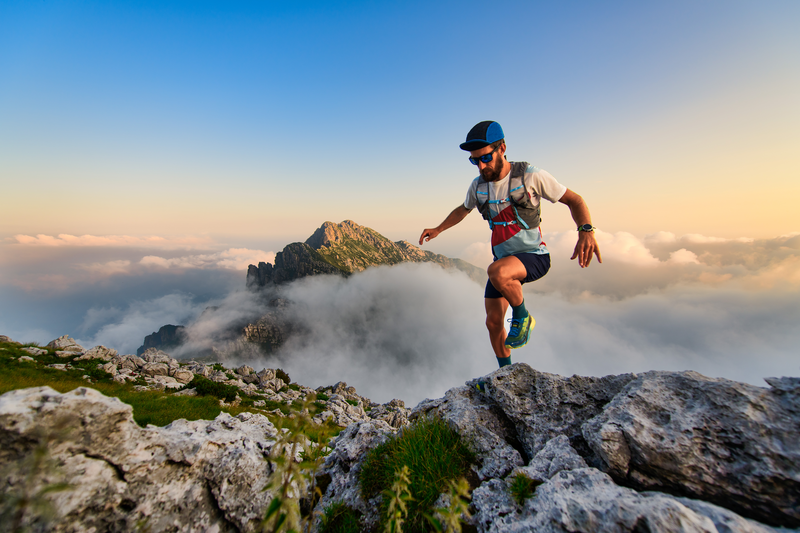
94% of researchers rate our articles as excellent or good
Learn more about the work of our research integrity team to safeguard the quality of each article we publish.
Find out more
REVIEW article
Front. Endocrinol. , 19 December 2024
Sec. Thyroid Endocrinology
Volume 15 - 2024 | https://doi.org/10.3389/fendo.2024.1472009
Thyroid diseases are increasingly prevalent, posing significant challenges to patients’ quality of life and placing substantial financial burdens on families and society. Despite these impacts, the underlying pathophysiology of many thyroid conditions remains poorly understood, complicating efforts in treatment, management, and prevention. Observational studies can identify associations between exposure variables and disease; however, they often struggle to account for confounding factors and reverse causation. Understanding disease occurrence, epidemiological trends, and clinical diagnosis, prevention, and treatment relies heavily on robust etiological research. Mendelian randomization, a method grounded in genetics and epidemiology, has been widely employed in studying the etiology of thyroid diseases, offering a solution to some of these challenges. This paper categorizes thyroid diseases into thyroid dysfunction and thyroid cancer, reviewing related Mendelian randomization studies. It further provides novel perspectives and approaches for investigating the mechanisms underlying thyroid diseases and designing intervention strategies.
Thyroid disorders can be broadly categorized into those primarily managed with medical treatments and those requiring surgical intervention. Thyroid diseases treated medically include hyperthyroidism, hypothyroidism, and thyroid inflammation, which are predominantly characterized by abnormalities in thyroid function. In contrast, surgical treatments address conditions such as goiter and thyroid tumors. With the increasing prevalence of thyroid diseases in clinical practice, understanding their causes is vital for effective treatment. Randomized controlled trials (RCTs) are often considered the “gold standard” for establishing causality due to their high level of evidence; however, they are constrained by substantial implementation costs and ethical considerations (1). Observational studies, while useful for identifying associations between environmental factors and disease, frequently struggle to demonstrate causality. Mendelian randomization (MR) offers a method to evaluate causal relationships between exposures and clinical outcomes by utilizing genes strongly associated with specific traits as instrumental variables. These genes serve as proxies for exposure factors in regression models, allowing for an indirect analysis of causal relationships (2). MR has distinct advantages in avoiding confounding factors, reverse causation, and selection bias. This is because genes are randomly assigned during gamete formation, are minimally influenced by external environmental factors post-birth, and exhibit a unidirectional relationship with exposure (3). In the context of thyroid diseases, MR is primarily applied to etiological research, investigating the effects of environmental factors, individual traits, and microorganisms on the occurrence, progression, and resolution of specific thyroid conditions. These studies provide innovative insights for improving treatment strategies and prognosis. This paper systematically reviews the application of MR in the study of thyroid dysfunction and thyroid cancer, along with their associated risk factors.
Data for this study were obtained from PubMed through a comprehensive search using keywords such as “Mendelian randomization,” “thyroid,” “thyroid function,” and “thyroid cancer.” The most recent search was performed on 1 June 2024. Non-English studies, reviews, case reports, observational studies, RCTs, and other non-MR research were excluded. Literature meeting the criteria for Mendelian randomized trials with exposure or outcome elements related to thyroid diseases was included. Two researchers independently evaluated the titles and abstracts of all identified studies. Discrepancies during the screening process were resolved through discussion or consultation with a third party.
The selection process for study inclusion and exclusion is illustrated in the flowchart (Figure 1). A total of 486 relevant articles were retrieved from the search, with 85 duplicates removed. After an initial screening of titles and abstracts, 299 articles were excluded, including RCTs, observational studies, reviews, and case reports that did not meet the inclusion criteria. Subsequently, 17 MR studies were excluded after full-text review due to incomplete data or other issues. Ultimately, 85 MR studies were included in the final review, comprising 67 studies on thyroid dysfunction and 18 on thyroid cancer.
Thyroid dysfunction is one of the most common endocrine disorders encountered in clinical practice. It primarily involves abnormal thyroid hormone secretion, which can significantly impact both physical and mental health. Patients with thyroid dysfunction are typically categorized into two groups: those with hyperthyroidism and those with hypothyroidism. Disorders such as Graves’ disease, Hashimoto’s thyroiditis, and autoimmune thyroiditis are also major contributors to thyroid dysfunction. Previous studies employing MR have explored causal relationships between factors such as gut microbiota, immune responses, cytokines, thyroid disorders, and related conditions.
Psychiatric disorders commonly encountered in clinical practice include various forms of depression (e.g., mild depression, major depression, and other subtypes), bipolar disorder, schizophrenia, anxiety disorders, and borderline personality disorder. Thyroid hormones are essential for neurocognitive development and overall neurological functioning. Consequently, the relationship between thyroid dysfunction and psychiatric conditions has been extensively studied over the past few decades (4, 5). Evidence suggests that mood disorders may disrupt the hypothalamic–pituitary–thyroid axis. For instance, individuals with major depression have been observed to exhibit a diminished thyroid-stimulating hormone (TSH) response to thyrotropin-releasing hormone (TRH) stimulation and a reduced nocturnal TSH surge (6).
Several MR studies have utilized free thyroxine (FT4) and TSH levels as exposure factors. These investigations found no association between genetically predicted FT4 and TSH levels and the risk of major depression, mild depression, schizophrenia, borderline personality disorder, or their subtypes. However, they observed that each standard deviation increase in FT4 levels reduced the overall risk of bipolar disorder by 11% and the risk of bipolar disorder type I by 13%. These results highlight the need for further clinical research on the role of thyroid hormones in bipolar disorder (7, 8). Another study demonstrated that higher TSH levels could decrease the risk of schizophrenia (9). Using hypothyroidism as an exposure factor, a study found significant genetic associations between hypothyroidism and anxiety disorders, schizophrenia, and major depression. Notably, major depression exhibited a causal effect, significantly increasing the risk of hypothyroidism (10). In a mediated Mendelian study, Zhang et al. (11) explored the relationships between major depression and thyroid diseases, identifying a significant risk of hypothyroidism, hyperthyroidism, and European-origin Hashimoto’s thyroiditis associated with major depression. Interestingly, alcohol consumption and antidepressant use were identified as mediators in reducing the risk of hypothyroidism. Furthermore, major depression was strongly linked to Hashimoto’s thyroiditis. These findings highlight the importance of larger RCTs to further explore the connection between thyroid function and psychiatric disorders.
