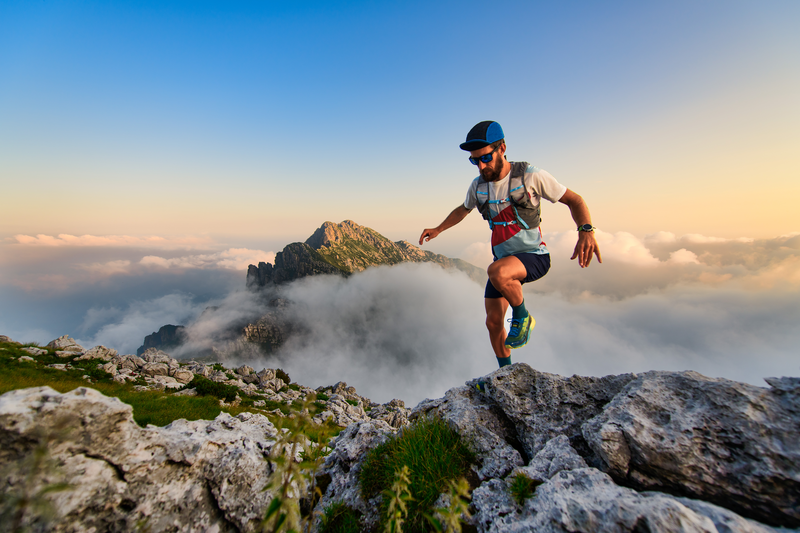
95% of researchers rate our articles as excellent or good
Learn more about the work of our research integrity team to safeguard the quality of each article we publish.
Find out more
ORIGINAL RESEARCH article
Front. Endocrinol. , 18 September 2024
Sec. Reproduction
Volume 15 - 2024 | https://doi.org/10.3389/fendo.2024.1440182
This article is part of the Research Topic Genetics and Epigenetics in Ovarian Aging View all 6 articles
Objective: This study aimed to evaluate the effectiveness of oral L-carnitine administration in patients after treatment failure to lay the groundwork for targeted in vivo use.
Methods and materials: A total of 515 In Vitro Fertilization (IVF) patients undergoing subsequent cycles were included after applying exclusion criteria. They were divided into a control group of 362 patients and a study group of 153 patients who received oral L-carnitine until oocyte retrieval.140 patients were matched according to maternal age, infertility duration, body mass index (BMI), day three top-quality embryos rate, by propensity score matching (PSM). The study investigated the relationship between L-carnitine treatment and in vivo oocyte maturation, normal fertilization, and subsequent embryo development.
Results: Following PSM, initial differences in BMI and Day3 top-quality embryo rate between groups were nullified, we created two comparable cohorts with highly similar characteristics. In the subsequent cycles, the study group showed significant improvements in in vivo oocyte maturation rate at retrieval (p=0.002), normal in vitro fertilization rate (p=0.003), blastocyst formation rate (p=0.003), and usable blastocyst rate compared to controls. Although there was no significant difference in the top-quality embryo rate on Day 3, the study group showed a 10% increase in the upper quartile (55.35% vs. 66.67%). The cumulative clinical pregnancy and live birth rates showed a significant improvement (59.82% vs. 68.42%,p=0.004, 47.41% vs. 59.80%, p=0.002). Furthermore, self-control analysis revealed substantial enhancements (p<0.001) in all outcome measures following L-carnitine administration, resulting in the birth of 74 healthy neonates without congenital anomalies.
Conclusion: We theorized that daily oral intake of L-carnitine before oocyte retrieval could boost oocyte quality and embryonic development, thus improving IVF outcomes. Ongoing investigations hold the potential to offer valuable insights into the applications and mechanisms underlying the therapeutic effectiveness of L-carnitine.
Achieving fertility and ensuring the birth of healthy offspring relies on a delicate balance of various physiological processes, including endocrine, cellular, and molecular mechanisms, all of which are heavily influenced by maternal health. Metabolic disorders have emerged as significant disruptors of reproductive physiology, frequently resulting in subfertility (1). The global prevalence of these metabolic health disorders is rapidly increasing and has been solidly linked to the issue of subfertility, as noted by the World Health Organization, the United Nations Population Fund, and the Practice Committee of the American Society for Reproductive Medicine (2, 3). Reproduction, being a multifaceted process rooted in folliculogenesis, intricately depends on maternal well-being and involves complex interactions among various physiological factors, including endocrine signaling, cellular processes, and molecular pathways (4, 5). Maternal metabolic disorders can induce lipotoxic effects on developing oocytes, as shown by elevated serum non-esterified fatty acid concentrations in follicular and oviductal fluids, ultimately affecting oocyte and embryo quality (6, 7).
Mitochondria, crucial regulators of cellular metabolism, play a central role in ensuring oocyte developmental competence. Dysfunctional mitochondria under metabolic stress conditions are increasingly associated with reduced oocyte quality (8). High-fat microenvironments have been found to induce mitochondrial dysfunction in both animal models and human studies (9, 10).
L-carnitine, the sole molecule capable of transporting fatty acids into mitochondria, facilitates long-chain fatty acid transport for β-oxidation and energy production. Acting as a mitochondrial modulator, it shows promise in mitigating lipotoxic stress in oocytes by promoting fatty acid uptake and β-oxidation (11–13). However, achieving therapeutic plasma levels of L-carnitine through oral administration poses challenges due to its poor absorption and bioavailability, necessitating efficient supplementation strategies (14). In contrast to reports showing L-carnitine treatment improving embryo development in vitro, little is known about the effects of circulating L-carnitine or in vivo L-carnitine supplementation on oocyte quality (15, 16).
