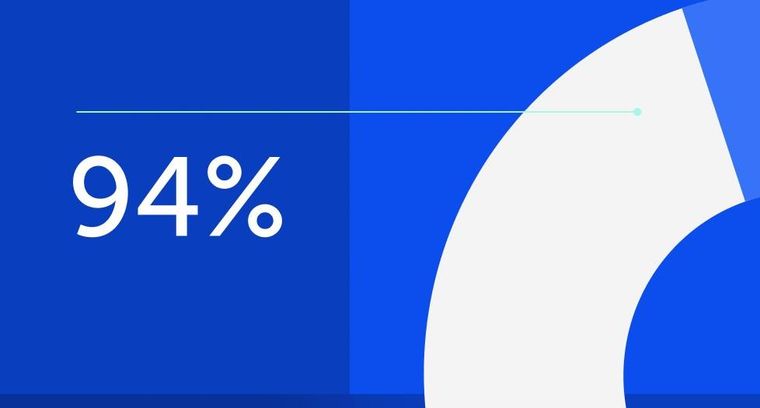
94% of researchers rate our articles as excellent or good
Learn more about the work of our research integrity team to safeguard the quality of each article we publish.
Find out more
REVIEW article
Front. Endocrinol., 03 January 2025
Sec. Renal Endocrinology
Volume 15 - 2024 | https://doi.org/10.3389/fendo.2024.1455783
Parathyroid hormone (PTH) is a pivotal hormone that regulates serum calcium and phosphate and is closely associated with higher risk of cardiovascular disease and mortality in patients with chronic kidney disease (CKD). PTH can undergo oxidation at methionine 8 and methionine 18 of the molecule. This oxidation process leads to a lower binding affinity to the PTH receptor due to molecular refolding, particularly for PTH oxidized at methionine 8. Although, the oxidation of PTH has been reported for several decades, it is only recently that a method has been developed to detect non-oxidized PTH (n-oxPTH) levels. The utilization of this assay enables the precise detection of n-oxPTH levels and facilitates the evaluation of their correlation with poor prognosis in patients with CKD. However, the current available clinical research findings indicate that n-oxPTH does not demonstrate clinical superiority over iPTH. Here, we provide a comprehensive review on the mechanism of PTH oxidation, the n-oxPTH assay method, and its correlation with iPTH and clinical outcomes.
Chronic kidney disease-mineral and bone disorder (CKD-MBD) is a common complication of CKD that involves dysregulated mineral and bone metabolism, abnormalities in bone structure, and vascular calcification. Parathyroid hormone (PTH), an 84-amino acid single-chain peptide, is synthesized by the parathyroid gland and plays a major role in the regulation of serum calcium and phosphate levels (1). Besides, PTH also serves as a significant risk factor for increased incidence of cardiovascular disease and overall mortality among CKD patients (2, 3). Therefore, the precise detection of PTH holds immense clinical significance.
Currently, the commonly employed methods for PTH detection in clinical practice are second- and third-generation immunoassays. The second-generation assays, referred to as iPTH assays, possess the capability to capture both full-length PTH and C-terminal PTH fragments present in the blood. On the contrary, the third-generation assay only detects the full-length PTH molecule, referred to as whole PTH assay or bioactive PTH assay.
The PTH hormone possesses two methionines, at position 8 and 18, which are susceptible to oxidation (4). This oxidative process generates three distinct oxidized PTH (oxPTH): ox (Met8) PTH, ox (Met18) PTH, and ox(Met8, 18) PTH (5). Studies have demonstrated that oxidation of PTH leading to its molecular structure change and influence its binding affinity to receptors, especially for ox (Met8) PTH (4, 6, 7). However, PTH oxidation has been neglected in the detection process for several decades. Recently, some researchers have developed a novel technique for determining non-oxidized PTH (n-oxPTH) by selectively removing oxPTH. With this emerging detection technology comes significant interest whether detection of n-oxPTH add more value in diagnosing and treating CKD. A review published in 2020 (8) examined the effects of oxidation on the biological activity of PTH, the mechanisms of PTH oxidation, and the clinical significance of n-oxPTH. However, several new articles have been published since that time, providing additional information regarding PTH oxidation and its measurement. We conducted a comprehensive review of the literature, emphasizing novel detection methods and the relationship between n-oxPTH and clinical outcomes in patients with CKD. In this review, we provided a comprehensive research progress on oxidation of PTH, encompassing the physiological role of PTH, the mechanism underlying PTH oxidation, the detection methodology for n-oxPTH, the relationship between n-oxPTH and iPTH, and the association between n-oxPTH and outcomes in patients with CKD.
PTH is a polypeptide consisting of 84 amino acids that is secreted by the chief cell of the parathyroid gland. The initial synthesis of PTH involves the production of pre-pro-PTH, a 115-amino acid polypeptide that undergoes further cleavage to generate pro-PTH consisting of 90 amino acids. Subsequently, this substance also undergoes intracellular cleavage and becomes an 84-amino acid polypeptide known as PTH, the biologically active form of the hormone (9). It has a molecular weight of approximately 9500 Dalton.