Recent research has identified thyroid hormones as contributors to cell proliferation and differentiation, suggesting a pro-cancer effect in various malignancies (12, 13). Consequently, thyroid dysfunction has been categorized as a potential risk factor for both the development and prevention of cancer. Wang et al. (14) conducted MR studies to establish a causal relationship between hyperthyroidism and ovarian cancer, emphasizing the role of thyroid hormones in the prevention and management of female reproductive disorders. In contrast, other studies have demonstrated that hypothyroidism may have a protective effect against certain cancers, including malignant melanoma of the skin (15), gastric cancer (16), lung cancer (both squamous cell carcinoma and adenocarcinoma) (17), and liver cancer (18). The protective role of hypothyroidism in gastric cancer, in particular, has been linked to factors such as elevated cholesterol levels and other related mechanisms (19).
The thyroid gland produces two key hormones, thyroxine (T4) and triiodothyronine (T3), which are essential for maintaining cardiovascular homeostasis by influencing heart rate, myocardial contractility, and vascular resistance. Thyroid function exerts a broad impact on the cardiometabolic system (20). Zhang et al. demonstrated that lower FT4 levels were significantly associated with increased carotid intima-media thickness, with apolipoproteins A-I and B acting as mediators in this relationship, highlighting the importance of monitoring thyroid function for early cardiovascular risk assessment (21). Additionally, reduced thyroid function has been linked to elevated cholesterol levels and an increased risk of cardiovascular diseases (22). Giontella et al. found that thyroid peroxidase antibodies (TPOAb) and hyperthyroidism were associated with a decreased risk of atrial fibrillation. MR analyses further revealed that TSH levels were inversely associated with systolic blood pressure and the risk of atrial fibrillation. Numerous studies have confirmed a causal relationship between hyperthyroidism and atrial fibrillation, with height identified as a significant mediator in these associations (23–25). The pituitary–thyroid–cardiac axis plays a key role in the development of cardiometabolic diseases. Wang et al. demonstrated causal associations between FT4 levels and conditions such as hypertension, hyperlipidemia, type 2 diabetes mellitus, ischemic heart disease, and non-rheumatic heart disease. Conversely, TSH levels were associated with heart failure and stroke (26). Using mediated MR analysis, Marouli et al. found that TSH levels were linked to a reduced risk of stroke, with atrial fibrillation serving as the primary mediator of this effect. Concurrently, Hashimoto’s thyroiditis was causally associated with an increased risk of coronary heart disease, with body mass index playing a significant role in this relationship (27). These findings emphasize the connection between thyroid function and cardiovascular diseases, suggesting that clinical focus on the cardiovascular health of patients with abnormal thyroid function is crucial for effective prevention of cardiovascular conditions.
The thyroid–gut axis has been proposed as a potential link between thyroid diseases and the gut microbiota (28). A magnetic resonance imaging study revealed that individuals with hyperthyroidism had significantly higher levels of Enterococcus and significantly lower levels of Bifidobacterium and Lactobacillus in their intestines compared to healthy individuals (29). Xie et al. (30) conducted MR analyses on 211 microbial taxa and four thyroid characteristics, identifying 34 outcome-related gut microbiota. After adjustment, the class Deltaproteobacteria (id.3087) and phylum Actinobacteria (id.400) were found to be protective against hyperthyroidism and hypothyroidism, respectively. Liu et al. identified genera Intestinimonas, Eubacterium brachy group, Ruminiclostridium 5, and Ruminococcaceae UCG004 as risk factors for decreased thyroid function, while phyla Actinobacteria and Verrucomicrobia, along with genera Bifidobacterium and Lachnospiraceae UCG008, were shown to have protective effects (31). In a study examining the causal relationship between gut microbiota, metabolites, and hypothyroidism, Liu et al. identified indolactic acid as the only metabolite associated with autoimmune hypothyroidism (32). Additionally, Defluviitaleaceae emerged as a promising probiotic for preventing influenza, subacute thyroiditis, and hypothyroidism. Zhang et al. demonstrated that this bacterium could causally inhibit the clinical pathway of influenza–subacute thyroiditis–hypothyroidism through its role in the gut microbiota (33). Liu et al. (34) applied MR techniques to investigate the connection between the gut microbiota and Graves’ disease, finding that Veillonella, Bacteroides, and the family Bacteroidaceae served as protective factors against the onset of Graves’ disease. Although these studies explore the associations between gut microbiota and thyroid function, further research is needed to clarify the precise effects of gut bacteria on thyroid health.
A recent large-scale epidemiological survey conducted in China reported a strong association between thyroid disease and metabolic syndrome (35). According to an MR analysis investigating the relationship between metabolic syndrome and thyroid autoimmunity, the syndrome and its triglyceride component may act as potential protective factors against thyroid autoimmunity (36). Another MR study on thyroid function and metabolic syndrome found that higher FT4 levels were associated with a reduced likelihood of developing metabolic syndrome (37).
Diabetes mellitus, a group of metabolic disorders characterized by hyperglycemia, has also been linked to thyroid dysfunction. MR studies exploring the association between thyroid function and microvascular complications in diabetes indicate that elevated TSH levels within the reference range are associated with an increased risk of diabetic nephropathy and a reduced glomerular filtration rate. Similarly, lower FT4 levels and higher FT3/FT4 ratios within the reference range were linked to increased urinary albumin–creatinine ratios in diabetic patients (38). Zhao et al. used MR analysis to explore the causal link between thyroid function and type 1 diabetes mellitus, revealing a significant association between type 1 diabetes and hypothyroidism. Additionally, variations in thyroid function were shown to influence type 2 diabetes mellitus (39, 40).
Fatty liver disease, a common metabolic disorder, has also been associated with thyroid dysfunction. MR studies have established a causal relationship between thyroid function and fatty liver, showing a significantly higher incidence of fatty liver in individuals with hypothyroidism, while hyperthyroidism may serve as a risk factor (41). Circulating TSH levels have been linked to an increased risk of fatty liver disease, as demonstrated by Fan et al. (42).
The relationship between thyroid function and lipid metabolism has been investigated using MR analysis. Wang et al. found significant correlations between elevated TSH levels and serum total cholesterol and low-density lipoprotein levels. The FT3/FT4 ratio was also significantly associated with total cholesterol and low-density lipoprotein levels (43). Additionally, Zhang et al. observed that higher triglyceride–glycemic index levels were linked to hypothyroidism and subclinical hypothyroidism (44).
