- 1Ecophysiological and Environmental Toxicological Research Group, HUN-REN Balaton Limnological Research Institute, Tihany, Hungary
- 2Bioorganic Research Institute, Suntory Foundation for Life Sciences, Kyoto, Japan
Despite the lack of endogenous synthesis and relevant nuclear receptors, several papers have been published over the decades claiming that the physiology of mollusks is affected by natural and synthetic sex steroids. With scant evidence for the existence of functional steroid nuclear receptors in mollusks, some scientists have speculated that the effects of steroids might be mediated via membrane receptors (i.e. via non-genomic/non-classical actions) - a mechanism that has been well-characterized in vertebrates. However, no study has yet investigated the ligand-binding ability of such receptor candidates in mollusks. The aim of the present study was to further trace the evolution of the endocrine system by investigating the presence of functional membrane sex steroid receptors in a mollusk, the great pond snail (Lymnaea stagnalis). We detected sequences homologous to the known vertebrate membrane sex steroid receptors in the Lymnaea transcriptome and genome data: G protein-coupled estrogen receptor-1 (GPER1); membrane progestin receptors (mPRs); G protein-coupled receptor family C group 6 member A (GPRC6A); and Zrt- and Irt-like protein 9 (ZIP9). Sequence analyses, including conserved domain analysis, phylogenetics, and transmembrane domain prediction, indicated that the mPR and ZIP9 candidates appeared to be homologs, while the GPER1 and GPRC6A candidates seemed to be non-orthologous receptors. All candidates transiently transfected into HEK293MSR cells were found to be localized at the plasma membrane, confirming that they function as membrane receptors. However, the signaling assays revealed that none of the candidates interacted with the main vertebrate steroid ligands. Our findings strongly suggest that functional membrane sex steroid receptors which would be homologous to the vertebrate ones are not present in Lymnaea. Although further experiments are required on other molluscan model species as well, we propose that both classical and non-classical sex steroid signaling for endocrine responses are specific to chordates, confirming that molluscan and vertebrate endocrine systems are fundamentally different.
1 Introduction
Despite the lack of endogenous synthesis and relevant nuclear receptors (1–9), several papers have been published over the past 70 years showing that the physiology of mollusks is affected by natural and synthetic sex steroids occurring in the environment, though the robustness of the bioassay data in most of these papers has been questioned (10). Recent reviews tried to fill in the gaps in steroid signaling of mollusks and raised the idea that vertebrate sex steroids affect molluscan species via membrane receptors (i.e. non-genomic/non-classical actions) (4, 11).
Most efforts to prove this possible mode of action were performed by the investigation of hemocytes of Mytilus spp. [reviewed by (4)]. These works demonstrated that estradiol (E2) rapidly induced lysosomal membrane instability, production of intracellular reactive oxygen species, lysozyme release and nitrogen-oxide production in Mytilus hemocytes; the underlying mechanisms, similar to those identified in mammalian cells, involved cytosolic Ca2+ increase, activation of MAPKs and PKC, as well as phosphorylation of signal transducers and transcription factors (12–15). However, it is unknown whether E2 binds to a specific membrane receptor in mollusks, if it cross-reacts with a receptor for other compound(s), or if there is a cognate sex steroid receptor at all. Furthermore, to our knowledge, no study has investigated the potential rapid effects of progesterone (P) and testosterone (T) on mollusks or conducted homology searches for their known membrane receptors in mollusks. Although recent studies have revealed sequences homologous to membrane progestin receptor beta (mPRβ) and gamma (mPRγ), two of the five vertebrate mPRs (16), in molluscan species such as the cuttlefish Sepia japonica (17), owl limpet Lottia gigantea, and Pacific oyster Crassostrea gigas (18), the ability of these receptor candidates to bind P has not been examined.
The aim of the present paper was to advance our understanding of the evolution of the endocrine system and to contribute to the ongoing debate about the role of vertebrate-type sex steroids in mollusks, by investigating the great pond snail (Lymnaea stagnalis). Lymnaea has long-served as a multipurpose molluscan model in neuroscience and neuroendocrinology [reviewed by (19–24)], as well as in ecotoxicological studies related to endocrine disruption (25–28). To accomplish our aim, we searched for sequences in the Lymnaea transcriptome and genome data that show homology with the known vertebrate membrane sex steroid receptors that are not related to nuclear receptors: G protein-coupled estrogen receptor-1 (GPER1), five mPRs, G protein-coupled receptor family C group 6 member A (GPRC6A), and Zrt- and Irt-like protein 9 (ZIP9). We cloned the coding sequence of selected candidates and transiently expressed them in Human Embryonic Kidney (HEK) cells to assess their membrane expression and ability to bind vertebrate sex steroids. Our findings represent the first evidence indicating the absence of specific, evolutionarily conserved membrane receptor-mediated sex steroid signaling in a mollusk.
2 Materials and methods
2.1 Experimental animals
5-month-old adult, mature specimens of Lymnaea were obtained from our laboratory-bred stocks. Snails were maintained in large plastic tanks containing 10 L oxygenated artificial snail water (composition in mM: 0.1309 NaHCO3, 0.0378 K2SO4, 0.4013 CaCl2.2H2O, 0.0390 Mg(NO3)2.6H2O; pH=7.6) at 20°C (± 1°C) on a 12:12 h light:dark regime with natural wavelength light. Specimens were fed on lettuce ad libitum three times a week.
2.2 In silico searches and bioinformatic analysis
The neural transcriptome data obtained in our previous study (29) were used to search for sequences homologous to vertebrate membrane sex steroid receptors. For homology-searching, the relevant molluscan and vertebrate sequences were used as search queries (Supplementary Table 1). We also compared the obtained sequences with the public Lymnaea CNS transcriptome shotgun assembly (sequence read archive: #DRR002012 (30);) and genome data (31). Conserved domain search using NCBI CDD/SPARCLE (32, 33) was first performed to check if the key regions were present in the deduced protein sequence. Phylogenetic analysis was made in the Molecular Evolutionary Genetics Analysis v7 software (34). Transmembrane domain prediction was performed with the TMHMM 2.0 and DeepTMHMM tools (35).
2.3 Plasmid constructions and transfection
Plasmid construction and transfection was according to the description of our previous study (36). The complete coding sequence of GPER1, mPR, and ZIP9 candidates was amplified with PrimeStar HS DNA Polymerase (#R010A, TaKaRa Bio, Japan). The applied gene-specific primers (designed with the SnapGene Viewer software (GSL Biotech)) with extensions to vector ends were as follows: 1) GPER1 candidate: AAT GCG GCC GCA TGG AGA CCA CAG TCG GGG (forward) and GCC TCT AGA CAG ATG GAC TGG TGG TTT CAG AAA GCC AT (reverse); 2) mPR candidate: AAT GCG GCC GCA TGT TGT TAC TGC CAG CAA CAT TGA G (forward) and GCC TCT AGA ATG GAT GAC CCC ATT TCT GTG ATG AA (reverse); 3) ZIP9 candidate: AAT GCG GCC GCA TGG ATG ATA TCT TGA CTC TCC TTT CTC TGT (forward) and GCC TCT AGA GTG CTT ATG ACC CAC AGC TAA AAA AAC A (reverse) (Integrated DNA Technologies, Belgium).
The amplicons were checked by gel electrophoresis, restricted with NotI – XbaI enzymes, purified by gel electrophoresis, and subcloned into the linearized pcDNA6-His-G16 plasmid [described in (37)] using T4 DNA ligase (#LGK-201, Toyobo, Japan). Since the coding sequence of GPRC6A candidate was too long to effectively amplify it, it was synthetized and ordered in pcDNA6-His-G16 plasmid as a ready-to-use vector (Eurofins Genomics). Codon usage optimized mPR and ZIP9 candidates in pcDNA6-His-G16 plasmids were synthesized by FASMAC (Kanagawa, Japan). After transformation and amplification in JM109 competent cells, colony PCR was carried out. Next, the plasmids were purified from the appropriate colonies with the QIAGEN Plasmid Midi Kit (#12143, Qiagen) and sequence verification by nucleotide sequencing was performed.