Hypocalcemia (10, 11), hyperphosphatemia (12), and decreased serum 1,25-dihydroxyvitamin D (1,25(OH)2D) (13, 14) can stimulate the secretion of PTH, while elevated serum calcium (15), 1,25(OH)2D or fibroblast growth factor 23 (FGF23) (16) can inhibit PTH secretion. The rapid secretion of PTH is primarily determined by the extracellular concentration of ionized calcium. After secretion, PTH is taken up by liver and kidney cells where it undergoes intracellular proteolysis to generate active amino-terminal (N-terminal) fragments and inactive carboxy-terminal (C-terminal) fragments. These two types of PTH fragments have distinct fates: the N-terminal fragment is rapidly degraded within liver and kidney cells, whereas the C-terminal fragment predominantly enters circulation and finally excreted by the kidney (17). The half-life of PTH is relatively short, lasting only about 2-5 minutes (18, 19).
PTH plays a crucial role in maintaining the homeostasis of calcium and phosphate levels, with its primary effects mediated through the activation of type 1 PTH receptors (PTH1R), which belong to the G protein-coupled receptor family (20, 21). The main effects of PTH on bone include promoting bone resorption and remodeling, mobilizing calcium from the bone into circulation, facilitating the release of calcium and phosphorus into the extracellular fluid, ultimately leading to an increase in serum calcium concentration (22, 23). In terms of renal effects, PTH promotes calcium reabsorption in renal tubules thereby enhances blood calcium levels (24, 25). Additionally, it reduces phosphate reabsorption in the renal tubules, leading to lower blood phosphorus levels (26). Furthermore, it increases the activity of renal tubular 1-α hydroxylase and promotes the synthesis of (1,25(OH)2D) (27, 28).
Methionine is easily to be oxidized and form methionine sulfoxide (29). The PTH molecules can undergo oxidation at the methionine residues 8 and 18 (Figure 1A), resulting in the generation of three distinct types of oxPTH: ox (Met8) PTH, ox (Met18) PTH, and ox(Met8, 18) PTH (8, 30, 31) (Figure 1B). The oxidation of PTH is frequently observed in patients with CKD or patients with maintenance hemodialysis, as these individuals are exposed to increased levels of oxidative stress.
Figure 1. Structure and oxidation products of parathyroid hormone (PTH). (A) Structure of PTH (1-84). (B) Types of oxidized PTH (oxPTH).
This oxidation process was found to induce structural modification, leading to subsequent alterations in the three-dimensional conformation of PTH (32, 33). Consequently, oxidation significantly impacts the interaction between PTH and its receptor (32). Biological activity of PTH could be assessed by quantifying the enzymatic activity of adenylyl cyclase, which catalyzes the conversion of adenosine triphosphate (ATP) to cyclic adenosine monophosphate (cAMP). Adenylyl cyclase plays a crucial role in PTH signal transduction, leading to an elevation in intracellular cAMP levels upon PTH stimulation (34). Numerous studies have demonstrated that oxPTH fails to induce cAMP production and consequently exhibits inadequate receptor activation compared to n-oxPTH (6, 35–42). This phenomenon can largely be attributed to the oxidation of Met8, rather than Met18 (6). Also, infusion of oxPTH in vivo did not induce any changes in serum calcium and urine phosphate concentrations, unlike unmodified PTH (4, 43). Daley et al. (6) reported a noteworthy finding that oxidation of Met8 in the long-acting analog of PTH caused little to no change in its functional interaction with the PTH receptor. This finding demonstrated that oxidation of Met8 in structurally distinct PTH ligands can have markedly different effects.
Additionally, various methodologies were employed by researchers to evaluate the biological activity of PTH, encompassing the assessment of renal and bone adenylate cyclase activity, renal excretion of calcium and phosphate, as well as mitochondrial ATPase activity. The findings demonstrated a hierarchical order in terms of PTH’s biological activity: n-oxPTH > ox (Met18) PTH > ox (Met8) PTH > ox(Met8, 18) PTH (7, 31, 44) (Figure 2). Furthermore, oxidation occurs more readily in ox(Met18)PTH compared to ox(Met8)PTH (7). The oxidation of Met(8) significantly reduces PTH binding to its receptor, while the oxidation of Met(18) has a limited influence on receptor binding (32, 45, 46). Notably, when both residues are oxidized, PTH exhibits the weakest affinity for the receptor. This result was aligning with observed biological activities.
First-generation PTH measurement kits employ an antibody targeting either the C-terminal or mid-terminal region of PTH (47), resulting in measurement of numerous biologically inactive C-terminal fragments; hence, this assay is no longer employed in clinical nowadays.
Second-generation assay utilizes two types of antibodies: one specifically targeting the N-terminal region of PTH (amino acids 1-34) and another designed to bind to the C-terminal of PTH (amino acids 39-84) (48). Consequently, this assay was designed to exclusively measure intact PTH molecules known as iPTH assay. This assay exhibits a substantial enhancement in both specificity and sensitivity. The iPTH results demonstrated greater consistency with the clinical presentation of patients; however, in certain patient populations such as those with severe secondary hyperparathyroidism, iPTH values were still overestimated (49–51). Due to the absence of N-terminal antibody targeting the first four amino acids, this method has been found to also detect C-terminal fragments, primarily the PTH fragment 7-84 (52).