This study aimed to investigate the impact of oral L-carnitine administration on IVF outcomes in patients with previous treatment failure. Self-control and PSM methodologies were used to ensure reliable results, comparing outcomes before and after L-carnitine supplementation within the same individual and creating comparable groups based on the likelihood of receiving L-carnitine. The findings could significantly influence reproductive medicine, potentially offering a safe, cost-effective, and non-invasive intervention to enhance reproductive success rates and alleviate the emotional and financial burden of infertility for affected couples.
We included patients undergoing their second IVF cycle from January 2018 to May 2023 at the Weifang People’s Hospital Reproductive Medicine Centre. The exclusion criteria were as follows: patients with multiple IVF failures, chromosomal abnormalities, azoospermia, obvious uterine malformation, repeated implantation failure, recurrent spontaneous abortion, and severe intrauterine adhesion. Patients who changed modes of fertilization and ovarian stimulation regimens were also excluded. In total, 515 patients were ultimately included.
All included patients were randomly assigned to the study or control group using computer-generated random numbers placed in sealed, unmarked envelopes. Eligible patients who agreed to participate received an envelope, with group assignment based on whether the generated number was odd or even; this formed our historical cohort. The PSM approach was then used to correct for post-randomization differences, creating a current cohort that was balanced for baseline characteristics between the study and control groups. The ratio between the study group and the control group was set at 1:2 to facilitate a higher PSM matching rate with the expanded control group. All patients provided written informed consent. Patients, nurses, and physicians were not blinded to the assigned groups.
The control group included 362 patients who had not undergone any adjuvant medication prior to the new IVF cycle, and 153 patients were selected as the study group. Women of all ages were included in this study, and ovarian reserve function and anti-Müllerian hormone (AMH) levels were disregarded during patient selection. The median baseline age of the study group was 35 years (IQR,31-40 years) and that of the control group was 35 years (IQR,31-40 years). There was no statistically significant difference in the median baseline levels of AMH between the study group and the control group (p=0.428). The median AMH level was 1.36 ng/mL (IQR, 0.55-2.53 ng/ml) in the study group and 1.10 ng/mL (IQR, 0.41-2.98 ng/ml) in the control group. The flowchart of the study design is shown in Figure 1.
The patients in the study group were administered an oral dose of 3,000 mg L-carnitine (Northeast Pharmaceutical Group, Shenyang, China) per day until the oocyte retrieval day in subsequent cycles. The average duration of oral L-carnitine administration was 44 days (30–52 days). We standardized the endpoint to the date of oocyte retrieval, but standardizing the duration was challenging due to significant individual variation in the time from when patients decided to start a new IVF cycle to the date of oocyte retrieval. The minimum duration was set as 30 days because a previous study confirmed that approximately 30 days of L-carnitine may be enough to increase fertility competence (17).
Appropriate ovarian stimulation protocols, such as gonadotropin-releasing hormone (GnRH) agonist long, GnRH antagonist, and microstimulation protocols, were selected according to the patients’ individual situation.
In the mid-luteal phase, short-acting GnRH-a (0.1, 0.05, or 0.03 mg/day) was administered for pituitary down regulation. Initiation occurred 14–21 days after administration of GnRH agonist considering the serum levels of follicle-stimulating hormone, luteinizing hormone, and estrogen were <5 IU/L, <5 IU/L, and <50 pg/ml, respectively, and follicle diameter was ≤10 mm. Then, 100–300 IU recombinant follicle stimulating hormone was administrated depending on the patient’s age, AMH level, and antral follicle counts.
On days 2–4 of the menstruation cycle when the sinus follicle diameter was 4–6 mm, 100–300 IU recombinant follicle-stimulating hormone was administered to initiate ovarian stimulation. If the dominant follicle was ≥12 mm or the serum levels of estradiol or luteinizing hormone were >500 pmol/l or >10 IU/L, respectively, GnRH antagonist was administered at a dose of 0.25 mg/day until the day before trigger.
The microstimulation protocol is suitable for patients with poor ovarian reserve; thus, controlled ovarian stimulation was initiated for such patients on day 3 of their menstrual cycle using clomiphene or letrozole. Additionally, 150–225 IU gonadotropin was administrated.
We monitored the ovarian follicle developmental progress using vaginal ultrasound scanning and estradiol levels during treatment. If the patients had at least three dominant follicles with diameters >18 mm and 50%–60% of the follicles were ≥16 mm, recombinant human chorionic gonadotropin (hCG) (250 μg) or normal hCG (6,000–10,000 IU) was injected for trigger.
Transvaginal follicle aspiration was performed with ultrasound guidance under intravenous anesthesia 35–37 h after hCG injection. The collected oocytes were fertilized through conventional IVF or intracytoplasmic sperm injection at 39–40 h after hCG injection depending on the patient’s condition. All embryos were cultured using sequential culture medium (Vitrolife, Gothenburg, Sweden) in incubators at 37°C under 5% O2, 6% CO2, and 89% N2 conditions with high moisture until day 6.
Number of Retrieved Oocytes: The total count of oocytes retrieved during the IVF procedure, assessed through stereomicroscopy assessment following oocyte retrieval.