Finally, the constructed vectors were transiently transfected into genetically engineered HEK293MSR cells using the Lipofectamine 3000 Transfection reagent (#L3000001, Thermo Fisher Scientific) following the manufacturer’s instructions. Transfection efficiency and expression for all candidates were evaluated by an immunodetection method as described below.
2.4 Fluorescence immunocytochemistry
Immunocytochemistry was performed according to the description of our previous studies (36, 38). Briefly, 5 μg of the constructed pcDNA6-His-G16 vectors containing the receptor candidates were transfected into HEK293MSR cells in glass bottom dishes (35 mm diameter). As method controls, 5 μg of pcDNA6-His-G16 vectors containing GFP or Ciona galanin-like peptide receptor (GALPR) (37) were also transfected into HEK293MSR cells. After blocking with 4% goat serum (#S-1000-20, Vector Laboratories, USA), immunoreactions were performed using an anti-mouse G16 antibody (1:50; #TA808136, Origene, USA). The secondary antibody was a goat anti-mouse IgG antibody conjugated with Alexa488 antibody (1:500; #A-11029, Thermo Fisher Scientific). Nuclei were stained with Hoechst 33342 (1:1000; #AS-83218, AnaSpec, USA). The immunostaining was analyzed with a Fluoview FV3000 confocal laser microscope (Olympus, Tokyo, Japan) equipped with appropriate wavelength-filter configuration settings and a transmitted light detector. Image processing was performed by the Fluoview software (Olympus).
2.5 Second messenger assays
Intracellular Ca2+ mobilization was following the description of our previous studies (36, 38). Briefly, 1 × 106 HEK293MSR cells were cultured on polystyrene culture dishes (100 mm in diameter). A day later, 10 μg of pcDNA6-His-G16 vectors containing the receptor candidates or empty pcDNA6-His-G16 vector (i.e. Mock) were transfected into the HEK293MSR cells. After incubating for 24 h, 6 × 105 cells were plated in each of a 96-well plate. For the assays, synthetic P (#5341; Merck), T (#86500; Merck), and E2 (#E8875; Merck) were used. The stock solutions were prepared in ethanol weekly. In the positive control experiment, Ciona GALP (PFRGQGGWTLNSVGYNAGLGALRKLFE (39);) was applied. The real-time fluorescence assessment of Ca2+ mobilization was performed using the FLIPR Calcium 5 Kit (#3808451, Molecular Devices, USA) following the manufacturer’s instructions. All measurements were performed with a FlexStation III Multi Mode microplate reader (Molecular Devices). The data were obtained at least from two independent transfections for Ciona GALPR, all Lymnaea receptor candidates, and the empty vector. Ciona GALPR and empty vector samples were applied to the plate in two technical replicates, while all Lymnaea receptor candidate samples were applied in three technical replicates.
2.6 Statistical analysis
The data obtained during the second messenger assays were plotted using four-parameters logistics-based non-linear regression curve fit feature in the GraphPad Prism5 software (GraphPad, San Diego, USA). Significant differences at a given data point was analyzed with the OriginPro 2018 software (OriginLab Corp., USA). The normality of the datasets was investigated using the Shapiro-Wilk test and the homogeneity of variances between groups was checked with the Levene-test. The data were then analyzed using the two-sample t-test.
3 Results
Our homology-searching revealed candidate sequences for all vertebrate membrane sex steroid receptors which are not related to the nuclear ones (Supplementary Figure 1). After the initial bioinformatic analysis, including conserved domain analysis (Supplementary Figure 2), phylogenetics (Supplementary Figures 3-5), and transmembrane domain prediction (Supplementary Figure 6), four sequences were investigated further: one GPER1 candidate, one mPR candidate, one GPRC6A candidate, and one ZIP9 candidate. Based on the in silico analyses, the mPR and ZIP9 candidates appeared to be genuine homologs. Although the GPER1 and GPRC6A candidates were unlikely to be the counterparts of the vertebrate receptors, we did not exclude the possibility that, during evolution, these deduced proteins might have acquired the ability of recognizing steroids as well [as reported in the case of vertebrate ZIP9 (40)].
First, we investigated the expression and subcellular localization of the candidates by transiently transfecting the G16-fused coding sequences into HEK293MSR cells. In the positive control experiments (Supplementary Figure 7), the expression level of GFP indicated an appropriate transfection efficiency (Supplementary Figure 7A). Moreover, the G16-fused Ciona GALPR showed a high expression and localization at the plasma membrane (Supplementary Figure 7B). Immunocytochemical fluorescence confocal microscopic detection confirmed that the Lymnaea membrane sex steroid receptor candidates were localized to the cell surface of HEK293MSR cells (Figure 1). The expression of the GPER1 and GPRC6A candidates was high. However, the expression of the mPR1 and ZIP9 candidates was very low and only a few cells showing immunosignal were detected. To evaluate the ligand-receptor interaction between the three main vertebrate sex steroids and the Lymnaea membrane sex steroid receptor candidates, we assessed the mobilization of intracellular Ca2+ using G16-fused receptor candidates (41). In the positive control experiment (Supplementary Figure 7C), the Ciona GALP specifically induced the elevation of intracellular Ca2+ in a concentration-dependent manner, as previously reported (37). In the case of the Lymnaea candidates, however, the assay showed that the respective ligand-receptor combinations failed to induce the elevation of intracellular Ca2+ (Figure 2), indicating that these transmembrane proteins are not bona fide membrane sex steroid receptors. Both in the case of GPRC6A and ZIP9 candidates, there was an increase in response at the 10-7 M concentration, but statistical analysis with two-sample t-test revealed no significant difference compared to the response of HEK cells expressing the empty vector.
