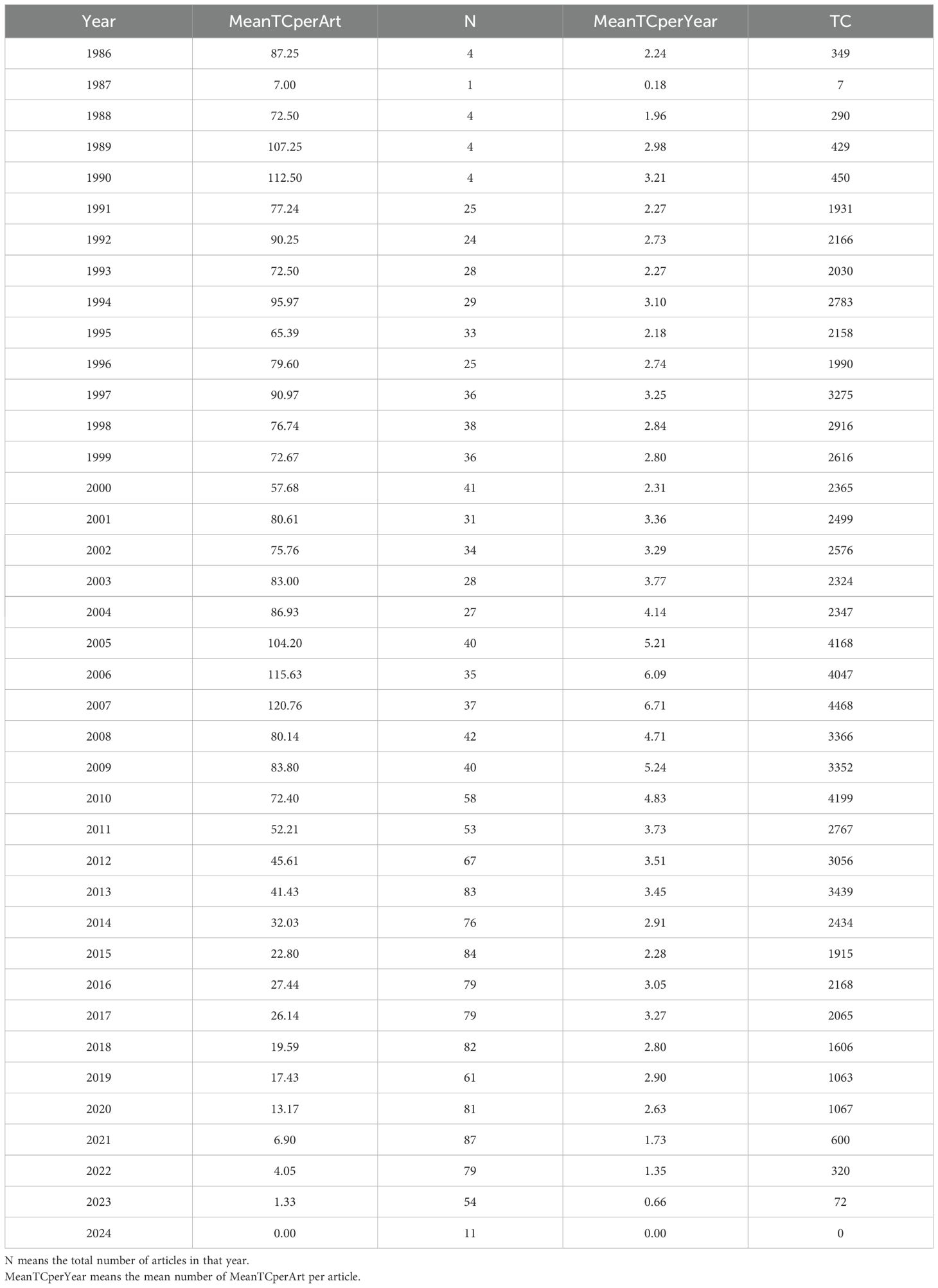
Table 1. Since 1986, the number of articles and citation counts in this field (MeanTCperArt means the mean number of total citations per article.
Quantity and citations among different nations
Figure 4 Analysis of research output in the field by various countries. The colour of the country represents the number of publications in that field. The darker the colour, the greater the number of articles published in that field. provides a global overview of the volume of scientific output in this field, with the United States accounting for the largest number of publications in this field. Table 2- Top 10 Countries with the Highest Productivity presents a comparative analysis of the performance of different countries in terms of scientific research output, based on three sets of data. The countries included in the analysis were selected based on their ranking in both the Countries’ Scientific Production and Most Cited Countries categories. The United States, with 2,411 scientific production articles, a total of 45,359 citations and an average citation rate of 81.7 citations, was at the top of the list, clearly outperforming China and Japan. China’s scientific production was 1,198 articles, with a cumulative total of 4,444 citations and an average citation rate of 16.5 citations. Japan’s scientific production amounted to 501 articles, with a cumulative total of 4,810 citations and an average citation rate of 37.6 citations, which is more than double that of China. The article with the highest number of citations in Japan was that which concluded that “osteocalcin was important not only for bone metabolism, but also for glucose and fat metabolism” (23). This was achieved by studying the parameters of atherosclerosis in men with type 2 diabetes mellitus and postmenopausal women. The highest number of citations for a single article in China was 198, and the article (24) concludes that “mucins could mimic the biological effects of insulin”.
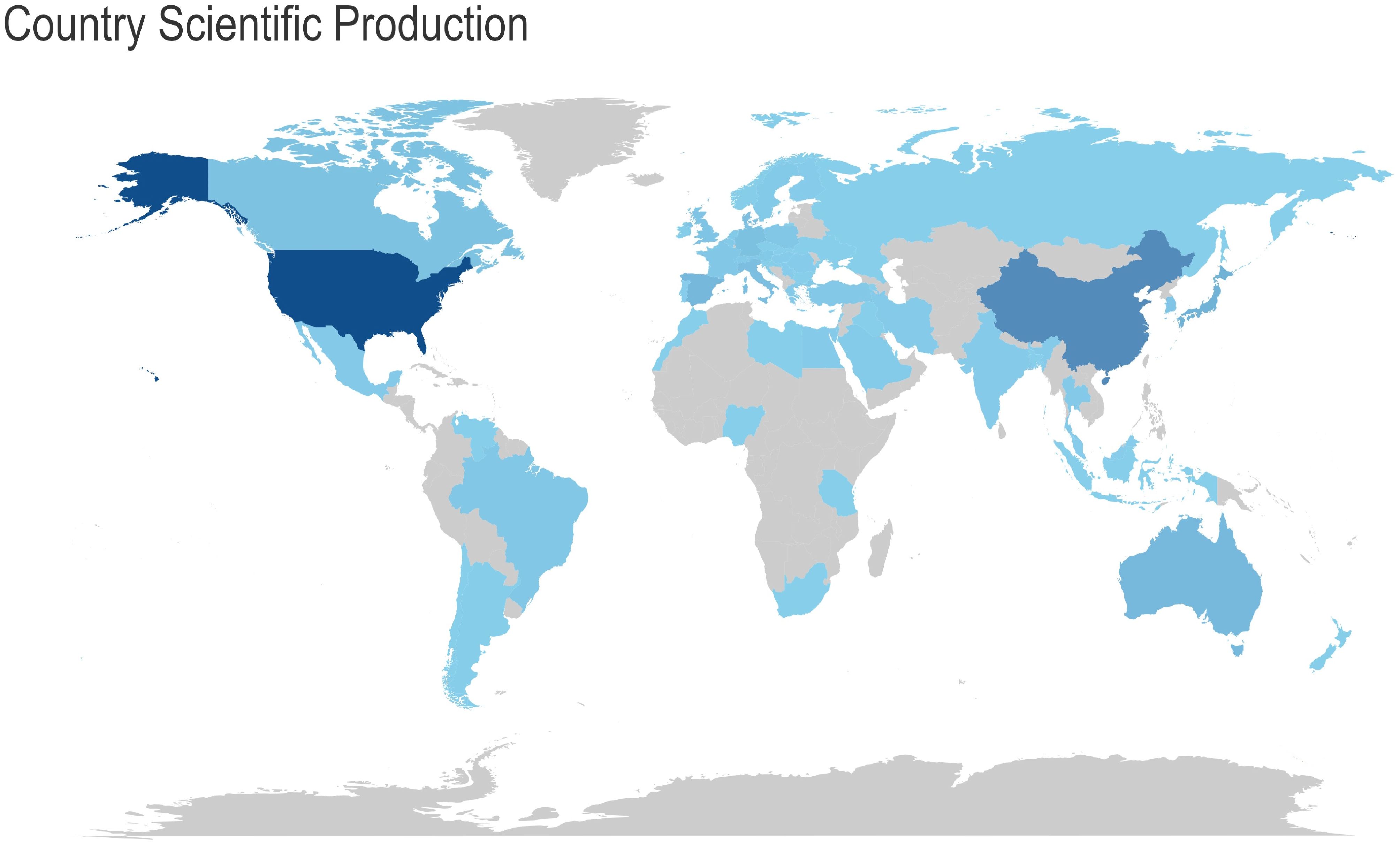
Figure 4. Analysis of research output in the field by various countries. The colour of the country represents the number of publications in that field. The darker the colour, the greater the number of articles published in that field.
As illustrated in Figure 5- Analysis of collaboration objectives among nations, with lines indicating cooperation projects between two countries, the United States had the greatest number of interrelated targets (36 countries, including China, Italy, Canada, the United Kingdom, Japan, Germany, France, and others) in terms of country cooperation. It is recommended that efforts be intensified to reinforce international cooperation and communication.
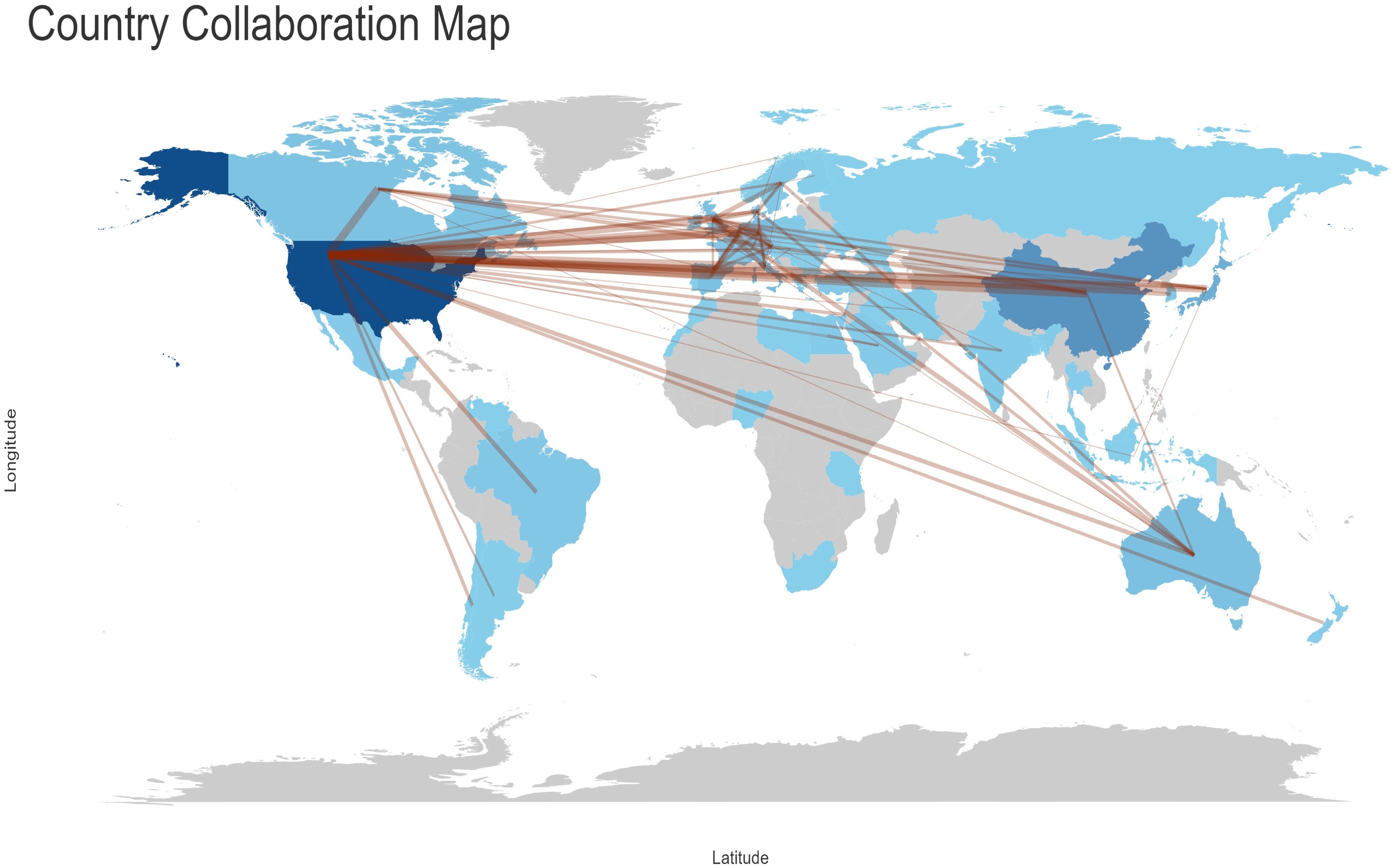
Figure 5. Analysis of collaboration objectives among nations, with lines indicating cooperation projects between two countries.
