- 1School of Chemistry and Environment, Jiaying University, Meizhou, China
- 2School of Physics and Electrical Engineering, Jiaying University, Meizhou, China
Introduction: Currently, although there have been a few reports on the endocrine-disrupting effects of neonicotinoids, the effect on Chironomidae during long-term exposure remains unknown.
Methods: Ecdysis and sex ratio, along with ecdysone-relevant gene expressions of representative neonicotinoid dinotefuran on Chironomus kiinensis were investigated at different environmental concentrations by long-term exposure.
Results: A low dose of dinotefuran delayed pupation and emergence via inhibiting ecdysis. Sex ratios of adults shifted toward male-dominated populations with the concentration of dinotefuran increasing. The corresponding transcriptions of ecdysis genes ecr, usp, E74, and hsp70 were significantly downregulated in the midge. For estrogen effects, the vtg gene expression was upregulated, but there was no significant change for the err gene.
Discussion: These results would improve our understanding of the endocrine-disrupting mechanisms of neonicotinoid insecticides to Chironomidae and provide data support for assessing their potential environmental risks.
Highlights
● Low dose of dinotefuran delayed growth and development via inhibiting ecdysis.
● Dinotefuran shifted sex ratios toward male-dominated populations.
● Dinotefuran downregulated gene expressions related to ecdysis hormones.
1 Introduction
Neonicotinoids are one of the most commonly used pesticides worldwide in agriculture, due to their broad spectrum, high efficiency, and low toxicity (1). Thus, neonicotinoids’ widespread use, combined with moderate persistence, leads to their ubiquity in the environment (2). Neonicotinoids are designed to target the nicotinic acetylcholine receptors (nAChRs) in insect selectively and disturb the central nervous system, leading to insect paralysis and death (3). Because of the unique mechanism of action, neonicotinoids cause less harm to non-target organisms and the environment compared with the highly toxic organophosphorus pesticides (4).
However, rising evidence revealed that neonicotinoids exhibited a number of additional toxicities (e.g., mitochondrial dysfunction, endocrine disruption, reproductive toxicity, and immunotoxicity) to non-target organisms, including vertebrates and even human beings (5, 6). The exploration of their additional toxic mechanism has become an emerging focus of public attention. Among them, the endocrine-disrupting effects of neonicotinoids have been confirmed by several studies. Computer molecular docking simulations and in vivo experiments showed that imidacloprid could bind to the thyroid receptor (TR) in mice by competing with T3, a thyroid hormone (7). Flupyradifurone, a new nicotinoid insecticide, induced sex-based dysregulation of the hypothalamic–pituitary–thyroid axis of rats, exhibiting the potential agonistic or antagonistic effect of flupyradifurone sex-dependent metabolites on liver thyroid hormone receptors (8). Imidacloprid also interfered with the pituitary–thyroid axis of red munia (9). Long-term exposure to acetamiprid altered hormone production and the related gene expression of the hypothalamic–pituitary–gonad (HPG) axis in a sex-dependent manner and caused feminization and reproductive dysfunction in zebrafish and their offspring (10). Imidacloprid disrupted larval molting regulation, causing developmental delay in honey bee (11). Although progress has been made in understanding the endocrine-disrupting effects of neonicotinoids on many non-target species, especially vertebrates, this toxicity remains poorly understood in aquatic insects that belong to Chironomidae.
Chironomid larvae play important ecological functions as they have a large biomass in freshwater ecosystems and are natural food for many aquatic organisms (12). In addition, Chironomidae are more sensitive to neonicotinoids than other aquatic invertebrates (13). Chironomids are metamorphosed species with four life stages (egg, larva, pupa, and adult). For the larva, it undergoes three ecdysis, then molts into a pupa, and finally molts into an adult. Therefore, the molting activity is crucial in the whole life process, which is regulated by ecdysone and juvenile hormones. Previous studies have shown that neonicotinoids arrested pupal ecdysis in Lepidoptera (14) and disrupted larval molting regulation, causing developmental delay in honey bee (11). Whether the molting activity of chironomids that are more sensitive to neonicotinoids is also affected by the insecticide, the question remains, which genes regulate their effects? Therefore, it is urgent to uncover the ecdysis effect of neonicotinoids on chironomids and its regulatory mechanism at different environmental concentrations for long-term exposure.
Dinotefuran, as a third-generation neonicotinoid insecticide, has accounted for more than a quarter of the global pesticide used due to its excellent properties, such as higher insecticidal activity, quicker uptake, smaller resistance, and more safety for the environment and humans (15–17). A few studies reported that the detection concentration was 0.012–0.8 μg/L in environmental waters (18–20). Raby et al. (21) reported the chronic toxicity of dinotefuran to Chironomus dilutus, showing that the 14-day lethal concentration of 50% (LC50) and emergence concentration of 50% (EC50) were 15.1 (13.4–16.8) μg/L and 8.15 (7.35–8.95) μg/L, respectively. However, the toxic mechanisms of the endocrine-disrupting effects of dinotefuran are poorly studied to date.
In the present study, the endocrine-disrupting effects of representative neonicotinoid dinotefuran on Chironomus kiinensis were investigated at different environmental concentrations by long-term exposure. Ecdysis and sex ratio, along with relevant gene expressions, were measured. These results would improve our understanding of the endocrine-disrupting mechanisms of neonicotinoid insecticides to Chironomidae and provide data support for assessing their potential environmental risks.
2 Materials and methods
2.1 Materials
Dinotefuran, thiamethoxam-d3 (internal standard), and imidacloprid-d4 (surrogate standard) were purchased from Dr. Ehrenstorfer GmbH (Augsburg, Germany) with purity >98%. C. kiinensis were cultured in Jiaying University according to the standard protocol of USEPA2000 proposed by the U.S. Environmental Protection Agency.