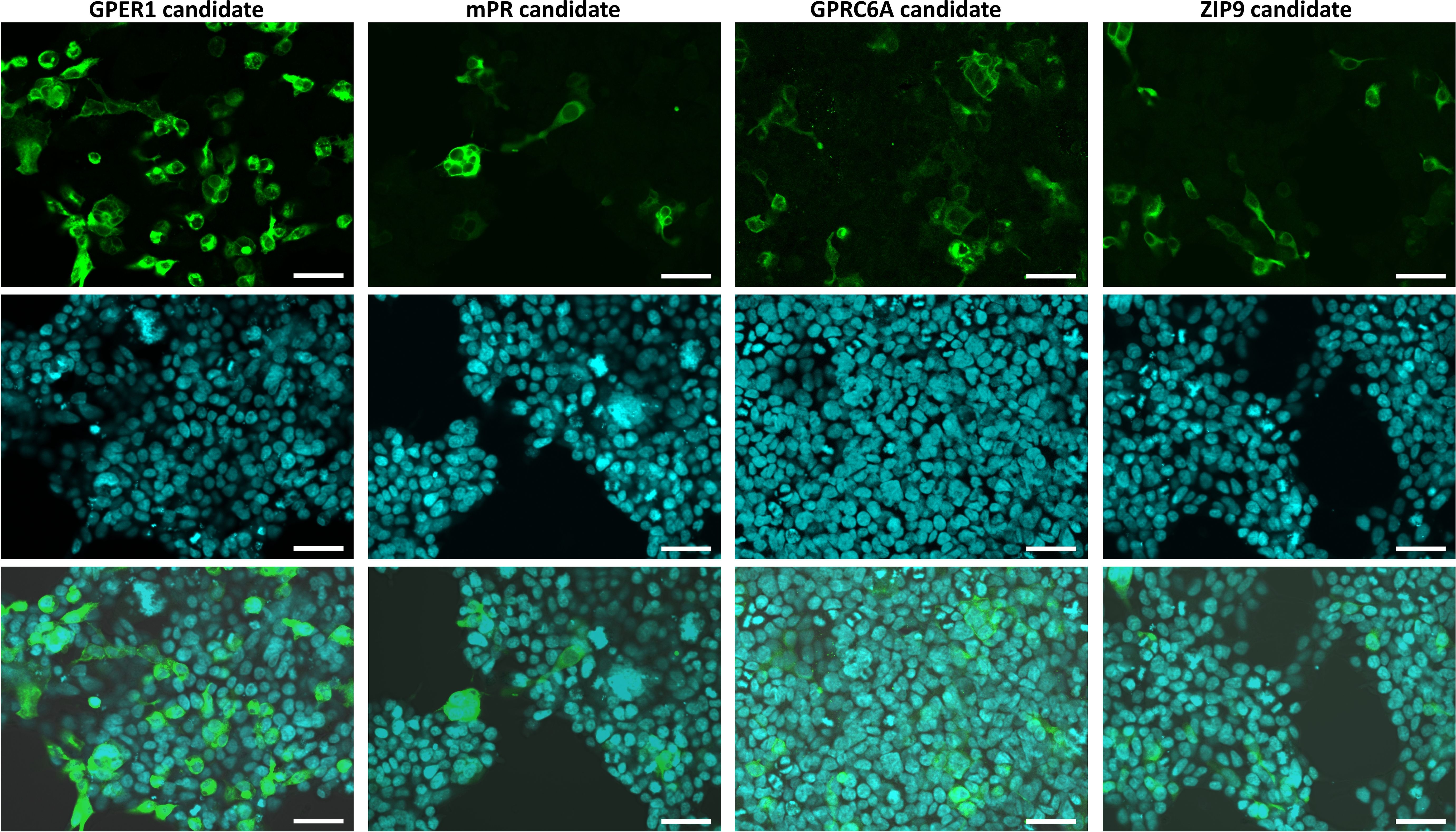
Figure 1. Confocal microscopic observation of immunostained HEK293MSR cells expressing the different Lymnaea membrane sex steroid receptor candidates fused with G16 protein. Expressed candidates stained with an anti-G16 antibody (green; top row), nuclei stained with Hoechst 33342 (blue; middle row), and merged views (bottom row) of the transfectants. The candidates were localized at the plasma membrane. The expression of the GPER1 and GPRC6A candidates were high, while the expression of the mPR1 and ZIP9 candidates were very low (only a few cells showing immunosignal were detected). Bars = 50 μm.
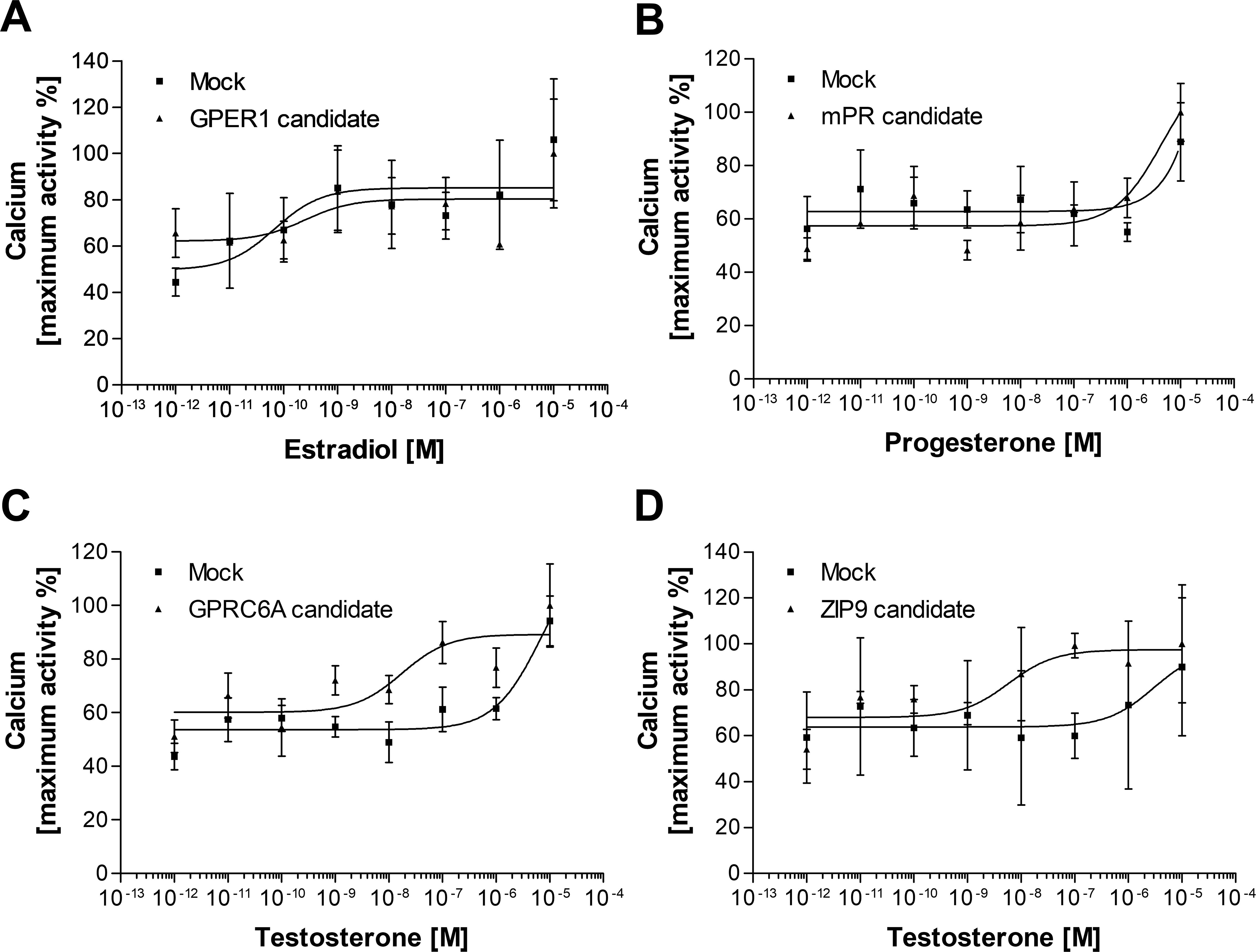
Figure 2. Second messenger assay (Ca2+ mobilization) for assessing the potential ligand-receptor interaction between vertebrate type-sex steroids and Lymnaea membrane sex steroid receptor candidates. Dose-response curve of E2 in GPER1 candidate-expressing HEK cells (A), P in mPR candidate-expressing cells (B), and T in GPCR6A candidate or ZIP9 candidate-expressing HEK cells (C, D). Empty vector was transfected as the negative control (Mock). None of the candidates were responsive to the main steroid ligand. Data points are means ± SEM of two (A, B, D) or three (C) independent transfections.
We did not exclude the possibility that the mPR and ZIP9 homologues were unresponsive due to their low expression. Hence, we applied codon usage optimization for both sequences (Supplementary Figure 8) and investigated their expression and ligand-binding interaction again (Figure 3). Although the expression of both candidates was improved after the codon usage optimization (Figure 3A), they were still found to be unresponsive to vertebrate sex steroids (Figures 3B, C).