Oocyte Maturation Rate: It is generally related to nuclear maturity, being defined as the proportion of oocytes at MII stage (18).
Normal fertilization: Defined as the appearance of two protonuclei and two polar bodies at 16~18h after insemination (18).
Day three (D3) top-quality embryos: They are generally defined as those originating from normally fertilized oocytes with a cell count of 7–9 on day three after fertilization, the fragmentation rate is less than 10%, and there are no multinucleated embryos (19).
Blastocyst formation rate: Defined as the proportion of 2PN zygotes (not just of cleaved zygotes) which are at the blastocyst stage at Day 5 (116 ± 2 h post-insemination) (18), We employed the Gardner system to evaluate the quality of blastocysts in terms of their expansion, inner cell mass, and trophoblast development (20).
Usable blastocyst rate: This term denotes the combined count of transferred and frozen blastocysts within a single oocyte retrieval cycle. In this study, we decided to exclude the embryo and blastocyst usable rates, as described in the Vienna Consensus. These rates were defined as the ratio of embryos (or blastocysts) suitable for transfer or cryopreservation based on the number of normally fertilized (2PN) oocytes observed on Day 1 (18). Our laboratory personalizes treatments for patients, some of whom undergo freezing on Day 3 or transfer, while the remaining embryos of the cohort are continuously cultured to blastocyst. Meanwhile, others extend embryo culture to blastocysts without Day 3 freezing or transfer. This approach introduces fluctuations in the metric due to the Day 3 freezing/transfer strategy, presenting challenges in standardizing the quality of Day 3 frozen/transfer embryos. To tackle this issue, we focused on the usable blastocyst rate indicator and introduced the concepts of maximum and minimum values. This approach aimed to mitigate the impact of embryo transfer/freezing on Day 3. The usable blastocyst rate—maximum value assumes that all embryos frozen/transfer on Day 3 can be cultured into usable blastocysts. Conversely, the usable blastocyst rate—minimum value assumes that none of the embryos frozen/transfer on Day 3 can be cultured into usable blastocysts.
Cumulative clinical pregnancy and cumulative live birth rate are defined as the delivery of at least one live birth resulting from the initial oocyte retrieval, including all fresh embryo transfers and any subsequent frozen embryo transfers, until the first clinical pregnancy/live birth delivery or until all embryos are used, whichever occurs first.
The sample size determination utilized the PASS software. Data acquisition involved results from 30 cases in both the study and control groups. Key outcome indicators included the normal fertilization rate and the usable blastocyst rate. The change was computed using a two-sided t-test at a significance level of 5%. Upon careful estimation, we determined that assigning 135 patients to both the study and control groups would yield more than 80% power to detect between-group differences.
The Data were analyzed using the Statistical Package for the Social Sciences version 27.0 (SPSS IBM Corp, Armonk, NY, USA). Measured continuous variables were shown as median (interquartile range, IQR), as none of them were subjected to normal distribution. In the preceding cycle, given to the data of the two groups were independent sample, Mann–Whitney U test was used for comparison between the two groups. Categorical variables were expressed as number (percentage) and the Chi-square test or Fisher’s exact test was selected for statistical analyses, as appropriate.
Propensity score matching (PSM) conducts up to 1:1 nearest neighbor matching with a caliper of 0.05 to balance the baseline and improve the comparability between groups. The matching items included age, infertility duration, BMI, AMH, and the day three (D3) top-quality embryos rate, which were collected from the preceding cycle, and matched patients were compared for relevant indicators in the subsequent cycle using Wilcoxon Signed-Rank test as properties of paired samples.
Figure 1 illustrates the flowchart detailing the patient inclusion and exclusion process. Prior to matching, 515 cycles meeting the inclusion criteria were considered for this study. Among them, 153 cycles involved patients who received an oral dose of 3,000 mg L-carnitine per day for a minimum duration of 30 days until the oocyte retrieval day in subsequent cycles. Following propensity score matching (PSM), 140 cycles with L-carnitine pretreatment were successfully matched with 140 routine IVF cycles. Tables 1–3 present the results of the non-parametric test, comparing individual patient percentages. Supplementary Tables S1, S2 include a chi-square test for grouped patients, considering the data as a whole. The consistency of our test results lends robustness to our findings.
Table 1. Baseline characteristics and laboratory outcomes for patients in the preceding cycle before and after PSM.
Table 2. Comparison of laboratory and clinical outcomes in subsequent cycles after propensity score matching (PSM).
Table 3. Analysis of outcomes following control group and study group: utilizing the previous IVF cycle as a self-control.
Baseline characteristics are presented in Table 1, reflecting the initial cycle outcomes of 515 patients before PSM. The study group comprised 153 patients, while the control group included 362 patients. Comparatively, the L-carnitine group exhibited a lower BMI(kg/m2) (23.40(21.11-25.96) versus 22.40(20.89-24.22), P=0.005 and a reduced D3 top-quality embryo rate (%) (25.00 [0.00-60.00] versus 0.00 [0.00-50.00] P=0.018) than the control group in our non-parametric tests, as summarized in Table 1.
To mitigate miscellaneous bias and ensure reliable results, the PSM method was employed to adjust for differences in BMI and the rate of D3 top-quality embryos between the groups. Following 1:1 propensity score matching, Table 1 demonstrates that all baseline characteristics were no longer statistically different between the two groups, creating two comparable cohorts with highly similar characteristics.