2.2 Toxicological assay
Test water was freshly prepared with 0.1, 1, and 10 μg/L of dinotefuran (DIN_1, DIN_2, and DIN_3) into reconstituted moderately hard water. Negative control and solvent (0.1% DMSO) controls were tested in the meantime. A 0.5-cm layer of quartz sand and 200 mL of the testing solution were introduced into each 500-mL beaker. Twenty newly hatched midge larvae (within 24 h) were randomly added into each beaker with three replicates per treatment group or control group. The organisms were fed ground fish food every 2 days per beaker and the test solution was changed every 5 days. Water quality parameters (i.e., conductivity, pH, temperature, and dissolved oxygen) in test solution were monitored every day and ammonia nitrogen was monitored on days 0, 5, and 10. Three experimental groups were tested in the chronic bioassays. For the first group, mortality, pupation, emergence time, and sex ratio were recorded daily from the first emergence at 11 days and ended until the last chironomid completed emergence (approximately 21 days). For the second group, the survival larvae at 11 days were evaluated for adenosine triphosphate (ATP) levels. The third group at 11 days was evaluated for the levels of gene expression.
2.3 Quantification of dinotefuran
Exposure samples were collected from test containers at 5 and 10 days of exposure in three replicates and analyzed for dinotefuran actual concentrations using HPLC-MS/MS following a previously developed method from Wei et al. (22). In brief, the sample was extracted using solid-phase extraction (SPE) cartridges packed with 200 mg of HLB absorbent. Before loading the sample, the SPE cartridge was conditioned with 3 mL of methanol and 10 mL of water, sequentially. Samples were then passed through the cartridges at a flow rate of 3–5 mL/min and eluted out of the cartridges with 10 mL of methanol. Eluants were evaporated to near dryness under a gentle flow of nitrogen and re-dissolved in 500 μL of acetonitrile. The HPLC-MS/MS analysis was conducted on a Shimadzu DGU-30A HPLC coupled with an AB SCIEX TRIPLE QUAD™ 5500 tandem MS system. The analytes were separated on an Agilent Zorbax Eclipse Plus C18 column (100 × 2.1 mm i.d., 1.8 μm) at 40°C. The mobile phase was a mixture of water containing 0.1% formic acid (A) and acetonitrile (B) and flow rate was 0.3 mL/min. The gradient elution condition was as follows: 0 min, 37% B; 1.2 min, 37% B; 3 min, 70% B; 3.5 min, 70% B; 3.6 min, 37% B; and 5.1 min, 37% B. The injection volume was 2 μL. The MS monitoring was performed using an electrospray ionization (ESI) source in positive mode and multiple reaction monitoring (MRM). The MS/MS conditions were as follows: source temperature, 550°C; curtain gas (CUR), 40 psi; collision gas (CAD), 7 psi; ion source gas 1 (GS1), 55 psi; ion source gas 2 (GS2), 55 psi; ion spray voltage (IS), 5,500 V; entrance potential (EP), 10 V; and collision cell exit potential (CXP), 16 V. Other MS parameters for dinotefuran qualification are listed in Supplementary Table S1. Quantification of dinotefuran was achieved using an internal standard calibration method, and the calibration curve for dinotefuran was linear over a range of 0.1–50 μg L−1.
2.4 Measurements of ATP level
For the second group, ATP was measured using a commercial ATP assay kit (Nanjing Jiancheng, China) according to the manufacturer’s protocols. Creatine kinase catalyzes ATP and creatine to produce creatine phosphate, which was detected by phosphomolybdate colorimetry. After 11 days of exposure, survival larvae in control and three treatment groups (DIN_1, DIN_2, and DIN_3) were homogenized in 1 mL of cool ultrapure water for 3 min. Part of the homogenate was centrifuged at 3,500 rpm at 4°C for 10 min and the supernatant was taken to measure the protein concentration. The other homogenates were then boiled in a boiling water bath for 10 min, mixed, and extracted for 1 min, and the supernatant was centrifuged at 3,500 rpm at 4°C for 10 min. Then, the supernatant was used for measuring the ATP level at 636 nm by the microplate reader.
2.5 Measurements of gene expression levels
For the third group, surviving larvae from DIN_1, DIN_2, and DIN_3 were immediately frozen with liquid nitrogen before use. Total RNA was isolated using an RNeasy Mini Kit (Qiagen, Hilden, Germany) according to the manufacturer’s protocol. Expressions of six genes (Table 1) were quantified using a real-time quantitative polymerase chain reaction (RT-qPCR). Primers were either chosen from previous studies or designed by NCBI/Primer-BLAST. β-actin was chosen as an internal control. The RNA samples were reversely transcribed into cDNA by using a Bestar™ qPCR-RT Kit (DBI-2220, German). RT-qPCR was performed in an ABI 7500 fluorescence quantitative PCR instrument (ThermoFisher, USA). The fold changes of the target genes were calculated using the 2−ΔΔCT method (25).
2.6 Statistical analysis
Differences among the treatments were analyzed with one-way ANOVA by SPSS 17.0 software (SPSS Inc., Chicago, IL, USA). Normality of each data set was assessed using the Kolmogorov–Smirnov one-sample test. Significant differences were evaluated by one-way ANOVA with LSD’s post-hoc test where data met the assumptions of normality and homogeneity of variance. p-value < 0.05 was considered statistically significant.
3 Results
3.1 Dinotefuran delayed the ecdysis activity of chironomids
Dinotefuran concentrations in exposure solutions varied little during the experiment duration (Supplementary Table S2). For the first group, durations of different life stages of midges were different among the dinotefuran exposure and control groups (Figure 1A and Supplementary Table S3). For the larva stage from first instar to fourth instar, life duration in the negative control, solvent control, and DIN_1, DIN_2, and DIN_3 groups was 16, 15, 16, 19, and 17 days, respectively, showing an extended trend compared to the control group, which indicated that the molt of larvae was delayed by dinotefuran in each instar. For the pupa stage, the time of the first pupa was 10, 11, 10, 13, and 13 days for the negative control, solvent control, and DIN_1, DIN_2, and DIN_3 groups, respectively, indicating that pupation of DIN_2 and DIN_3 groups was delayed. For the adult stage, the emergence of the first pupa was also delayed at DIN_2 and DIN_3 groups (14 and 14 days) relative to the negative control (11 days) and solvent control (12 days) except for DIN_1 (11 days). Meanwhile, the time of the last emergence was delayed at DIN_2 and DIN_3 groups (21 and 20 days) relative to the controls (18 days). For Figure 1B and Supplementary Table S4, the emergence rate decreased with the increase of exposure concentration of dinotefuran. These results suggested that molting of larva and pupa was inhibited by dinotefuran.