Primary cholangitis, an autoimmune disease, is frequently accompanied by extrahepatic autoimmune conditions, with 73% of patients affected. MR analyses have identified bidirectional causality between primary cholangitis and seven extrahepatic autoimmune diseases, demonstrating that primary cholangitis increases the risk of autoimmune thyroid disorders (45). Another MR study linked primary cholangitis to thyroid dysfunction, particularly mild hypothyroidism (46). Additionally, a reverse MR analysis suggested that both hypothyroidism and hyperthyroidism may act as risk factors for primary cholangitis (47).
Systemic sclerosis, also known as scleroderma, is a connective tissue disease with complex and poorly understood autoimmune and genetic mechanisms. Han et al. applied MR analysis to show that systemic sclerosis was negatively influenced by hypothyroidism and autoimmune thyroiditis (48).
Systemic lupus erythematosus (SLE), an autoimmune connective tissue disease affecting multiple organs and systems, has also been linked to thyroid dysfunction. MR analysis conducted by Qin et al. identified a causal association between SLE and hypothyroidism, but not with hyperthyroidism. A reverse MR analysis further demonstrated a causal relationship between SLE and both hypothyroidism and hyperthyroidism. Another MR study also reported a higher incidence of hyperthyroidism and hypothyroidism in patients with SLE (49, 50).
Inflammatory bowel disease (IBD), an autoimmune condition characterized by immune system disturbances, includes ulcerative colitis and Crohn’s disease. Wu et al. used MR analysis to reveal that hypothyroidism significantly lowered the risk of IBD. TSH levels were associated with IBD, Crohn’s disease, and ulcerative colitis. Multivariate MR results further suggested that IP-10 may mediate the causal relationship between hypothyroidism and IBD (51).
Rheumatoid arthritis is an autoimmune disease characterized by erosive arthritis. Gu et al. used MR analysis to investigate the relationship between thyroid function and rheumatoid arthritis, revealing a familial susceptibility to hypothyroidism and an increased risk of rheumatoid arthritis. Reverse MR evidence further indicates that genetic susceptibility to rheumatoid arthritis is associated with an increased risk of both hypothyroidism and hyperthyroidism (52). Additional MR studies suggest a bidirectional causal relationship between rheumatoid arthritis and hyperthyroidism, hypothyroidism, Hashimoto’s thyroiditis, and autoimmune thyroiditis (53–55).
Myasthenia gravis, an autoimmune disorder resulting from impaired neuromuscular junction transmission, has also been linked to thyroid dysfunction. Wang et al. identified a causal relationship between Hashimoto’s thyroiditis, hypothyroidism, and myasthenia gravis through MR analysis, though FT4, TPOAb, and TSH levels were not associated with myasthenia gravis. Reverse MR analysis revealed a causal link between myasthenia gravis and Graves’ disease, while another study demonstrated causal relationships between myasthenia gravis and both hypothyroidism and hyperthyroidism (56, 57).
Autoimmune diseases arise from pathological autoimmune reactions that damage the body’s own tissues and cellular components, leading to tissue damage and organ dysfunction. The studies outlined above suggest that autoimmune thyroid diseases, as T-cell-mediated organ-specific autoimmune disorders, share a causal relationship with other autoimmune conditions. These findings highlight the importance of recognizing the connections between various autoimmune diseases to improve prevention, diagnosis, and management strategies.
In a two-sample MR study, Ellervik et al. explored the causal relationship between coagulation, fibrinolysis, and genetically predicted hyperthyroidism, hypothyroidism, TSH, and FT4. The study found that elevated TSH was associated with reduced vascular hemophilic factor and fibrinogen levels, while genetically predicted hyperthyroidism was linked to increased vascular hemophilic factor. Furthermore, hyperthyroidism and hypothyroidism were respectively associated with elevated and reduced tissue fibrinolysis plasminogen activator levels (58). An MR study examining the relationship between thyroid disorders and deep vein thrombosis indicated that hyperthyroidism slightly increased the risk of deep vein thrombosis, while no association was identified for other thyroid disorders (59). Another MR study supported a causal relationship between hyperthyroidism and an increased risk of venous thromboembolism (60). Regarding thyroid function and anemia, MR analysis demonstrated that both hypothyroidism and hyperthyroidism were linked to an increased risk of anemia. Genetic variants in the DIO3OS gene, associated with intracellular thyroid hormone regulation, were found to increase the risk of anemia (61). However, an MR study by Huang et al. found a significant causal relationship only between hypothyroidism and iron deficiency anemia, without evidence of a causal link between thyroid dysfunction and serum iron status markers (62). Additionally, autoimmune thyroid disease has been associated with pernicious anemia. However, this relationship is causally linked to impaired erythropoiesis rather than autoimmune hemolysis (63).
An MR study investigating the relationship between thyroid function and periodontitis found a causal link between genetic susceptibility to periodontitis and an increased risk of hypothyroidism. However, no evidence supported a relationship between periodontitis and the risk of hyperthyroidism or autoimmune thyroid disease (64). Chen et al. used an MR approach to evaluate the impact of thyroid function on sudden sensorineural deafness. The analysis showed that TSH levels were not a risk factor for sudden sensorineural deafness, but higher genetically predicted FT4 levels significantly reduced the risk (65). Chen et al.’s MR analysis indicated that hypothyroidism was causally linked to a higher risk of temporomandibular joint (TMJ) disorders, whereas no significant associations were found between FT4, TSH, or genetically predicted hyperthyroidism and TMJ disorders (66). MR analysis by Liu et al. demonstrated a positive correlation between genetically predicted hypothyroidism and an increased risk of cataract development. However, TSH levels, FT4, and hyperthyroidism were not significantly associated with cataracts (67). Li et al. examined the relationship between thyroid function and age-related macular degeneration, finding that genetic variations associated with higher FT4 levels within the normal range were linked to a higher risk of the disease (68).
Hong et al. identified a causal relationship between hypothyroidism and the development of sepsis, including asthma-related pneumonia and other types of sepsis, but no such association was found for hyperthyroidism (69). Zhang et al. employed MR methods to investigate the relationship between thyroid dysfunction and neocoronary pneumonia. Their findings indicated that thyroid dysfunction had no effect on susceptibility or severity of neocoronary pneumonia. A reverse MR study revealed that susceptibility to neocoronary pneumonia was a risk factor for hypothyroidism, but no evidence supported an association between any phenotype of neocoronary pneumonia and hyperthyroidism (70).
Qi et al., in an MR investigation, found a positive correlation between thyroid dysfunction and the risk of osteoporosis, with both hyperthyroidism and hypothyroidism contributing to an elevated risk of the condition (71). Additionally, a single-sample MR analysis investigating the causal relationship between serum TSH and fracture risk revealed that genetically increased serum TSH concentrations were associated with a reduced risk of fractures in men (72). Xiong et al. demonstrated a causal link between hypothyroidism and hallux valgus, with hypothyroidism increasing the incidence of this condition (73).
In an MR investigation, Zhao et al. identified a link between hyperthyroidism and an increased risk of polycystic ovary syndrome (PCOS) (74). Another study using magnetic resonance imaging on thyroid function and PCOS found a significant correlation between TSH levels and the condition, suggesting that maintaining normal TSH levels may help prevent PCOS. Additionally, hyperthyroidism was also associated with the development of PCOS (75). Huang et al. demonstrated that hypothyroidism and TSH levels affect the genetically predicted risk of prostate enlargement and prostatitis (76). A summary of MR applications in thyroid dysfunction is presented in Tables 1 and 2.