Table 2 presents the outcomes of patients following the subsequent follow-up, subsequent to successful PSM. The study group exhibited significantly higher oocyte maturation rates (%) (IQR) (85.71(62.50-100.00) versus 100.00(80.00-100.00), P=0.002), normal fertilization rate (%) (IQR) (75.00(50.00-100.00) versus 80.00(66.67-100.00), p=0.002), and blastocyst formation (%) (IQR) (60.00(0.00-88.89) versus 71.43(50.00-100.00), p=0.003) compared to the control group.
Given the limited number of patients undergoing fresh cycle embryo transfers, we introduced the cumulative clinical pregnancy rate and cumulative live birth rate to strengthen our conclusions. The study group showed higher cumulative clinical pregnancy rates (68.42%) compared to the control group (59.82%, p=0.004). In the study group of 140 patients, 61 have already had live births, and 38 still have frozen embryos or ongoing pregnancies. In the control group, 55 patients have had live births, with 24 still having frozen embryos or ongoing pregnancies. The statistical difference between the two groups (p=0.002) provides strong evidence for our conclusions.
Figures 2A–F display box plots visualizing the distribution of IVF laboratory outcomes for 140 patients after PSM. On the indicators of maturation rate, normal fertilization rate, blastocyst formation rate and usable blastocyst rate -the max and the min, the distribution of the study group’s data shifted significantly to the right. This trend is also seen in Figure 2G, which presents a scatter plot of the study group subtraction from control group.
Figure 2. Box plots and difference scatter plots of laboratory outcomes in the matched pairs of 140 patients after PSM. In Panels (A, B, D–F), compared to the control group, the distribution in the study group is noticeably shifted to the right. In Panel (C), although the median of the two groups is equal, the upper quartile Q3 of the study group is noticeably shifted to the right (55.35% vs. 66.67%). In Panel (G), each point represents the difference between the study group and the control group after 1:1 matching, with the upper number indicating the average of the differences.
To assess the impact of L-carnitine, we compared outcomes from cycles following its administration with those from prior cycles, which served as the self-control group. None of the patients in this study achieved live births in their previous cycles.
The results, outlined in Table 3, reveal substantial improvements in laboratory and clinical outcomes. Although no significant difference in gonadotropin dosage was observed, there was a notable increase in the mean number of retrieved oocytes (IQR) (5(2-9) vs. 6(3-11), p < 0.001). Notably, seven patients who initially did not produce viable oocytes successfully retrieved oocytes following L-carnitine treatment (mean age: 37.7 years, mean AMH concentration: 0.295 ng/ml). A total of 11 oocytes were retrieved after the administration of L-carnitine, resulting in four frozen embryos containing three D3 embryos and one blastocyst.
The average oocyte maturation rate(%) (IQR) also experienced a significant rise (87.50(66.67-100.00) vs.100.00(80.00-100.00), p < 0.001), and the normal fertilization rate (%) (IQR) showed an increase (66.67(33.33-92.58) vs. 78.57(65.48-100.00), p < 0.001). A significant increase was found in the blastocyst formation rate (%) (IQR) (50.00(0.00-75.00) vs. 71.43(50.00-100.00), p < 0.001) and the usable blastocyst rate (%) (IQR)[16.67(0.00-33.33)] vs. 30.38(15.10-50.00), p < 0.001). Given the patients’ history of IVF failure, 50% initially had no D3 top-quality embryos, but this decreased to 25% after taking L-carnitine.
Before oral L-carnitine administration, 63 patients had no usable embryos (neither transferred nor frozen). These patients had an average age of 35.79 ± 5.98 years and an average of 4.69 ± 5.68 retrieved oocytes. After L-carnitine administration, only 26 patients had no usable embryos, with 17 of them having no usable embryos both before and after the treatment. These 17 patients had an average age of 38.54 ± 6.51 years and an average of 1.73 ± 1.48 retrieved oocytes. Following L-carnitine treatment, 46 patients obtained usable embryos, including 18 transferred embryos and 95 frozen embryos (59 at the Day 3 cleavage stage and 36 at the Day 5/6 blastocyst stage).
Table 3 also showed the results of the previous cycle and the subsequent cycle for 362 controls, without any adjuvant medication. Compared to the study group, which showed statistically significant improvements in all indicators (p<.001), the control group showed improvements in the number of retrieved oocytes (p<.001), oocyte maturation rate (p=0.021), normal fertilization rate (p=0.012). However, the rates of D3 top-quality embryos, blastocyst formation, and usable blastocysts-the max did not show any improvement.
Figure 3 presents box plots and difference scatter plots illustrating laboratory results before as self-control and after oral L-carnitine administration for 153 patients in the study group. It is noticeable from Figures 3A–F that there is a significant rightward shift in the distribution of laboratory indicators after administration of L-carnitine. All metrics show improvement in Figure 3G, with average gains ranging from 6%-20%.
Figure 3. Box plots and difference scatter plots illustrating laboratory results before and after oral L-carnitine administration as self-control. In panels (A–F), the overall data in the group after oral L-carnitine administration show a rightward shift. In panel (G), the scatter data are densely distributed above 0% and sparse below 0%, indicating that, on average, all laboratory data have improved.