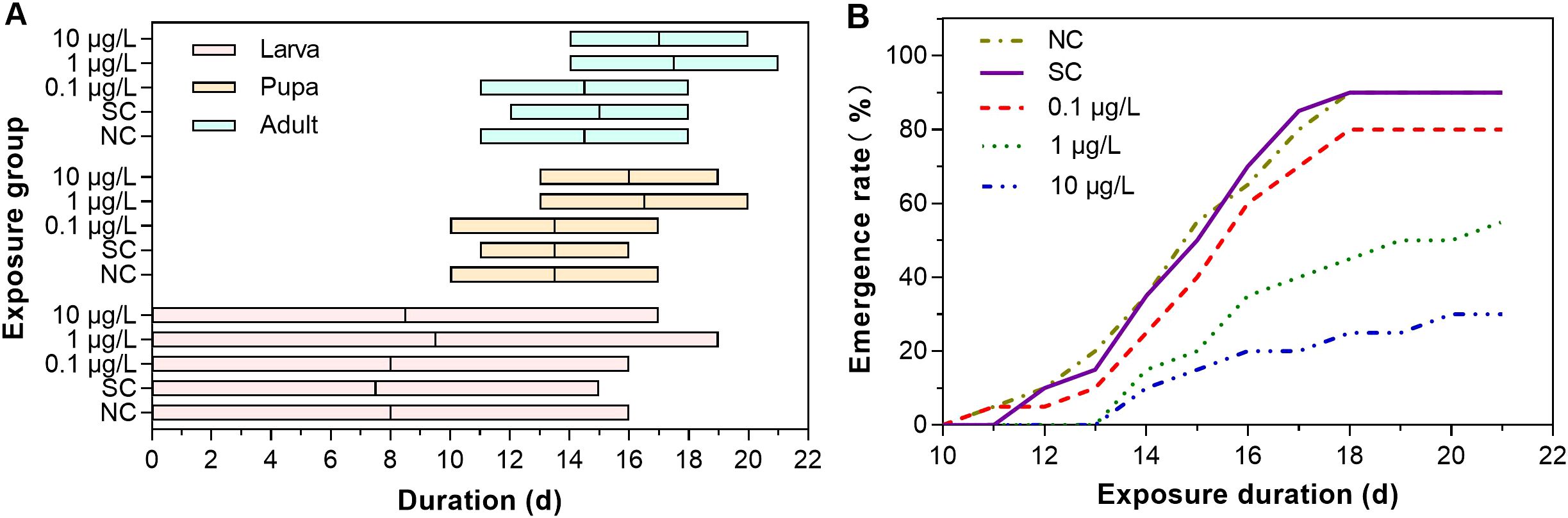
Figure 1. Duration of ecdysis of midges (A) and total emergence (B) of C. kiinensis adults after exposure to different concentrations of dinotefuran. NC, negative control; SC, solvent control.
3.2 Dinotefuran altered sex ratios of adults
After the larva of C. kiinensis was chronically exposed to different concentrations of dinotefuran, dinotefuran revealed its effects on the sex of emerged adults for the first group (Figure 2 and Supplementary Table S5). In the negative control and solvent control groups, 49.8% ± 7.1% and 51.7% ± 4.3% of the larvae were male, respectively. For the dinotefuran exposure groups DIN_1 to DIN_3, 50.6% ± 8.0%, 63.8% ± 5.4%, and 66.0% ± 5.7% of the larvae were male, respectively. Chronic exposures to dinotefuran elicited trends of significant sex ratio shifts toward male-dominant populations with concentrations increasing relative to the control.
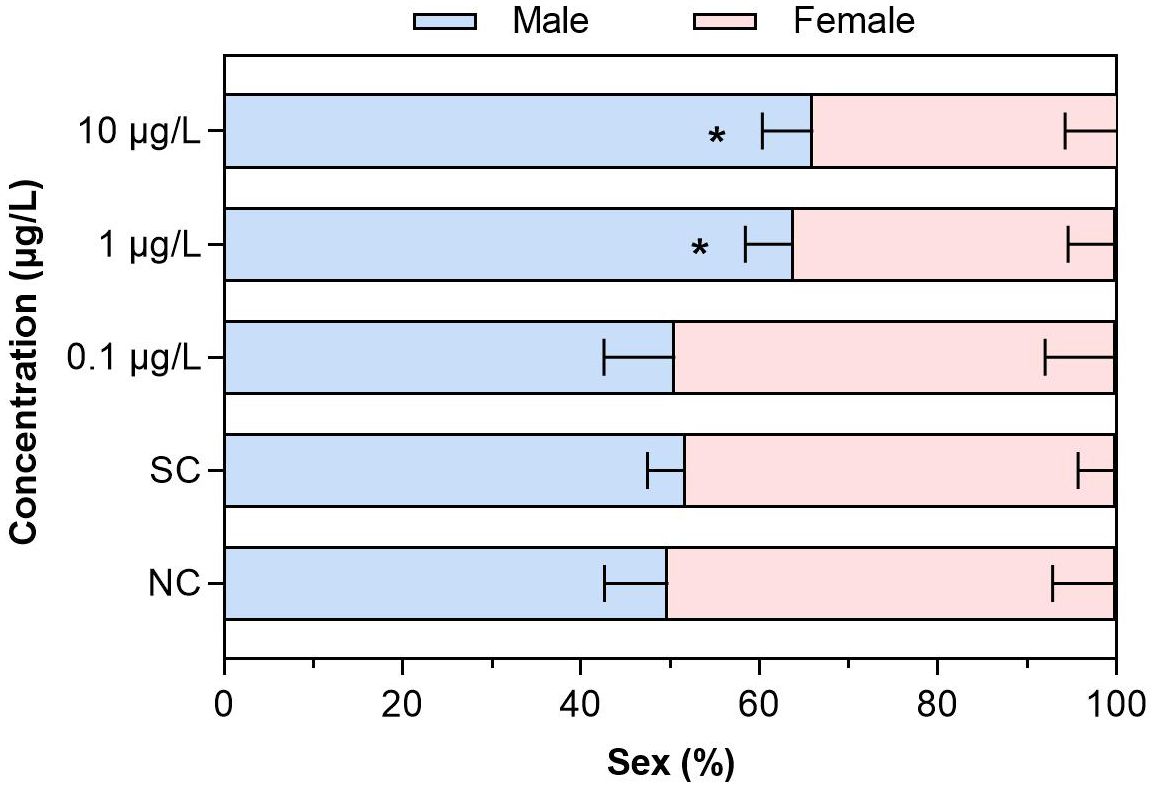
Figure 2. The sex (%) of C. kiinensis adults after chronic exposure to different concentrations of dinotefuran. Significance compared with solvent control was tested by ANOVA (n = 3, *p < 0.05). NC, negative control; SC, solvent control.