The third-generation of PTH testing is referred to as whole PTH assay or bioactive PTH assay. This method incorporates an antibody targets C-terminal PTH, along with an antibody specifically targets the initial four amino acids of PTH (53). Consequently, this approach exclusively quantifies full-length PTH in circulation.
Both second- and third-generation PTH immunoassays represented a significant advancement toward the objective of measuring bioactive PTH. Though these methods are widely utilized in clinical settings, there are still some limitations of these assays. On one hand, the PTH testing lacks internationally recognized standards for traceability. Manufacturers use their own calibration standards leading to incomparable results, particularly for CKD patients (54–57). It is worth noting that new international traceability standards for PTH have already been established (IS 95/646), and they will be gradually implemented in the future for clinical detection of PTH. On the other hand, currently used PTH assays overlook the crucial post-translational modification process involving PTH oxidation.
Previous studies have revealed that the oxidation of PTH can significantly impact its biological activity, hence, it is imperative to detect n-oxPTH for accurate assessment of the real PTH level. To address this limitation, Hocher et al. (30) established a method for determining n-oxPTH recently. This novel test process comprises of two sequential steps. Initially, a specific affinity chromatography column is employed to eliminate any oxPTH including ox (Met8) PTH and/or ox (Met18) PTH from the sample using antibody targeting oxPTH. Subsequently, the remaining n-oxPTH is subjected to analysis by third-generation PTH assay system (Figure 3). It is worth noting that the n-oxPTH detection method eliminates all forms of oxPTH and does not differentiate between ox (Met8) PTH, ox (Met18) PTH, and ox (Met8, 18) PTH, which may have different biological activities. Liquid chromatographs coupled with tandem mass spectrometers (LC-MS/MS) have recently been employed for the detection of PTH (58, 59). This detection offers the advantage of exceptional sensitivity; however, it is accompanied by the drawback of complex sample preparation. Specifically, tryptic digestion and subsequent fragment quantification are required for large peptides like PTH. This process may lead to the loss of additional information, such as oxidation or newly formed fragments. Ideally, a selective and gentle technique is needed to differentiate PTH and its derivatives in their native state.
With the development of the n-oxPTH assay by Hocher et al., the detection of bioactive non-oxidizing PTH levels in vivo has been facilitated, leading to an increased interest in the clinical significance of n-oxPTH measurement. In the initial clinical research article conducted by Hocher’s team (30), they examined the levels of n-oxPTH and iPTH in 18 dialysis patients and demonstrated that only 7-34% traditionally measured PTH were real intact PTH. Subsequently, they evaluated n-oxPTH concentrations in a larger cohort (32) consisting of 620 children with CKD stages 2-4, 342 adult patients on dialysis, 602 kidney transplant recipients, and 89 healthy controls. The results indicated a significant correlation between n-oxPTH and age, while no significant association was found with sex. The level of n-oxPTH were 1.5-2.25 times increased in patients with renal failure compared with controls. Zuo et al. (60) assessed the relationship of n-oxPTH and iPTH in 531 stable kidney transplant recipients and found that the association between iPTH and oxPTH was stronger than the association between iPTH and n-oxPTH. The findings of this study indicate that over 85% of iPTH underwent oxidation. Cavalier et al. (61) examined the levels of iPTH and n-oxPTH in 66 stable hemodialysis patients at baseline and after 1, 3, 6, and 12 months. The findings demonstrated that the n-oxPTH fraction consistently accounted for 11.3% (9.0-13.7%) of iPTH throughout the study duration, indicating a stable proportion of n-oxPTH in stable hemodialysis patients. All of these results suggest that the measurement of n-oxPTH may provide a more accurate reflection of the hormonal status, as opposed to iPTH which solely indicates oxidative stress in patients with renal failure.
However, contrary findings have been reported in some studies. Kritmetapak et al. (62) developed a liquid chromatography-high resolution mass spectrometry (LC-HRMS) method for the measurement of PTH in 221 patients with stages 1-5 CKD. Their results showed that LC-HRMS could identify the existance of PTH1-84, C-terminal PTH fragments, and mid-region PTH fragments in blood of patients with CKD. The levels of both PTH1-84 and PTH fragments were elevated in patients with decreased renal function. Furthermore, it was noted that concentrations of PTH measured using LC-HRMS tended to be lower than those obtained through iPTH detection. Importantly, the results from this study did not support the presence of PTH7-84 or oxPTH in blood. Thus, whether n-oxPTH should be detected in clinical diagnosis and treatment is still controversial.
Zeng et al. (63) evaluated the relationship between n-oxPTH levels and FGF23 concentrations in two distinct cohorts of patients, including 620 children with CKD (eGFR 10-60 ml/min/1.73m2) and 600 kidney transplant patients. Their findings revealed a significant association between n-oxPTH and FGF23 concentrations, while no such relationship was observed for oxPTH. Furthermore, as kidney function declined progressively, both iPTH and oxPTH exhibited substantial increases, whereas n-oxPTH showed only moderate elevation. This study revealed that n-oxPTH may be related to mineral metabolism in patients with CKD. However, Ursem et al. (64) compared the efficacy of n-oxPTH and iPTH as markers for assessing bone turnover in 31 patients with end stage kidney disease (ESKD) and found that both iPTH and n-oxPTH were strongly correlated with histomorphometric and circulating parameters of bone turnover. Consequently, at present, measuring n-oxPTH does not offer any additional value compared with iPTH when evaluating bone turnover in patients with renal failure.