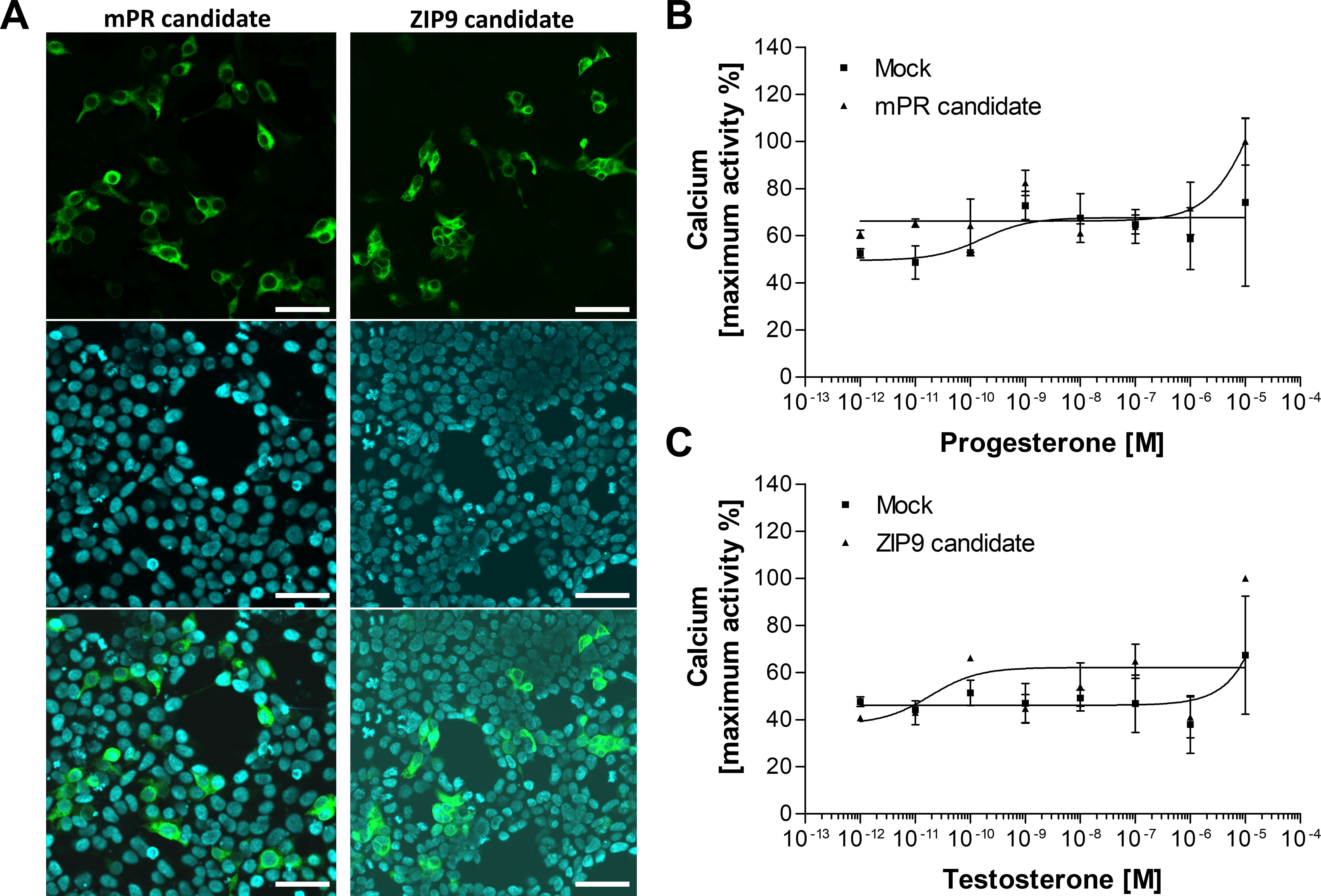
Figure 3. Confocal microscopic observation of immunostained HEK293 cells expressing codon usage optimized mPR and ZIP9 candidates fused with G16 protein (A) and functional receptor assay for assessing ligand-binding (B, C). (A) Expressed receptor candidates stained with anti-G16 antibody (green), nuclei stained with Hoechst 33342 (blue), and merged views of the transfectants. The candidates were localized at the plasma membrane. The expression of both candidates significantly improved due to the codon usage optimization. Bars = 50 μm. Dose-response curve of P in mPR candidate-expressing cells (B) and T in ZIP9 candidate-expressing HEK cells (C). Empty vector was transfected as the negative control (Mock). Both candidates were unresponsive to the main steroid ligand. Data points are means ± SEM of two independent transfections.
4 Discussion
There is a long-standing debate regarding whether vertebrate sex steroids can affect the physiology of mollusks. Sex steroids are present in tissues of a wide range of mollusks, and their concentration sometimes show an association with stages of gonad development or differences between tissues and sexes [reviewed by (1)]. However, since the 2000s, it has become evident that mollusks possess a remarkable ability to absorb and retain vertebrate sex steroids for extended periods, even months (42–51). Consequently, their presence in molluscan tissues does not necessarily indicate an endogenous origin. Given that vertebrate sex steroids have been present in the aquatic environment - and thus in molluscan tissues - for a considerable part of molluscan evolutionary history, the question arises as to whether evolution has favored the development of signaling systems to recognize these compounds.
Sex steroid signaling in vertebrates is regulated via three possible pathways (Figure 4). First, through nuclear receptors localized in the cytoplasm or nucleus (Figure 4A) (52). Second, following post-translational modification, the nuclear receptors relocate to the plasma membrane to facilitate the rapid effects of sex steroids (Figure 4B) (52, 53). Considering the absence of relevant functional nuclear receptors in mollusks, the nuclear receptor-mediation of sex steroids via these pathways can be excluded. Previous screenings of Lymnaea genome and transcriptome data did not identify functional nuclear sex steroid receptors either (2, 31, 54). We are careful to use the word ‘functional’ in this context, as molluscan genomes all contain a gene that was initially annotated as being a homologue of the vertebrate nuclear estrogen receptor (nER), although it is actually orthologous to the common ancestor of vertebrate estrogen and oxosteroid receptors (55). Moreover, all studies on mollusks to date, except one (56), have shown that molluscan nERs do not bind to E2 and are, in fact, in an already activated state (57–59). Previously, the nER of the scallop was reported to be bind to E2 (56). Nevertheless, the hybrid vector used in that study was made up of fish and scallop sequences, and the binding evidence was a two-fold increase at one dose of E2. Hence, we would argue that data are insufficient to refer to the scallop nER as a ‘functional’ E2 receptor and support the proposal of (60) that sequences previously termed molluscan nER should be renamed NR3D. The third possible pathway involves rapid effects mediated by membrane receptors which are distinct from nuclear receptors (Figure 4C) (16, 40, 61–63). Given that GPCRs trace their origins back into history over one billion years, whereas the original ancestral nuclear steroid receptor appeared about 500-600 million years ago (7), other works (4, 11) have very reasonably suggested that sex steroids affect the physiology of mollusks through this mechanism.