3.3 Dinotefuran reduced ATP levels
The levels of ATP in the second group were decreased after exposure to dinotefuran (Figure 3 and Supplementary Table S6). At 0.1 μg/L (DIN_1) of exposure concentration, the ATP was decreased but without significant difference. At higher concentrations, 1 and 10 μg/L (DIN_2 and DIN_3), significant reduction (p < 0.05) was observed.
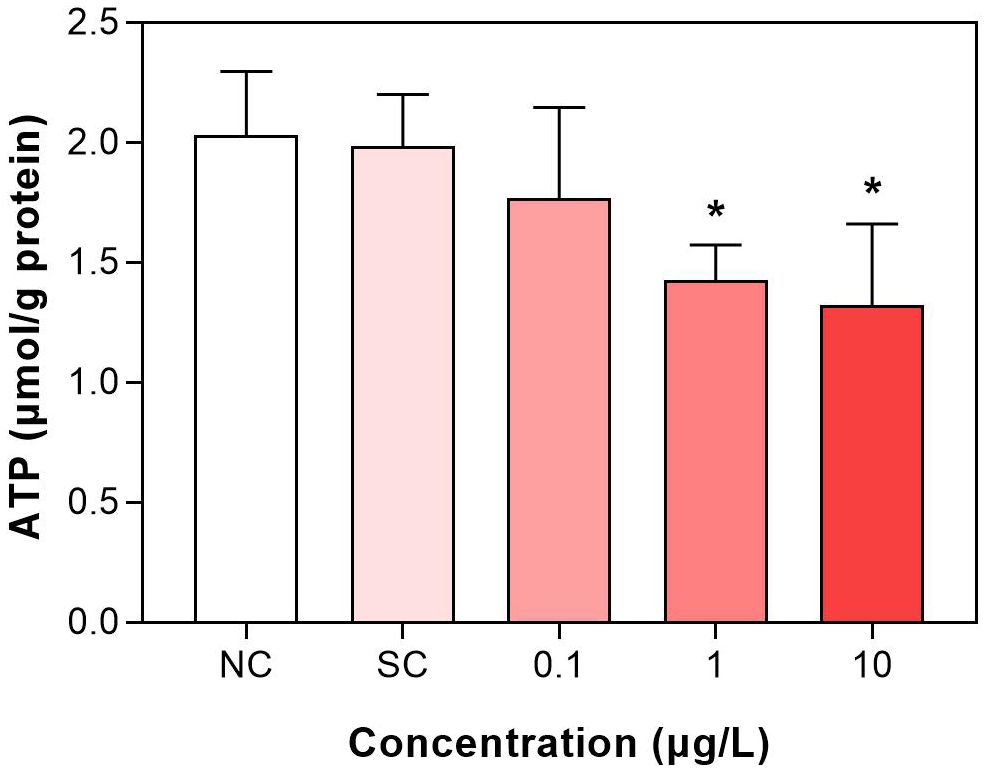
Figure 3. Adenosine triphosphate (ATP) level of C. kiinensis after 11 days of exposure to different concentrations of dinotefuran. Significance compared with solvent control was tested by ANOVA (n = 3, *p < 0.05). NC, negative control; SC, solvent control.
3.4 Dinotefuran dysregulated the levels of gene expressions related to ecdysone
For the third group, the gene expressions in the larvae were showed in Figure 4 and Supplementary Table S7. In terms of the molting, the gene expressions of ecr (ecdysone receptor) at DIN_2 and DIN_3 groups (1 and 10 μg/L) were significantly downregulated relative to the control group (Figure 4A), showing that with the increase of exposure concentration, the greater the downregulation on the gene expression. For usp (ultraspiracle protein, related to transcriptional effects of ecdysone) gene, its expression was significantly decreased only in the middle group (DIN_2, 1 μg/L) (Figure 4B). For E74 (an inducible factor related to ecdysone) gene, its expression was significantly decreased in the three exposure groups (DIN_1 to DIN_3) compared with the control group (Figure 4C). The gene expression of hsp (heat shock protein, playing a key role in the synthesis and maturation of steroid hormone receptors) was only significantly decreased in the highest exposure group (DIN_3, 10 μg/L, Figure 4D). For estrogen effects, gene expression of err (estrogen-related receptor) was not significantly altered in all exposure groups of dinotefuran, while the expression of vtg (vitellogenin, related to the estrogen effect) gene was upregulated in the DIN_2 group (1 μg/L) compared to the control (Figures 4E, F).
