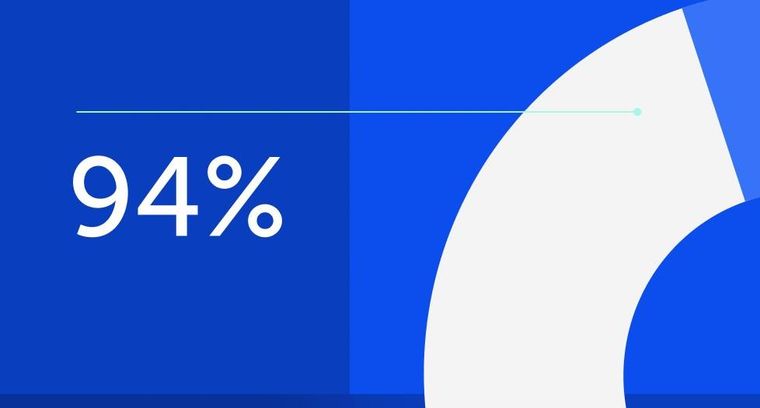
94% of researchers rate our articles as excellent or good
Learn more about the work of our research integrity team to safeguard the quality of each article we publish.
Find out more
ORIGINAL RESEARCH article
Front. Endocrinol., 01 October 2024
Sec. Cancer Endocrinology
Volume 15 - 2024 | https://doi.org/10.3389/fendo.2024.1437197
Background: Some experimental data suggest that myokines may play an important role in developing cancer-associated cachexia (CAC), but their relevance in humans remains poorly explored. In our study, we tested the hypothesis that circulating myokines are associated with the pathogenesis of CAC in a model population of gastric cancer.
Methods: A group of 171 treatment naïve patients with adenocarcinoma of the stomach were prospectively examined. Cachexia was defined as weight loss >5% or weight loss >2% with either BMI <20 kg/m2 or sarcopenia. A panel of 19 myokines was measured in portal and peripheral blood as well as tumour tissue and surrounding gastric mucosa. Moreover, a serum proteomic signature of cachexia was identified by a label-free quantitative proteomics with a nano LC-MS/MS system and stored in a ProteomeXchange database (PXD049334).
Results: One hundred (58%) patients were diagnosed with CAC. The concentrations of fatty acid-binding protein 3 (FABP3), follistatin-like 1 protein (FSTL−1), interleukin 6 (IL 6), and interleukin 8 (IL 8) were significantly higher in the peripheral blood of cachectic subjects, while leptin levels were lower. Of all the evaluated myokines, tumour tissues showed higher expression levels only for IL-15 and myostatin. However, the analysis of paired samples failed to demonstrate a decreasing concentration gradient between the portal and peripheral blood for any of the myokines, evidencing against their release by the primary tumour. Proteomic analysis identified 28 proteins upregulated and 24 downregulated in the peripheral blood of patients with cachexia. Differentially expressed proteins and 5 myokines with increased serum levels generated a significant protein-protein interaction network.
Conclusions: Our study provides clinical evidence that some myokines are involved in the pathogenesis of cachexia and are well integrated into the regulatory network of circulating blood proteins identified among cachectic patients with gastric cancer.
Cancer-associated cachexia (CAC) is a metabolic wasting syndrome associated with an involuntary progressive loss of skeletal muscle and fat tissue (1). The resulting multifaceted functional impairment is responsible for reduced compliance and efficacy of anticancer treatment, increased treatment-related toxicity, and higher patient mortality (2). Clinical trials utilizing standard regimens of nutritional support showed only moderate efficacy, emphasizing the urgent need for new pharmacological interventions to reverse metabolic disturbances associated with CAC (3–5). Therefore, the search for clinically relevant molecular pathways responsible for the pathogenesis of cachexia is still required.
There is a common consensus that some circulating factors orchestrating the crosstalk between the primary tumor and the involved organs play an important role in the pathogenesis of CAC (6). Since the loss of skeletal muscle is a hallmark of cachexia, much attention has been paid to a rapidly expanding group of signaling molecules called myokines (7). Members of this heterogenous family were shown to act in autocrine, paracrine, or endocrine manners affecting the muscle and distant organs, including adipose tissue, brain, bone, or liver. Although these interactions were shown to contribute to impaired muscle mass and functional changes typical for CAC, some important questions are still to be answered (8, 9). It remains unclear whether there exists a common myokine-dependent mechanism of cachexia. In fact, some previous studies already emphasized marked diversity not only between cancer and non-cancer cachexia, but also between individual cancer types and the prevalence of CAC, possibly related to expression of pro-cachectic mediators (10, 11). Moreover, there have been no satisfactory explanations demonstrating the origins of pro-cachectic factors circulating in the blood. Finally, many previous observations were made solely from experimental data without proper validation in clinical settings.