High contribution journals
The top 10 journals by publication volume collectively published 576 articles, representing 34.29% of the total (Table 3- Top 10 Relevant Popular Journals). Among the journals, the Journal of Clinical Endocrinology & Metabolism had the highest number of publications (105 articles) and the most total articles published (89,559). Meanwhile, the Journal of Bone and Mineral Research had the highest Journal Citation Indicator (JCI) (1.38) and Impact Factor (6.2).
The number of articles published by different institutions
In terms of the number of publications, Harvard University in the United States is the leading institution, with 53 articles, as illustrated in Figure 6- Statistical analysis of the number of articles published by institutions. The larger the cluster, the more articles. Columbia University follows with 51 articles. The third-ranked institution is Shanghai Jiao Tong University from China, with 50 articles, which also represents the highest number of publications from a single institution in China. Among the top ten research institutions, eight are from the United States, with the remaining two being Shanghai Jiao Tong University from China and Shimane University from Japan.
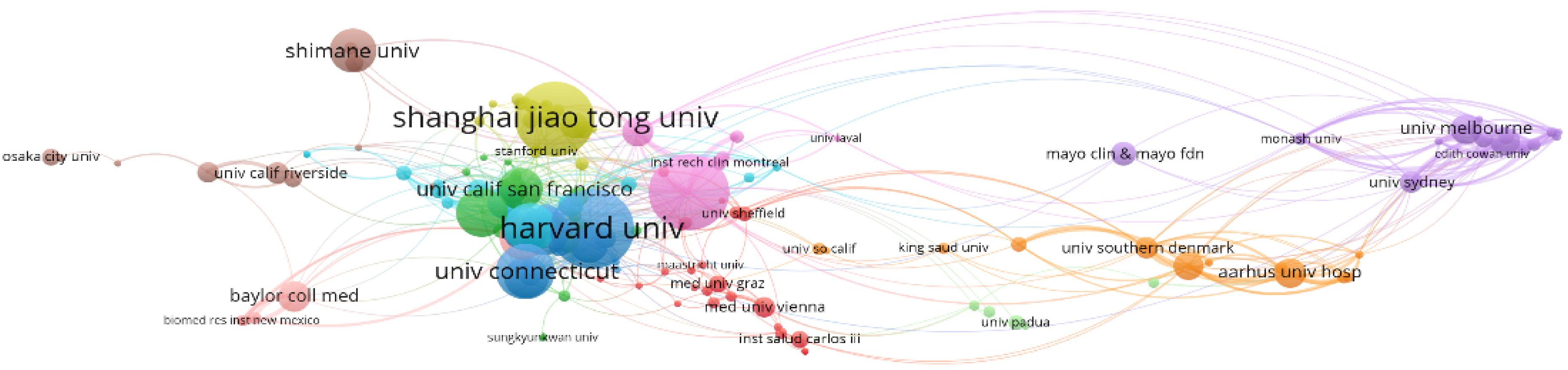
Figure 6. Statistical analysis of the number of articles published by institutions. The larger the cluster, the more articles published.
Collaboration network
The author collaboration network graph generated by Bibliometrix has eight clusters after excluding individual authors as clusters. The first cluster is purple, the second is blue, the third is pink and the fourth is grey. The first group consisted mainly of Chinese researchers from Shanghai Jiao Tong University (SJTU), and its core Figure was Prof. Yuxian Bao, who had the strongest collaborative relationship among the four of them, namely Academician Weiping Jia, Prof. Xiaojing Ma and Scholar Yiting Xu. It should be noted that the graph shows that the scholar in the first group, Jing Zhang, had made contact with the second group, but subsequent checking of the information revealed that the researcher working with Prof Bao in the first group was from SJTU, and the researcher working with L R McCabe in the second group was from Michigan State University. The names of these two people are repeated, resulting in an assignment error. Meanwhile, the first group shown in Figure 7-Collaborative relationships among authors. Divided into 8 clusters, the connections between nodes indicate the existence of collaboration, with the thickness of the lines representing the strength or frequency of the collaboration. The author names at the centre of each cluster represent the key Figures of that cluster. worked closely with the fourth group. However, due to the abbreviation of the names, Xinmei Zhang collaborated with Prof. Levinger, Itamar in group 4, and Xiaohui Zhang collaborated with Prof. liu y, neither of whom was from SJTU. The liu y in the fourth group also refers to different people, which does not indicate that the first and fourth groups worked closely together. The second group was led by Prof. Stein, Gary S. from the University of Vermont, and had close cooperation with Prof. Lian, Jane B. and Prof. Stein, Janet L. The members of this group came from different research institutions in the United States, such as the University of Vermont, the University of Massachusetts, Harvard University, the University of Wisconsin, and Michigan State University, and so on. In Figure 7-Collaborative relationships among authors. Divided into 8 clusters, the connections between nodes indicate the existence of collaboration, with the thickness of the lines representing the strength or frequency of the collaboration. The author names at the centre of each cluster represent the key Figures of that cluster., the members of the second group worked closely with the third group. In fact, it is Prof. Yamauchi, Miki, who had collaborated with the members of the second group, not Yamauchi, Mika, who was the researcher of the third group, and the central Figure of the third group was Prof. Sugimoto, Toshitsugu of Shimane University, who had collaborated with Prof. Kanazawa, Ippei, Prof. Yamauchi, Mika, and Prof. Yamaguchi, Mika. Professor Sugimoto, Toshitsugu of Shimane University, who had a strong collaborative relationship with Professor Kanazawa, Ippei, Professor Yamauchi, Mika and Professor Yamaguchi, Toru, and the four of them had a total of seven articles related to the topic of this paper on the Web of Science, compared with the four in the first group who had a total of eight articles related to the topic of this paper.
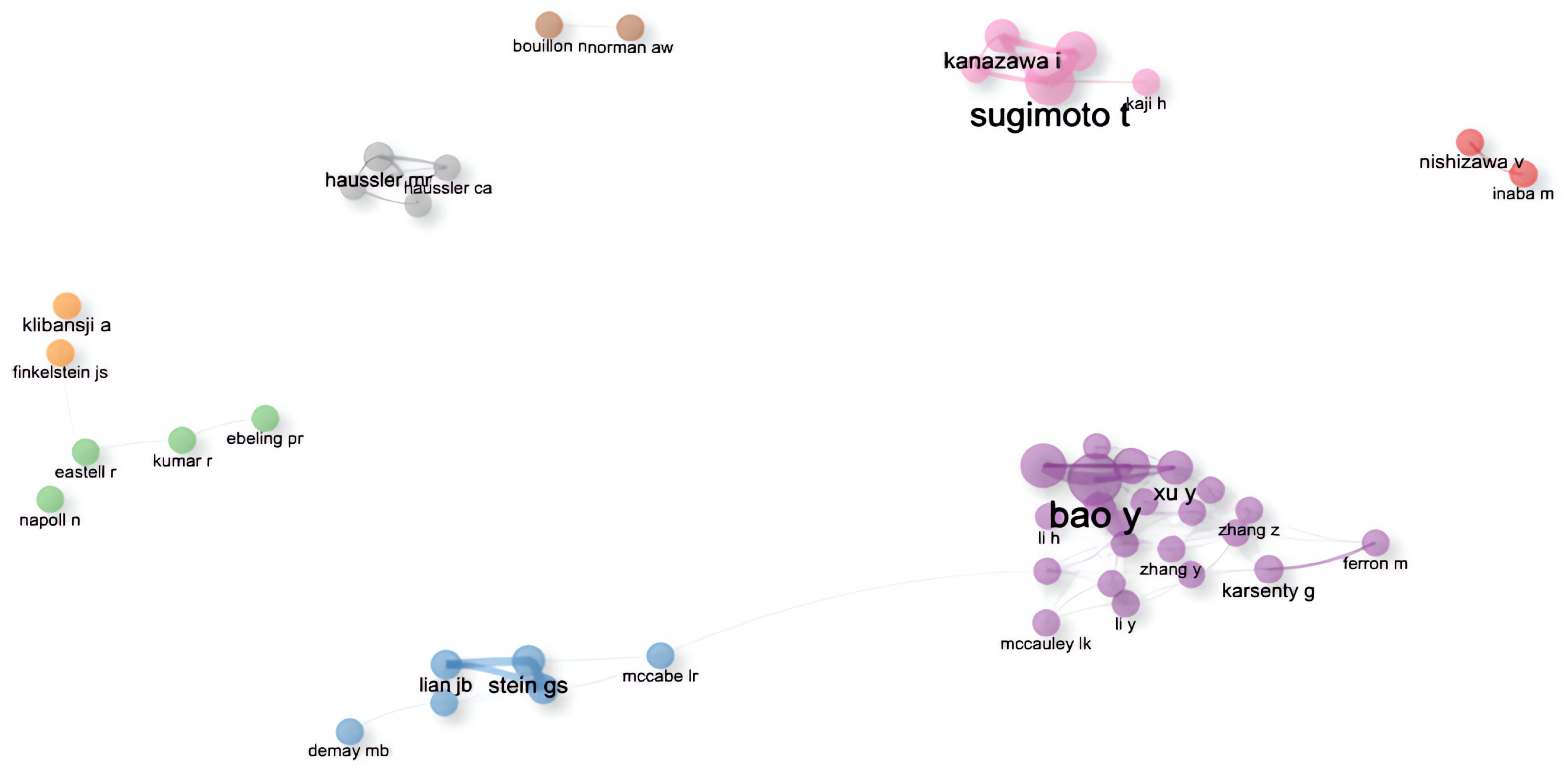
Figure 7. Collaborative relationships among authors. Divided into 8 clusters, the connections between nodes indicate the existence of collaboration, with the thickness of the lines representing the strength or frequency of the collaboration. The author names at the centre of each cluster represent the key Figures of that cluster.
Keywords have been filtered from the existing data, resulting in 395 words, which can be divided into four clusters, as it is shown in the Figure 8-Keywords scientific analysis Cluster 1, “Expression and Function of the Osteocalcin Gene,” is represented by green. Cluster 2, “Differential Expression of the Osteocalcin Gene and Its Impact on Diabetes,” is represented by blue. Cluster 3, “Role of Osteocalcin in Bone Turnover,” is represented by red. Cluster 4, “Indirect Involvement of Osteocalcin in Metabolic Processes,” is represented by yellow. The size of the keyword circles within each cluster corresponds to their frequency of occurrence.
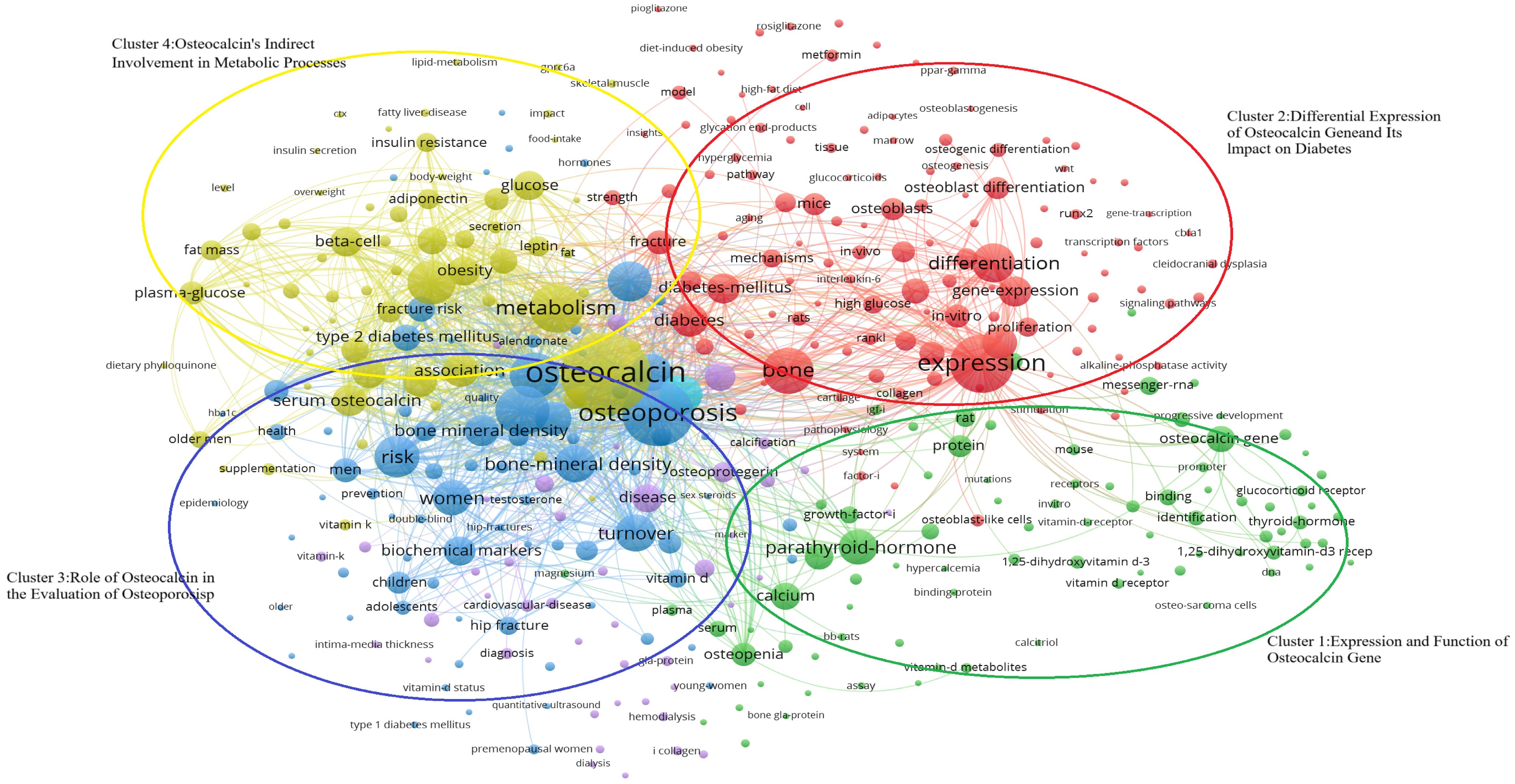
Figure 8. Keywords scientific analysis clustering. (Cluster 1:Expression and Function of Osteocalcin Gene; Cluster 2:Differential Expression of Osteocalcin gene and Its Impact on Diabetes; Cluster 3;Role of Osteocalcin in the Evaluation of Osteoporosis; Cluster 4:Expression and function of Osteocalcin’s Indirect Involvement in Metabolic Processes).