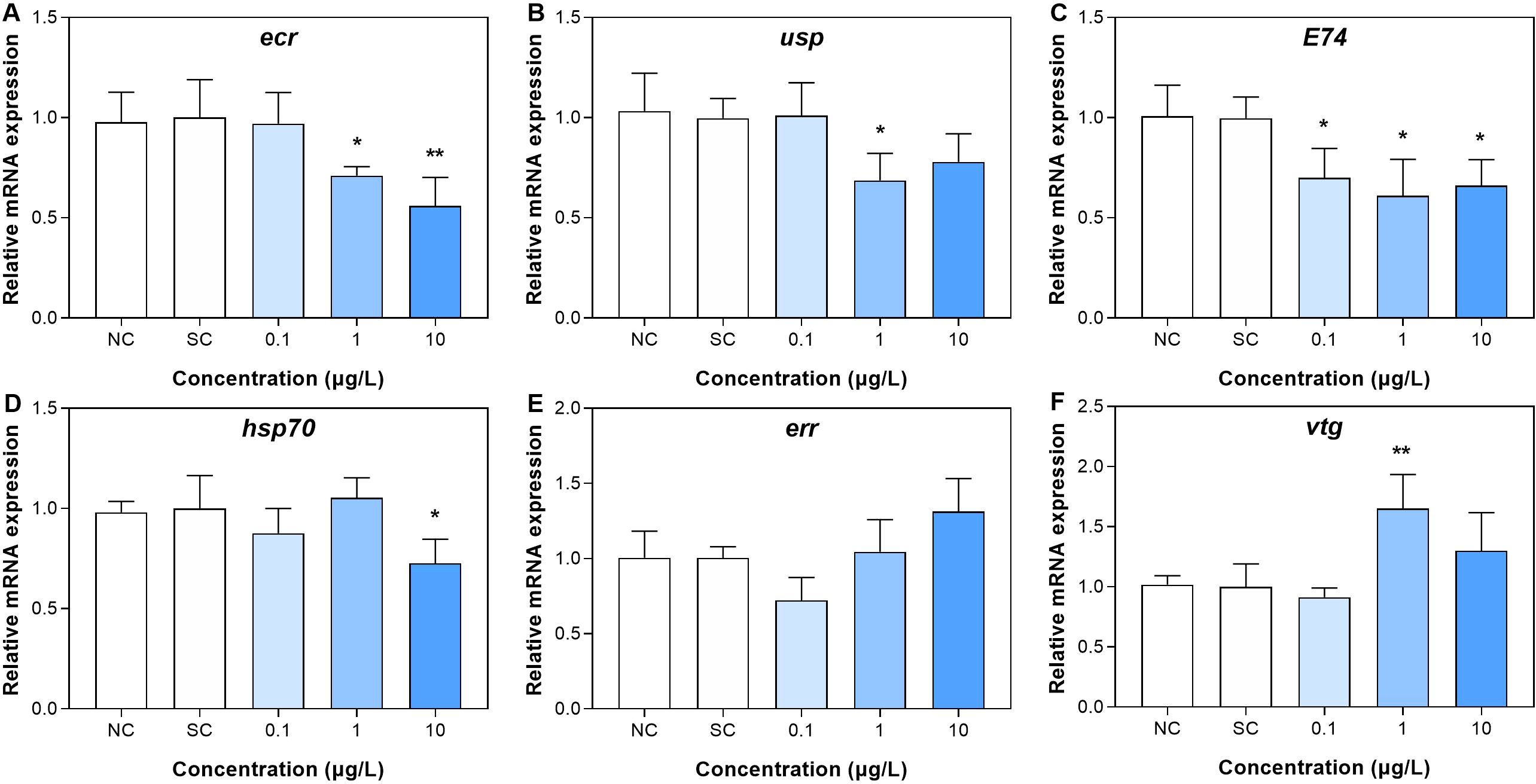
Figure 4. Gene expressions related to ecdysone (A–D) and estrogen (E, F) effect in larva after 11 days of exposure to dinotefuran. Data are expressed as mean ± standard error (n = 3). Significance compared with solvent control was tested by ANOVA (n = 3, *p < 0.05, **p < 0.01). NC, negative control; SC, solvent control.
4 Discussion
Neonicotinoid insecticides are widely used as alternatives of older classes of pesticides because they are less persistent in the environment and less toxic to mammals (including humans). It is well known that neonicotinoids damage the nervous system by binding to nAChRs, eventually leading to paralysis and even death of target insects (26). However, more and more studies found that besides targeting nAChRs, neonicotinoids could also induce other effects, such as interfering with energy metabolism in Chironomidae (22) and Xenopus laevis (27), leading to endocrine-disrupting effects in zebrafish (10). In particular, in terms of endocrine-disrupting effects, the mechanism of toxicity of neonicotinoid insecticides in Chironomidae is unclear.
4.1 Dinotefuran delayed the ecdysis activity of chironomids by regulating ecdysone-related gene expressions and reducing the ATP levels
In this study, the endocrine-disrupting effects of the representative neonicotinoid pesticide, dinotefuran, were investigated on Chironomidae. Our results found that dinotefuran delayed the ecdysis behavior of midges, especially during pupation and emergence at 1 and 10 μg/L. A few studies reported detected concentrations of dinotefuran in various water bodies. For example, Putri et al. (19) presented that the highest concentration of dinotefuran was 0.023 μg/L in estuaries and mangrove areas in tropical environmental waters of Indonesia. Xiong et al. (18) detected dinotefuran from a paddy field to receiving waters in Poyang Lake basin of China, with a mean concentration of 0.200 ± 0.296 μg/L and a maximum concentration of 0.802 ± 0.139 μg/L. Dinotefuran was also detected with a concentration of 0.0127–0.0755 μg/L in rivers near maize field in Ontario, Canada (20) and 0.0016–0.134 μg/L in streams across the United States (28). Thompson et al. (29) investigated dinotefuran in well tap water in eastern Iowa with a maximum concentration of 0.0039 μg/L. From the above reports, the range of dinotefuran was approximately 0.002–0.8 μg/L. The present study showed that 1 μg/L of dinotefuran delayed the ecdysis activity of chironomids, which was close to the highest environmental concentrations. Our results indicated that the effects of long-term exposure to environmental concentrations of dinotefuran on the growth and development of chironomids cannot be ignored.