In this prospective study, we sought to explore the importance of selected myokines in a homogenous population of patients with gastric cancer characterized by high rates of CAC (1). Myokine levels in peripheral blood were compared between patients with and without cachexia to identify mediators potentially involved in the pathogenesis of CAC. Moreover, we compared myokine concentrations in paired samples of portal and peripheral blood, as well as cancer tissue and surrounding healthy mucosa, to verify whether they are released by the primary tumor. Finally, a label-free quantitative proteomics was applied to explore other potential circulating mediators of CAC and to analyze their interactions with myokines.
Treatment naïve patients with histologically proven adenocarcinoma of the stomach diagnosed between January 2015 and December 2019 were prospectively examined. The extent of surgery, definitions for lymph node dissection, and tumor staging were adapted to the recent guidelines (12, 13). All data was collected prospectively and recorded in a dedicated database.
Cachexia was defined using international consensus criteria as weight loss >5% over past 6 months, or weight loss > 2% in individuals with body-mass index (BMI) <20 kg/m2 or reduced skeletal muscle mass (sarcopenia) (14). Nutritional status was evaluated using clinical (body mass index [BMI], weight loss) and laboratory indices (albumin, C-reactive protein (CRP), lymphocyte counts), as well as composite scores (Nutrition Risk Screening-2002 [NRS-2002], Prognostic Nutrition Index [PNI]). Muscle mass was evaluated using the lumbar skeletal muscle index (SMI) using CT scans as previously described (15).
Blood samples were collected before starting any tumor-oriented treatment. Peripheral venous blood (5 ml) was collected into sterile BD Vacutainer® tubes under fasting conditions. In a subgroup of consecutive 24 patients selected for proteomic analysis, another 5 ml was drawn intraoperatively from the portal circulation. For paired sample analyses, both blood samples were collected on the same day. After being allowed to clot at room temperature for 60 minutes, the samples were centrifuged at 2000×g for 10 min at 4°C. The serum was removed and stored at −80°C until analysis. Moreover, pairs of primary tumors and corresponding normal gastric mucosa were sampled from patients’ surgical specimens immediately after resection. The fresh specimens were snap frozen in liquid nitrogen and stored at −80°C until processing. Some portions of the collected specimens were used for routine histopathology to verify adequate cellularity of samples corresponding to tumor tissue and normal gastric mucosa.
A set of 19 myokines most probably related to cachexia was selected based on previous experimental and clinical studies (8, 9, 16). Serum myokine levels were evaluated using a multiplex immunofluorescent assay platform (Luminex MAGPIX, Merck KGaA, Germany). Human Myokine Magnetic Bead Panel (HMYOMAG-56K, Merck KGaA, Germany) and Human Circulating Cancer Biomarker Magnetic Bead Panel 1 (HCCBP1MAG-58K, Merck KGaA, Germany) were used following the manufacturer’s instructions. Human Parathyroid Hormone Related Protein ELISA Kit (orb406495, Biorbyt Ltd., UK) was used for PTHrP assays.
The complete analytical procedures were carried out as detailed in Supplementary Methods. Briefly, after selective immunodepletion of albumin and immunoglobulins with the Multiple Affinity Removal System (MARS), serum samples were prefractionated with Pierce™ C18 spin columns. Tissue samples were processed after homogenization. Protein fractions were subsequently separated with a Proxeon nanoscale liquid chromatography system (Bruker Daltonics) and identified using an amaZon ETD mass spectrometer (Bruker Daltonics) (nano LC-MS/MS). For label-free quantitative proteomic analysis, three independent MS runs were completed for each sample and the relative differences in protein levels were determined by the ProfileAnalysis package (Bruker-Daltonics) according to the manufacturers’ recommendations. The acquired spectra were identified using the Mascot algorithm against the Swiss-Prot/TrEMBL sequence database. The mass spectrometry proteomics data have been deposited to the ProteomeXchange Consortium via the PRIDE partner repository with the dataset identifier PXD049334 (17). The Panther classification system was used to identify gene ontology terms (pantherdb.org) (18). Potential associations between proteins related to cachexia identified by myokine assays and proteomic analysis were assessed by the STRING EMBL software (version 11.5, https://string-db.org/) (19).