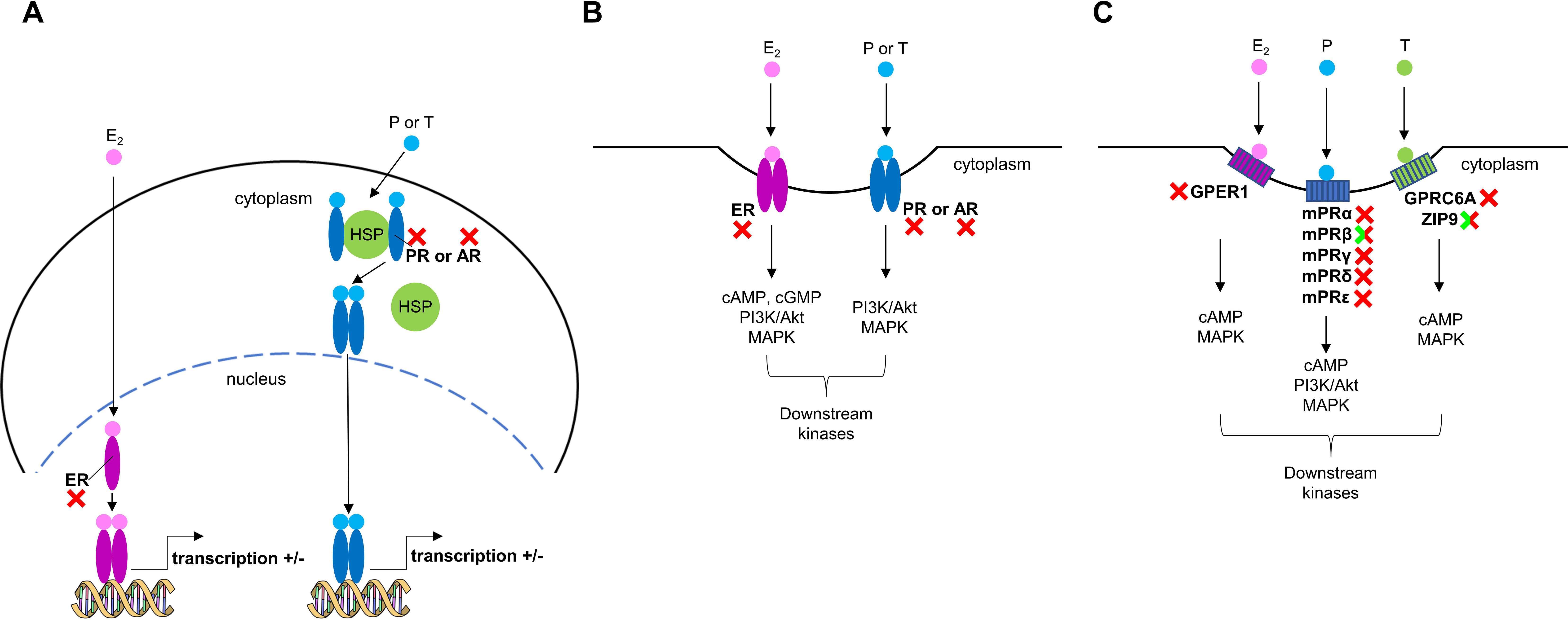
Figure 4. Sex steroid signaling in vertebrates. (A) Classic nuclear pathway involving estrogen (ER), progesterone (PR), and androgen (AR) receptors, which are primarily located as monomers in the nucleus or as monomers bound to heat shock proteins (HSPs) in the cytoplasm. After steroid binding, dimerization, changes in receptor conformation (and nuclear localization), the receptors bind to specific steroid-response elements (SREs) to modulate gene transcription. (B) The membrane forms of ER, PR, and AR are tethered to the cell membrane and can also bind sex steroids activating G proteins (e.g., Gs) and/or kinases (e.g., PI3K/Akt pathway). (C) Membrane sex steroid receptors (GPCRs and non-GPCRs) which are unrelated to ER, PR, and AR can also bind sex steroids activating G proteins and kinases. Red X symbols mark vertebrate receptors which have no homologs in Lymnaea, while green/red X symbols indicate vertebrate receptors which have structural but, in terms of the sex steroid signaling, not functional homologs in Lymnaea.
The rapid effects of E2 in vertebrates are mediated by a single GPCR called GPER1 [for detailed reviews, see (62, 63)]. Our candidate was unresponsive to E2, suggesting that no GPER1 homolog is present in Lymnaea. The identified sequence likely belongs to the protostome allatostatin-C receptors, which are closely related to echinoderm somatostatin/allatostatin-C-type receptors and chordate somatostatin-type and opioid-type receptors (Supplementary Figure 3) (64, 65). Without assessing the robustness of previously reported rapid effects of E2 on Mytilus hemocytes, we note that only the lysosomal membrane stability-decreasing effect has so far been properly verified. This classic ‘immune response’, as also referenced by the authors of the original study (4), led to the hypothesis the E2 may act as a xenobiotic ‘inflammatory agent’ for mollusks. Consistent with the previous idea that recognition and deactivation of xenobiotics were significant evolutionary pressures for receptor development (66), we hypothesize that mollusks have an unknown specific membrane receptor, though not a GPER1 homolog, capable of binding E2 and initiating a rapid immune response.
Rapid actions of P are implemented by five mPR subtypes, which are not GPCRs but belong to the progestin and adipoQ receptor (PAQR) family, in vertebrates [for recent detailed reviews, see (16, 61)]. The previous scenario was that mPRs are exclusive to chordates (67). However, a recent comprehensive genomic analysis of metazoans revealed homologs for mPRβ and mPRγ in bivalves, suggesting that the five vertebrate mPRs evolved from an ancestral metazoan mPRγ (18). It is also noteworthy that mPRα, which has been shown to mediate most mPR functions in vertebrate cells (even when they express all isoforms), is absent in invertebrates. Our in silico revealed only one potential mPR candidate in Lymnaea. Conservation analysis of this sequence yielded similar results to those previously reported in mollusks (Supplementary Figures 9, 10) (17). However, our ligand-receptor interaction experiment showed that this candidate did not respond to P, suggesting the absence of an mPR homolog in Lymnaea. Adiponectin receptor, a member of PAQR family, was recently identified and showed to be involved in learning and memory formation of Lymnaea (68). Our identified sequence may also belong to the PAQR family (Supplementary Figure 4) and use adiponectin as its ligand, but further studies are required to confirm this assumption. Collectively, the present study supports the original theory that functional mPRs are exclusive to chordates.
Non-genomic effects of T in vertebrates are mediated by two proteins, GPRC6A (GPCR) and ZIP9 (non-GPCR) [reviewed by (40, 69)]. Our homology-searches revealed one candidate for both proteins in Lymnaea, but neither responded to T, suggesting the absence of mAR homologs in this species. GPRC6A is also known to be activated by L-α-amino acids (40). Based on our in silico analyses (Supplementary Figures 2, 5), we suppose that the identified Lymnaea sequence functions as a metabotropic glutamate receptor, although experimental validation awaits further studies. In vertebrate cells, ZIP9 has dual functions as a zinc transporter and as a mAR activating second messengers (40). Since Lymnaea ZIP9 was unresponsive to T, we hypothesize that its sole function is to participate in zinc homeostasis.
In summary, our findings strongly suggest that functional homologs of vertebrate membrane sex steroid receptors are not present in Lymnaea. Although further experiments are required on other molluscan model species as well, we propose that both classical and non-classical sex steroid signaling for endocrine responses are specific to chordates. In light of this, it remains an important and unanswered question how vertebrate steroids affect the physiology of mollusks. Vertebrate sex steroids are probably xenobiotic ‘inflammatory agents’ for mollusks and may be recognized by membrane receptors involved in rapid immune responses. Another potential target for endocrine disruption by vertebrate steroids in mollusks might be an ecdysone-like pathway. Ecdysone signaling was long thought to be exclusive to arthropods; however, studies from 2010 have demonstrated the presence of an orthologue of the EcR/NR1H receptor in other protostome taxa, including mollusks and annelids (70). In insects, 20-hydroxyecdysone exerts both genomic and non-genomic actions (71). It is noteworthy that GPCR-mediated effects of 20-hydroxyecdysone have also been described in mammals, demonstrating the activation of an endogenous signaling pathway by a clearly exogenous steroid (72). Our homology searches revealed Lymnaea sequences homologous to both insect nuclear and membrane (GPCR) ecdysone receptors (Supplementary Figure 11), paving the way for future studies aimed at investigating this potential target pathway. Our findings have implications not only for the fields of endocrinology and evolution but also for ecology: it is important to consider the signaling pathways and responsiveness of mollusks to vertebrate sex steroids when assessing the impact of these compounds on ecosystems.
Data availability statement
The datasets presented in this study can be found in online repositories. The names of the repository/repositories and accession number(s) can be found in the article/Supplementary Material.