After 67 IVF cycles following L-carnitine administration, 74 healthy babies were born, including 10 from fresh cycle transfers and 64 from frozen-thawed cycles. The mean gestational age was 38.07 ± 2.58 weeks, five of them less than 37 weeks. The mean birth height of the neonates was 50.33 ± 1.05 cm, with an average birth weight of 3,028.91 ± 662.92 g. Among them, nine had low birth weight, defined as less than 2,500 g, and three had very low birth weight, defined as less than 1,500 g. The proportion of male neonates was 43.24%, possibly influenced by the small sample size in this study (see Table 4).
In this study, we highlight the importance of L-carnitine supplementation in enhancing reproductive outcomes, particularly among patients with varying responses to L-carnitine intake. Responsive patients demonstrate improved outcomes, emphasizing the significance of maintaining adequate L-carnitine levels for reproductive health (Figure 4). Conversely, unresponsive patients show minimal changes in outcomes, suggesting that factors beyond L- carnitine adequacy may contribute to their unresponsiveness. Our methodological approaches ensure a rigorous assessment of L-carnitine supplementation’s impact, yielding valuable insights into its relationship with reproductive outcomes. However, it’s essential to acknowledge the limitations of our current hypothesis.
Figure 4. L-carnitine’s crucial role in regulating fatty acid metabolism and energy production ensures cellular lipid balance and prevents lipid toxicity. This figure illustrates its pivotal role in cellular metabolism and reproductive health. While mainly acquired from external sources, follicle cells produce minimal L-carnitine internally, indicating a potential deficiency within ovarian follicles, which can alter physiology and the microenvironment (A1). L-carnitine() facilitates the transport of long-chain fatty acids (
) across the mitochondrial membrane via the carnitine shuttle (
) system, ensuring efficient entry into mitochondria for β-oxidation. Key enzymes like CPT-1 and CPT-2, with CPT-1 acting as the rate-limiting step (
), control fatty acid β-oxidation at the mitochondrial membrane. L-carnitine also maintains the balance of acyl-CoA and free CoA within mitochondria, crucial for optimal fatty acid metabolism. Oral L-carnitine supplementation is introduced to assess its impact on follicle health and reproductive outcomes, aiming to address L-carnitine deficiency and enhance cellular metabolism and reproductive function. Fatty acids undergo conversion to fatty acyl-CoA and are transported into mitochondria via the L-carnitine shuttle for β-oxidation (A2). A thorough assessment of the effects of L-carnitine supplementation is conducted by comparing the difference in in vivo oocyte maturation rates between the previous cycle and the L-carnitine-supplemented cycle of the study group. Data points above zero are represented by the light orange area, indicating L-carnitine responsiveness, while those below zero are represented by the light green area, indicating unresponsiveness (B1-3, C1-3).
Moreover, elevated levels of non-esterified fatty acids (NEFAs) in follicles can contribute to lipid toxicity, potentially leading to oxidative stress, mitochondrial dysfunction, and cellular damage, adversely affecting follicle and oocyte health (21). While L-carnitine plays a crucial role in fatty acid transport and mitochondrial function, offering a potential avenue for improving oocyte quality and in vitro fertilization (IVF) outcomes (22), addressing L- carnitine unresponsiveness requires personalized approaches. This may involve genetic testing, metabolic profiling, and optimization of L-carnitine supplementation strategies, including adjusting dosage, duration, or mode of administration. In cases where L-carnitine supplementation proves ineffective or contraindicated, exploring alternative interventions such as dietary modifications, lifestyle changes, or pharmacological agents targeting related pathways may be necessary to address metabolic stress and improve oocyte quality. While our study sheds light on L-carnitine responsiveness and its implications for fertility treatment, acknowledging these limitations is crucial for refining future research and clinical interventions in this domain.
Randomized treatment assignment achieved balance in both known and unknown confounding factors between treatment groups. However, in practice, investigators could only introduce a small amount of stratification and could not balance all important variables simultaneously (23). The laboratory and clinical outcomes of IVF are influenced by a multitude of confounding factors, and the randomization of subgroups makes it difficult to achieve a completely balanced baseline. Additionally, the small sample size at our center further increased the risk of an unbalanced baseline.
Propensity score matching (PSM) was defined as the conditional probability of a subject being assigned to the treatment group given the observed covariates. Exact matching of treated and control subjects on the propensity score balances all observed covariates. This approach might provide a more informative evaluation of the efficacy of a new therapy by addressing potential confounding factors arising from imbalanced baseline features (24).
Skewed distributions associated with L-carnitine non-effective patients posed challenges for mean and standard deviation in accurately depicting data tendencies and variability. This underscores the importance of utilizing methods like the quartile-based IQR for a more accurate depiction of data spread. Unlike methods that assume data normality, the IQR avoids such assumptions, rendering it suitable for skewed datasets. By focusing on the middle 50% of the data, the IQR is resilient to outliers, in contrast to percentages, which can be skewed by outliers, potentially leading to misinterpretation.
Our findings, supported by propensity score matching between study and control groups, indicated an approximate 10% increase in oocyte maturation rate, normal fertilization rate, and blastocyst formation rate, along with a 5% rise in the usable blastocyst rate. Consistently, observations from self-control data, involving 153 patients before and after oral L-carnitine administration, revealed significant improvements in laboratory indicators, highlighting the positive impact of L-carnitine on IVF outcomes. Notably, blastocyst formation rate exhibited the highest increase at 20%, followed by the normal fertilization rate at approximately 15%. These results underscore the potential of L-carnitine to enhance reproductive interventions, offering hope for improved outcomes in IVF patients, particularly those with previous IVF failures.