For metamorphosed species, ecdysis is an important part of their life development. Li et al. (11) reported that imidacloprid caused honey bee larval developmental retardation by disrupting larval molting regulation. Krishnan et al. (14) revealed that neonicotinoids can cause arrested pupal ecdysis and adult eclosion in Lepidoptera. Ecdysis requires a large amount of energy supply, so the delay of ecdysis by neonicotinoids may be related to the energy interference effect of neonicotinoids. In this study, the levels of ATP were significantly decreased after exposure to dinotefuran at 1 and 10 μg/L (DIN_2 and DIN_3). A number of studies have reported that neonicotinoids could induce mitochondrial dysfunction. For example, Zhu et al. (27) revealed that chronic exposure to environment-related concentrations (5 μg/L) of dinotefuran led to mitochondria fusion and disturbed the mitochondrial respiratory chain of X. laevis, reducing ATP levels. Our previous study also showed that after exposure to the neonicotinoid insecticide imidacloprid, mitochondria cristae of Chironomidae disappeared and the morphology of mitochondria was altered using ultrastructural analysis; subsequently, the ATP levels were also reduced (22). Li et al. (11) also revealed that disruption of nutrient energy metabolism induced by imidacloprid caused developmental delay in honey bee.
The metamorphosis development of chironomids is mainly regulated by the combination of ecdysone and juvenile hormone, which control morphological events during ecdysis. Ecdysteroids promote pupation, while juvenile hormones maintain larval characteristics and prevent metamorphosis by countering the effects of 20-ecdysterone (20E) (30, 31). Dinotefuran inhibited the molting of chironomids, thus delaying their growth and development. It is possible that dinotefuran affected the secretion of ecdysone and juvenile hormone. The transcriptional effects of insect ecdysteroids require the action of two nuclear receptor superfamilies, the ecdysone receptor (EcR) and the ultraspiracle (USP). Activation of EcR/USP heterodimers initiates a series of expressions of ecdysone effect genes, leading to the disappearance of larval organs, as well as the differentiation of adult tissues (24). Our results showed that the gene expressions of ecr and usp were downregulated in groups treated with 1 and 10 μg/L of dinotefuran, manifesting that the transcriptions of ecdysteroids were inhibited, which interfered with normal molting activities. Li et al. (2023) reported that imidacloprid reduced 20E titer and inhibited Br-c expression, thereby blocking molting and causing stunting of honey bee larvae. Meanwhile, the developmental regulatory gene juvenile hormone acid methyl transferase (jhamt) was downregulated. In this study, environmental concentration of dinotefuran also downregulated the gene expression of E74. Among the ecdysone response genes, E74 transcription factor is an early gene of ecdysone induction (24). It plays a key role in the time of insect metamorphosis, and the expression pattern of this gene is associated with pupation (24). In addition, the hsp70 gene was downregulated in the 10 μg/L dinotefuran group. It is known that HSP70 plays important roles in the folding and maturation of steroid hormone receptors (32). In conclusion, environmental concentrations of dinotefuran delayed the ecdysis activity of chironomids via affecting the transcriptions of key genes ecr, usp, E74, and hsp70.
4.2 Dinotefuran shifted sex ratios toward male by regulating related gene expressions
The endocrine-disrupting effect of neonicotinoids may not only affect the secretion of ecdysone but also induce a sex-dependent response. Our results observed that dinotefuran shifted sex ratios of adult midges toward male-dominated populations with increasing concentration. A previous study has reported that after chronic exposure to imidacloprid, clothianidin, and thiamethoxam, the sex of C. dilutus was affected with the proportion of males increasing (33). Sandrock et al. (34) observed that in laboratory experiments, chronic neonicotinoid exposure led to a male-biased offspring sex ratio in the solitary bee species Osmia bicornis. However, the molecular mechanisms affecting the sex ratio are not well understood. In this study, a preliminary analysis on the causes of sex ratio changes at the genetic level was made. Firstly, we did not observe significant changes in err gene expression. Gene err, as the estrogen-related receptor gene, together with estrogen receptor genes, is involved in the estrogen signaling pathway and induces transcriptional activation of estrogen response genes. In addition, it also participates in the regulation of glycolysis and plays an important role in metabolic regulation and growth and development. Even so, there was upregulation of vtg gene under 1 μg/L of dinotefuran. Vtg gene is considered to be a key biomarker in assessing the vertebrate and non-vertebrate estrogenic effects of pollutants (35). Our results indicated that environmental concentrations of dinotefuran may induce estrogen effect via regulating the expression of vtg gene. In addition, ecdysone-responsive genes play an important role in sexual differentiation. Therefore, sex-dependent changes could determine transcriptional processes (24). During the fourth larval stage, the gene expressions of ecr, usp, E74, and vtg exhibited a sex-dependent response with significant differences between males and females of Chironomidae. In this study, although we did not compare the differences in the expression of these genes (ecr, usp, E74, and vtg) between the sexes, the expression of these genes was dysregulated, suggesting that changes in the sex ratio may be associated with changes in these genes’ expressions. There have been relevant findings that neonicotinoids caused sex-related difference effects. For example, clothianidin exposure led to sex-related behavioral effects, including decreased motor activity and elevated anxiety-like behaviors, that were more pronounced in males. The concentrations of clothianidin, along with most metabolites in blood and urine, were higher in males than in females (36). These results manifested that neonicotinoids produced sex-related differential effects. However, there is a lack of research on the mechanism of sex ratio change. Our results provided a preliminary insight into the causes of the change in sex ratio.
5 Conclusion
In summary, the environmental concentration of dinotefuran delayed pupation and emergence via inhibiting ecdysis. Sex ratios of adults shifted toward male-dominated populations with dinotefuran increasing. The corresponding transcription of molting genes was significantly altered in the midge. The delayed development was closely related to energy disturbance and the regulation of ecdysone. The mechanism between energy interference and molting needs to be further explored. In conclusion, these results would improve our understanding of the potential endocrine-disrupting mechanisms of neonicotinoid insecticides to Chironomidae.
Data availability statement
All datasets generated for this study are included in the article/Supplementary Material. More detailed data are available on request to the corresponding author.
Ethics statement
Ethical approval was not required for the study involving animals in accordance with the local legislation and institutional requirements because Chironomidae belong to the class of insects, a relatively low invertebrate, and do not require ethical certification.