Ethics statement
Ethical approval was not required for the studies on humans in accordance with the local legislation and institutional requirements because only commercially available established cell lines were used. The manuscript presents research on animals that do not require ethical approval for their study.
Author contributions
IF: Conceptualization, Data curation, Funding acquisition, Investigation, Visualization, Writing – original draft. SM: Data curation, Funding acquisition, Investigation, Methodology, Visualization, Writing – review & editing. TO: Data curation, Funding acquisition, Investigation, Methodology, Visualization, Writing – review & editing. AS: Investigation, Methodology, Writing – review & editing. TK: Investigation, Writing – review & editing. HS: Funding acquisition, Writing – review & editing. ZP: Funding acquisition, Writing – review & editing.
Funding
The author(s) declare financial support was received for the research, authorship, and/or publication of this article. This work was supported by the National Brain Project (#NAP2022-I-10/2022, ZP), the Hungarian Scientific Research Fund (#138039, ZP; #146787, IF), the HUN-REN Mobility Program (#ELKH-KMP-2023/26, IF), the FY2023 JSPS Postdoctoral Fellowship for Research in Japan (#PE23039, IF), and the Grants-in-Aid for Scientific Research from JSPS (#JP22K06327, TO; #JP22K06307, SM; #JP22H02658, HS).
Acknowledgments
The authors thank Prof. Alexander P. Scott (CEFAS, Weymouth, UK) for checking the English of the manuscript and providing useful suggestions. The authors thank the Reviewers for providing constructive comments and suggestions on the manuscript.
Conflict of interest
The authors declare that the research was conducted in the absence of any commercial or financial relationships that could be construed as a potential conflict of interest.
The author(s) declared that they were an editorial board member of Frontiers, at the time of submission. This had no impact on the peer review process and the final decision.
Publisher’s note
All claims expressed in this article are solely those of the authors and do not necessarily represent those of their affiliated organizations, or those of the publisher, the editors and the reviewers. Any product that may be evaluated in this article, or claim that may be made by its manufacturer, is not guaranteed or endorsed by the publisher.
Supplementary material
The Supplementary Material for this article can be found online at: https://www.frontiersin.org/articles/10.3389/fendo.2024.1458422/full#supplementary-material
References
1. Scott AP. Do mollusks use vertebrate sex steroids as reproductive hormones? Part I. Critical appraisal of the evidence for the presence, biosynthesis and uptake of steroids. Steroids. (2012) 77:1450–68. doi: 10.1016/j.steroids.2012.08.009
2. Fodor I, Urban P, Scott AP, Pirger Z. A critical evaluation of some of the recent so-called 'evidence' for the involvement of vertebrate-type sex steroids in the reproduction of mollusks. Mol Cell Endocrinol. (2020) 516:110949. doi: 10.1016/j.mce.2020.110949
3. Horiguchi T, Ohta Y. A critical review of sex steroid hormones and the induction mechanisms of imposex in gastropod mollusks. In: Saber Saleuddin ABL, Orchard I, editors. Advances in invertebrate (Neuro)Endocrinology, 1st edition. Boca Raton, Florida, USA: Apple Academic Press (2020).
4. Balbi T, Ciacci C, Canesi L. Estrogenic compounds as exogenous modulators of physiological functions in molluscs: Signaling pathways and biological responses. Comp Biochem Physiol C Toxicol Pharmacol. (2019) 222:135–44. doi: 10.1016/j.cbpc.2019.05.004
5. Fodor I, Pirger Z. From dark to light - an overview of over 70 years of endocrine disruption research on marine mollusks. Front Endocrinol. (2022) 13:903575. doi: 10.3389/fendo.2022.903575
6. Markov GV, Tavares R, Dauphin-Villemant C, Demeneix BA, Baker ME, Laudet V. Independent elaboration of steroid hormone signaling pathways in metazoans. Proc Natl Acad Sci USA. (2009) 106:11913–8. doi: 10.1073/pnas.0812138106
7. Markov GV, Gutierreze-Mazariegos J, Pitrat D, Billas IML, Bonneton F, Moras D, et al. Origin of an ancient hormone/receptor couple revealed by resurrection of an ancestral estrogen. Sci Adv. (2017) 3:e1601778. doi: 10.1126/sciadv.1601778
8. Baker ME. Steroid receptors and vertebrate evolution. Mol Cell Endocrinol. (2019) 496:110526. doi: 10.1016/j.mce.2019.110526
9. Fernandes D, Loi B, Porte C. Biosynthesis and metabolism of steroids in molluscs. J Steroid Biochem Mol Biol. (2011) 127:189–95. doi: 10.1016/j.jsbmb.2010.12.009
10. Scott AP. Do mollusks use vertebrate sex steroids as reproductive hormones? II. Critical review of the evidence that steroids have biological effects. Steroids. (2013) 78:268–81. doi: 10.1016/j.steroids.2012.11.006
11. Tran TKA, Yu RMK, Islam R, Nguyen THT, Bui TLH, Kong RYC, et al. The utility of vitellogenin as a biomarker of estrogenic endocrine disrupting chemicals in molluscs. Environ pollut. (2019) 248:1067–78. doi: 10.1016/j.envpol.2019.02.056
12. Canesi L, Ciacci C, Lorusso LC, Betti M. Effects of endocrine disrupting chemicals on the immune system of the edible bivalve mollusc mytilus. In: Marino M, Mita DG, editors. The Endocrine Disruptors. Trivandrum, India: Transworld Research Network (2007). p. 1–12.
13. Canesi L, Ciacci C, Lorusso LC, Betti M, Guarnieri T, Tavolari S, et al. Immunomodulation by 17beta-estradiol in bivalve hemocytes. Am J Physiol Regul Integr Comp Physiol. (2006) 291:R664–73. doi: 10.1152/ajpregu.00139.2006
14. Canesi L, Lorusso LC, Ciacci C, Betti M, Rocchi M, Pojana G, et al. Immunomodulation of Mytilus hemocytes by individual estrogenic chemicals and environmentally relevant mixtures of estrogens: in vitro and in vivo studies. Aquat Toxicol. (2007) 81:36–44. doi: 10.1016/j.aquatox.2006.10.010
15. Canesi L, Ciacci C, Betti M, Lorusso LC, Marchi B, Burattini S, et al. Rapid effects of 17beta-estradiol on cell signaling and function of Mytilus hemocytes. Gen Comp Endocrinol. (2004) 136:58–71. doi: 10.1016/j.ygcen.2003.12.003
16. Thomas P, Pang Y, Camilletti MA, Castelnovo LF. Functions of membrane progesterone receptors (mPRs, PAQRs) in nonreproductive tissues. Endocrinology. (2022) 163:bqac147. doi: 10.1210/endocr/bqac147
17. Pang Z, Lu Z, Wang M, Gong L, Liu B, Jiang L, et al. Characterization, relative abundances of mRNA transcripts, and subcellular localization of two forms of membrane progestin receptors (mPRs) in the common Chinese cuttlefish, Sepiella japonica. Anim Reprod Sci. (2019) 208:106107. doi: 10.1016/j.anireprosci.2019.106107
18. Ren J, Chung-Davidson YW, Jia L, Li W. Genomic sequence analyses of classical and non-classical lamprey progesterone receptor genes and the inference of homologous gene evolution in metazoans. BMC Evol Biol. (2019) 19:136. doi: 10.1186/s12862-019-1463-7