The correlation between n-oxPTH and prognosis has been examined in several clinical studies, as summarized in Table 1. However, the predictive value of n-oxPTH for poor prognosis in CKD patients remains a subject of controversy. Lu et al. (65) detected circulating PTH concentrations in 600 kidney transplant patients. Then these patients were followed-up for graft loss for 3 years. Their results demonstrated a significant association between graft loss and n-oxPTH, while no significant associations were observed with oxPTH or iPTH. In another study, Seiler-Mussler et al. (66) measured PTH levels in 535 patients with CKD (eGFR 10-60 ml/min/1.73m2) and followed them for five years to assess the risk of poor prognosis. The findings demonstrated that there was no significant association between n-oxPTH and clinical outcomes in non-dialysis CKD patients, including atherosclerotic events, acute heart failure, CKD progression, and all-cause mortality. The correlation between n-oxPTH and risk of death in 340 hemodialysis patients was analyzed over a 5-year follow-up period (67). The results revealed that patients with elevated n-oxPTH levels exhibited a higher survival rate in comparison to the group with lower n-oxPTH levels. However, the mortality in a subset of patients with iPTH concentrations exceeding the upper limit of the iPTH assay (70 ng/L) was found to be associated with oxPTH concentrations, rather than n-oxPTH concentrations. Hence, the controversy persists regarding whether measures of iPTH primarily reflect the oxidative stress status in CKD patients rather than their PTH hormone levels. The potential of n-oxPTH detection to enhance clinical diagnosis, treatment, and prognosis analysis necessitates further exploration by more RCT studies.
Currently, apart from the utilization of human n-oxPTH monoclonal antibody for the elimination of oxPTH from blood, there is no superior approach available for quantifying n-oxPTH levels. Furthermore, different instruments yield disparate quantitative outcomes for PTH levels. Existing PTH immunoassays also employ inconsistent standards. It should be noted that the widespread implementation of n-oxPTH measurements has been impeded due to the aforementioned limits. Measuring n-oxPTH levels may provide a more accurate and real PTH status in patients with CKD, nevertheless, current evidence does not support the clinical value of measuring n-oxPTH.
PTH is an important biomarker need to be monitored regularly in patients with CKD. At present, detection of PTH primarily relies on second- or third-generation immunoassays. The oxidation of PTH in vivo can result in a loss of its biological activity. Recently, researchers have made significant advancements in the detection of n-oxPTH in blood. It is important to note that this assay does not differentiate different oxidation forms of PTH, whereas ox (Met8) PTH, ox (Met18) PTH, and ox (Met8,18) PTH exhibit different biological activities. Therefore, further optimization of bioactive PTH measurement methods is still required to determine if current techniques excessively exclude all forms of oxPTH.
Many clinical studies have revealed the correlation between all-cause mortality, cardiovascular events and iPTH levels. Furthermore, it has been reported that iPTH exhibits a robust correlation with oxPTH and a relatively weak correlation with n-oxPTH. Therefore, accurate assessment of n-oxPTH may hold greater value in determining the correlation between PTH and patient prognosis. Several clinical studies analyzed the correlation between n-oxPTH and outcomes, results showed that measuring n-oxPTH does not yield any additional clinical value. The suboptimal outcome may be attributed to the fact that current n-oxPTH tests fail to differentiate between different forms of oxPTH. It is imperative to develop novel PTH detection methods capable of discerning different oxidation states, including n-oxPTH, ox (Met8) PTH, ox (Met18) PTH, and ox (Met8, 18) PTH. Such an innovative test has the potential to bring more clinical significance. Until a more refined assay is devised, it is advisable to continue employing second- or third-generation PTH tests currently utilized in clinical practice.
LZ: Conceptualization, Investigation, Visualization, Writing – original draft, Data curation. HC: Conceptualization, Funding acquisition, Writing – review & editing.
The author(s) declare financial support was received for the research, authorship, and/or publication of this article. This study was funded by the Natural Science Foundation of Henan Province (202300410401) and Medical Science and Technology Research Project of Henan Province (LHGJ20190615).
The authors declare that the research was conducted in the absence of any commercial or financial relationships that could be construed as a potential conflict of interest.
All claims expressed in this article are solely those of the authors and do not necessarily represent those of their affiliated organizations, or those of the publisher, the editors and the reviewers. Any product that may be evaluated in this article, or claim that may be made by its manufacturer, is not guaranteed or endorsed by the publisher.