Author contributions
FW: Conceptualization, Data curation, Funding acquisition, Methodology, Visualization, Writing – original draft. SW: Conceptualization, Investigation, Supervision, Writing – review & editing. LL: Data curation, Funding acquisition, Investigation, Writing – review & editing.
Funding
The author(s) declare financial support was received for the research, authorship, and/or publication of this article. This work was supported by the Natural Science Foundation of China (No. 42307369), the 2022 Featured Innovation Project for Ordinary University in Guangdong Provincial Department of Education (2022KTSCX130), the 2021 Young Innovative Talent Project for Ordinary University in Guangdong Provincial Department of Education (2021KQNCX087), and the Research Project of Jiaying University (2021KJZ03).
Conflict of interest
The authors declare that the research was conducted in the absence of any commercial or financial relationships that could be construed as a potential conflict of interest.
Publisher’s note
All claims expressed in this article are solely those of the authors and do not necessarily represent those of their affiliated organizations, or those of the publisher, the editors and the reviewers. Any product that may be evaluated in this article, or claim that may be made by its manufacturer, is not guaranteed or endorsed by the publisher.
Supplementary material
The Supplementary Material for this article can be found online at: https://www.frontiersin.org/articles/10.3389/fendo.2024.1459329/full#supplementary-material
References
1. Wang Z, Brooks BW, Zeng EY, You J. Comparative mammalian hazards of neonicotinoid insecticides among exposure durations. Environ Int. (2019) 125:9–24. doi: 10.1016/j.envint.2019.01.040
2. Hladik ML, Main AR, Goulson D. Environmental risks and challenges associated with neonicotinoid insecticides. Toxicol Rep. (2018) 52:3329–35. doi: 10.1021/acs.est.7b06388
3. Casida JE. Neonicotinoids and other insect nicotinic receptor competitive modulators: progress and prospects. Annu Rev Entomol. (2018) 63:125–44. doi: 10.1146/annurev-ento-020117-043042
4. Thompson DA, Lehmler HJ, Kolpin DW, Hladik ML, Vargo JD, Schilling KE, et al. A critical review on the potential impacts of neonicotinoid insecticide use: current knowledge of environmental fate, toxicity, and implications for human health. Environ Sci.: Process. Impacts. (2020) 22:1315–46. doi: 10.1039/c9em00586b
5. Zhang XD, Huang YH, Chen WJ, Wu SY, Lei QQ, Zhou Z, et al. Environmental occurrence, toxicity concerns, and biodegradation of neonicotinoid insecticides. Environ Res. (2023) 218:114953. doi: 10.1016/j.envres.2022.114953
6. Zhang D, Lu SY. Human exposure to neonicotinoids and the associated health risks: A review. Environ Int. (2022) 163:107201. doi: 10.1016/j.envint.2022.107201
7. Bhaskar R, Mohanty B. Pesticides in mixture disrupt metabolic regulation: in silico and in vivo analysis of cumulative toxicity of mancozeb and imidacloprid on body weight of mice. Gen Comp Endocrinol. (2014) 205:226–34. doi: 10.1016/j.ygcen.2014.02.007
8. Xu LW, Xu XX, Wu XL, Kuang H, Xu CL. Sex-dependent environmental health risk analysis of flupyradifurone. Environ Sci Technol. (2022) 56:1841–53. doi: 10.1021/acs.est.1c07726
9. Pandey SP, Mohanty B. The neonicotinoid pesticide imidacloprid and the dithiocarbamate fungicide mancozeb disrupt the pituitary–thyroid axis of a wildlife bird. Chemosphere. (2015) 122:227–34. doi: 10.1016/j.chemosphere.2014.11.061
10. Ma X, Xiong JJ, Li HZ, Brooks BW, You J. Long-term exposure to neonicotinoid insecticide acetamiprid at environmentally relevant concentrations impairs endocrine functions in zebrafish: bioaccumulation, feminization, and transgenerational effects. Environ Sci Technol. (2022) 56:12494–505. doi: 10.1021/acs.est.2c04014
11. Li Z, Wang YD, Qin QQ, Chen LC, Dang XQ, Ma ZG, et al. Imidacloprid disrupts larval molting regulation and nutrient energy metabolism, causing developmental delay in honey bee Apis mellifera. eLife. (2024) 12:88772. doi: 10.7554/eLife.88772
12. Taenzler V, Bruns E, Dorgerloh M, Pfeifle V, Weltje L. Chironomids: suitable test organisms for risk assessment investigations on the potential endocrine disrupting properties of pesticides. Ecotoxicology. (2007) 16:221–30. doi: 10.1007/s10646-006-0117-x
13. Morrissey CA, Mineau P, Devries JH, Sanchez-Bayo F, Liess M, Cavallaro MC, et al. Neonicotinoid contamination of global surface waters and associated risk to aquatic invertebrates: a review. Environ Int. (2015) 74:291–303. doi: 10.1016/j.envint.2014.10.024
14. Krishnan N, Jurenka RA, Bradbury SP. Neonicotinoids can cause arrested pupal ecdysis in Lepidoptera. Sci Rep. (2021) 11:15787. doi: 10.1038/s41598-021-95284-0
15. Sluijs JPVD, Simon-Delso N, Goulson D, Maxim L, Bonmatin JM, Belzunces LP. Neonicotinoids, bee disorders and the sustainability of pollinator services. Curr Opin Env. (2013) Sust. 5:293–305. doi: 10.1016/j.cosust.2013.05.007
16. Chen X, Dong F, Liu X, Xu J, Li J, Li Y, et al. Enantioselective separation and determination of the dinotefuran enantiomers in rice, tomato and apple by HPLC. J Sep. Sci. (2015) 35:200–5. doi: 10.1002/jssc.201100823
17. Hem L, Abd El-Aty AM, Park J-H, Shim J-H. Determination of dinotefuran in pepper using liquid chromatography: Contribution to safety evaluation. J Korean Soc Appl Bi. (2012) 55:765–8. doi: 10.1007/s13765-012-2140-3