19. Fodor I, Hussein AA, Benjamin PR, Koene JM, Pirger Z. The unlimited potential of the great pond snail, Lymnaea stagnalis. eLife. (2020) 9:e56962. doi: 10.7554/eLife.56962
20. Rivi V, Benatti C, Colliva C, Radighieri G, Brunello N, Tascedda F, et al. Lymnaea stagnalis as model for translational neuroscience research: From pond to bench. Neurosci Biobehav Rev. (2020) 108:602–16. doi: 10.1016/j.neubiorev.2019.11.020
21. Rivi V, Benatti C, Lukowiak K, Colliva C, Alboni S, Tascedda F, et al. What can we teach Lymnaea and what can Lymnaea teach us? Biol Rev Camb Philos Soc. (2021) 96:1590–602. doi: 10.1111/brv.1271622
23. Koene JM. Neuro-endocrine control of reproduction in hermaphroditic freshwater snails: mechanisms and evolution. Front Behav Neurosci. (2010) 4:167. doi: 10.3389/fnbeh.2010.00167
24. Nakai J, Chikamoto N, Fujimoto K, Totani Y, Hatakeyama D, Dyakonova VE, et al. Insulin and memory in invertebrates. Front Behav Neurosci. (2022) 16:882932. doi: 10.3389/fnbeh.2022.882932
25. Zrinyi Z, Maasz G, Zhang L, Vertes A, Lovas S, Kiss T, et al. Effect of progesterone and its synthetic analogs on reproduction and embryonic development of a freshwater invertebrate model. Aquat Toxicol. (2017) 190:94–103. doi: 10.1016/j.aquatox.2017.06.029
26. Giusti A, Lagadic L, Barsi A, Thome JP, Joaquim-Justo C, Ducrot V. Investigating apical adverse effects of four endocrine active substances in the freshwater gastropod Lymnaea stagnalis. Sci Total Environ. (2014) 493:147–55. doi: 10.1016/j.scitotenv.2014.05.130
27. Giusti A, Leprince P, Mazzucchelli G, Thome JP, Lagadic L, Ducrot V, et al. Proteomic analysis of the reproductive organs of the hermaphroditic gastropod Lymnaea stagnalis exposed to different endocrine disrupting chemicals. PloS One. (2013) 8:e81086. doi: 10.1371/journal.pone.0081086
28. Svigruha R, Fodor I, Padisak J, Pirger Z. Progestogen-induced alterations and their ecological relevance in different embryonic and adult behaviours of an invertebrate model species, the great pond snail (Lymnaea stagnalis). Environ Sci pollut Res Int. (2021) 28:59391–402. doi: 10.1007/s11356-020-12094-z
29. Pirger Z, Urban P, Galik B, Kiss B, Tapodi A, Schmidt J, et al. Same same, but different: exploring the enigmatic role of the pituitary adenylate cyclase- activating polypeptide (PACAP) in invertebrate physiology. J Comp Physiol A Neuroethol Sens Neural Behav Physiol. (2024). doi: 10.1007/s00359-024-01706-5
30. Sadamoto H, Takahashi H, Okada T, Kenmoku H, Toyota M, Asakawa Y. De novo sequencing and transcriptome analysis of the central nervous system of mollusc Lymnaea stagnalis by deep RNA sequencing. PloS One. (2012) 7:e42546. doi: 10.1371/journal.pone.0042546
31. Koene JM, Jackson DJ, Nakadera Y, Cerveau N, Madoui MA, Noel B, et al. The genome of the simultaneously hermaphroditic snail Lymnaea stagnalis reveals an evolutionary expansion of FMRFamide-like receptors. Res Square Preperint. (2024). doi: 10.21203/rs.3.rs-3948809/v1
32. Lu S, Wang J, Chitsaz F, Derbyshire MK, Geer RC, Gonzales NR, et al. CDD/SPARCLE: the conserved domain database in 2020. Nucleic Acids Res. (2020) 48:D265–D8. doi: 10.1093/nar/gkz991
33. Marchler-Bauer A, Bo Y, Han L, He J, Lanczycki CJ, Lu S, et al. CDD/SPARCLE: functional classification of proteins via subfamily domain architectures. Nucleic Acids Res. (2017) 45:D200–D3. doi: 10.1093/nar/gkw1129
34. Kumar S, Stecher G, Tamura K. MEGA7: molecular evolutionary genetics analysis version 7.0 for bigger datasets. Mol Biol Evol. (2016) 33:1870–4. doi: 10.1093/molbev/msw054
35. Hallgren J, Tsirigos KD, Pedersen MD, Armenteros JJA, Marcatili P, Nielsen H, et al. DeepTMHMM predicts alpha and beta transmembrane proteins using deep neural networks. BioRxiv. (2022). doi: 10.1101/2022.04.08.487609
36. Nagasawa K, Matsubara S, Satake H, Osada M. Functional characterization of an invertebrate gonadotropin-releasing hormone receptor in the Yesso scallop Mizuhopecten yessoensis. Gen Comp Endocrinol. (2019) 282:113201. doi: 10.1016/j.ygcen.2019.06.005
37. Shiraishi A, Okuda T, Miyasaka N, Osugi T, Okuno Y, Inoue J, et al. Repertoires of G protein-coupled receptors for Ciona-specific neuropeptides. Proc Natl Acad Sci USA. (2019) 116:7847–56. doi: 10.1073/pnas.1816640116
38. Matsubara S, Shiraishi A, Sakai T, Okuda T, Satake H. Heterodimerization of the prostaglandin E2 receptor EP2 and the calcitonin receptor CTR. PloS One. (2017) 12:e0187711. doi: 10.1371/journal.pone.0187711
39. Kawada T, Ogasawara M, Sekiguchi T, Aoyama M, Hotta K, Oka K, et al. Peptidomic analysis of the central nervous system of the protochordate, Ciona intestinalis: homologs and prototypes of vertebrate peptides and novel peptides. Endocrinology. (2011) 152:2416–27. doi: 10.1210/en.2010-1348
40. Thomas P. Membrane androgen receptors unrelated to nuclear steroid receptors. Endocrinology. (2019) 160:772–81. doi: 10.1210/en.2018-00987
41. Milligan G, Marshall F, Rees S. G16 as a universal G protein adapter: implications for agonist screening strategies. Trends Pharmacol Sci. (1996) 17:235–7. doi: 10.1016/0165-6147(96)10026-2
42. Schwarz TI, Katsiadaki I, Maskrey BH, Scott AP. Mussels (Mytilus spp.) display an ability for rapid and high capacity uptake of the vertebrate steroid, estradiol-17beta from water. J Steroid Biochem Mol Biol. (2017) 165:407–20. doi: 10.1016/j.jsbmb.2016.08.007
43. Schwarz TI, Katsiadaki I, Maskrey BH, Scott AP. Rapid uptake, biotransformation, esterification and lack of depuration of testosterone and its metabolites by the common mussel, Mytilus spp. J Steroid Biochem Mol Biol. (2017) 171:54–65. doi: 10.1016/j.jsbmb.2017.02.016
44. Fodor I, Schwarz T, Kiss B, Tapodi A, Schmidt J, Cousins ARO, et al. Studies on a widely-recognized snail model species (Lymnaea stagnalis) provide further evidence that vertebrate steroids do not have a hormonal role in the reproduction of mollusks. Front Endocrinol. (2022) 13:981564. doi: 10.3389/fendo.2022.981564
45. Schwarz TI, Katsiadaki I, Maskrey BH, Scott AP. Uptake and metabolism of water-borne progesterone by the mussel, Mytilus spp. (Mollusca). J Steroid Biochem Mol Biol. (2018) 178:13–21. doi: 10.1016/j.jsbmb.2017.10.016
46. Katsiadaki I, Schwarz TI, Cousins ARO, Scott AP. The uptake of ethinyl-estradiol and cortisol from water by mussels (Mytilus spp.). Front Endocrinol. (2021) 12:794623. doi: 10.3389/fendo.2021.794623
47. Gooding MP, LeBlanc GA. Biotransformation and disposition of testosterone in the eastern mud snail Ilyanassa obsoleta. Gen Comp Endocrinol. (2001) 122:172–80. doi: 10.1006/gcen.2001.7630