The main terms constituting cluster 1 are “osteocalcin gene” (25, 26), “protein” (27, 28), “parathyroid hormone” (29, 30) and “1,25-dihydroxyvitamin-D3 receptor” (29, 31). Meanwhile, cluster 2 is primarily characterized by “expression” (25, 26), “difference” (25, 32), “diabetes” (33, 34) and “osteoblast” (31, 35). Cluster 3 focuses on keywords such as “osteoporosis” (36, 37), “bone turnover” (38, 39), “male” (40, 41), and “female” (42, 43). Lastly, cluster 4 includes keywords like “osteocalcin” (37, 44), “metabolic syndrome” (45, 46), “insulin resistance” (33, 47), and “plasma glucose” (41, 44).
VoS viewer marks keywords in the graph with different colours based on the average year of occurrence, with purple keywords appearing earlier than blue and yellow ones. The transition from purple to green and finally to yellow illustrates the developmental process of the keywords. The graph provides a comprehensive overview of the researchers’ investigation focus, including four clusters of keywords such as “Expression and Function of Osteocalcin Gene,” “Differential Expression of Osteocalcin Gene and Its Impact on Diabetes,” “ Role of Osteocalcin in the Evaluation of Osteoporosis and Diabetes” and “Osteocalcin’s Indirect Involvement in Metabolic Processes.”
Through bibliometrics, one can quantitatively and systematically assess trends in a specific field and predict potential research directions, as it is shown in the Figure 9-. In this study, keywords were categorized into four clusters:
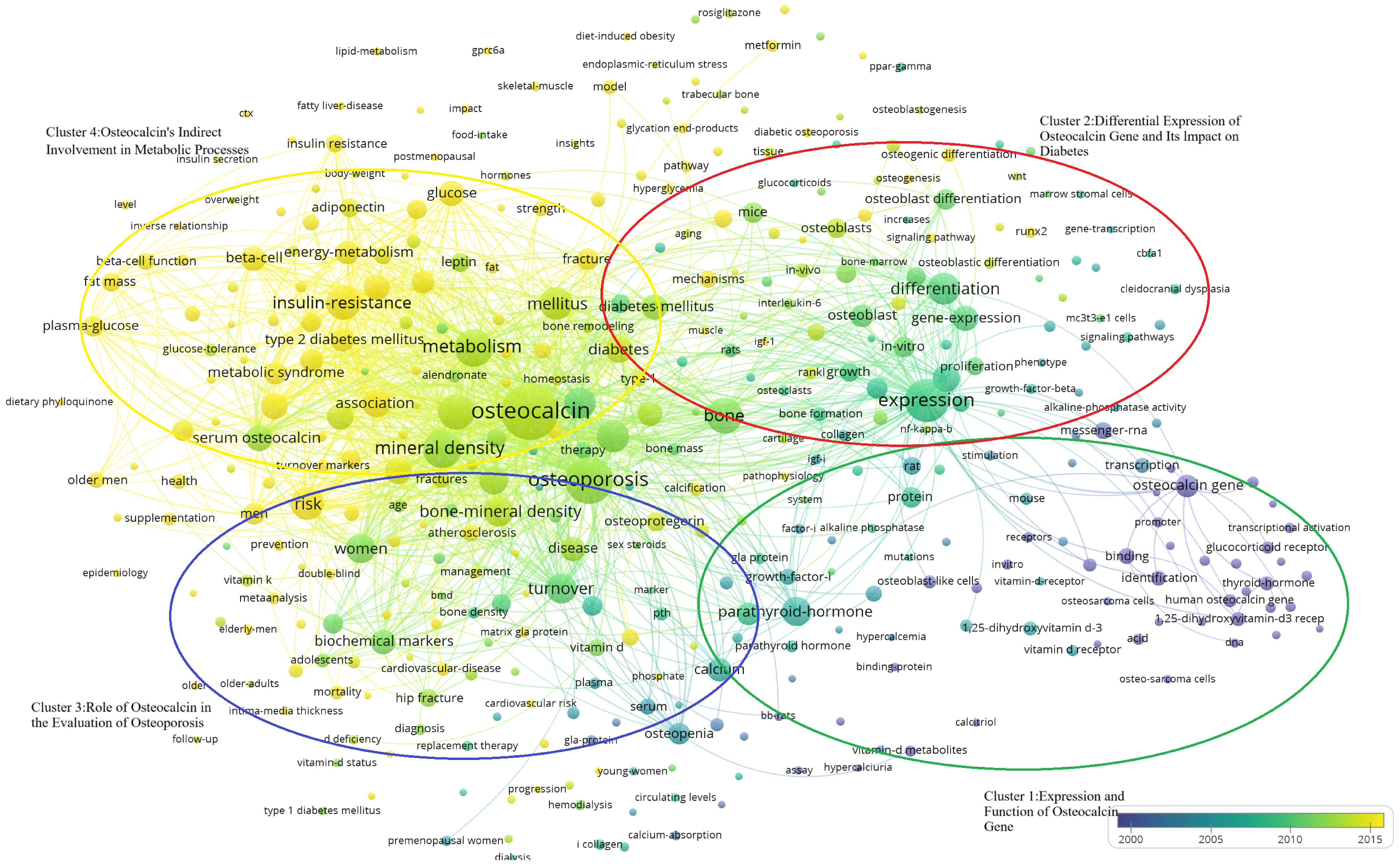
Figure 9. Temporal distribution of the four keyword clusters. (Cluster 1:Expression and Function of Osteocalcin Gene; Cluster 2:Differential Expression of Osteocalcin gene and Its Impact on Diabetes; Cluster 3;Role of Osteocalcin in the Evaluation of Osteoporosis; Cluster 4:Expression and function of Osteocalcin’s Indirect Involvement in Metabolic Processes).
“Expression and Function of Osteocalcin Gene”(Cluster 1, Green) “Differential Expression of Osteocalcin Gene and Its Impact on Diabetes”(Cluster 2, Blue) “ Role of Osteocalcin in the Evaluation of Osteoporosis and Diabetes”(Cluster 3, Red) “Osteocalcin’s Indirect Involvement in Metabolic Processes”(Cluster 4, Yellow). In the Figure 9-, it shows that the researchers’ investigative interests shifted from Cluster 1 to Cluster 2, then to Cluster 3, and finally to Cluster 4.
Characteristics of the top 10 most cited research articles
The 10 most cited articles were cited a total of 8493 times (10.40%, Temporal distribution of the four keyword clusters. (Cluster 1:Expression and Function of Osteocalcin Gene; Cluster 2:Differential Expression of Osteocalcin gene and Its Impact on Diabetes; Cluster 3;Role of Osteocalcin in the Evaluation of Osteoporosis; Cluster 4:Expression and function of Osteocalcin’s Indirect Involvement in Metabolic Processes) (Figure 10- Top 10). The third most cited article was a review, and Prof. Na Kyung Lee’s 2007 article (48) ranked first in both total citations and citation frequency per year, with 1902 and 105.67, respectively. This article accounted for 42.6% of the total citations in 2007. In 2005, articles by Tripti Gaur (26) and Christina N Bennett (28) ranked second and seventh in total citations, with 923 and 702, respectively. These two articles combined account for 38.99% of the citations for the year. Mathieu Ferron’s articles in 2010 (27) and 2008 (25) ranked fourth and sixth at 831 and 721, respectively, accounting for 19.79% and 21.42% of that year’s articles. His two articles accounted for 18.27% of the 10 most cited articles. Thus, the average number of citations per year is determined by a combination of annual scientific output and high-impact articles, with the latter playing a more pronounced role. The ten articles are presented in Table 4-Top 10 Most Cited Research Paper for the reader’s convenience.
Discussion
Expression and function of osteocalcin gene
As shown in Figure 8-Keywords scientific analysis In cluster 1, the main terms are “osteocalcin gene”, “protein”, “parathyroid hormone”, “thyroid hormones” and “1,25-dihydroxy vitamin-D3 receptor”. This group of terms is summarized as the physiological mechanism where osteocalcin interacts with other hormones.
Osteocalcin exerts an influence on the absorption and regulation of calcium, operating in conjunction with other hormones. It has been demonstrated that thyroid hormone (TSH) exerts a pivotal influence on bone growth and development (50). The thyroid glands secrete substantial quantities of thyroxine (T4) and 3,5,3′-triiodothyronine (T3). Although the circulating levels of T3 are considerably lower than those of T4, T3 activates T3 receptors (TRα and TRβ) on osteoblasts, thereby stimulating osteocalcin secretion (51). Further investigation has revealed that TRα is the predominant isoform expressed in bone tissue, while TRβ deficiency results in a hyperthyroid effect in osteoblasts (52). Although T4 does not have as strong an affinity for the receptor as T3 (53), it has also been demonstrated in experimental studies that its osteoblastic differentiation can have a stimulatory effect (51). Furthermore, T4 and T3 have been demonstrated to directly stimulate bone resorption in vitro (54). Furthermore, one study indicates that estrogen status may influence the TSH response to bone (55), which could potentially elucidate the elevated prevalence of osteoporosis observed in postmenopausal women. As a consequence of TSH suppression and elevated FT4 and/or free triiodothyronine (FT3) levels in hyperthyroid patients, there is an increased rate of bone conversion, a reduction in bone mineral density (BMD), and an elevated risk of fragility fractures (56). A controlled trial of a cohort of patients with hyperthyroidism, hypothyroidism, and euthyroidism revealed that blood osteocalcin levels were markedly elevated in the hyperthyroid group and markedly reduced in the hypothyroid group when compared to the euthyroid control group (57). In patients with hyperthyroidism, antithyroid hormone medication has been demonstrated to reduce blood osteocalcin concentrations. Conversely, in patients with hypothyroidism, L-T4 has been shown to be an effective means of restoring osteocalcin levels (58). It has been demonstrated that thyroid hormone can facilitate osteocalcin synthesis in osteoblasts by activating p38 MAP kinase (59). Consequently, the thyroid hormone plays a pivotal role in regulating bone function, and influencing bone metabolism. Hyperthyroidism is a recognized cause of secondary osteoporosis (60), which is characterized by accelerated bone turnover and a negative calcium and phosphorus balance (61). In addition to its role in bone conversion and formation, the promotion of osteocalcin synthesis, which leads to increased bone conversion, may also be a pathway by which hyperthyroidism causes osteoporosis.
With regard to parathyroid hormone (PTH), it is once more an essential peptide hormone that regulates bone formation and osteoblast activity. Parathyroid hormone is capable of regulating extracellular calcium and phosphorus levels and stimulating bone resorption. Two principal pathways are responsible for the regulatory effects of parathyroid hormone on bone: the PKA pathway and the PKC pathway. The regulatory effects of parathyroid hormone on bone are mediated by direct activation of parathyroid hormone receptor 1 (PTHR1) on osteoblasts, which stimulates Gαs-mediated activation of adenylate cyclase and promotes the production of cAMP, followed by the activation of protein kinase (PKA) (62). Subsequently, the activation of the Raf-MEK-ERK mitogen-activated protein kinase (MAPK) cascade reaction (63) results in a proliferative effect on bone (64). Furthermore, the production of cAMP and the activation of PKA can also activate Gq/11-mediated PLCβ stimulation, which in turn leads to the production of inositol 1,4,5-trisphosphate (IP3), the mobilization of calcium and the activation of protein kinase C (PKC) (65). PKC then activates MAP kinase and exogenous signal-regulated kinase (ERK), which in turn results in the onset of osteoblast proliferation (64). Furthermore, it has been demonstrated that parathyroid hormone can act synergistically through the PKA and PKC pathways to co-stimulate osteocalcin gene expression in osteoblasts (66). This pathway directly activates osteoblast OCN gene transcription by facilitating the binding of the OSE1 sequence proximal to the mOG2 promoter to nuclear proteins (67). Clinical trials have demonstrated that increased parathyroid hormone levels lead to elevated osteocalcin levels (68) and are effective in ameliorating idiopathic osteoporosis in men (69). Notably, several experiments have shown that both instantaneous and intermittent administration of parathyroid hormone are effective in stimulating bone formation and repair (70, 71), which appears to be related to the gradient sensitivity of parathyroid hormone (71).
OSE2 is a specific cis-acting element present in osteoblasts, and Osf2/Cbfa1 is a protein that binds to OSE2, regulated by Bone Morphogenetic Protein 7(BMP7) and Vitamin D-3. Osf2/Cbfa1 binds to and regulates the expression of multiple genes in osteoblasts (72). Research indicates that 1,25D regulates bone mineral remodelling by inducing Receptor Activator of Nuclear Factor κB Ligand(RANKL), Secreted Phosphoprotein 1 Gene(SPP1), and Bone Gla Protein(BGP), and also controls calcium and phosphorus reabsorption in the kidneys, with the skeleton acting as a sensor for phosphorus levels (73). Upon binding with the Vitamin D receptor, 1,25-dihydroxyvitamin D3(1,25D) directly inhibits parathyroid hormone gene transcription and antagonizes osteocalcin to prevent hypercalcemia (29). Controlling osteocalcin expression may delay age-related chronic diseases such as osteoporosis, type 2 diabetes, cardiovascular disease, and cancer.