Cancer remains a significant threat to global health, with the mechanisms underlying most malignancies still poorly understood. This limited understanding contributes to delays in cancer diagnosis and treatment, exacerbating the global increase in cancer-related morbidity and mortality. Thyroid cancer, one of the most common endocrine tumors, has seen a global rise in incidence over the past three decades due to the expanded use of diagnostic imaging techniques and ultrasound-guided fine-needle aspiration (77, 78). Despite the increasing incidence, the mortality rate of thyroid cancer has remained relatively stable. Risk factors for thyroid cancer include environmental pollution, unhealthy lifestyle choices, and metabolic syndrome; however, these factors do not fully explain the disease’s pathogenesis. Thus, identifying additional modifiable risk factors is crucial for improving prevention and management strategies.
Thyroid and breast cancers are the two most common types of cancer among women worldwide, both influenced by hormonal changes and driven by similar endocrine signals. Emerging evidence suggests a potential bidirectional pathogenic relationship between these two cancers (79). Several studies have explored the relationship between thyroid cancer and various subtypes of breast cancer. Some have identified a causal link between ER-positive breast cancer and an increased risk of thyroid cancer (80, 81). Additional MR studies have examined the relationship bidirectionally, revealing potential connections (82). One study suggested an association between thyroid cancer and ER-negative breast cancer as well (83). Furthermore, an MR study evaluating the causal relationship between prostate cancer and 12 other types of cancer found that thyroid cancer might increase the risk of prostate cancer (84).
An MR study analyzing the causal relationship between gut microbiota and thyroid cancer identified nine microbial taxa associated with thyroid cancer risk. Among these, Christensenellaceae, family Victivallaceae, genus Methanobrevibacter, genus Ruminococcus 2, genus Subdolinglanlum, and phylum Verrucomicrobia were linked to an increased risk of thyroid cancer, while class Betaproteobacteria, family XI, and genus Sutterella were associated with a reduced risk (85). Zhu et al. identified seven microbial taxa significantly associated with thyroid cancer through MR analysis: genera Butyrivibrio, Fusicatenibacter, Oscillospira, Ruminococcus 2, and Terrisporobacter were identified as risk factors, whereas genera Olsenella and Ruminococcus UCG004 were associated with a reduced risk. Reverse MR analysis indicated that thyroid cancer may cause an increase in genus Holdemanella and a decrease in Bacillales (86). Quan et al. conducted an MR study suggesting that genus Ruminiclostridium 9, class Mollicutes, genus Ruminococcaceae UCG004, genus Paraprevotella, and phylum Tenericutes were associated with an increased risk of differentiated thyroid cancer, while phylum Actinobacteria was linked to a reduced risk (87). Similarly, Hu et al. identified four microbial communities, class Mollicutes, phylum Tenericutes, genus Eggerthella, and order Rhodospirillales, as being associated with the risk of differentiated thyroid cancer (88).
Numerous MR studies have demonstrated a positive causal relationship between psychiatric disorders, particularly schizophrenia and depression, and thyroid cancer (89–91). Yuan et al. investigated the genetic association between 13 types of cancer and schizophrenia. Their findings revealed that individuals with schizophrenia have a higher likelihood of developing thyroid cancer (92).
Chronic inflammation is known to accelerate cancer development, and rosacea, a persistent inflammatory skin condition, has been associated with an increased risk of various malignancies. Li et al. conducted a comprehensive study on the relationship between rosacea and cancer in American women, concluding that individuals with a history of rosacea are more likely to develop thyroid cancer (93). However, a bidirectional, two-sample MR study by Luo et al. found no causal relationship between rosacea and thyroid cancer (94).
The MR investigation by Xu et al. found no evidence supporting the hypothesis that severe COVID-19 cases increase the risk of thyroid cancer or that susceptibility to COVID-19 elevates thyroid cancer risk (95).
Gao et al. used MR analysis to explore the relationship between thyroid cancer and various cardiovascular diseases. Their results indicated a potential causal relationship between thyroid cancer and heart failure. However, no association was identified between thyroid cancer and other cardiovascular conditions, including atrial fibrillation, coronary artery disease, myocardial infarction, and ischemic stroke (96). A summary of the application of MR in thyroid cancer is presented in Table 3.
Etiological research plays a crucial role in identifying risk factors essential for treating, preventing, and improving patient outcomes. MR analysis offers a valuable tool by bypassing the labor-intensive data collection process typical of observational studies, instead relying on genetic information from study subjects. By effectively establishing causal relationships between exposure factors and outcomes, while avoiding issues such as reverse causality and confounding variables, MR analysis has gained widespread application in thyroid research and broader medical fields. Given the diverse manifestations of thyroid disorders, MR analysis is frequently employed to identify less obvious risk factors associated with these conditions.
Despite its significant contributions, MR analysis has limitations that warrant further refinement. One key issue is linkage disequilibrium (LD), which arises when alleles segregate in a manner deviating from Mendel’s second law. This interference between related genetic variants can introduce bias into results, as the strength of association between instrumental variables and outcomes is critical. To address this, MR can be applied in populations with different LD structures. Another challenge is gene pleiotropy, where a single gene influences multiple phenotypic traits. Type I pleiotropy, in which a single locus directly affects multiple phenotypes, can complicate study outcomes due to high correlations between traits (97, 98). This issue can be identified and addressed through sensitivity analyses. Finally, the genetic data used in MR analysis often come from biobanks, such as the UK Biobank. This reliance introduces potential biases due to allele frequency and the prevalence of associated disorders within the study population. Variability in instrumental variables, exposures, and outcomes across diverse groups can result in substantial errors. These issues can be minimized by restricting the study population’s background or, when unavoidable, using adaptive switching methods to reduce errors.
Although significant progress has been made in understanding the risk factors for thyroid disease, effective therapies and prevention strategies have yet to be fully implemented. Current thyroid research still faces gaps in understanding the pathophysiology of complex disorders and the translation of findings into clinical practice, such as identifying therapeutic targets and advancing gene therapy. To address these challenges, an innovative approach for applying MR in thyroid research could involve integrating GWAS with multi-omics data, including proteomics and metabolomics, to identify drug-targeting proteins. Furthermore, genes could serve as instrumental variables to pinpoint pathogenic genes and potential targets for intervention.
This review has certain limitations. First, it focused on studies published within a specific time frame and database, potentially excluding relevant articles. Second, it was not a systematic evaluation, and the eligibility criteria were not explicitly defined. Despite these limitations, this review categorizes and discusses findings from MR studies related to thyroid disorders, offering valuable insights that could inform prevention and treatment strategies in clinical practice.
ZL: Data curation, Writing – original draft. RW: Funding acquisition, Supervision, Writing – review & editing. LL: Conceptualization, Validation, Writing – review & editing. ZJ: Formal analysis, Visualization, Writing – original draft. PZ: Conceptualization, Validation, Writing – review & editing. QH: Funding acquisition, Supervision, Writing – review & editing.