48. Labadie P, Peck M, Minier C, Hill EM. Identification of the steroid fatty acid ester conjugates formed in vivo in Mytilus edulis as a result of exposure to estrogens. Steroids. (2007) 72:41–9. doi: 10.1016/j.steroids.2006.10.003
49. Puinean A-M, Labadie P, Hill EM, Osada M, Kishida M, Nakao R, et al. Laboratory exposure to 17b-estradiol fails to induce vitellogenin and estrogen receptor gene expression in the marine invertebrate Mytilus edulis. Aquat Toxicol. (2006) 79:376–83. doi: 10.1016/j.aquatox.2006.07.006
50. Janer G, Mesia-Vela S, Porte C, Kauffman FC. Esterification of vertebrate-type steroids in the Eastern oyster (Crassostrea virginica). Steroids. (2004) 69:129–36. doi: 10.1016/j.steroids.2003.12.002
51. Dimastrogiovanni G, Fernandes D, Bonastre M, Porte C. Progesterone is actively metabolized to 5alpha-pregnane-3,20-dione and 3beta-hydroxy-5alpha-pregnan-20-one by the marine mussel Mytilus galloprovincialis. Aquat Toxicol. (2015) 165:93–100. doi: 10.1016/j.aquatox.2015.05.018
52. Levin ER, Hammes SR. Nuclear receptors outside the nucleus: extranuclear signalling by steroid receptors. Nat Rev Mol Cell Biol. (2016) 17:783–97. doi: 10.1038/nrm.2016.122
53. Levin ER. Membrane estrogen receptors signal to determine transcription factor function. Steroids. (2018) 132:1–4. doi: 10.1016/j.steroids.2017.10.014
54. Fodor I, Koene JM, Pirger Z. Neuronal transcriptome analysis of a widely recognised molluscan model organism highlights the absence of key proteins involved in the de novo synthesis and receptor-mediation of sex steroids in vertebrates. Malacologia. (2021) 64:69–77. doi: 10.4002/040.064.0103
55. Hochberg GKA, Liu Y, Marklund EG, Metzger BPH, Laganowsky A, Thornton JW. A hydrophobic ratchet entrenches molecular complexes. Nature. (2020) 588:503–8. doi: 10.1038/s41586-020-3021-2
56. Gu W, Thitiphuree T, Otoki Y, Marquez EC, Kitano T, Itoh N, et al. Expression and functional analyses for estrogen receptor and estrogen related receptor of Yesso scallop, Patinopecten yessoensis. J Steroid Biochem Mol Biol. (2023) 231:106302. doi: 10.1016/j.jsbmb.2023.106302
57. Keay J, Bridgham JT, Thornton JW. The Octopus vulgaris estrogen receptor is a constitutive transcriptional activator: evolutionary and functional implications. Endocrinology. (2006) 147:3861–9. doi: 10.1210/en.2006-0363
58. Thornton JW, Need E, Crews D. Resurrecting the ancestral steroid receptor: ancient origin of estrogen signaling. Science. (2003) 301:1714–7. doi: 10.1126/science.1086185
59. Bannister R, Beresford N, Granger DW, Pounds NA, Rand-Weaver M, White R, et al. No substantial changes in estrogen receptor and estrogen-related receptor orthologue gene transcription in Marisa cornuarietis exposed to estrogenic chemicals. Aquat Toxicol. (2013) 140-141:19–26. doi: 10.1016/j.aquatox.2013.05.002
60. Markov GV, Laudet V. Origin and evolution of the ligand-binding ability of nuclear receptors. Mol Cell Endocrinol. (2011) 334:21–30. doi: 10.1016/j.mce.2010.10.017
61. Thomas P. Membrane progesterone receptors (mPRs, PAQRs): review of structural and signaling characteristics. Cells. (2022) 11:1785. doi: 10.3390/cells11111785
62. Filardo EJ, Thomas P. Minireview: G protein-coupled estrogen receptor-1, GPER-1: its mechanism of action and role in female reproductive cancer, renal and vascular physiology. Endocrinology. (2012) 153:2953–62. doi: 10.1210/en.2012-1061
63. Barton M, Filardo EJ, Lolait SJ, Thomas P, Maggiolini M, Prossnitz ER. Twenty years of the G protein-coupled estrogen receptor GPER: Historical and personal perspectives. J Steroid Biochem Mol Biol. (2018) 176:4–15. doi: 10.1016/j.jsbmb.2017.03.021
64. Zhang Y, Guerra LAY, Egertová M, Zampronio CG, Jones AM, Elphick MR. Molecular and functional characterization of somatostatin-type signalling in a deuterostome invertebrate. Open Biol. (2020) 10:200172. doi: 10.1098/rsob.200172
65. Kreienkamp HJ, Larusson HJ, Witte I, Roeder T, Birgül N, Hönck HH, et al. Functional annotation of two orphan G-protein-coupled receptors, Drostar1 and -2, from Drosophila melanogaster and their ligands by reverse pharmacology. J Biol Chem. (2002) 277:39937–43. doi: 10.1074/jbc.M206931200
66. Baker ME. Xenobiotics and the evolution of multicellular animals: Emergence and diversification of ligand-activated transcription factors'. Integr Comp Biol. (2005) 45:172–8. doi: 10.1093/icb/45.1.172
67. Thomas P, Pang Y, Dong J, Groenen P, Kelder J, de Vlieg J, et al. Steroid and G protein binding characteristics of the seatrout and human progestin membrane receptor alpha subtypes and their evolutionary origins. Endocrinology. (2007) 148:705–18. doi: 10.1210/en.2006-0974
68. Fujimoto K, Totani Y, Nakai J, Chikamoto N, Namiki K, Hatakeyama D, et al. Identification of putative molecules for adiponectin and adiponectin receptor and their roles in learning and memory in Lymnaea stagnalis. Biol (Basel). (2023) 12:375. doi: 10.3390/biology12030375
69. Thomas P, Converse A, Berg HA. ZIP9, a novel membrane androgen receptor and zinc transporter protein. Gen Comp Endocrinol. (2018) 257:130–6. doi: 10.1016/j.ygcen.2017.04.016
70. Taubenheim J, Kortmann C, Fraune S. Function and evolution of nuclear receptors in environmental-dependent postembryonic development. Front Cell Dev Biol. (2021) 9:653792. doi: 10.3389/fcell.2021.653792
71. Zhao XF. G protein-coupled receptors function as cell membrane receptors for the steroid hormone 20-hydroxyecdysone. Cell Commun Signal. (2020) 18:146. doi: 10.1186/s12964-020-00620-y
Keywords: progesterone, testosterone, estradiol, membrane receptor, mollusk, Lymnaea stagnalis
Citation: Fodor I, Matsubara S, Osugi T, Shiraishi A, Kawada T, Satake H and Pirger Z (2024) Lack of membrane sex steroid receptors for mediating rapid endocrine responses in molluscan nervous systems. Front. Endocrinol. 15:1458422. doi: 10.3389/fendo.2024.1458422
Received: 02 July 2024; Accepted: 30 July 2024;
Published: 12 August 2024.
Edited by:
Shogo Haraguchi, Showa University, JapanReviewed by:
Takashi Yazawa, Asahikawa Medical University, JapanGabriel V. Markov, UMR8227 Laboratoire de Biologie Intégrative des Modèles Marins, France
Copyright © 2024 Fodor, Matsubara, Osugi, Shiraishi, Kawada, Satake and Pirger. This is an open-access article distributed under the terms of the Creative Commons Attribution License (CC BY). The use, distribution or reproduction in other forums is permitted, provided the original author(s) and the copyright owner(s) are credited and that the original publication in this journal is cited, in accordance with accepted academic practice. No use, distribution or reproduction is permitted which does not comply with these terms.
*Correspondence: István Fodor, fodor.istvan@blki.hu
†These authors have contributed equally to this work