While not directly involved in bone mineralization, osteocalcin has been identified as a factor that plays a role in preventing excessive bone mineralization and increased bone fragility in osteoporosis. The absence of osteocalcin in experimental animals led to the observation that, despite the normal survival of osteocalcin-free mutant mice, their cortical bone width was significantly higher than that of the wild type after six months. This was observed in the absence of an increase in osteoclast number, mineralization rate, and bone mineral content (74). This indicates that osteocalcin is not a factor in the process of mineralization. Subsequently, the researchers induced osteoporosis in the mutant and wild-type mice by removing their ovaries. This resulted in more pronounced osteoporosis symptoms in the mutant mice than in the wild-type, which may be attributed to an increased number of osteoclasts in the mutant mice. This indicates that osteocalcin exerts its effect by limiting bone matrix formation, without influencing resorption or mineralization. The relative amount of hydroxyapatite phosphate is diminished in osteocalcin-deficient mice, which exhibit reduced bone maturation (75), indicating that osteocalcin deficiency may be associated with increased bone fragility. It may therefore be posited that the administration of vitamin K (76), 1,25-dihydroxy-D3 (77) to patients exhibiting low osteocalcin levels may prove an efficacious method of reducing the risk of osteoporosis, particularly in those already diagnosed with the condition. In the context of long-term warfarin anticoagulation, the potential for reducing the risk of osteoporosis may be realized through the replacement of warfarin with a non-vitamin K-dependent anticoagulant, such as dabigatran (78).
The association between osteocalcin levels and type 2 diabetes has been demonstrated in numerous studies across diverse populations (36, 79, 80). In animal experiments, it has been demonstrated that osteocalcin is associated with the regulation of blood glucose levels and improved insulin sensitivity and secretion (48). Intermittent osteocalcin injections have been shown to increase glucose tolerance and insulin sensitivity, improve pancreatic β-cell mass, and prevent hepatic steatosis in mice (44). While this treatment regimen has not yet been implemented in clinical practice (81), it represents a promising avenue for future diabetes treatment and prevention strategies.
Despite evidence from statistical experiments indicating a potential correlation between osteocalcin and cardiovascular disease (82–85), the precise mechanism of action remains unclear. Osteocalcin has been shown to exert protective effects on the vascular endothelium in both animal (86) and in vitro (87) experiments. The transformation of vascular smooth muscle cells into osteoblast-like cells is a key mechanism underlying vascular calcification in advanced atherosclerosis (88). Higher osteocalcin levels were observed in calcified plaques and aortic valves (89), and there was a positive correlation between vascular smooth muscle cell shift to osteoblast-like cells and osteocalcin expression (90). However, a clinical meta-analysis has indicated that in certain cardiovascular diseases, particularly atherosclerosis, no discernible correlation was observed between osteocalcin and markers of atherosclerosis and calcification (91). In contrast, studies of young adults have revealed a strong correlation between osteocalcin and cardiovascular disease, with this association becoming more pronounced with age (92). Given the role of osteocalcin in improving blood glucose, blood lipids and alleviating diabetes, its effects may be indirect and related to the regulation of blood glucose and lipid levels and metabolism. Despite the fact that numerous experiments have demonstrated that the role of osteocalcin in cardiovascular diseases is complex and still unclear, further research is required, the close association between osteocalcin and some diseases, in particular atherosclerosis, may prove to be a significant breakthrough in this field.
Given the regulatory role of osteocalcin in bone formation and remodeling, there has been considerable interest in its association with tumors, especially primary bone tumors. A reduction in osteocalcin expression has been observed in tumor cells in numerous animal experiments on osteosarcoma (93, 94). This may be associated with defective collagen fiber mineralization in osteosarcoma (95). Furthermore, rearrangements and loss of expression of the p53 gene have been observed in the vast majority of osteosarcomas (96). It has been postulated in animal studies that p53 rearrangement may contribute to the maintenance of a tumourigenic phenotype in osteosarcoma (93). Furthermore, it has been suggested that the p53 gene is a positive regulator of osteocalcin (97). It is therefore challenging to ascertain whether reduced osteocalcin levels contribute to elevated osteosarcoma incidence, whether increased osteosarcoma incidence is attributable to p53 rearrangements, or if both factors are involved. Further study is required to elucidate the precise effects of osteocalcin on osteosarcoma. While the precise role of osteocalcin in osteoblast differentiation remains unclear, its potential as a discriminating criterion for the degree of differentiation has been clinically validated (98). It is noteworthy that a viral toxicity therapy based on the intravenous injection of the osteocalcin promoter was observed to significantly inhibit the growth of osteosarcoma lung metastases and significantly improve survival in animal experiments (99). This appears to indicate that osteocalcin may also exert an inhibitory effect on osteosarcoma metastases. This offers a novel perspective on the potential treatment of osteosarcoma and its metastases.
Differential expression of osteocalcin gene in relation to diabetes mellitus
Cluster 2 is characterised by the core terms ‘expression’, ‘differential’, ‘diabetes’ and ‘osteoblasts’. In this group, differential gene protein expression in osteoblasts may be associated with diabetes and the development of diabetic bone disease.
On the one hand, bone trap cells, osteoblasts produce rankl which is an important factor for osteoclast differentiation and activation (100), and osteoclasts have an extremely important role for the conversion of osteocalcin to ucOC, and ucOC has an effect on insulin that enhances its sensitivity phenotype, so that osteoblasts have an indirect regulatory role for insulin and glucose. In diabetic patients, high blood glucose decreases osteoblast and osteoclast activity, and Wittrant, Y. et al. showed that high blood glucose inhibits osteoclast formation, which may be due to the inhibition of redox-sensitive NF-kappaB activity through antioxidant mechanisms to alter RANKL-induced osteoclast formation (101).
Also, in osteoblasts, sympathetic tone stimulates the expression of Esp, a gene that inhibits osteocalcin activity by acting on its own to reduce osteocalcin secretion (102). In addition, the transcription factors forkhead box O1 (Foxo1) and activating transcription factor 4 (ATF4) inhibit osteocalcin metabolic activity by increasing ESP expression in osteoblasts (103). In a cross-sectional Danish study, Starup-Linde, J. et al. found that elevated non-fasting blood glucose levels were negatively correlated with p-CTX, p-P1NP, p-OC and p-ucOC, with the strongest effect being seen for hypo-carboxylated OC, which was reduced by 38% (104). In addition, the diabetes treatment drug, metformin, may ameliorate the inhibitory effect of hyperglycaemia on osteoblast proliferation and gene expression (105).
The role of inflammation in T2DM is unclear and may be indirectly mediated, whereby adipocytes can activate inflammation through the production of (ROS), which in turn increases the production of inflammatory cytokines that can reduce the number of osteoblasts and stimulate osteoclast bone resorption via apoptosis at the same time. Interestingly, this process becomes sustained as ROS stimulate MSCs to differentiate preferentially into adipocytes rather than osteoblasts, leading to a reduction in the transcription of Wnt proteins and further inhibiting bone formation (106).
The role of osteocalcin in the evaluation of osteoporosis and diabetes
Cluster 3 is characterized by the core terms “osteoporosis”, “bone turnover”, “male” and “female”. This group primarily elucidates studies on the association between bone alkaline phosphatase levels and 42 mixed gender patients(male and femal), including research on osteoporosis and diabetes.
Firstly, osteoporosis is an imbalance between the activities of osteoclasts and osteoblasts. Factors influencing this imbalance include calcium intake (25), vitamin D receptors (107), vitamin D receptors (107), physical activity or mechanical stress (108), hormones (parathyroid hormone, testosterone, estrogen) (109), and the Receptor Activator Of Nuclear Factor-Kappa-B Ligand(RANKL) (110).
Secondly, osteocalcin, as a Bone Turnover Marker(BTM), may serve as one of the criteria for evaluating the risk of osteoporosis. In cross-sectional studies of women, an increase in osteocalcin(OC) may reflect enhanced bone turnover, partially contributing to the development of osteoporosis (111). Moreover, in the diagnosis of osteoporosis, bone turnover markers(btms) can be widely used to improve prognosis and monitor the response to anti-resorptive therapy (112). Therefore, serum osteocalcin may play a role in investigating patients with osteoporosis (113). Studies on men reveal that after the age of 60, an increase in bone resorption markers(rather than bone formation markers) is observed in some men, correlating with lower bone mineral density(BMD), indicating that this imbalance is a cause of increased bone loss in elderly men (114, 115). Retrospective studies show that low bone density in male osteoporosis is primarily due to reduced bone formation (116).
Ultimately, bone gla protein levels may serve as a potentially useful indicator for predicting the risk of type 2 diabetes(T2D) onset. In a controlled study of gestational diabetes in women, serum bone gla protein levels were elevated throughout pregnancy in women with gestational diabetes (117). In a cross-sectional analysis of postmenopausal women, bone gla protein levels were significantly lower in those with type 2 diabetes (118), and bone gla protein levels were significantly associated with the development of type 2 diabetes (79), suggesting that bone gla protein may play a role in regulating blood glucose levels in postmenopausal women (118). A cross-sectional study of men with type 2 diabetes found that bone gla protein was significantly correlated with glucose metabolism (119). Retrospective studies showed that in men with T2D, bone gla protein was negatively correlated with the triglyceride-glucose(tyg) index, a surrogate marker for insulin resistance (120), and undercarboxylated bone gla protein(ucoc) was associated with plasma glucose levels in men with type 2 diabetes (41), indicating that undercarboxylated bone gla protein may play a significant role in the pathogenesis of diabetes (119). Overall, bone gla protein levels may be a potentially useful indicator for predicting the risk of Type 2 Diabetes(T2D) onset (121, 122), but further research is needed.
It is worthy of note that a particular form of osteoporosis, known as diabetic osteoporosis, merits specific mention. Disturbed calcium and phosphorus metabolism in diabetic patients (123) results in abnormal collagen cross-linking, which in turn leads to reduced bone strength and increased bone fragility (124). Patients with diabetic osteoporosis are at a higher risk of sustaining a fracture than patients with normal osteoporosis (125), and the healing time for such fractures is significantly longer (126). The prevailing view on diabetic osteoporosis is currently based on the oxidative stress theory (127), which posits that excess reactive oxygen species (ROS) disrupt antioxidant defense mechanisms, leading to increased bone loss and inhibition of bone formation and inducing apoptosis in osteoblasts (128). This inflammatory mechanism is dependent on NOX2 (129). Given its involvement in bone remodeling and maturation, low osteocalcin levels are associated with reduced bone resorption, increased bone fragility, and a markedly elevated risk of fracture (130). Despite the long-standing use of total osteocalcin as a bone turnover marker for the assessment of bone turnover status (131), however, it has also been proposed that the accuracy of this method may be questionable, with no significant difference in total osteocalcin levels observed between osteoporotic and healthy individuals (132). Nevertheless, further investigation is warranted to elucidate the potential association between osteocalcin and diabetic osteoporosis. Furthermore, although the correlation between low osteocalcin levels and the onset of diabetes is not consistent across studies (133, 134), it would be beneficial to investigate this link in larger groups. This could provide insights into the specific mechanisms of diabetic osteoporosis.
Osteocalcin’s indirect involvement in metabolic processes
Finally, “Osteocalcin action on islet cells and adipocytes” is the topic of Cluster 4, which includes the keywords “osteocalcin”, “metabolism”, “insulin resistance” and “plasma glucose”. For example, in the study investigating the association between osteocalcin and time interval (TIR), researchers focused on the correlation between osteocalcin and continuous glucose monitoring (CGM) metrics in patients with type 2 diabetes mellitus (T2DM) (135). This topic focuses on the potential of osteocalcin as a new direction in the treatment of diabetes.
To gain a full understanding of this topic, we will look at two types of studies: animal and clinical.
Osteocalcin was shown to regulate insulin in animal studies as early as 2007 (48), and a large number of studies have now elucidated the associated regulatory signalling mechanisms and functional roles, particularly in mouse models. Currently, much of the research in this area is focused on changes in osteocalcin levels in diabetic animal models in response to interventions aimed at promoting bone regeneration and thus better treating osteoporosis, one of the complications of diabetes. In a diabetic rat model of critical size defects in the skull, osteocalcin expression levels can be increased by the administration of vitamin K2 , which facilitates bone healing (136). Similarly, many drugs may have potential clinical therapeutic value, such as mogroside V (MV), which promotes the ability of bone marrow mesenchymal stem cells (BMSCs) to differentiate into osteoblasts in diabetic mice (137), Icariin (ICA), which induces osteogenic-angiogenic coupling in a rat model of type 1 diabetic bone defects (138), and the combination of insulin (I) and naringin (NAR), which prevented osteoclast proliferation in type 1 diabetic rats (139). In insulin-targeted tissues, ucOC can increase glucose and fatty acid uptake (10), insulin sensitivity (11), nutrient utilisation and mitochondrial capacity, and reduces glycogen production in muscle and lipid synthesis in the liver (12, 13). Moreover, non-carboxylated osteocalcin ucOC is regulated by insulin and increases β-cell proliferation as well as insulin production and secretion, whereas skeletal muscle and adipose tissue respond to osteocalcin by increasing insulin sensitivity (14). In the pancreas, ucOC has been demonstrated to directly promote β-cell proliferation and insulin secretion via GPRC6A (7, 8). Additionally, ucOC has been shown to indirectly promote insulin secretion by increasing intestinal glucagon-like peptide-1 (GLP-1) levels, which in turn promotes GPRC6A secretion (9). And insulin also has the capacity to elevate ucOC levels via insulin receptors in two distinct ways. Firstly, insulin inhibits the expression of the Runx2 repressor Twist2, which in turn stimulates bone formation and osteocalcin production (140). Secondly, insulin reduces the production of the anti-osteoclastic factor osteoprotegerin. This results in an increase in the number of osteoclasts and enhanced bone resorption. The subsequent acid environment of the bone resorption traps (pH 4.5) promotes bone matrix decarboxylation of osteocalcin (27). This results in a positive feedback regulatory pathway between insulin and osteocalcin. UcOC also enhances the production of delta like-1 (DLK1) in pancreatic β-cells, which inhibits insulin-dependent OC production in osteoblasts (141), which in turn negatively feedback regulates ucOC production, thereby maintaining insulin levels within a reasonable range.