The author(s) declare financial support was received for the research, authorship, and/or publication of this article. This work was supported by the National Key R&D Program–Animal Experiment and Clinical Trial of Minimally Invasive Thyroid Tumor Surgery Robot (2019YFC0119200), the Shandong Provincial Natural Science Foundation General Project (ZR2021MH328), and the Dongying Natural Science Foundation Project (2023ZR030).
The authors declare that the research was conducted in the absence of any commercial or financial relationships that could be construed as a potential conflict of interest.
All claims expressed in this article are solely those of the authors and do not necessarily represent those of their affiliated organizations, or those of the publisher, the editors and the reviewers. Any product that may be evaluated in this article, or claim that may be made by its manufacturer, is not guaranteed or endorsed by the publisher.
1. Sekula P, Del GMF, Pattaro C, Kottgen A. Mendelian randomization as an approach to assess causality using observational data. J Am Soc Nephrol. (2016) 27:3253–65. doi: 10.1681/ASN.2016010098
2. Lawlor DA, Harbord RM, Sterne JA, Timpson N, Davey SG. Mendelian randomization: using genes as instruments for making causal inferences in epidemiology. Stat Med. (2008) 27:1133–63. doi: 10.1002/sim.3034
3. Davey SG, Ebrahim S. What can mendelian randomisation tell us about modifiable behavioural and environmental exposures? Bmj. (2005) 330:1076–9. doi: 10.1136/bmj.330.7499.1076
4. Esposito S, Prange AJ, Golden RN. The thyroid axis and mood disorders: overview and future prospects. Psychopharmacol Bull. (1997) 33:205–17.
5. Dayan CM, Panicker V. Hypothyroidism and depression. Eur Thyroid J. (2013) 2:168–79. doi: 10.1159/000353777
6. Jackson IM. The thyroid axis and depression. Thyroid. (1998) 8:951–6. doi: 10.1089/thy.1998.8.951
7. Babajide O, Kjaergaard AD, Deng W, Kus A, Sterenborg R, Asvold BO, et al. The role of thyroid function in borderline personality disorder and schizophrenia: a mendelian randomisation study. Borderline Pers Disord Emot Dysregul. (2024) 11:2. doi: 10.1186/s40479-024-00246-3
8. Kus A, Kjaergaard AD, Marouli E, Del GMF, Sterenborg R, Chaker L, et al. Thyroid function and mood disorders: a mendelian randomization study. Thyroid. (2021) 31:1171–81. doi: 10.1089/thy.2020.0884
9. Freuer D, Meisinger C. Causal link between thyroid function and schizophrenia: a two-sample mendelian randomization study. Eur J Epidemiol. (2023) 38:1081–8. doi: 10.1007/s10654-023-01034-z
10. Zhou J, Zhu L. Shared genetic links between hypothyroidism and psychiatric disorders: evidence from a comprehensive genetic analysis. Front Endocrinol (Lausanne). (2024) 15:1370019. doi: 10.3389/fendo.2024.1370019
11. Zhang X, Lu Q, Luo Y, Wang L, Tian Y, Luo X. The causal relationship between major depression disorder and thyroid diseases: a mendelian randomization study and mediation analysis. J Affect Disord. (2024) 359:287–99. doi: 10.1016/j.jad.2024.05.097
12. Balasubramaniam S, Ron E, Gridley G, Schneider AB, Brenner AV. Association between benign thyroid and endocrine disorders and subsequent risk of thyroid cancer among 4.5 million u.s. Male veterans. J Clin Endocrinol Metab. (2012) 97:2661–9. doi: 10.1210/jc.2011-2996
13. Khan SR, Chaker L, Ruiter R, Aerts JG, Hofman A, Dehghan A, et al. Thyroid function and cancer risk: the rotterdam study. J Clin Endocrinol Metab. (2016) 101:5030–6. doi: 10.1210/jc.2016-2104
14. Wang T, Wang X, Wu J, Li X. Causal relationship between thyroid dysfunction and ovarian cancer: a two-sample mendelian randomization study. BMC Cancer. (2024) 24:629. doi: 10.1186/s12885-024-12385-5
15. Dong H, Pan L, Shen Y, Xu Q, Hu J, Hu Z, et al. Thyroid dysfunction and risk of cutaneous Malignant melanoma: a bidirectional two-sample mendelian randomization study. Front Endocrinol (Lausanne). (2023) 14:1239883. doi: 10.3389/fendo.2023.1239883
16. Zhang Q, Mu Y, Jiang X, Zhao Y, Wang Q, Shen Z. Causal relationship between thyroid dysfunction and gastric cancer: a two-sample mendelian randomization study. Front Endocrinol (Lausanne). (2024) 15:1335149. doi: 10.3389/fendo.2024.1335149
17. Wang X, Liu X, Li Y, Tang M, Meng X, Chai Y, et al. The causal relationship between thyroid function, autoimune thyroid dysfunction and lung cancer: a mendelian randomization study. BMC Pulm Med. (2023) 23:338. doi: 10.1186/s12890-023-02588-0
18. Lu L, Wan B, Li L, Sun M. Hypothyroidism has a protective causal association with hepatocellular carcinoma: a two-sample mendelian randomization study. Front Endocrinol (Lausanne). (2022) 13:987401. doi: 10.3389/fendo.2022.987401
19. Zhang T, Qiao J, Wang Y, Zhou Y, Jabbar ASHH, Hendi M, et al. Causal link between hypothyroidism and gastric cancer risk: insights gained through multivariable mendelian randomization and mediation analysis. Front Endocrinol (Lausanne). (2024) 15:1388608. doi: 10.3389/fendo.2024.1388608
20. Jabbar A, Pingitore A, Pearce SH, Zaman A, Iervasi G, Razvi S. Thyroid hormones and cardiovascular disease. Nat Rev Cardiol. (2017) 14:39–55. doi: 10.1038/nrcardio.2016.174
21. Zhang MZ, Zhao C, Xing XM, Lv J. Deciphering thyroid function and cimt: a mendelian randomization study of the u-shaped influence mediated by apolipoproteins. Front Endocrinol (Lausanne). (2024) 15:1345267. doi: 10.3389/fendo.2024.1345267
22. van Vliet NA, Bos MM, Thesing CS, Chaker L, Pietzner M, Houtman E, et al. Higher thyrotropin leads to unfavorable lipid profile and somewhat higher cardiovascular disease risk: evidence from multi-cohort mendelian randomization and metabolomic profiling. BMC Med. (2021) 19:266. doi: 10.1186/s12916-021-02130-1
23. Larsson SC, Allara E, Mason AM, Michaelsson K, Burgess S. Thyroid function and dysfunction in relation to 16 cardiovascular diseases. Circ Genom Precis Med. (2019) 12:e2468. doi: 10.1161/CIRCGEN.118.002468
24. Ruan W, Zhou X, Li J, Wang T, Liu H, Zhang G, et al. Thyroid function effect on cardiac structure, cardiac function, and disease risk: evidence of causal associations in european ancestry. Heart Rhythm. (2024) 2272–81. doi: 10.1016/j.hrthm.2024.05.021
25. Shi M, Manouchehri AM, Shaffer CM, Vaitinadin NS, Hellwege JN, Salem JE, et al. Genetic thyrotropin regulation of atrial fibrillation risk is mediated through an effect on height. J Clin Endocrinol Metab. (2021) 106:2124–32. doi: 10.1210/clinem/dgab272
26. Wang JJ, Zhuang ZH, Yu CQ, Wang WY, Wang WX, Zhang K, et al. Assessment of causal direction between thyroid function and cardiometabolic health: a mendelian randomization study. J Geriatr Cardiol. (2022) 19:61–70. doi: 10.11909/j.issn.1671-5411.2022.01.004