1. Evenepoel P, Bover J, Ureña Torres P. Parathyroid hormone metabolism and signaling in health and chronic kidney disease. Kidney Int. (2016) 90:1184–90. doi: 10.1016/j.kint.2016.06.041
2. Block GA, Kilpatrick RD, Lowe KA, Wang W, Danese MD. CKD-mineral and bone disorder and risk of death and cardiovascular hospitalization in patients on hemodialysis. Clin J Am Soc Nephrol CJASN. (2013) 8:2132–40. doi: 10.2215/CJN.04260413
3. Lee SA, Lee MJ, Ryu GW, Jhee JH, Kim HW, Park S, et al. Low serum intact parathyroid hormone level is an independent risk factor for overall mortality and major adverse cardiac and cerebrovascular events in incident dialysis patients. Osteoporos Int J Establ Result Coop Eur Found Osteoporos Natl Osteoporos Found USA. (2016) 27:2717–26. doi: 10.1007/s00198-016-3636-1
4. Horiuchi N. Effects of oxidation of human parathyroid hormone on its biological activity in continuously infused, thyroparathyroidectomized rats. J Bone Miner Res Off J Am Soc Bone Miner Res. (1988) 3:353–8. doi: 10.1002/jbmr.5650030316
5. Chu JW, Yin J, Wang DIC, Trout BL. A structural and mechanistic study of the oxidation of methionine residues in hPTH(1-34) via experiments and simulations. Biochemistry. (2004) 43:14139–48. doi: 10.1021/bi049151v
6. Daley EJ, Khatri A, Dean T, Vilardaga J-P, Zaidi SA, Katritch V, et al. Ligand-dependent effects of methionine-8 oxidation in parathyroid hormone peptide analogues. Endocrinology. (2021) 162:bqaa216. doi: 10.1210/endocr/bqaa216
7. Frelinger AL, Zull JE. The role of the methionine residues in the structure and function of parathyroid hormone. Arch Biochem Biophys. (1986) 244:641–9. doi: 10.1016/0003-9861(86)90632-6
8. Ursem SR, Vervloet MG, de Jongh RT, Heijboer AC. Oxidation of parathyroid hormone. Clin Chim Acta Int J Clin Chem. (2020) 506:84–91. doi: 10.1016/j.cca.2020.03.020
9. Habener JF, Rosenblatt M, Potts JT. Parathyroid hormone: biochemical aspects of biosynthesis, secretion, action, and metabolism. Physiol Rev. (1984) 64:985–1053. doi: 10.1152/physrev.1984.64.3.985
10. Maye GP, Keaton JA, Hurst JG, Habener JF. Effects of plasma calcium concentration on the relative proportion of hormone and carboxyl fragments in parathyroid venous blood*. Endocrinology. (1979) 104:1778–84. doi: 10.1210/endo-104-6-1778
11. D’Amour P, Palardy J, Bahsali G, Mallette LE, DeLéan A, Lepage R. The modulation of circulating parathyroid hormone immunoheterogeneity in man by ionized calcium concentration. J Clin Endocrinol Metab. (1992) 74:525–32. doi: 10.1210/jcem.74.3.1740486
12. Nakajima K, Umino K-I, Azuma Y, Kosaka S, Takano K, Obara T, et al. Stimulating parathyroid cell proliferation and PTH release with phosphate in organ cultures obtained from patients with primary and secondary hyperparathyroidism for a prolonged period. J Bone Miner Metab. (2009) 27:224–33. doi: 10.1007/s00774-008-0032-8
13. Kremer R, Bolivar I, Goltzman D, Hendy GN. Influence of calcium and 1,25-dihydroxycholecalciferol on proliferation and proto-oncogene expression in primary cultures of bovine parathyroid cells*. Endocrinology. (1989) 125:935–41. doi: 10.1210/endo-125-2-935
14. Russell J, Lettieri D, Sherwood LM. Suppression by 1,25(OH)2D3 of transcription of the pre-proparathyroid hormone gene. Endocrinology. (1986) 119:2864–6. doi: 10.1210/endo-119-6-2864
15. Kilav R, Silver J, Naveh-Many T. A conserved cis-acting element in the parathyroid hormone 3′-untranslated region is sufficient for regulation of RNA stability by calcium and phosphate. J Biol Chem. (2001) 276:8727–33. doi: 10.1074/jbc.M005471200
16. Ben-Dov IZ, Galitzer H, Lavi-Moshayoff V, Goetz R, Kuro-o M, Mohammadi M, et al. The parathyroid is a target organ for FGF23 in rats. J Clin Invest. (2007) 117:4003–8. doi: 10.1172/JCI32409
17. Bringhurst FR, Stern AM, Yotts M, Mizrahi N, Segre GV, Potts JT. Peripheral metabolism of PTH: fate of biologically active amino terminus. vivo Am J Physiol-Endocrinol Metab. (1988) 255:E886–93. doi: 10.1152/ajpendo.1988.255.6.E886
18. Yamashita H, Gao P, Cantor T, Futata T, Murakami T, Uchino S, et al. Large carboxy-terminal parathyroid hormone (PTH) fragment with a relatively longer half-life than 1-84 PTH is secreted directly from the parathyroid gland in humans. Eur J Endocrinol. (2003) 149:301–6. doi: 10.1530/eje.0.1490301
19. Bieglmayer C, Kaczirek K, Prager G, Niederle B. Parathyroid hormone monitoring during total parathyroidectomy for renal hyperparathyroidism: pilot study of the impact of renal function and assay specificity. Clin Chem. (2006) 52:1112–9. doi: 10.1373/clinchem.2005.065490