Many clinical studies also support the existence of the same complex regulatory mechanism between non-carboxylated osteocalcin and insulin in humans. In elderly subjects, negative correlations between serum ferritin and soluble transferrin receptor (sTfR) and osteocalcin led to the conclusion that factors related to iron metabolism may contribute to the development of insulin resistance and type 2 diabetes (142). In obese middle-aged men, insulin sensitivity after acute exercise is associated with individual levels of circulating ucOC (143). Changes in sub-glucocorticoid-induced osteocalcin concentrations were associated with reduced hepatic insulin sensitivity in a randomised controlled trial in normal men (144). In young adult Japanese patients with childhood-onset type 1 diabetes mellitus, serum osteocalcin concentrations were negatively correlated with patient body fat. By recruiting subjects from the Shanghai community, it was also concluded that serum osteocalcin levels were significantly lower in patients with diabetes mellitus, overweight/obesity or central obesity than in patients without diabetes mellitus (all P<0.01) (145).
Similarly, some studies were unable to establish a causal relationship between changes in osteocalcin and incident diabetes and insulin levels. There were no clinically important effects of antiresorptive therapy to reduce ucOC levels on fasting glucose, body weight or risk of diabetes in postmenopausal women (146). Another study also showed that changes in body composition measures or markers of fat or glucose metabolism in postmenopausal women were not associated with changes in non-carboxylated osteocalcin. This suggests that the effect of osteocalcin may be influenced by a variety of factors such as the metabolic state of the subject, other endocrine regulation and other factors (147).
Future studies need to further explore the fine regulation mechanism of osteocalcin in the human body and how it can be effectively used as a target for the treatment of metabolic diseases. In particular, the therapeutic potential of osteocalcin for diabetes and other metabolic diseases should be validated by large-scale, multicentre, randomised controlled trials.
Hot topics
Current research focuses on three main areas: the association between osteocalcin levels and diabetic complications, the clinical efficacy of drugs or vitamins for osteoporosis in diabetic patients of different ages and sexes, and the potential mechanism of insulin regulation by osteocalcin. Recent studies have correlated it with diabetic complications such as atherosclerosis (147) and cataracts (148) to provide a more accurate guide for prognostic assessment and therapeutic strategies in diabetic patients. Therefore, recent studies have focused on evaluating the clinical efficacy of different drugs or vitamins for osteoporosis in diabetic patients in order to explore the optimal therapeutic regimen and optimise patients’ bone health. In addition, as osteocalcin is thought to influence the pathogenesis and progression of diabetes mellitus by regulating insulin secretion and sensitivity, future studies may aim to further explore the role of osteocalcin in the development of diabetes mellitus. Future studies may be devoted to exploring the fine regulatory mechanisms between osteocalcin and insulin metabolism, such as signalling pathways and gene regulatory mechanisms in the human body, to reveal its specific biological functions in the pathogenesis of diabetes and to provide theoretical basis and experimental evidence for the development of novel therapeutic strategies.
Strengths and limitations
Inevitably, there are some limitations to this study. Firstly, relevant articles were screened only in the WOS database, which is the most widely used database in the world. Scopus and other databases such as SciELO, Google Scholar, and Scopus were not included in the search. Secondly, our study focused exclusively on original research and other types of publications were excluded, which may have led to omissions. Third, authors were analysed differently based on their bylines. However, over the years, some authors can have sign their papers in different ways and can create important biases in such analyses. Fourth, changes in author affiliation or dual affiliation at the time of publication may also create difficulties when analysing the data. In this study, we are cautious about these limitations. Finally, due to the low number of citations to some high-quality literature published in recent years and the fact that the databases are kept open, new papers published after the search date were not included in the study, which could have been lost in the vast ocean of literature data.
Conclusion
The number of publications in the field of diabetes and osteocalcin research has increased significantly since 2013. Mainland China and the United States have become high-productivity countries, while the countries with the highest article citations are the United States and Japan. As research has progressed, the focus has shifted to the relationship between osteocalcin levels and diabetic complications, the clinical efficacy of drugs or vitamins for osteoporosis in diabetic patients, and the potential mechanism of insulin regulation by osteocalcin(as shown in Figure 11-summary of the osteocalcin). To make substantial progress in this area, it is recommended that the terms “osteocalcin gene”, “osteoporosis”, “type 2 diabetes mellitus”, “different populations”, “older men”, “pregnant women”, “diabetes-related complications”, “treatment and correlation studies” and other key topics.
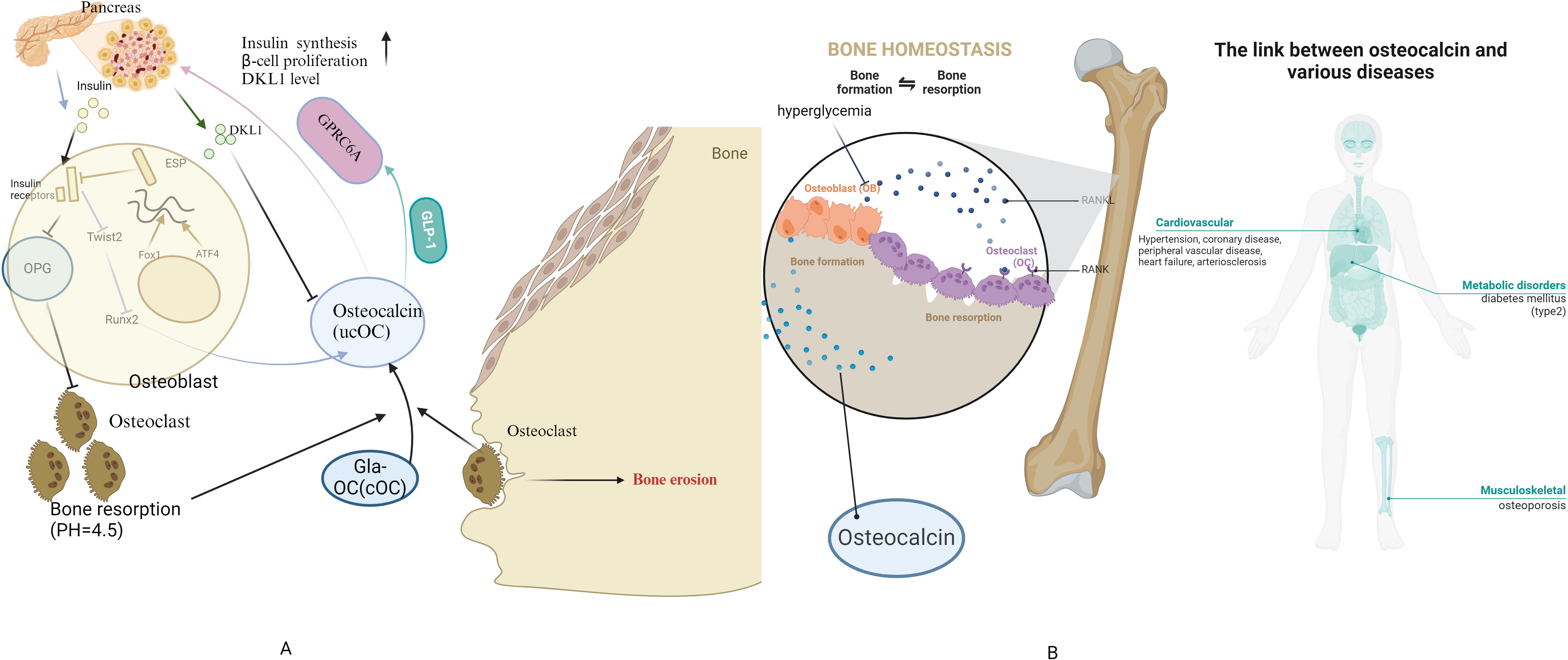
Figure 11. Summary of the osteocalcin (A) Physiological mechanisms of osteocalcin-insulin interactions (B) link between osteocalcin and diseases.
Data availability statement
The original contributions presented in the study are included in the article/supplementary material. Further inquiries can be directed to the corresponding author.
Author contributions
ZL: Conceptualization, Data curation, Formal analysis, Methodology, Software, Writing – original draft. YM: Investigation, Data curation, Writing – original draft, Writing – review & editing. KY: Writing – original draft, Writing – review & editing. SW: Conceptualization, Data curation, Supervision, Validation, Visualization, Writing – original draft. FZ: Funding acquisition, Resources, Writing – review & editing.
Funding
The author(s) declare financial support was received for the research, authorship, and/or publication of this article. Grant NO. 20224ACB216003, Science&Technology Department of JiangXi Province, Grant NO. 82260169, National Natural Science Foundation of China.
Acknowledgments
Thanks to VoSviewer and BiblioShiny for providing technical support in image processing for this paper, and special thanks to Professor Sheng Huang for valuable comments and suggestions. Due to strict page limitations, we are unable to include as many important original papers as we would like in the references, and we sincerely apologize to many of our colleagues for this.
Conflict of interest
The authors declare that the research was conducted in the absence of any commercial or financial relationships that could be construed as a potential conflict of interest.
Publisher’s note
All claims expressed in this article are solely those of the authors and do not necessarily represent those of their affiliated organizations, or those of the publisher, the editors and the reviewers. Any product that may be evaluated in this article, or claim that may be made by its manufacturer, is not guaranteed or endorsed by the publisher.
Abbreviations
BMD, bone mineral density; Gla, γ-carboxyglutamic acid; BGP, Bone Gla Protein; OC, Osteocalcin; cOC, carboxylated; OC, ucOC udercarboxylated OC; GLP-1,glucagon-like peptide-1; DLK1, delta like-1; WoS, Web of Science; WoSCC, Web of science core collection; JIC, Journal Citation Indicator; TC, total citation; BMP7, Bone Morphogenetic Protein 7; RANKL, Receptor Activator of Nuclear Factor-κ B Ligand; SPP1, secreted phosphoprotein 1 Gene 1; 25D, 1,25-dihydroxyvitamin D3; Runx2, Runt-related transcription factor 2; PTH, parathyroid hormone; BTM, bone turnover marker; BTMs, bone turnover markers; T2D, type 2 diabetes TyG, triglyceride-glucose; TIR, time-range; CGM, continuous glucose monitoring; T2DM, type 2 diabetes mellitus; MV, Mogroside V; BMSCs, bone marrow mesenchymal stem cells; ICA, Icariin; NAR, naringin sTfR:soluble transferrin receptor.