27. Marouli E, Kus A, Del GMF, Chaker L, Peeters R, Teumer A, et al. Thyroid function affects the risk of stroke via atrial fibrillation: a mendelian randomization study. J Clin Endocrinol Metab. (2020) 105:2634–41. doi: 10.1210/clinem/dgaa239
28. Su X, Zhao Y, Li Y, Ma S, Wang Z. Gut dysbiosis is associated with primary hypothyroidism with interaction on gut-thyroid axis. Clin Sci (Lond). (2020) 134:1521–35. doi: 10.1042/CS20200475
29. Zhou L, Li X, Ahmed A, Wu D, Liu L, Qiu J, et al. Gut microbe analysis between hyperthyroid and healthy individuals. Curr Microbiol. (2014) 69:675–80. doi: 10.1007/s00284-014-0640-6
30. Xie L, Zhao H, Chen W. Relationship between gut microbiota and thyroid function: a two-sample mendelian randomization study. Front Endocrinol (Lausanne). (2023) 14:1240752. doi: 10.3389/fendo.2023.1240752
31. Liu X, Liu J, Zhang T, Wang Q, Zhang H. Complex relationship between gut microbiota and thyroid dysfunction: a bidirectional two-sample mendelian randomization study. Front Endocrinol (Lausanne). (2023) 14:1267383. doi: 10.3389/fendo.2023.1267383
32. Liu X, Yuan J, Liu S, Tang M, Meng X, Wang X, et al. Investigating causal associations among gut microbiota, metabolites and autoimmune hypothyroidism: a univariable and multivariable mendelian randomization study. Front Immunol. (2023) 14:1213159. doi: 10.3389/fimmu.2023.1213159
33. Zhang X, Li PH, Wang D, Li H, Kong X, Zhang G, et al. Causal effect of gut microbiota of defluviitaleaceae on the clinical pathway of “influenza-subacute thyroiditis-hypothyroidism. Front Microbiol. (2024) 15:1354989. doi: 10.3389/fmicb.2024.1354989
34. Liu S, Li F, Cai Y, Ren L, Sun L, Gang X, et al. Bacteroidaceae, bacteroides, and veillonella: emerging protectors against graves’ disease. Front Cell Infect Microbiol. (2024) 14:1288222. doi: 10.3389/fcimb.2024.1288222
35. He J, Lai Y, Yang J, Yao Y, Li Y, Teng W, et al. The relationship between thyroid function and metabolic syndrome and its components: a cross-sectional study in a chinese population. Front Endocrinol (Lausanne). (2021) 12:661160. doi: 10.3389/fendo.2021.661160
36. Chen K, Sun W, He L, Dong W, Zhang D, Zhang T, et al. Exploring the bidirectional relationship between metabolic syndrome and thyroid autoimmunity: a mendelian randomization study. Front Endocrinol (Lausanne). (2024) 15:1325417. doi: 10.3389/fendo.2024.1325417
37. Pleic N, Gunjaca I, Babic LM, Zemunik T. Thyroid function and metabolic syndrome: a two-sample bidirectional mendelian randomization study. J Clin Endocrinol Metab. (2023) 108:3190–200. doi: 10.1210/clinem/dgad371
38. Li H, Li M, Dong S, Zhang S, Dong A, Zhang M. Assessment of the association between genetic factors regulating thyroid function and microvascular complications in diabetes: a two-sample mendelian randomization study in the european population. Front Endocrinol (Lausanne). (2023) 14:1126339. doi: 10.3389/fendo.2023.1126339
39. Zhao Y, Si S, Zhang K, Yuan J, Li J, Xue F. Causal relationship between type 1 diabetes and hypothyroidism: a mendelian randomization study. Clin Endocrinol (Oxf). (2022) 97:740–6. doi: 10.1111/cen.14801
40. Kus A, Marouli E, Del GMF, Chaker L, Bednarczuk T, Peeters RP, et al. Variation in normal range thyroid function affects serum cholesterol levels, blood pressure, and type 2 diabetes risk: a mendelian randomization study. Thyroid. (2021) 31:721–31. doi: 10.1089/thy.2020.0393
41. Zhang HF, Zhang QX, Feng YH, Li SJ, Xie BY. Association of thyroid disease and risk of fatty liver disease: an exposure-wide mendelian randomization study. Eur Rev Med Pharmacol Sci. (2023) 27:11402–11. doi: 10.26355/eurrev_202312_34579
42. Fan H, Liu Z, Zhang X, Wu S, Shi T, Zhang P, et al. Thyroid stimulating hormone levels are associated with genetically predicted nonalcoholic fatty liver disease. J Clin Endocrinol Metab. (2022) 107:2522–9. doi: 10.1210/clinem/dgac393
43. Wang JJ, Zhuang ZH, Shao CL, Yu CQ, Wang WY, Zhang K, et al. Assessment of causal association between thyroid function and lipid metabolism: a mendelian randomization study. Chin Med J (Engl). (2021) 134:1064–9. doi: 10.1097/CM9.0000000000001505
44. Zhang C, Wang H, Li Y, Wang X, Han Y, Gao X, et al. Association between the triglyceride-glucose index and thyroid disorders: a cross-sectional survey and mendelian randomization analysis. Endocrine. (2024) 173–85. doi: 10.1007/s12020-024-03858-5
45. Ma G, Yang J, Wang X, Xia E, Yu J, Zhang M, et al. Dissecting causal relationships between primary biliary cholangitis and extrahepatic autoimmune diseases based on mendelian randomization. Sci Rep. (2024) 14:11528. doi: 10.1038/s41598-024-62509-x
46. Huang P, Hou Y, Zou Y, Ye X, Yu R, Yang S. The causal effects of primary biliary cholangitis on thyroid dysfunction: a two-sample mendelian randomization study. Front Genet. (2021) 12:791778. doi: 10.3389/fgene.2021.791778
47. Zhang W, Lang R. Genetic link between primary sclerosing cholangitis and thyroid dysfunction: a bidirectional two-sample mendelian randomization study. Front Immunol. (2023) 14:1276459. doi: 10.3389/fimmu.2023.1276459
48. Han Z, Han P, Wang F, Zheng H, Chen X, Meng H, et al. Negative causal exploration of systemic sclerosis: a mendelian randomization analysis. Sci Rep. (2024) 14:5200. doi: 10.1038/s41598-024-55808-w
49. Qin Q, Zhao L, Ren A, Li W, Ma R, Peng Q, et al. Systemic lupus erythematosus is causally associated with hypothyroidism, but not hyperthyroidism: a mendelian randomization study. Front Immunol. (2023) 14:1125415. doi: 10.3389/fimmu.2023.1125415
50. Duan L, Shi Y, Feng Y. Systemic lupus erythematosus and thyroid disease: a mendelian randomization study. Clin Rheumatol. (2023) 42:2029–35. doi: 10.1007/s10067-023-06598-5
51. Wu S, Yi J, Wu B. Casual associations of thyroid function with inflammatory bowel disease and the mediating role of cytokines. Front Endocrinol (Lausanne). (2024) 15:1376139. doi: 10.3389/fendo.2024.1376139
52. Gu P, Pu B, Ma Y, Yue D, Xin Q, Li H, et al. Appraising the causal relationship between thyroid function and rheumatoid arthritis: a two-sample bidirectional mendelian randomization study. Front Immunol. (2023) 14:1238757. doi: 10.3389/fimmu.2023.1238757
53. Tan Y, Yao H, Lin C, Lai Z, Li H, Zhang J, et al. Investigating the bidirectional association of rheumatoid arthritis and thyroid function: a methodologic assessment of mendelian randomization. Arthritis Care Res (Hoboken). (2024) 1162–72. doi: 10.1002/acr.25335
54. Liang J, Jing Z, Cai Y, Lv L, Zhang G, Nan K, et al. Association between hashimoto’s thyroiditis and rheumatoid arthritis: a bidirectional mendelian randomization study. Genet Test Mol Biomarkers. (2024) 28:169–75. doi: 10.1089/gtmb.2023.0594