Our study delved into the potential of circulating L- carnitine to enhance oocyte quality in vivo, building upon in vitro observations. We theorized that daily oral intake of L-carnitine before oocyte retrieval could boost oocyte quality and embryonic development, thus improving IVF outcomes. Our investigation indeed demonstrated significant enhancements in embryo quality on days 3 and 5 following L-carnitine administration, aligning with prior research (17). This finding underscores L-carnitine’s positive influence on embryonic development, with implications for IVF success rates. In contrast to Kitano’s findings, our study revealed statistically significant improvements in rates of mature (MII) oocytes and fertilization in the study group compared to the self-control group (17).
The exclusive reliance of oocytes on L-carnitine uptake from follicular fluid emphasizes its crucial role in oocyte development. L-carnitine helps mitigate the harmful effects of non-esterified fatty acids (NEFAs) during the follicular phase and oocyte maturation, optimizing mitochondrial function and reducing lipid toxicity and oxidative stress (25).
L-carnitine is acquired both internally and externally. Research by Montjean et al. has shown minimal expression of L-carnitine synthesis pathway genes in human oocytes, and an absence of L-carnitine synthesis enzymes in cumulus cells (26). This indicates that oocytes are dependent on L-carnitine absorbed externally from follicular fluid, highlighting the importance of regulating L-carnitine in reproductive physiology. The reliance of cumulus-oocyte complexes on externally sourced L-carnitine due to the lack of synthesizing enzymes adds complexity to understanding its role in oocyte development.
The dosage of L-carnitine could explain the discrepancies between our study and Kitano’s. While Kitano administered 1000 mg daily, we used 3000 mg, emphasizing the critical role of precise dosing for consistent fertility outcomes. This difference in dosage likely contributes to the varying rates of mature oocytes and fertilization, underscoring the importance of dosage precision in achieving optimal results with L-carnitine supplementation.
Interestingly, in the control group, despite the absence of adjuvant medication, there was a statistically significant increase in the number of retrieved oocytes and the cumulative clinical pregnancy rate in subsequent cycles. This improvement may be due to clinicians adjusting the treatment protocol based on the patient’s specific characteristics during subsequent ovarian stimulations, such as modifying the dose of ovulation-promoting medication and the timing of the trigger. Additionally, lifestyle changes, such as weight loss or refraining from smoking, may also contribute to better IVF outcomes (27, 28). However, in the study group, the improvement in laboratory and clinical indicators was more pronounced, suggesting that our intervention (oral L-carnitine) had a synergistic effect. This is further supported by improved outcomes in the matched cohort comparisons within the study group relative to the control group.
However, it is important to note that studies by Sheida et al. reported varied outcomes when adding L-carnitine to antagonist ovarian stimulation protocols in IVF/ICSI cycles, particularly in women with polycystic ovarian syndrome (PCOS) (29, 30). Despite differences, these studies concluded that L-carnitine supplementation during ovulation induction in PCOS women did not significantly improve outcomes compared to control groups. Our study included a small number of PCOS patients—specifically, 5 out of 153 in our self-control group and 5 out of 140 in the PSM group—which limits our ability to investigate potential differences in this subset.
Addressing the clinical effects of L-carnitine at the intraovarian level presents challenges due to the intricate cellular and molecular aspects of PCOS, highlighting the need for further research to comprehend its clinical mechanisms across diverse patient populations, including those with PCOS, and to potentially discover new applications for ovarian dysfunctions associated with redox imbalance. Meanwhile, understanding the reasons for carnitine unresponsiveness can refine its utilization in fertility care, allowing for adjustments in factors such as dosage, duration, or administration method to enhance effectiveness for specific patient groups. This nuanced approach, which may involve exploring alternative interventions like dietary adjustments or lifestyle changes, ensures personalized treatment tailored to individual needs, thus maximizing the potential benefits for enhancing oocyte quality and fertility outcomes.
Our primary objective was to provide robust evidence supporting our hypothesis that daily oral consumption of L-carnitine before oocyte retrieval is beneficial. If L-carnitine supplementation proves ineffective, alternative interventions, such as dietary adjustments or targeted pharmacological agents affecting related pathways, should be explored. Recognizing these limitations is essential for refining future research and clinical strategies. Further investigations are imperative to discern L-carnitine’s clinical impacts on various patient demographics, identify factors contributing to L-carnitine unresponsiveness, and enable personalized treatment adjustments to optimize oocyte quality and fertility outcomes.
The raw data supporting the conclusions of this article will be made available by the authors, without undue reservation.
The studies involving humans were approved by The Ethics Committee of Weifang People’s Hospital. The studies were conducted in accordance with the local legislation and institutional requirements. The participants provided their written informed consent to participate in this study.
WZ: Conceptualization, Data curation, Formal analysis, Investigation, Methodology, Software, Visualization, Writing – original draft. KL: Data curation, Formal analysis, Investigation, Software, Writing – original draft. YZ: Formal analysis, Methodology, Visualization, Writing – review & editing. PS: Methodology, Software, Writing – original draft. EZ: Methodology, Writing – review & editing. LM: Methodology, Visualization, Writing – original draft. HM: Methodology, Project administration, Writing – review & editing.