20. Murray TM, Rao LG, Divieti P, Bringhurst FR. Parathyroid hormone secretion and action: evidence for discrete receptors for the carboxyl-terminal region and related biological actions of carboxyl-terminal ligands. Endocr Rev. (2005) 26:78–113. doi: 10.1210/er.2003-0024
21. Jüppner H, Abou-Samra AB, Freeman M, Kong XF, Schipani E, Richards J, et al. A G protein-linked receptor for parathyroid hormone and parathyroid hormone-related peptide. Science. (1991) 254:1024–6. doi: 10.1126/science.1658941
22. Rouleau MF, Mitchell J, Goltzman D. Characterization of the major parathyroid hormone target cell in the endosteal metaphysis of rat long bones. J Bone Miner Res. (2020) 5:1043–53. doi: 10.1002/jbmr.5650051008
23. Li X, Liu H, Qin L, Tamasi J, Bergenstock M, Shapses S, et al. Determination of dual effects of parathyroid hormone on skeletal gene expression in vivo by microarray and network analysis. J Biol Chem. (2007) 282:33086–97. doi: 10.1074/jbc.M705194200
24. Toka HR, Al-Romaih K, Koshy JM, DiBartolo S, Kos CH, Quinn SJ, et al. Deficiency of the calcium-sensing receptor in the kidney causes parathyroid hormone–independent hypocalciuria. J Am Soc Nephrol. (2012) 23:1879–90. doi: 10.1681/ASN.2012030323
25. Loupy A, Ramakrishnan SK, Wootla B, Chambrey R, De La Faille R, Bourgeois S, et al. PTH-independent regulation of blood calcium concentration by the calcium-sensing receptor. J Clin Invest. (2012) 122:3355–67. doi: 10.1172/JCI57407
26. Segawa H, Yamanaka S, Onitsuka A, Tomoe Y, Kuwahata M, Ito M, et al. Parathyroid hormone-dependent endocytosis of renal type IIc Na-P i cotransporter. Am J Physiol-Ren Physiol. (2007) 292:F395–403. doi: 10.1152/ajprenal.00100.2006
27. Klaus G, May T, Hügel U, von Eichel B, Rodriguez J, Fernandez P, et al. Parathyroid hormone prevents 1,25 (OH)2D3 induced down-regulation of the vitamin D receptor in growth plate chondrocytes in vitro. Kidney Int. (1997) 52:45–51. doi: 10.1038/ki.1997.302
28. Shigematsu T, Horiuchi N, Ogura Y, Miyahara T, Suda T. Human parathyroid hormone inhibits renal 24-hydroxylase activity of 25-hydroxyvitamin D3 by a mechanism involving adenosine 3’,5’-monophosphate in rats. Endocrinology. (1986) 118:1583–9. doi: 10.1210/endo-118-4-1583
29. Vogt W. Oxidation of methionyl residues in proteins: Tools, targets, and reversal. Free Radic Biol Med. (1995) 18:93–105. doi: 10.1016/0891-5849(94)00158-G
30. Hocher B, Armbruster FP, Stoeva S, Reichetzeder C, Grön HJ, Lieker I, et al. Measuring parathyroid hormone (PTH) in patients with oxidative stress–do we need a fourth generation parathyroid hormone assay? PloS One. (2012) 7:e40242. doi: 10.1371/journal.pone.0040242
31. Frelinger AL, Zull JE. Oxidized forms of parathyroid hormone with biological activity. Separation and characterization of hormone forms oxidized at methionine 8 and methionine 18. J Biol Chem. (1984) 259:5507–13. doi: 10.1016/S0021-9258(18)91041-0
32. Hocher B, Oberthür D, Slowinski T, Querfeld U, Schaefer F, Doyon A, et al. Modeling of oxidized PTH (oxPTH) and non-oxidized PTH (n-oxPTH) receptor binding and relationship of oxidized to non-oxidized PTH in children with chronic renal failure, adult patients on hemodialysis and kidney transplant recipients. Kidney Blood Press Res. (2013) 37:240–51. doi: 10.1159/000350149
33. Zull JE, Smith SK, Wiltshire R. Effect of methionine oxidation and deletion of amino-terminal residues on the conformation of parathyroid hormone. Circular dichroism studies. J Biol Chem. (1990) 265:5671–6. doi: 10.1016/S0021-9258(19)39415-3
34. Chase LR, Aurbach GD. Renal adenyl cyclase: anatomically separate sites for parathyroid hormone and vasopressin. Science. (1968) 159:545–7. doi: 10.1126/science.159.3814.545
35. Sham JSK, Kenny AD, Pang PKT. Cardiac actions and structural-activity relationship of parathyroid hormone on isolated frog atrium. Gen Comp Endocrinol. (1984) 55:373–7. doi: 10.1016/0016-6480(84)90006-6
36. Zanelli JM, O’Hare MJ, Nice EC, Corran PH. Purification and assay of bovine parathyroid hormone by reversed-phase high-performance liquid chromatography. J Chromatogr B BioMed Sci App. (1981) 223:59–67. doi: 10.1016/S0378-4347(00)80068-7
37. Pang PKT, Yang MCM, Kenny AD, Tenner TE. Structure and vascular activity relationship of parathyroid hormone and some hypotensive peptides. Clin Exp Hypertens A. (1982) 4:189–99. doi: 10.3109/10641968209061584