55. Liu X, Yuan J, Wang X, Tang M, Meng X, Zhang L, et al. Association between rheumatoid arthritis and autoimmune thyroid disease: evidence from complementary genetic methods. Endocrine. (2024) 84:171–8. doi: 10.1007/s12020-023-03571-9
56. Wang S, Wang K, Chen X, Chen D, Lin S. Autoimmune thyroid disease and myasthenia gravis: a study bidirectional mendelian randomization. Front Endocrinol (Lausanne). (2024) 15:1310083. doi: 10.3389/fendo.2024.1310083
57. Li K, Ouyang Y, Yang H. Myasthenia gravis and five autoimmune diseases: a bidirectional mendelian randomization study. Neurol Sci. (2024) 45:1699–706. doi: 10.1007/s10072-023-07163-3
58. Ellervik C, Mora S, Kus A, Asvold B, Marouli E, Deloukas P, et al. Effects of thyroid function on hemostasis, coagulation, and fibrinolysis: a mendelian randomization study. Thyroid. (2021) 31:1305–15. doi: 10.1089/thy.2021.0055
59. Zhang L, Li K, Yang Q, Lin Y, Geng C, Huang W, et al. Associations between deep venous thrombosis and thyroid diseases: a two-sample bidirectional mendelian randomization study. Eur J Med Res. (2024) 29:327. doi: 10.1186/s40001-024-01933-1
60. Han F, Zhang C, Xuan M, Xie Z, Zhang K, Li Y. Effects of hyperthyroidism on venous thromboembolism: a mendelian randomization study. J Immunol Res. (2022) 2022:2339678. doi: 10.1155/2022/2339678
61. van Vliet NA, Kamphuis A, den Elzen W, Blauw GJ, Gussekloo J, Noordam R, et al. Thyroid function and risk of anemia: a multivariable-adjusted and mendelian randomization analysis in the uk biobank. J Clin Endocrinol Metab. (2022) 107:e643–52. doi: 10.1210/clinem/dgab674
62. Huang X, Mao M, Guo T, Wu Y, Xu Q, Dai J, et al. Iron status, thyroid dysfunction, and iron deficiency anemia: a two-sample mendelian randomization study. Ann Nutr Metab. (2024) 80(6):295–306. doi: 10.1159/000539610
63. Kjaergaard AD, Teumer A, Marouli E, Deloukas P, Kus A, Sterenborg R, et al. Thyroid function, pernicious anemia and erythropoiesis: a two-sample mendelian randomization study. Hum Mol Genet. (2022) 31:2548–59. doi: 10.1093/hmg/ddac052
64. Gao Y, Huang D, Liu Y, Qiu Y, Lu S. Periodontitis and thyroid function: a bidirectional mendelian randomization study. J Periodontal Res. (2024) 59:491–9. doi: 10.1111/jre.13240
65. Chen J, Wu C, He J, Wu L, Yang Y, Zhong S, et al. Causal associations of thyroid function and sudden sensorineural hearing loss: a bidirectional and multivariable mendelian randomization study. Front Neurol. (2023) 14:1269545. doi: 10.3389/fneur.2023.1269545
66. Chen X, Xu J, Cheng Z, Wang Q, Zhao Z, Jiang Q. Causal relationship between hypothyroidism and temporomandibular disorders: evidence from complementary genetic methods. BMC Oral Health. (2024) 24:247. doi: 10.1186/s12903-024-03999-z
67. Liu S, Sun Q, Gu Q, Bao Y, Wang W, Qin X, et al. Hypothyroidism is a causal determinant of age-related cataract risk in european population: a mendelian randomization study. Front Endocrinol (Lausanne). (2024) 15:1254793. doi: 10.3389/fendo.2024.1254793
68. Li X, Li H, Cheng J, Wang M, Zhong Y, Shi G, et al. Causal associations of thyroid function and age-related macular degeneration: a two-sample mendelian randomization study. Am J Ophthalmol. (2022) 239:108–14. doi: 10.1016/j.ajo.2022.01.026
69. Hong J, Zhang L, Lai Y, Chen X, Chen Y, Yang J. Causal association between thyroid dysfunction and sepsis: a two-sample mendelian randomization study. Front Endocrinol (Lausanne). (2024) 15:1348248. doi: 10.3389/fendo.2024.1348248
70. Zhang Z, Fang T, Lv Y. Causal associations between thyroid dysfunction and covid-19 susceptibility and severity: a bidirectional mendelian randomization study. Front Endocrinol (Lausanne). (2022) 13:961717. doi: 10.3389/fendo.2022.961717
71. Qi W, Wang D, Hong Y, Yao J, Wang H, Zhu L, et al. Investigating the causal relationship between thyroid dysfunction diseases and osteoporosis: a two-sample mendelian randomization analysis. Sci Rep. (2024) 14:12784. doi: 10.1038/s41598-024-62854-x
72. Soto-Pedre E, Siddiqui MK, Mordi I, Maroteau C, Soto-Hernaez J, Palmer C, et al. Evidence of a causal relationship between serum thyroid-stimulating hormone and osteoporotic bone fractures. Eur Thyroid J. (2021) 10:439–46. doi: 10.1159/000518058
73. Xiong B, Bai Z, Cao X, Nie D, Zhang C, Sun X, et al. Causal relationship between thyroid dysfunction and hallux valgus: a two-sample mendelian randomization study. Front Endocrinol (Lausanne). (2023) 14:1115834. doi: 10.3389/fendo.2023.1115834
74. Zhao Z, Gao Y, Pei X, Wang W, Wang R, Zhang H. Thyroid function and polycystic ovary syndrome: a mendelian randomization study. Front Endocrinol (Lausanne). (2024) 15:1364157. doi: 10.3389/fendo.2024.1364157
75. Zhang Q, Ke W, Ye J, Zhang P, Yang Q, Pan F, et al. Is thyroid function associated with polycystic ovary syndrome? A bidirectional mendelian randomization study. Endocrine. (2024) 85:380–91. doi: 10.1007/s12020-024-03756-w
76. Huang Y, Chen C, Zhou W, Zhang Q, Zhao Y, He D, et al. Genetically predicted alterations in thyroid function are associated with the risk of benign prostatic disease. Front Endocrinol (Lausanne). (2023) 14:1163586. doi: 10.3389/fendo.2023.1163586
77. Haupt S, Caramia F, Klein SL, Rubin JB, Haupt Y. Sex disparities matter in cancer development and therapy. Nat Rev Cancer. (2021) 21:393–407. doi: 10.1038/s41568-021-00348-y
78. Lim H, Devesa SS, Sosa JA, Check D, Kitahara CM. Trends in thyroid cancer incidence and mortality in the United States, 1974-2013. Jama. (2017) 317:1338–48. doi: 10.1001/jama.2017.2719
79. Ikeda Y, Kiyotani K, Yew PY, Kato T, Tamura K, Yap KL, et al. Germline parp4 mutations in patients with primary thyroid and breast cancers. Endocr Relat Cancer. (2016) 23:171–9. doi: 10.1530/ERC-15-0359
80. Tan H, Wang S, Huang F, Tong Z. Association between breast cancer and thyroid cancer risk: a two-sample mendelian randomization study. Front Endocrinol (Lausanne). (2023) 14:1138149. doi: 10.3389/fendo.2023.1138149
81. Wang H, Li S, Shi J, Feng C, Wang Y, Zhang F. Unbalanced bidirectional causal association between thyroid cancer and er-positive breast cancer: should we recommend screening for thyroid cancer in breast cancer patients? BMC Genomics. (2023) 24:762. doi: 10.1186/s12864-023-09854-9
82. Li Z, Xia L, Li X, He H. Assessing the causality between thyroid and breast neoplasms: a bidirectional mendelian randomization study. Front Oncol. (2022) 12:973161. doi: 10.3389/fonc.2022.973161
83. Tokita H, Tanaka N, Ueno T, Fujimoto S, Nakano K. An in vitro chemosensitivity test for human breast cancer based on morphological changes in the nucleus. Gan No Rinsho. (1986) 32:1803–8.