The author(s) declare that no financial support was received for the research, authorship, and/or publication of this article.
We thank Prof. Suzhen Wang of the department of Health Statistics, Shandong Second Medical University, for her guidance on statistical methodology.
Authors EZ, and LM were employed by company California IVF Fertility Center.
The remaining authors declare that the research was conducted in the absence of any commercial or financial relationships that could be construed as a potential conflict of interest.
All claims expressed in this article are solely those of the authors and do not necessarily represent those of their affiliated organizations, or those of the publisher, the editors and the reviewers. Any product that may be evaluated in this article, or claim that may be made by its manufacturer, is not guaranteed or endorsed by the publisher.
The Supplementary Material for this article can be found online at: https://www.frontiersin.org/articles/10.3389/fendo.2024.1440182/full#supplementary-material
IVF, in vitro fertilization; PSM, propensity score matching; IQR, interquartile range; BMI, body mass index; AMH, anti-Müllerian hormone; GnRH, gonadotropin-releasing hormone; HCG, human chorionic gonadotropin; PCOS, polycystic ovary syndrome; NEFAs, non-esterified fatty acids; GN, gonadotropin; LBW, low birth weight; VLBW, very low birth weight.
1. Lotti F, Marchiani S, Corona G, Magg M. Metabolic syndrome and reproduction. Int J Mol Sci. (2021) 22(4):1988. doi: 10.3390/ijms22041988
2. Diagnostic evaluation of the infertile female: a committee opinion. Fertil Steril. (2015) 103:e44–50. doi: 10.1016/j.fertnstert.2015.03.019
3. Joint news release WHO /UNFPA: top level push to takle priorities in sexual and reproductive health. Indian J Med Sci. (2006) 60:255–7.
4. Vo KCT, Sato Y, Kawamura K. Improvement of oocyte quality through the SIRT signaling pathway. Reprod Med Biol. (2023) 22:e12510. doi: 10.1002/rmb2.12510
5. Marchiani S, Tamburrino L, McPherson N, Bald E. Editorial: the role of obesity and metabolic syndrome in couple infertility. Front Endocrinol. (2021) 12:784716. doi: 10.3389/fendo.2021.784716
6. Van Hoeck V, Bols PEJ, Binelli M, Leroy JL. Reduced oocyte and embryo quality in response to elevated non-esterified fatty acid concentrations: A possible pathway to subfertility? Anim Reprod Sci. (2014) 149:19–29. doi: 10.1016/j.anireprosci.2014.07.015
7. Desmet KLJ, Marei WFA, Pintelon I, Bols PEJ, Leroy JLMR. The effect of elevated non-esterified fatty acid concentrations on bovine spermatozoa and on oocyte in vitro fertilisation. Reprod Fertil Dev. (2018) 30(11):1553–65. doi: 10.1071/RD17507
8. Kirillova A, Smitz JEJ, Sukhikh GT, Mazunin I. The role of mitochondria in oocyte maturation. Cells. (2021) 10(11):2484. doi: 10.3390/cells10092484
9. Bradley J, Swann K. Mitochondria and lipid metabolism in mammalian oocytes and early embryos. Int J Dev Biol. (2019) 63:93–103. doi: 10.1387/ijdb.180355ks
10. Liu T, Qu JX, Tian MY, Yang R, Song XL, Li R, et al. Lipid metabolic process involved in oocyte maturation during folliculogenesis. Front Cell Dev Biol. (2022) 10:806890. doi: 10.3389/fcell.2022.806890
11. Longo N, Frigeni M, Pasquali M. Carnitine transport and fatty acid oxidation. Biochim Biophys Acta (BBA) - Mol Cell Res. (2016) 1863:2422–35. doi: 10.1016/j.bbamcr.2016.01.023
12. Placidi M, Emidio GD, Virmani A, D'Alfonso A, Artin PG, D'Alessandro AM, et al. Carnitines as mitochondrial modulators of oocyte and embryo bioenergetics. Antioxidants. (2022) 11:745. doi: 10.3390/antiox11040745
13. Agarwal A, Sengupta P, Durairajanayagam D. Role of L-carnitine in female infertility. Reprod Biol Endocrinol. (2018) 16:5. doi: 10.1186/s12958-018-0323-4
14. Rebouche CJ. Kinetics, pharmacokinetics, and regulation of l-carnitine and acetyl-l-carnitine metabolism. Ann N Y Acad Sci. (2009) 1033:30–41. doi: 10.1196/annals.1320.003
15. Morimoto N, Hashimoto S, Yamanaka M, Satoh M, Nakaoka Y, Fukui A, et al. Treatment with Laevo (l)-carnitine reverses the mitochondrial function of human embryos. J Assist Reprod Genet. (2020) 38:71–8. doi: 10.1007/s10815-020-01973-6
16. Kalehoei E, Mojtaba M, Mehri A, Hossein Z, Samaneh ALA, Hamid M, et al. Therapeutic effects of L-arginine, L-carnitine, and mesenchymal stem cell-conditioned medium on endometriosis-induced oocyte poor quality in an experimental mouse model. J Obstet Gynaecol Res. (2023) 49:1180–8. doi: 10.1111/jog.15569
17. Kitano Y, Hashimoto S, Matsumoto H, Yamochi T, Yamanaka M, Nakaoka Y, et al. Oral administration of l-carnitine improves the clinical outcome of fertility in patients with IVF treatment. Gynecol Endocrinol. (2018) 34:684–8. doi: 10.1080/09513590.2018.1431769
18. The Vienna consensus: report of an expert meeting on the development of ART laboratory performance indicators. Reprod Biomed Online. (2017) 35:494–510. doi: 10.1016/j.rbmo.2017.06.015
19. Sun Q, Huang GN, Sun HX, Fan LQ, Feng Y, Shen H, et al. CSRM consensus on key indicators for quality control in IVF laboratory. J Reprod Med. (2018) 29:836–51.