References
1. Collaborators GBDD. Global, regional, and national burden of diabetes from 1990 to 2021, with projections of prevalence to 2050: a systematic analysis for the Global Burden of Disease Study 2021. Lancet. (2023) 402:203–34. doi: 10.1016/S0140-6736(23)01301-6
2. Lv Q, Li Z, Sui A, Yang X, Han Y, Yao R. The role and mechanisms of gut microbiota in diabetic nephropathy, diabetic retinopathy and cardiovascular diseases. Front Microbiol. (2022) 13:977187. doi: 10.3389/fmicb.2022.977187
3. Martiniakova M, Biro R, Kovacova V, Babikova M, Zemanova N, Mondockova V, et al. Current knowledge of bone-derived factor osteocalcin: its role in the management and treatment of diabetes mellitus, osteoporosis, osteopetrosis and inflammatory joint diseases. J Mol Med. (2024) 102:435–52. doi: 10.1007/s00109-024-02418-8
4. Kanazawa I. Osteocalcin as a hormone regulating glucose metabolism. World J Diabetes. (2015) 6:1345–54. doi: 10.4239/wjd.v6.i18.1345
5. Hauschka PV, Lian JB, Cole DE, Gundberg. Osteocalcin CM. and matrix Gla protein: vitamin K-dependent proteins in bone. Physiol Rev. (1989) 69:990–1047. doi: 10.1152/physrev.1989.69.3.990
6. Mizokami A, Kawakubo-Yasukochi T, Hirata. Osteocalcin M. and its endocrine functions. Biochem Pharmacol. (2017) 132:1–8. doi: 10.1016/j.bcp.2017.02.001
7. Pi M, Chen L, Huang MZ, Zhu W, Ringhofer B, Luo J, et al. GPRC6A null mice exhibit osteopenia, feminization and metabolic syndrome. PloS One. (2008) 3:e3858. doi: 10.1371/journal.pone.0003858
8. Ferron M, Hinoi E, Karsenty G, Ducy. Osteocalcin differentially regulates beta cell P. and adipocyte gene expression and affects the development of metabolic diseases in wild-type mice. Proc Natl Acad Sci United States America. (2008) 105:5266–70. doi: 10.1073/pnas.0711119105
9. Mizokami A, Yasutake Y, Gao J, Matsuda M, Takahashi I, Takeuchi H, et al. Osteocalcin induces release of glucagon-like peptide-1 and thereby stimulates insulin secretion in mice. PloS One. (2013) 8:e57375. doi: 10.1371/journal.pone.0057375
10. Lin X, Parker L, McLennan E, Zhang X, Hayes A, McConell G, et al. Recombinant uncarboxylated osteocalcin per se enhances mouse skeletal muscle glucose uptake in both extensor digitorum longus and soleus muscles. Front Endocrinol (Lausanne). (2017) 8:330. doi: 10.3389/fendo.2017.00330
11. Levinger I, Lin X, Zhang X, Brennan-Speranza TC, Volpato B, Hayes A, et al. The effects of muscle contraction and recombinant osteocalcin on insulin sensitivity ex vivo. Osteoporosis international: J established as result cooperation between Eur Foundation Osteoporosis Natl Osteoporosis Foundation USA. (2016) 27:653–63. doi: 10.1007/s00198-015-3273-0
12. Mera P, Laue K, Wei JW, Berger JM, Karsenty G. Osteocalcin signaling in myofibers is necessary and sufficient for optimum adaptation to exercise. Cell Metab. (2016) 23:1078–92. doi: 10.1016/j.cmet.2016.05.004
13. Zhang XL, Wang YN, Ma LY, Liu ZS, Ye F, Yang JH. Uncarboxylated osteocalcin ameliorates hepatic glucose and lipid metabolism in KKAy mice via activating insulin signaling pathway. Acta Pharmacol Sin. (2020) 41:383–93. doi: 10.1038/s41401-019-0311-z
14. Zoch ML, Clemens TL, Riddle. RC. New insights into the biology of osteocalcin. Bone. (2016) 82:42–9. doi: 10.1016/j.bone.2015.05.046
15. Dirckx N, Moorer MC, Clemens TL, Riddle RC. The role of osteoblasts in energy homeostasis. NATURE REVIEWS ENDOCRINOLOGY (2019) 15:651–65. doi: 10.1038/s41574-019-0246-y
16. Kunutsor SK, Apekey TA, Laukkanen. JA. Association of serum total osteocalcin with type 2 diabetes and intermediate metabolic phenotypes: systematic review and meta-analysis of observational evidence. Eur J Epidemiol. (2015) 30:599–614. doi: 10.1007/s10654-015-0058-x
17. Liu DM, Mosialou I, Liu. JM. Bone: Another potential target to treat, prevent and predict diabetes. Diabetes Obes Metab. (2018) 20:1817–28. doi: 10.1111/dom.13330
18. Li Y, Gu Z, Wang J, Wang Y, Chen X, Dong B. The emerging role of bone-derived hormones in diabetes mellitus and diabetic kidney disease. Front Endocrinol (Lausanne). (2022) 13:938830. doi: 10.3389/fendo.2022.938830
19. van Eck NJ, Waltman L. Software survey: VOSviewer, a computer program for bibliometric mapping. Scientometrics. (2010) 84:523–38. doi: 10.1007/s11192-009-0146-3
20. van Eck NJ, Waltman L. Citation-based clustering of publications using CitNetExplorer and VOSviewer. Scientometrics. (2017) 111:1053–70. doi: 10.1007/s11192-017-2300-7
21. Kerner SA, Scott RA, Pike JW. Sequence elements in the human osteocalcin gene confer basal activation and inducible response to hormonal vitamin-D3. Proc Natl Acad Sci U. S. A. (1989) 86:4455–9. doi: 10.1073/pnas.86.12.4455
22. Booth SL, Centi A, Smith SR, Gundberg C. The role of osteocalcin in human glucose metabolism: marker or mediator. Nat Rev Endocrinol. (2013) 9:43–55. doi: 10.1038/nrendo.2012.201
23. Wang YR, Zhang HJ. Serum 25-hydroxyvitamin D3 levels are associated with carotid intima-media thickness and carotid atherosclerotic plaque in type 2 diabetic patients. J Diabetes Res. (2017) 2017:6. doi: 10.1155/2017/3510275
24. Xie H, Tang SY, Luo XH, Huang J, Cui RR, Yuan LQ, et al. Insulin-like effects of visfatin on human osteoblasts. Calcified Tissue Int. (2007) 80:201–10. doi: 10.1007/s00223-006-0155-7
25. Ferron M, Hinoi E, Karsenty G, Ducy P. Osteocalcin differentially regulates β cell and adipocyte gene expression and affects the development of metabolic diseases in wild-type mice. Proc Natl Acad Sci United States America. (2008) 105:5266–70. doi: 10.1073/pnas.0711119105
26. Gaur T, Lengner CJ, Hovhannisyan H, Bhat RA, Bodine PVN, Komm BS, et al. Canonical WNT signaling promotes osteogenesis by directly stimulating Runx2 gene expression. J Biol Chem. (2005) 280:33132–40. doi: 10.1074/jbc.M500608200
27. Ferron M, Wei JW, Yoshizawa T, Del Fattore A, DePinho RA, Teti A, et al. Insulin signaling in osteoblasts integrates bone remodeling and energy metabolism. Cell. (2010) 142:296–308. doi: 10.1016/j.cell.2010.06.003
28. Bennett CN, Longo KA, Wright WS, Suva LJ, Lane TF, Hankenson KD, et al. Regulation of osteoblastogenesis and bone mass by Wnt10b. Proc Natl Acad Sci United States America. (2005) 102:3324–9. doi: 10.1073/pnas.0408742102
29. Lips P. Vitamin D physiology. Prog Biophysics Mol Biol. (2006) 92:4–8. doi: 10.1016/j.pbiomolbio.2006.02.016
30. Lindsay R, Nieves J, Formica C, Henneman E, Woelfert L, Shen V, et al. 1997 Randomised controlled study of effect of parathyroid hormone on vertebral-bone mass and fracture incidence among postmenopausal women on oestrogen with osteoporosis. Lancet. (1997) 350:550–5. doi: 10.1016/s0140-6736(97)02342-8
31. Demay MB, Kiernan MS, Deluca HF, Kronenberg HM. Sequences in the human parathyroid-hormone gene that bind the 1,25-dihydroxyvitamin-D3 receptor and mediate transcriptional repression in response to 1,25-dihydroxyvitamin-D3. Proc Natl Acad Sci United States America. (1992) 89:8097–101. doi: 10.1073/pnas.89.17.8097
32. Grey A, Bolland M, Gamble G, Wattie D, Horne A, Davidson J, et al. The peroxisome proliferator-activated receptor-γ agonist rosiglitazone decreases bone formation and bone mineral density in healthy postmenopausal women:: A randomized, controlled trial. J Clin Endocrinol Metab. (2007) 92:1305–10. doi: 10.1210/jc.2006-2646
33. Fulzele K, Riddle RC, DiGirolamo DJ, Cao XM, Wan C, Chen DQ, et al. Insulin receptor signaling in osteoblasts regulates postnatal bone acquisition and body composition. Cell. (2010) 142:309–19. doi: 10.1016/j.cell.2010.06.002
34. Welt CK, Chan JL, Bullen J, Murphy R, Smith P, DePaoli AM, et al. Recombinant human leptin in women with hypothalamic amenorrhea. New Engl J Med. (2004) 351:987–97. doi: 10.1056/NEJMoa040388
35. Thomas T, Gori F, Khosla S, Jensen MD, Burguera B, Riggs BL. Leptin acts on human marrow stromal cells to enhance differentiation to osteoblasts and to inhibit differentiation to adipocytes. Endocrinology. (1999) 140:1630–8. doi: 10.1210/en.140.4.1630
36. Kindblom JM, Ohlsson C, Ljunggren Ö, Karlsson MK, Tivesten Å, Smith U, et al. Plasma osteocalcin is inversely related to fat mass and plasma glucose in elderly swedish men. J Bone Mineral Res. (2009) 24:785–91. doi: 10.1359/jbmr.081234
37. Kanazawa I, Yamaguchi T, Yamamoto M, Yamauchi M, Kurioka S, Yano S, et al. Serum osteocalcin level is associated with glucose metabolism and atherosclerosis parameters in type 2 diabetes mellitus. J Clin Endocrinol Metab. (2009) 94:45–9. doi: 10.1210/jc.2008-1455
38. Pittas AG, Harris SS, Eliades M, Stark P, Dawson-Hughes. Association between Serum Osteocalcin B. and markers of metabolic phenotype. J Clin Endocrinol Metab. (2009) 94:827–32. doi: 10.1210/jc.2008-1422
39. Rix M, Andreassen H, Eskildsen P, Langdahl B, Olgaard. Bone mineral density K. and biochemical markers of bone turnover in patients with predialysis chronic renal failure. Kidney Int. (1999) 56:1084–93. doi: 10.1046/j.1523-1755.1999.00617.x
40. Aoki A, Muneyuki T, Yoshida M, Munakata H, Ishikawa SE, Sugawara H, et al. Circulating osteocalcin is increased in early-stage diabetes. Diabetes Res Clin Pract. (2011) 92:181–6. doi: 10.1016/j.diabres.2011.01.009
41. Kanazawa I, Yamaguchi T, Yamauchi M, Yamamoto M, Kurioka S, Yano S, et al. Serum undercarboxylated osteocalcin was inversely associated with plasma glucose level and fat mass in type 2 diabetes mellitus. Osteoporosis Int. (2011) 22:187–94. doi: 10.1007/s00198-010-1184-7
42. Papastefanou I, Eleftheriades M, Kappou D, Lambrinoudaki I, Lavranos D, Pervanidou P, et al. Maternal serum osteocalcin at 11-14 weeks of gestation in gestational diabetes mellitus. Eur J Clin Invest. (2015) 45:1025–31. doi: 10.1111/eci.12500
43. Saucedo R, Rico G, Vega G, Basurto L, Cordova L, Galvan R, et al. Osteocalcin, under-carboxylated osteocalcin and osteopontin are not associated with gestational diabetes mellitus but are inversely associated with leptin in non-diabetic women. J Endocrinological Invest. (2015) 38:519–26. doi: 10.1007/s40618-014-0220-4
44. Ferron M, McKee MD, Levine RL, Ducy P, Karsenty G. Intermittent injections of osteocalcin improve glucose metabolism and prevent type 2 diabetes in mice. Bone. (2012) 50:568–75. doi: 10.1016/j.bone.2011.04.017
45. Yeap BB, Chubb SAP, Flicker L, McCaul KA, Ebeling PRE, Beilby JP, et al. Reduced serum total osteocalcin is associated with metabolic syndrome in older men via waist circumference, hyperglycemia, and triglyceride levels. Eur J Endocrinol. (2010) 163:265–72. doi: 10.1530/eje-10-0414
46. Haussler MR, Haussler CA, Jurutka PW, Thompson PD, Hsieh JC, Remus LS, et al. The vitamin D hormone and its nuclear receptor: molecular actions and disease states. J Endocrinol. (1997) 154:S57–S73. doi: 10.1677/joe.1670.1154S1057
47. Fernández-Real JM, Izquierdo M, Ortega F, Gorostiaga E, Gomez-Ambrosi J, Moreno-Navarrete JM, et al. The relationship of serum osteocalcin concentration to insulin secretion, sensitivity, and disposal with hypocaloric diet and resistance training. J Clin Endocrinol Metab. (2009) 94:237–45. doi: 10.1210/jc.2008-0270
48. Lee NK, Sowa H, Hinoi E, Ferron M, Ahn JD, Confavreux C, et al. Endocrine regulation of energy metabolism by the skeleton. Cell. (2007) 130:456–69. doi: 10.1016/j.cell.2007.05.047
49. Rodda SJ, McMahon. Distinct roles for Hedgehog AP. and canonical Wnt signaling in specification, differentiation and maintenance of osteoblast progenitors. Development. (2006) 133:3231–44. doi: 10.1242/dev.02480
50. Harvey CB, O'Shea PJ, Scott AJ, Robson H, Siebler T, Shalet SM, et al. Molecular mechanisms of thyroid hormone effects on bone growth and function. Mol Genet Metab. (2002) 75:17–30. doi: 10.1006/mgme.2001.3268