38. Neuman WF, Neuman MW, Lane K, Miller L, Sammon PJ. The metabolism of labeled parathyroid hormone: V. Collected biological studies. Calcif Tissue Res. (1975) 18:271–87. doi: 10.1007/BF02546246
39. O’Riordan JLH, Woodhead JS, Hendy GN, Parsons JA, Robinson CJ, Keutmann HT, et al. Effect of oxidation on biological and immunological activity of porcine parathyroid hormone. J Endocrinol. (1974) 63:117–24. doi: 10.1677/joe.0.0630117
40. Goltzmann D, Peytremann A, Callahan E, Tregear G, Potts J. Analysis of the requirements for parathyroid hormone action in renal membranes with the use of inhibiting analogues. J Biol Chem. (1975) 250:3199–203. doi: 10.1016/S0021-9258(19)41611-6
41. Chu LLH, Forte LR, Anast CS, Cohn DV. Interaction of parathyroid hormone with membranes of kidney cortex: degradation of the hormone and activation of adenylate cyclase 1. Endocrinology. (1975) 97:1014–23. doi: 10.1210/endo-97-4-1014
42. Demay M, Mitchell J, Goltzman D. Comparison of renal and osseous binding of parathyroid hormone and hormonal fragments. Am J Physiol. (1985) 249:E437–446. doi: 10.1152/ajpendo.1985.249.5.E437
43. Galceran T, Lewis-Finch J, Martin KJ, Slatopolsky E. Absence of biological effects of oxidized parathyroid hormone-(1–3 4) in dogs and rats*. Endocrinology. (1984) 115:2375–8. doi: 10.1210/endo-115-6-2375
44. Laethem R, Zull JE. Characterization of the interaction of parathyroid hormone with the mitochondrial ATPase. Arch Biochem Biophys. (1990) 282:161–9. doi: 10.1016/0003-9861(90)90100-d
45. Sutcliffe HS, Martin TJ, Eisman JA, Pilczyk R. Binding of parathyroid hormone to bovine kidney-cortex plasma membranes. Biochem J. (1973) 134:913–21. doi: 10.1042/bj1340913
46. Nabuchi Y, Fujiwara E, Ueno K, Kuboniwa H, Asoh Y, Ushio H. Oxidation of recombinant human parathyroid hormone: effect of oxidized position on the biological activity. Pharm Res. (1995) 12:2049–52. doi: 10.1023/a:1016281031373
47. Berson SA, Yalow RS, Aurbach GD, Potts JT. Immunoassay of bovine and human parathyroid hormone. Proc Natl Acad Sci. (1963) 49:613–7. doi: 10.1073/pnas.49.5.613
48. Nussbaum SR, Zahradnik RJ, Lavigne JR, Brennan GL, Nozawa-Ung K, Kim LY, et al. Highly sensitive two-site immunoradiometric assay of parathyrin, and its clinical utility in evaluating patients with hypercalcemia. Clin Chem. (1987) 33:1364–7. doi: 10.1093/clinchem/33.8.1364
49. Kritmetapak K, Pongchaiyakul C. Parathyroid hormone measurement in chronic kidney disease: from basics to clinical implications. Int J Nephrol. (2019) 2019:5496710. doi: 10.1155/2019/5496710
50. Soliman M, Hassan W, Yaseen M, Rao M, Sawaya BP, El-Husseini A. PTH assays in dialysis patients: Practical considerations. Semin Dial. (2019) 32:9–14. doi: 10.1111/sdi.12743
51. Hocher B, Yin L. Why current PTH assays mislead clinical decision making in patients with secondary hyperparathyroidism. Nephron. (2017) 136:137–42. doi: 10.1159/000455289
52. Lepage R, Roy L, Brossard JH, Rousseau L, Dorais C, Lazure C, et al. A non-(1–84) circulating parathyroid hormone (PTH) fragment interferes significantly with intact PTH commercial assay measurements in uremic samples. Clin Chem. (1998) 44:805–9. doi: 10.1093/clinchem/44.4.805
53. John MR, Goodman WG, Gao P, Cantor TL, Salusky IB, Jüppner H. A Novel Immunoradiometric Assay Detects Full-Length Human PTH but not Amino-Terminally Truncated Fragments: Implications for PTH Measurements in Renal Failure. J Clin Endocrinol Metab. (1999) 84:4287–90. doi: 10.1210/jcem.84.11.6236
54. Sturgeon CM, Sprague SM, Metcalfe W. Variation in parathyroid hormone immunoassay results–a critical governance issue in the management of chronic kidney disease. Nephrol Dial Transplant Off Publ Eur Dial Transpl Assoc - Eur Ren Assoc. (2011) 26:3440–5. doi: 10.1093/ndt/gfr614
55. Cavalier E, Souberbielle JC, Delanaye P. PTH determination in hemodialyzed patients-A laboratory perspective. Semin Dial. (2019) 32:490–2. doi: 10.1111/sdi.12844
56. Cavalier E, Delanaye P, Vranken L, Bekaert A-C, Carlisi A, Chapelle J-P, et al. Interpretation of serum PTH concentrations with different kits in dialysis patients according to the KDIGO guidelines: importance of the reference (normal) values. Nephrol Dial Transplant. (2012) 27:1950–6. doi: 10.1093/ndt/gfr535
57. Almond A, Ellis AR, Walker SW. Current parathyroid hormone immunoassays do not adequately meet the needs of patients with chronic kidney disease. Ann Clin Biochem Int J Lab Med. (2012) 49:63–7. doi: 10.1258/acb.2011.011094