20. Gardner D, Michelle L, John S, Terry S, William B. Blastocyst score affects implantation and pregnancy outcome: towards a single blastocyst transfer. Fertil Steril. (2000) 73:1155–8. doi: 10.1016/S0015-0282(00)00518-5
21. Shi M, Sirard M-A. Metabolism of fatty acids in follicular cells, oocytes, and blastocysts. Reprod Fertil. (2022) 3:R96–R108. doi: 10.1530/RAF-21-0123
22. Li JJ, Liu LY, Weng J, Yin TL, Yang J, Feng HL. Biological roles of l-carnitine in oocyte and early embryo development. Mol Reprod Dev. (2021) 88:673–85. doi: 10.1002/mrd.23542
23. Xu Z, Kalbfleisch JD. Propensity score matching in randomized clinical trials. Biometrics. (2009) 66:813–23. doi: 10.1111/j.1541-0420.2009.01364.x
24. Teng SW, Su YC, Pallantla R, Channavazzala M, Kumar R, Sheng YC, et al. Can a propensity score matching method be applied to assessing efficacy from single-arm proof-of-concept trials in oncology? CPT: Pharmacometrics Syst Pharmacol. (2023) 12:1347–57. doi: 10.1002/psp4.13014
25. Catandi GD, Cheng MH, Chicco AJ, Chen T, Carnevale EM. L-carnitine enhances developmental potential of bovine oocytes matured under high lipid concentrations in vitro. Anim Reprod Sci. (2023) 252:107249. doi: 10.1016/j.anireprosci.2023.107249
26. Montjean D, Entezami F, Lichtblau I, Belloc S, Gurgan T, Menezo Y, et al. Carnitine content in the follicular fluid and expression of the enzymes involved in beta oxidation in oocytes and cumulus cells. J Assist Reprod Genet. (2012) 29:1221–5. doi: 10.1007/s10815-012-9855-2
27. Chen Y, Yang SH, Zheng W, Zu RW, Ran SY, Wu H, et al. Effect of a 60-day weight reduction intervention prior to IVF/ICSI on perinatal outcomes in overweight or obese infertile women. Front Endocrinol. (2022) 13:1626–9. doi: 10.3389/fendo.2022.1062790
28. Hornstein MD. Lifestyle and IVF outcomes. Reprod Sci. (2016) 23:1626–9. doi: 10.1177/1933719116667226
29. Hafezi M, Arabipoor A, Ghaffari F, Vesali S, Zareei M, Hessari ZH. Adding L-carnitine to antagonist ovarian stimulation doesn’t improve the outcomes of IVF/ ICSI cycle in patients with polycystic ovarian syndrome: a double-blind randomized clinical trial. J Ovarian Res. (2024) 17:9. doi: 10.1186/s13048-023-01319-7
30. Sheida A, Davar R, Tabibnejad N, Eftekhar M. The effect of adding L-Carnitine to the GnRH-antagonist protocol on assisted reproductive technology outcome in women with polycystic ovarian syndrome: a randomized clinical trial. Gynecol Endocrinol. (2021) 39:1878135. doi: 10.1080/09513590.2021.1878135
Keywords: L-carnitine, propensity score matching, oocyte, normal fertilization, blastocyst formation
Citation: Zhao W, Liu K, Zhang Y, Sun P, Zeringue E, Meng L and Ma H (2024) The efficacy of orally administered L-carnitine in alleviating ovarian dysfunctions has laid the foundation for targeted in vivo use: a study employing self-control and propensity score matching. Front. Endocrinol. 15:1440182. doi: 10.3389/fendo.2024.1440182
Received: 29 May 2024; Accepted: 26 August 2024;
Published: 18 September 2024.
Edited by:
Kuan-Hao Tsui, Kaohsiung Veterans General Hospital, TaiwanReviewed by:
Xiuliang Dai, Changzhou Maternal and Child Health Care Hospital, ChinaCopyright © 2024 Zhao, Liu, Zhang, Sun, Zeringue, Meng and Ma. This is an open-access article distributed under the terms of the Creative Commons Attribution License (CC BY). The use, distribution or reproduction in other forums is permitted, provided the original author(s) and the copyright owner(s) are credited and that the original publication in this journal is cited, in accordance with accepted academic practice. No use, distribution or reproduction is permitted which does not comply with these terms.
*Correspondence: Huagang Ma, bWFodWFnYW5nQDEyNi5jb20=; Li Meng, bGltZW5naXZmQHlhaG9vLmNvbQ==
Disclaimer: All claims expressed in this article are solely those of the authors and do not necessarily represent those of their affiliated organizations, or those of the publisher, the editors and the reviewers. Any product that may be evaluated in this article or claim that may be made by its manufacturer is not guaranteed or endorsed by the publisher.
Research integrity at Frontiers
Learn more about the work of our research integrity team to safeguard the quality of each article we publish.