51. Cheng S, Xing W, Pourteymoor S, Mohan S. Effects of thyroxine (T4), 3,5,3’-triiodo-L-thyronine (T3) and their metabolites on osteoblast differentiation. Calcif Tissue Int. (2016) 99:435–42. doi: 10.1007/s00223-016-0159-x
52. Lindsey RC, Aghajanian P, Mohan S. Thyroid hormone signaling in the development of the endochondral skeleton. Vitamins hormones. (2018) 106:351–81. doi: 10.1016/bs.vh.2017.06.003
53. Murphy E, Williams GR. The thyroid and the skeleton. Clin Endocrinol (Oxf). (2004) 61:285–98. doi: 10.1111/j.1365-2265.2004.02053.x
54. Mundy GR, Shapiro JL, Bandelin JG, Canalis EM, Raisz. LG. Direct stimulation of bone resorption by thyroid hormones. J Clin Invest. (1976) 58:529–34. doi: 10.1172/jci108497
55. Martini G, Gennari L, De Paola V, Pilli T, Salvadori S, Merlotti D, et al. The effects of recombinant TSH on bone turnover markers and serum osteoprotegerin and RANKL levels. Thyroid. (2008) 18:455–60. doi: 10.1089/thy.2007.0166
56. Nicholls JJ, Brassill MJ, Williams GR, Bassett JH. The skeletal consequences of thyrotoxicosis. J Endocrinol. (2012) 213:209–21. doi: 10.1530/joe-12-0059
57. Mihaljević O, Živančević-Simonović S, Lučić-Tomić A, Živković I, Minić R, Mijatović-Teodorović L, et al. The association of circulating sclerostin level with markers of bone metabolism in patients with thyroid dysfunction. J Med Biochem. (2020) 39:436–43. doi: 10.5937/jomb0-24943
58. Kojima N, Sakata S, Nakamura S, Nagai K, Takuno H, Ogawa T, et al. Serum concentrations of osteocalcin in patients with hyperthyroidism, hypothyroidism and subacute thyroiditis. J Endocrinol Invest. (1992) 15:491–6. doi: 10.1007/bf03348786
59. Ishisaki A, Tokuda H, Yoshida M, Hirade K, Kunieda K, Hatakeyama D, et al. Activation of p38 mitogen-activated protein kinase mediates thyroid hormone-stimulated osteocalcin synthesis in osteoblasts. Mol Cell Endocrinol. (2004) 214:189–95. doi: 10.1016/j.mce.2003.10.049
60. Gorka J, Taylor-Gjevre R, Arnason T. Metabolic and clinical consequences of hyperthyroidism on bone density. Int J Endocrinol. (2013) 2013:638727. doi: 10.1155/2013/638727
61. Akalin A, Colak Ö, Alatas Ö, Efe. Bone remodelling markers B. and serum cytokines in patients with hyperthyroidism. Clin Endocrinol. (2002) 57:125–9. doi: 10.1046/j.1365-2265.2002.01578.x
62. Jilka. Molecular RL. and cellular mechanisms of the anabolic effect of intermittent PTH. Bone. (2007) 40:1434–46. doi: 10.1016/j.bone.2007.03.017
63. Verheijen MH, Defize LH. Parathyroid hormone activates mitogen-activated protein kinase via a cAMP-mediated pathway independent of Ras. J Biol Chem. (1997) 272:3423–9. doi: 10.1074/jbc.272.6.3423
64. Swarthout JT, Doggett TA, Lemker JL, Partridge NC. Stimulation of extracellular signal-regulated kinases and proliferation in rat osteoblastic cells by parathyroid hormone is protein kinase C-dependent. J Biol Chem. (2001) 276:7586–92. doi: 10.1074/jbc.M007400200
65. Jüppner H, Abou-Samra AB, Freeman M, Kong XF, Schipani E, Richards J, et al. A G protein-linked receptor for parathyroid hormone and parathyroid hormone-related peptide. Science. (1991) 254:1024–6. doi: 10.1126/science.1658941
66. Boguslawski G, et al. Activation of osteocalcin transcription involves interaction of protein kinase A- and protein kinase C-dependent pathways. (2000) 275:999–1006. doi: 10.1074/jbc.275.2.999
67. Jiang D, Franceschi RT, Boules H, Xiao G. Parathyroid hormone induction of the osteocalcin gene. Requirement for an osteoblast-specific element 1 sequence in the promoter and involvement of multiple-signaling pathways. J Biol Chem. (2004) 279:5329–37. doi: 10.1074/jbc.M311547200
68. Schafer AL, Sellmeyer DE, Schwartz AV, Rosen CJ, Vittinghoff E, Palermo L, et al. Change in undercarboxylated osteocalcin is associated with changes in body weight, fat mass, and adiponectin: parathyroid hormone (1-84) or alendronate therapy in postmenopausal women with osteoporosis (the PaTH study). J Clin Endocrinol Metab. (2011) 96:E1982–1989. doi: 10.1210/jc.2011-0587
69. Kurland ES, Cosman F, McMahon DJ, Rosen CJ, Lindsay R, Bilezikian JP. Parathyroid hormone as a therapy for idiopathic osteoporosis in men: effects on bone mineral density and bone markers. J Clin Endocrinol Metab. (2000) 85:3069–76. doi: 10.1210/jcem.85.9.6818
70. Li Y, Hu Z, Zhou C, Xu Y, Huang L, Wang X, et al. Intermittent parathyroid hormone (PTH) promotes cementogenesis and alleviates the catabolic effects of mechanical strain in cementoblasts. BMC Cell Biol. (2017) 18:19. doi: 10.1186/s12860-017-0133-0
71. Chen AB, Minami K, Raposo JF, Matsuura N, Koizumi M, Yokota H, et al. Transient modulation of calcium and parathyroid hormone stimulates bone formation. Endocrine. (2016) 54:232–40. doi: 10.1007/s12020-016-1066-7
72. Ducy P, Zhang R, Geoffroy V, Ridall AL, Karsenty G. Osf2/Cbfa1: A transcriptional activator of osteoblast differentiation. Cell. (1997) 89:747–54. doi: 10.1016/s0092-8674(00)80257-3
73. Picotto G, Liaudat AC, Bohl L, Talamoni NT. Molecular aspects of vitamin D anticancer activity. Cancer Invest. (2012) 30:604–14. doi: 10.3109/07357907.2012.721039
74. Ducy P, Desbois C, Boyce B, Pinero G, Story B, Dunstan C, et al. Increased bone formation in osteocalcin-deficient mice. Nature. (1996) 382:448–52. doi: 10.1038/382448a0
75. Boskey AL, Gadaleta S, Gundberg C, Doty SB, Ducy P, Karsenty G. Fourier transform infrared microspectroscopic analysis of bones of osteocalcin-deficient mice provides insight into the function of osteocalcin. Bone. (1998) 23:187–96. doi: 10.1016/S8756-3282(98)00092-1
76. Shearer MJ. Role of vitamin K and Gla proteins in the pathophysiology of osteoporosis and vascular calcification. Curr Opin Clin Nutr Metab Care. (2000) 3:433–8. doi: 10.1097/00075197-200011000-00004
77. Wu YY, Yu T, Zhang XH, Liu YS, Li F, Wang YY. 1,25(OH)2D3 inhibits the deleterious effects induced by high glucose on osteoblasts through undercarboxylated osteocalcin and insulin signaling. J Steroid Biochem Mol Biol. (2012) 132:112–9. doi: 10.1016/j.jsbmb.2012.05.002
78. Usman N, Qaseem A, Jayaraj JS, Fathima N, Janapala RN. Drug-induced reduction of gamma carboxylation in osteocalcin: what is the fallback. Cureus. (2019) 11:e5504. doi: 10.7759/cureus.5504
79. Urano T, Shiraki M, Kuroda T, Tanaka S, Urano F, Uenishi K, et al. Low serum osteocalcin concentration is associated with incident type 2 diabetes mellitus in Japanese women. J Bone Mineral Metab. (2018) 36:470–7. doi: 10.1007/s00774-017-0857-0
80. Shu H, Pei Y, Chen K, Lu. Significant inverse association between serum osteocalcin JM. and incident type 2 diabetes in a middle-aged cohort. Diabetes-Metabolism Res Rev. (2016) 32:867–74. doi: 10.1002/dmrr.2808
81. Jin S, Chang XC, Wen J, Yang J, Ao N, Zhang KY, et al. Decarboxylated osteocalcin, a possible drug for type 2 diabetes, triggers glucose uptake in MG63 cells. World J Diabetes. (2021) 12:1102–15. doi: 10.4239/wjd.v12.i7.1102
82. Zhang X, Shen Y, Xu Y, Xiong Q, Lu Z, Ma X, et al. Association of serum osteocalcin levels with major adverse cardiovascular events: A 4.4-year retrospective cohort study. Clin Exp Pharmacol Physiol. (2018) 45:3–9. doi: 10.1111/1440-1681.12853
83. Keryakos HKH, Okaily NI, Boulis MAY, Salama. Osteocalcin AMS. and vascular calcification in hemodialysis patients: an observational cohort study. Int Urol Nephrol. (2021) 53:1015–23. doi: 10.1007/s11255-020-02753-y
84. Hwang YC, Kang M, Cho IJ, Jeong IK, Ahn KJ, Chung HY, et al. Association between the circulating total osteocalcin level and the development of cardiovascular disease in middle-aged men: A mean 8.7-year longitudinal follow-up study. J Atheroscl Thromb. (2015) 22:136–43. doi: 10.5551/jat.25718
85. Hu T, Shen Y, Xu Y, Wang Y, Bao Y, Ma X, et al. Low total osteocalcin levels are associated with the risk of cardiovascular events among women with hyperglycaemia: a 7.6-year prospective study. Endocrine. (2023) 82:47–56. doi: 10.1007/s12020-023-03428-1
86. Huang L, Yang L, Luo L, Wu P, Yan. Osteocalcin Improves Metabolic Profiles S. Body composition and arterial stiffening in an induced diabetic rat model. Exp Clin Endocrinol Diabetes. (2017) 125:234–40. doi: 10.1055/s-0042-122138
87. Jung CH, Lee WJ, Hwang JY, Lee MJ, Seol SM, Kim YM, et al. The preventive effect of uncarboxylated osteocalcin against free fatty acid-induced endothelial apoptosis through the activation of phosphatidylinositol 3-kinase/Akt signaling pathway. Metabolism-Clinical Exp. (2013) 62:1250–7. doi: 10.1016/j.metabol.2013.03.005
88. Evrard S, Delanaye P, Kamel S, Cristol JP, Cavalier E. Vascular calcification: from pathophysiology to biomarkers. Clinica Chimica Acta. (2015) 438:401–14. doi: 10.1016/j.cca.2014.08.034
89. Levy RJ, Gundberg C, Scheinman R. The identification of the vitamin K-dependent bone protein osteocalcin as one of the gamma-carboxyglutamic acid containing proteins present in calcified atherosclerotic plaque and mineralized heart-valves. Atherosclerosis. (1983) 46:49–56. doi: 10.1016/0021-9150(83)90163-6
90. Proudfoot D, Davies JD, Skepper JN, Weissberg PL, Shanahan CM. Acetylated low-density lipoprotein stimulates human vascular smooth muscle cell calcification by promoting osteoblastic differentiation and inhibiting phagocytosis. Circulation. (2002) 106:3044–50. doi: 10.1161/01.CIR.0000041429.83465.41
91. Millar SA, Patel H, Anderson SI, England TJ, O’Sullivan. SE. Osteocalcin, vascular calcification, and atherosclerosis: A systematic review and meta-analysis. Front Endocrinol. (2017) 8:183. doi: 10.3389/fendo.2017.00183
92. Polgreen LE, Jacobs DR Jr, Nathan BM, Steinberger J, Moran A, Sinaiko AR. Association of osteocalcin with obesity, insulin resistance, and cardiovascular risk factors in young adults. Obes (Silver Spring Md.). (2012) 20:2194–201. doi: 10.1038/oby.2012.108
93. Chandar N, Campbell P, Novak J, Smith M. Dependence of induction of osteocalcin gene-expression on the presence of wild-type P53 in a murine osteosarcoma cell-line. Mol Carcinogenesis. (1993) 8:299–305. doi: 10.1002/mc.2940080413
94. Amaral CB, Leite JD, Fonseca ABM, Ferreira. Vimentin AMR. osteocalcin and osteonectin expression in canine primary bone tumors: diagnostic and prognostic implications. Mol Biol Rep. (2018) 45:1289–96. doi: 10.1007/s11033-018-4285-6
95. Benayahu D, Shur I, Marom R, Meller I, Issakov. Cellular J. and molecular properties associated with osteosarcoma cells. J Cell Biochem. (2002) 84:108–14. doi: 10.1002/jcb.1270
96. Masuda H, Miller C, Koeffler HP, Battifora H, Cline MJ. Rearrangement of the P53 gene in human osteogenic sarcomas. Proc Natl Acad Sci United States America. (1987) 84:7716–9. doi: 10.1073/pnas.84.21.7716
97. Chen H, Hays E, Liboon J, Neely C, Kolman K, Chandar N. Osteocalcin gene expression is regulated by wild-type p53. Calcified Tissue Int. (2011) 89:411–8. doi: 10.1007/s00223-011-9533-x
98. El-Badawi ZH, Muhammad EMS, Noaman HH. Role of immunohistochemical cyclo-oxygenase-2 (COX-2) and osteocalcin in differentiating between osteoblastomas and osteosarcomas. Malaysian J Pathol. (2012) 34:15–23. doi: 10.1016/s0006-2952(00)00582-7
99. Shirakawa T, Ko SC, Gardner TA, Cheon J, Miyamoto T, Gotoh A, et al. In vivo suppression of osteosarcoma pulmonary metastasis with intravenous osteocalcin promoter-based toxic gene therapy. Cancer Gene Ther. (1998) 5:274–80. doi: 10.3892/or.9.2.337
100. Dougall WC, Glaccum M, Charrier K, Rohrbach K, Brasel K, De Smedt T, et al. RANK is essential for osteoclast and lymph node development. Genes Dev. (1999) 13:2412–24. doi: 10.1101/gad.13.18.2412
101. Wittrant Y, Gorin Y, Woodruff K, Horn D, Abboud HE, Mohan S, et al. High d(+)glucose concentration inhibits RANKL-induced osteoclastogenesis. Bone. (2008) 42:1122–30. doi: 10.1016/j.bone.2008.02.006
102. Hinoi E, Gao N, Jung DY, Yadav V, Yoshizawa T, Myers MG, et al. The sympathetic tone mediates leptin’s inhibition of insulin secretion by modulating osteocalcin bioactivity. J Cell Biol. (2008) 183:1235–42. doi: 10.1083/jcb.200809113