84. Zhan X, Chen T, Xiong S, Li S, Deng X, Xu S, et al. Causal relationship between prostate cancer and 12 types of cancers: multivariable and bidirectional mendelian randomization analyses. Int Urol Nephrol. (2024) 56:547–56. doi: 10.1007/s11255-023-03793-w
85. Sun X, Chen S, Zhao S, Wang J, Cheng H. Causal relationship of genetically predicted gut microbiota with thyroid cancer: a bidirectional two-sample mendelian randomization study. Front Endocrinol (Lausanne). (2024) 15:1284472. doi: 10.3389/fendo.2024.1284472
86. Zhu F, Zhang P, Liu Y, Bao C, Qian D, Ma C, et al. Mendelian randomization suggests a causal relationship between gut dysbiosis and thyroid cancer. Front Cell Infect Microbiol. (2023) 13:1298443. doi: 10.3389/fcimb.2023.1298443
87. Quan Z, Zhang X, Wang S, Meng Y. Causal analysis of the gut microbiota in differentiated thyroid carcinoma: a two-sample mendelian randomization study. Front Genet. (2023) 14:1299930. doi: 10.3389/fgene.2023.1299930
88. Hu S, Tang C, Wang L, Feng F, Li X, Sun M, et al. Causal relationship between gut microbiota and differentiated thyroid cancer: a two-sample mendelian randomization study. Front Oncol. (2024) 14:1375525. doi: 10.3389/fonc.2024.1375525
89. Zhou K, Zhu L, Chen N, Huang G, Feng G, Wu Q, et al. Causal associations between schizophrenia and cancers risk: a mendelian randomization study. Front Oncol. (2023) 13:1258015. doi: 10.3389/fonc.2023.1258015
90. Han X, Lin X, Li G, Wang J, Meng X, Chen T, et al. Association of cancer and schizophrenia, major depression and bipolar disorder: a mendelian randomization study. J Psychosom Res. (2024) 183:111806. doi: 10.1016/j.jpsychores.2024.111806
91. Qiu R, Lin H, Jiang H, Shen J, He J, Fu J. Association of major depression, schizophrenia and bipolar disorder with thyroid cancer: a bidirectional two-sample mendelian randomized study. BMC Psychiatry. (2024) 24:261. doi: 10.1186/s12888-024-05682-7
92. Yuan K, Song W, Liu Z, Lin GN, Yu S. Mendelian randomization and gwas meta analysis revealed the risk-increasing effect of schizophrenia on cancers. Biol (Basel). (2022) 11(9):1345. doi: 10.3390/biology11091345
93. Li WQ, Zhang M, Danby FW, Han J, Qureshi AA. Personal history of rosacea and risk of incident cancer among women in the us. Br J Cancer. (2015) 113:520–3. doi: 10.1038/bjc.2015.217
94. Luo M, Wei B. Novel insights into rosacea’s role in cancer risk: a mendelian randomization approach. Skin Res Technol. (2024) 30:e13737. doi: 10.1111/srt.13737
95. Xu D, Wang X, Zhou D. Exploring the potential association between covid-19 and thyroid cancer: a mendelian randomization study. ACS Omega. (2023) 8:49158–64. doi: 10.1021/acsomega.3c07287
96. Gao Y, Wang Z, Yu J, Chen L. Thyroid cancer and cardiovascular diseases: a mendelian randomization study. Front Cardiovasc Med. (2024) 11:1344515. doi: 10.3389/fcvm.2024.1344515
97. Wagner GP, Zhang J. The pleiotropic structure of the genotype-phenotype map: the evolvability of complex organisms. Nat Rev Genet. (2011) 12:204–13. doi: 10.1038/nrg2949
Keywords: Mendelian randomization, thyroid diseases, risk factors, etiological research, casual relationship
Citation: Li Z, Wang R, Liu L, Jia Z, Zhou P and He Q (2024) Application of Mendelian randomization in thyroid diseases: a review. Front. Endocrinol. 15:1472009. doi: 10.3389/fendo.2024.1472009
Received: 28 July 2024; Accepted: 21 November 2024;
Published: 19 December 2024.
Edited by:
Fabio Monzani, University of Pisa, ItalyReviewed by:
Yin Li, Tianjin University, ChinaCopyright © 2024 Li, Wang, Liu, Jia, Zhou and He. This is an open-access article distributed under the terms of the Creative Commons Attribution License (CC BY). The use, distribution or reproduction in other forums is permitted, provided the original author(s) and the copyright owner(s) are credited and that the original publication in this journal is cited, in accordance with accepted academic practice. No use, distribution or reproduction is permitted which does not comply with these terms.
*Correspondence: Qingqing He, MTM1NTMxNjkzMzJAMTYzLmNvbQ==
†These authors have contributed equally to this work and share first authorship
Disclaimer: All claims expressed in this article are solely those of the authors and do not necessarily represent those of their affiliated organizations, or those of the publisher, the editors and the reviewers. Any product that may be evaluated in this article or claim that may be made by its manufacturer is not guaranteed or endorsed by the publisher.
Research integrity at Frontiers
Learn more about the work of our research integrity team to safeguard the quality of each article we publish.