58. Lopez MF, Rezai T, Sarracino DA, Prakash A, Krastins B, Athanas M, et al. Selected reaction monitoring–mass spectrometric immunoassay responsive to parathyroid hormone and related variants. Clin Chem. (2010) 56:281–90. doi: 10.1373/clinchem.2009.137323
59. Kumar V, Barnidge DR, Chen LS, Twentyman JM, Cradic KW, Grebe SK, et al. Quantification of serum 1–84 parathyroid hormone in patients with hyperparathyroidism by immunocapture in situ digestion liquid chromatography–tandem mass spectrometry. Clin Chem. (2010) 56:306–13. doi: 10.1373/clinchem.2009.134643
60. Zuo J, Hasan AA, Hocher CF, Kalk P, Kleuser B, Krämer BK, et al. Inverse correlation of intact PTH, oxidized PTH as well as non-oxidized PTH with 25-hydroxyvitamin D3 in kidney transplant recipients. Front Endocrinol. (2023) 14:1178166. doi: 10.3389/fendo.2023.1178166
61. Cavalier E, Lukas P, Warling X, Moonen M, Smelten N, Delanaye P. The percentage of non-oxidized PTH concentration remains stable over a period of 1 year in hemodialyzed patients. Clin Chim Acta Int J Clin Chem. (2020) 506:107–9. doi: 10.1016/j.cca.2020.03.025
62. Kritmetapak K, Losbanos LA, Hines JM, O’Grady KL, Ulmer CZ, Vesper HW, et al. Chemical characterization and quantification of circulating intact PTH and PTH fragments by high-resolution mass spectrometry in chronic renal failure. Clin Chem. (2021) 67:843–53. doi: 10.1093/clinchem/hvab013
63. Zeng S, Querfeld U, Feger M, Haffner D, Hasan AA, Chu C, et al. Relationship between GFR intact PTH, oxidized PTH, non-oxidized PTH as well as FGF23 in patients with CKD. FASEB J Off Publ Fed Am Soc Exp Biol. (2020) 34:15269–81. doi: 10.1096/fj.202000596R
64. Ursem SR, Heijboer AC, D’Haese PC, Behets GJ, Cavalier E, Vervloet MG, et al. Non-oxidized parathyroid hormone (PTH) measured by current method is not superior to total PTH in assessing bone turnover in chronic kidney disease. Kidney Int. (2021) 99:1173–8. doi: 10.1016/j.kint.2020.12.024
65. Lu YP, Zeng S, Chu C, Hasan AA, Slowinski T, Yin L-H, et al. Non-oxidized PTH (n-oxPTH) is associated with graft loss in kidney transplant recipients. Clin Chim Acta Int J Clin Chem. (2020) 508:92–7. doi: 10.1016/j.cca.2020.05.022
66. Seiler-Mussler S, Limbach AS, Emrich IE, Pickering JW, Roth HJ, Fliser D, et al. Association of nonoxidized parathyroid hormone with cardiovascular and kidney disease outcomes in chronic kidney disease. Clin J Am Soc Nephrol CJASN. (2018) 13:569–76. doi: 10.2215/CJN.06620617
67. Tepel M, Armbruster FP, Grön HJ, Scholze A, Reichetzeder C, Roth HJ, et al. Nonoxidized, biologically active parathyroid hormone determines mortality in hemodialysis patients. J Clin Endocrinol Metab. (2013) 98:4744–51. doi: 10.1210/jc.2013-2139
68. Ursem S, Francic V, Keppel M, Schwetz V, Trummer C, Pandis M, et al. The effect of vitamin D supplementation on plasma non-oxidised PTH in a randomised clinical trial. Endocr Connect. (2019) 8:518–27. doi: 10.1530/EC-19-0097
Keywords: oxidized, parathyroid hormone, chronic kidney disease, prognosis, immunoassay
Citation: Zhang L and Cao H (2025) Unlocking the mysteries of n-oxPTH: implications for CKD patients. Front. Endocrinol. 15:1455783. doi: 10.3389/fendo.2024.1455783
Received: 27 June 2024; Accepted: 11 December 2024;
Published: 03 January 2025.
Edited by:
Nehal Mohsen Elsherbiny, Mansoura University, EgyptReviewed by:
Kunihiro Sakuma, Tokyo Institute of Technology, JapanCopyright © 2025 Zhang and Cao. This is an open-access article distributed under the terms of the Creative Commons Attribution License (CC BY). The use, distribution or reproduction in other forums is permitted, provided the original author(s) and the copyright owner(s) are credited and that the original publication in this journal is cited, in accordance with accepted academic practice. No use, distribution or reproduction is permitted which does not comply with these terms.
*Correspondence: Huixia Cao, aHVpeGlhY2FvMzEwQDEyNi5jb20=
Disclaimer: All claims expressed in this article are solely those of the authors and do not necessarily represent those of their affiliated organizations, or those of the publisher, the editors and the reviewers. Any product that may be evaluated in this article or claim that may be made by its manufacturer is not guaranteed or endorsed by the publisher.
Research integrity at Frontiers
Learn more about the work of our research integrity team to safeguard the quality of each article we publish.