18. Xiong JJ, Tan BX, Ma X, Li HZ, You J. Tracing neonicotinoid insecticides and their transformation products from paddy field to receiving waters using polar organic chemical integrative samplers. J Hazard. Mater. (2021) 413:125421. doi: 10.1016/j.jhazmat.2021.125421
19. Putri ZS, Sato T, Yamamuro M. Neonicotinoid occurrence and its potential toxicity level in tropical environmental waters of Indonesia. Limnology. (2023) 24:205–15. doi: 10.1007/s10201-023-00718-5
20. Schaafsma A, Limay-Rios V, Baute T, Smith J, Xue YG. Neonicotinoid insecticide residues in surface water and soil associated with commercial maize (corn) fields in Southwestern Ontario. PloS One. (2015) 10:e0118139. doi: 10.1371/journal.pone.0118139
21. Raby M, Zhao X, Hao C, Poirier DG, Sibley PK. Chronic toxicity of 6 neonicotinoid insecticides to Chironomus dilutus and Neocloeon triangulifer. Environ Toxicol Chem. (2018) 37:2727–39. doi: 10.1002/etc.4234
22. Wei FH, Wang DL, Li HZ, Xia P, Ran Y, You J. Toxicogenomics provides insights to toxicity pathways of neonicotinoids to aquatic insect, Chironomus dilutus. Environ. Pollut. (2020) 260:114011. doi: 10.1016/j.envpol.2020.114011
23. Park K, Kwak IS. Disrupting effects of antibiotic sulfathiazole on developmental process during sensitive life-cycle stage of Chironomus riparius. Chemosphere. (2018) 190:25–34. doi: 10.1016/j.chemosphere.2017.09.118
24. Planelló R, Herrero Ó, Gómez-Sande P, Ozáez I, Cobo F, Servia MJ. Ecdysone-related biomarkers of toxicity in the model organism Chironomus riparius: stage and sex-dependent variations in gene expression profiles. PloS One. (2015) 10:140239. doi: 10.1371/journal.pone.0140239
25. Livak KJ, Schmittgen TD. Analysis of relative gene expression data using realtime quantitative PCR and the 2–ΔΔCT method. Methods. (2001) 25:402–8. doi: 10.1006/meth.2001.1262
26. Anderson J, Dubetz C, Palace V. Neonicotinoids in the Canadian aquatic environment: a literature review on current use products with a focus on fate, exposure, and biological effects. Sci Total Environ. (2015) 505:409–22. doi: 10.1016/j.scitotenv.2014.09.090
27. Zhu JP, Tao Q, Du GY, Huang L, Li M, Wang MC, et al. Mitochondrial dynamics disruption: unraveling dinotefuran’s impact on cardiotoxicity. Environ pollut. (2024) 343:123238. doi: 10.1016/j.envpol.2023.123238
28. Hladik ML, Kolpin DW. First national-scale reconnaissance of neonicotinoid insecticides in streams across the USA. Environ Chem. (2016) 13:12–20. doi: 10.1071/EN15061
29. Thompson DA, Kolpin DW, Hladik ML, Lehmler HJ, Meppelink SM, Poch MC, et al. Prevalence of neonicotinoid insecticides in paired private-well tap water and human urine samples in a region of intense agriculture overlying vulnerable aquifers in eastern Iowa. Chemosphere. (2023) 319:137904. doi: 10.1016/j.chemosphere.2023.137904
30. Yuan D, Zhou S, Liu S, Li K, Zhao H, Long S, et al. The AMPK-PP2A axis in insect fat body is activated by 20- hydroxyecdysone to antagonize insulin/IGF signaling and restrict growth rate. PNAS. (2020) 117:9292–301. doi: 10.1073/pnas.2000963117
31. Luo W, Liu S, Zhang W, Yang L, Huang J, Zhou S, et al. Juvenile hormone signaling promotes ovulation and maintains egg shape by inducing expression of extracellular matrix genes. PNAS. (2021) 118:2104461118. doi: 10.1073/pnas.2104461118
32. Wegele H, Müller L, Buchner J. Hsp70 and Hsp90—a relay team for protein folding. Rev Physiol Biochem Pharmacol. (2004) 151:1–44. doi: 10.1007/s10254-003-0021-1
33. Maloney EM, Morrissey CA, Headley JV, Peru KM, Liber K. Can chronic exposure to imidacloprid, clothianidin, and thiamethoxam mixtures exert greater than additive toxicity in Chironomus dilutus? Ecotox Environ Safe. (2018) 156:354–65. doi: 10.1016/j.ecoenv.2019.109437
34. Sandrock C, Tanadini LG, Pettis JS, Biesmeijer JC, Potts SG, Neumann P. Sublethal neonicotinoid insecticide exposure reduces solitary bee reproductive success. Agr. For Entomol. (2014) 16:119–28. doi: 10.1111/afe.12041
35. Hahn T, Schenk K, Schulz R. Environmental chemicals with known endocrine potential affect yolk protein content in the aquatic insect Chironomus riparius. Environ. Pollut. (2002) 120:525–8. doi: 10.1016/S0269-7491(02)00189-6
Keywords: neonicotinoid insecticides, environmental concentration, endocrine disruption, Chironomidae, sex ratio
Citation: Wei F, Wu S and Li L (2024) Long-term exposure to environmental concentration of dinotefuran disrupts ecdysis and sex ratio by dysregulating related gene expressions in Chironomus kiinensis. Front. Endocrinol. 15:1459329. doi: 10.3389/fendo.2024.1459329
Received: 04 July 2024; Accepted: 12 August 2024;
Published: 05 September 2024.
Edited by:
Rui Zhang, University of Jinan, ChinaReviewed by:
Jie Li, Shandong Normal University, ChinaYuwei Xie, Ministry of Ecology and Environment, China
Copyright © 2024 Wei, Wu and Li. This is an open-access article distributed under the terms of the Creative Commons Attribution License (CC BY). The use, distribution or reproduction in other forums is permitted, provided the original author(s) and the copyright owner(s) are credited and that the original publication in this journal is cited, in accordance with accepted academic practice. No use, distribution or reproduction is permitted which does not comply with these terms.
*Correspondence: Shuangxin Wu, 409794313@qq.com