103. Kode A, Mosialou I, Silva BC, Joshi S, Ferron M, Rached MT, et al. FoxO1 protein cooperates with ATF4 protein in osteoblasts to control glucose homeostasis. J Biol Chem. (2012) 287:8757–68. doi: 10.1074/jbc.M111.282897
104. Starup-Linde J, Lykkeboe S, Gregersen S, Hauge EM, Langdahl BL, Handberg A, et al. Differences in biochemical bone markers by diabetes type and the impact of glucose. Bone. (2016) 83:149–55. doi: 10.1016/j.bone.2015.11.004
105. Shao X, Cao X, Song G, Zhao Y, Shi B. Metformin rescues the MG63 osteoblasts against the effect of high glucose on proliferation. J Diabetes Res. (2014) 2014:453940. doi: 10.1155/2014/453940
106. Meier C, Eastell R, Pierroz DD, Lane NE, Al-Daghri N, Suzuki A, et al. Biochemical markers of bone fragility in patients with diabetes. J Clin Endocrinol Metab. (2023) 108(10):e923–e936. doi: 10.1210/clinem/dgad255
107. Holick MF, Eastell R, Pierroz DD, Lane NE, Al-Daghri N, Suzuki A, et al. Evaluation, treatment, and prevention of vitamin D deficiency: an endocrine society clinical practice guideline. J Clin Endocrinol Metab. (2011) 96:1911–30. doi: 10.1210/jc.2011-0385
108. Booth FW, Roberts CK, Laye MJ. Lack of exercise is a major cause of chronic diseases. Compr Physiol. (2012) 2:1143–211. doi: 10.1002/cphy.c110025
109. Teitelbaum SL. Bone resorption by osteoclasts. Science. (2000) 289:1504–8. doi: 10.1126/science.289.5484.1504
110. Klibanski A, Adams-Campbell L, Bassford T, Blair SN, Boden SD, Dickersin K, et al. Osteoporosis prevention, diagnosis, and therapy. Jama-Journal Am Med Assoc. (2001) 285:785–95. doi: 10.1001/jama.285.6.785
111. Lumachi F, Ermani M, Camozzi V, Tombolan V, Luisetto G.Changes of bone formation markers osteocalcin and bone-specific alkaline phosphatase in postmenopausal women with osteoporosis[J]. Annals of the New York Academy of Sciences (2009) 1173(Suppl 1):E60–63. doi: 10.1111/j.1749-6632.2009.04953.x
112. Jagtap VR, Ganu JV, Nagane. BMD NS. and serum intact osteocalcin in postmenopausal osteoporosis women. Indian J Clin biochemistry: IJCB. (2011) 26:70–3. doi: 10.1007/s12291-010-0074-2
113. Brown JP, Delmas PD, Malaval L, Edouard C, Chapuy MC, Meunier PJ. Serum bone gla-protein - A specific marker for bone-formation in postmenopausal osteoporosis. Lancet. (1984) 1:1091–3. doi: 10.1016/s0140-6736(84)92506-6
114. Pernow Y, Granberg B, Sääf M, Weidenhielm L. Osteoblast dysfunction in male idiopathic osteoporosis. Calcified Tissue Int. (2006) 78:90–7. doi: 10.1007/s00223-005-0158-9
115. Szulc P, Garnero P, Munoz F, Marchand F, Delmas PD. Cross-sectional evaluation of bone metabolism in men. J Bone Mineral Res. (2001) 16:1642–50. doi: 10.1359/jbmr.2001.16.9.1642
116. Ruiz-Gaspà S, Blanch-Rubió J, Ciria-Recasens M, Monfort J, Tío L, Garcia-Giralt N, et al. Reduced proliferation and osteocalcin expression in osteoblasts of male idiopathic Osteoporosis. Calcified Tissue Int. (2010) 86:220–6. doi: 10.1007/s00223-010-9336-5
117. Tabatabaei N, Giguère Y, Forest JC, Rodd CJ, Kremer R, Weiler HA. Osteocalcin is higher across pregnancy in caucasian women with gestational diabetes mellitus. Can J Diabetes. (2014) 38:307–13. doi: 10.1016/j.jcjd.2014.02.007
118. Im JA, Yu BP, Jeon JY, Kim SH. Relationship between osteocalcin and glucose metabolism in postmenopausal women. Clinica Chimica Acta. (2008) 396:66–9. doi: 10.1016/j.cca.2008.07.001
119. Yano S, Nabika T, Nagai A, Hamano T, Yamasaki M, Isomura M, et al. Interrelationship between glucose metabolism and undercarboxylated osteocalcin: a cross-sectional study in a community-dwelling population. Asia Pacific J Clin Nutr. (2015) 24:489–95. doi: 10.6133/apjcn.2015.24.4.02
120. Huang HJ, Wang A, Cong L, Zeng YJ. Osteocalcin is associated with triglyceride glucose index rather than HOMA-IR in men with type 2 diabetes. Front Endocrinol. (2022) 13:1067903. doi: 10.3389/fendo.2022.1067903
121. García-Martín A, Cortés-Berdonces M, Luque-Fernández I, Rozas-Moreno P, Quesada-Charneco M, Muñoz-Torres M. Osteocalcin as a marker of metabolic risk in healthy postmenopausal women. Menopause-the J North Am Menopause Soc. (2011) 18:537–41. doi: 10.1097/gme.0b013e3181f8565e
122. Liu XY, Yeap BB, Brock KE, Levinger I, Golledge J, Flicker L, et al. Associations of osteocalcin forms with metabolic syndrome and its individual components in older men: the health in men study. J Clin Endocrinol Metab. (2021) 106:E3506–18. doi: 10.1210/clinem/dgab358
123. Vestergaard P. Bone metabolism in type 2 diabetes and role of thiazolidinediones. Curr Opin Endocrinol Diabetes Obes. (2009) 16:125–31. doi: 10.1097/MED.0b013e328325d155
124. Saito M, Marumo K. Collagen cross-links as a determinant of bone quality: a possible explanation for bone fragility in aging, osteoporosis, and diabetes mellitus. Osteoporosis international: J established as result cooperation between Eur Foundation Osteoporosis Natl Osteoporosis Foundation USA. (2010) 21:195–214. doi: 10.1007/s00198-009-1066-z
125. Herskind AM, Christensen K, Nørgaard-Andersen K, Andersen. Diabetes mellitus JF. and healing of closed fractures. Diabete metabolisme. (1992) 18:63–4. doi: 10.1097/00003086-198807000-00028
126. Loder RT. The influence of diabetes mellitus on the healing of closed fractures. Clin Orthop Relat Res. (1988) 232:210–6. doi: 10.1097/00003086-198807000-00028
127. Rains JL, Jain SK. Oxidative stress, insulin signaling, and diabetes. Free Radical Biol Med. (2011) 50:567–75. doi: 10.1016/j.freeradbiomed.2010.12.006
128. Pisoschi AM, Pop A. The role of antioxidants in the chemistry of oxidative stress: A review. Eur J medicinal Chem. (2015) 97:55–74. doi: 10.1016/j.ejmech.2015.04.040
129. An Y, Zhang H, Wang C, Jiao F, Xu H, Wang X, et al. Activation of ROS/MAPKs/NF-κB/NLRP3 and inhibition of efferocytosis in osteoclast-mediated diabetic osteoporosis. FASEB journal: Off Publ Fed Am Societies Exp Biol. (2019) 33:12515–27. doi: 10.1096/fj.201802805RR
130. Wu CC, Econs MJ, DiMeglio LA, Insogna KL, Levine MA, Orchard PJ, et al. Diagnosis and management of osteopetrosis: consensus guidelines from the osteopetrosis working group. J Clin Endocrinol Metab. (2017) 102:3111–23. doi: 10.1210/jc.2017-01127
131. Burch J, Rice S, Yang H, Neilson A, Stirk L, Francis R, et al. Systematic review of the use of bone turnover markers for monitoring the response to osteoporosis treatment: the secondary prevention of fractures, and primary prevention of fractures in high-risk groups. Health Technol Assess (Winchester England). (2014) 18:1–180. doi: 10.3310/hta18110
132. Biver E, Chopin F, Coiffier G, Brentano TF, Bouvard B, Garnero P, et al. Bone turnover markers for osteoporotic status assessment? A systematic review of their diagnosis value at baseline in osteoporosis. Joint Bone Spine. (2012) 79:20–5. doi: 10.1016/j.jbspin.2011.05.003
133. Ye X, Yu R, Jiang FS, Hou XH, Wei L, Bao YQ, et al. Osteocalcin and risks of incident diabetes and diabetic kidney disease: A 4.6-year prospective cohort study. Diabetes Care. (2022) 45:830–6. doi: 10.2337/dc21-2113
134. Babey ME, Ewing SK, Strotmeyer ES, Napoli N, Schafer AL, Vittinghoff E, et al. No evidence of association between undercarboxylated osteocalcin and incident type 2 diabetes. J Bone mineral research: Off J Am Soc Bone Mineral Res. (2022) 37:876–84. doi: 10.1002/jbmr.4519
135. Liu J, Wei YH, Zang P, Wang W, Feng ZQ, Yuan YY, et al. Circulating osteocalcin is associated with time in range and other metrics assessed by continuous glucose monitoring in type 2 diabetes. Diabetol Metab Syndr. (2022) 14:8. doi: 10.1186/s13098-022-00863-4
136. Duman I, Tanriverdi G, Ozener HO. Effects of vitamin K2 administration on guided bone regeneration in diabetic rats. J periodontal Res. (2024) 59(5):993–1004. doi: 10.1111/jre.13287
137. Luo YC, Ye ZM, Li CP, Hong L, Li H. Mogroside V promotes osteogenic differentiation of bone marrow mesenchymal stem cells from diabetic mice by altering microRNA profiles. Comb. Chem High Throughput Screen. (2024) 108(10):e923–36. doi: 10.2174/0113862073299904240416114653
138. Zheng S, Hu GY, Li JH, Zheng J, Li YK. Icariin accelerates bone regeneration by inducing osteogenesis-angiogenesis coupling in rats with type 1 diabetes mellitus. World J Diabetes. (2024) 15:15. doi: 10.4239/wjd.v15.i4.769
139. Rodríguez VA, Picotto G, Rivoira MA, Rigalli A, Talamoni. NT. The combined treatment of insulin and naringin improves bone properties in rats with type 1 diabetes mellitus. 25Applied Physiol Nutr Metab. (2024) 49:213–22. doi: 10.1139/apnm-2023-0267
140. Liu JM, Rosen CJ, Ducy P, Kousteni S. Regulation of glucose handling by the skeleton: insights from mouse and human studies. Diabetes. (2016) 65:3225–32. doi: 10.2337/db16-0053
141. Abdallah BM, Ditzel N, Laborda J, Karsenty G, Kassem M. DLK1 regulates whole-body glucose metabolism: A negative feedback regulation of the osteocalcin-insulin loop. Diabetes. (2015) 64:3069–80. doi: 10.2337/db14-1642
142. Juanola-Falgarona M, Cándido-Fernández J, Salas-Salvadó J, Martínez-González MA, Estruch R, Fiol M, et al. Association between serum ferritin and osteocalcin as a potential mechanism explaining the iron-induced insulin resistance. PloS One. (2013) 8:7. doi: 10.1371/journal.pone.0076433
143. Levinger I, Jerums G, Stepto NK, Parker L, Serpiello FR, McConell GK, et al. The effect of acute exercise on undercarboxylated osteocalcin and insulin sensitivity in obese men. J Bone Mineral Res. (2014) 29:2571–6. doi: 10.1002/jbmr.2285
144. van Bommel EJM, de Jongh RT, Brands M, Heijboer AC, den Heijer M, Serlie MJ, et al. The osteoblast: Linking glucocorticoid-induced osteoporosis and hyperglycaemia? A post-hoc analysis of a randomised clinical trial. Bone. (2018) 112:173–6. doi: 10.1016/j.bone.2018.04.025
145. Xu YT, Wang YS, Ma XJ, Xiao YF, Wang YF, Bao YQ. The mediating role of the visceral fat area in the correlation between the serum osteocalcin levels and a prolonged QTc interval. Cytokine. (2020) 136:5. doi: 10.1016/j.cyto.2020.155261
146. Schwartz AV, Schafer AL, Grey A, Vittinghoff E, Palermo L, Lui LY, et al. Effects of antiresorptive therapies on glucose metabolism: Results from the FIT, HORIZON-PFT, and FREEDOM trials. Journal. Bone Mineral Res. (2013) 28:1348–54. doi: 10.1002/jbmr.1865
147. Sharma VC, Vidyasagar S, Sukumar CA, Krishna BN, Shree. Association between serum osteocalcin S. and atherosclerosis in Type-2 diabetes mellitus: a cross-sectional study. BMC Endocr. Disord. (2023) 23:8. doi: 10.1186/s12902-023-01462-8
Keywords: osteocalcin, diabetes mellitus, bibliometric analysis, VOSviewer, visualization
Citation: Liu Z, Mao Y, Yang K, Wang S and Zou F (2025) A trend of osteocalcin in diabetes mellitus research: bibliometric and visualization analysis. Front. Endocrinol. 15:1475214. doi: 10.3389/fendo.2024.1475214
Received: 03 August 2024; Accepted: 16 December 2024;
Published: 13 January 2025.
Edited by:
Marcia Hiriart, Universidad Nacional Autonoma de Mexico, MexicoReviewed by:
Ajay D. Rao, Temple University, United StatesHumberto Garcia-Ortiz, National Institute of Genomic Medicine (INMEGEN), Mexico
Copyright © 2025 Liu, Mao, Yang, Wang and Zou. This is an open-access article distributed under the terms of the Creative Commons Attribution License (CC BY). The use, distribution or reproduction in other forums is permitted, provided the original author(s) and the copyright owner(s) are credited and that the original publication in this journal is cited, in accordance with accepted academic practice. No use, distribution or reproduction is permitted which does not comply with these terms.
*Correspondence: Fang Zou, TmRlZnkxMzE4OEBuY3UuZWR1LmNu
†These authors have contributed equally to this work