The differences in proportions between groups were evaluated using the chi-square test, and the Mann–Whitney U test was used to evaluate differences in quantitative variables A t-test model was used to evaluate the fold change in the bucket tables generated based on the ProfileAnalysis. Principal Component Analysis (PCA) with a scaling algorithm was conducted for data overview. The significance level (P) <0.05 in a two-tailed test was considered statistically significant. All statistical analyses were performed using the IBM® SPSS® Statistics 28 software package (IBM Corporation, NY) and RStudio (Integrated Development Environment for R) version 2021.9.2.382.
A total of 171 patients with gastric cancer diagnosed between January 2015 and December 2019 were recruited to participate in the study. Cachexia was diagnosed in 100 of 171 (58%) subjects. The comparison of demographic and clinical characteristics between patients with and without cachexia is summarized in Table 1. Generally, patients with cachexia were older and had clinical and laboratory findings typical for malnutrition. Gastric cancers among cachectic patients were larger and had more advanced stages. The absence of cachexia was associated with a significantly higher proportions of resectable (95% vs 83%, P=0.019) and curatively resected (95% vs 69%, P=0.001) disease.
The peripheral levels of circulating myokines in patients with and without cachexia are summarized in Table 2 and sex-related variability is presented in Supplementary Table S1. Patients with cachexia had significantly higher levels of fatty acid-binding protein 3 (FABP3), follistatin-like 1 protein (FSTL−1), interleukin 6 (IL 6), and interleukin 8 (IL 8), while leptin concentrations in the peripheral blood were significantly lower (Figure 1). Individual myokines were poor predictors of cachexia with AUC for ROC curves not exceeding 0.690 (Supplementary Table S2, Supplementary Figure S1).
Figure 1. Serum myokine concentrations in the peripheral blood (n=171). Data are expressed as median (center line) and interquartile range (box). Mann–Whitney U test was used to compare median values between patients with and without cachexia.
Figure 2 shows results of the correlation analysis between individual myokines as well as myokines and clinical variables. Of all tested myokines, only leptin showed significant age- and sex-dependent variability. Overall blood levels of leptin were higher in females (2229 vs 1126 pg/mL, P=0.017) and were positively correlated with age (R=0.22, P=0.012). There was a weak negative association of patients’ BMI with FABP3 (R= –0.20, P=0.011) and IL 8 (R= –0.18, P=0.017), while leptin levels showed a moderate positive correlation (R= 0.59, P=0.001) (Supplementary Figure S2). Furthermore, leptin was negatively correlated with preoperative percentage weight loss (R= –0.23, P=0.003), but IL 6 (R= 0.27, P=0.001) and IL 8 (R= 0.32, P=0.001) showed positive correlations (Supplementary Figure S3). FSTL−1, FABP3 and IL 6 were not associated with tumor stage (Supplementary Figure S4). However, serum levels of interleukin 8 were significantly lower among patients with stage I/II disease compared to stage III and IV. An opposite relationship was found for leptin, where median levels for stage IV disease (776 pg/mL) were higher than either I/II (1834 pg/mL, P<0.001) or III (1637 pg/mL, P=0.006).
Figure 2. Spearman correlation matrix for variables. Only correlations with P <0.05 are shown and correlation strength is indicated by colour intensity.
Serum and tissue samples from 24 patients, including 16 diagnosed with cachexia, were selected for comparative myokine analysis and label-free quantitative proteomics. There were no significant differences in demographic and clinical characteristics between this subgroup and the overall population. Analysis of paired portal and peripheral blood samples failed to identify any myokine with elevated levels in the portal circulation (Supplementary Table S3). However, portal concentrations of apelin, fibroblast growth factor 21 (FGF21), fractalkine, and leptin were significantly reduced compared to the peripheral blood (Figure 3). Supplementary Figure S5 shows Spearman correlation matrix for portal and peripheral blood levels of myokines.
Figure 3. Myokine concentrations in paired samples of portal and peripheral blood (n=24). Data are expressed as median (center line) and interquartile range (box). Wilcoxon signed-rank test was used to compare paired samples.
Relative myokine expression between the primary tumor and adjacent healthy gastric mucosa was evaluated by LC-MS/MS. No signal for erythropoeitin, fractalkine, leukemia inhibitory factor (LIF), osteocrin, and osteonectin could be detected in the samples. Of all the remaining myokines, tumor tissues showed higher expression only for IL-15 and myostatin with relative abundance ratios of 2.4 and 3.1, respectively.
The proteomic analysis identified 2507 unique proteins in all serum samples, including 1455 found only in patients with cachexia (Supplementary Tables S4, S5). There were 28 proteins upregulated and 24 downregulated among cachectic patients (Figures 4, 5). The protein-protein interaction (PPI) network for all 52 differentially expressed proteins and 5 myokines with increased serum levels generated 55 nodes and 77 edges with an average node degree of 2.8 and the PPI enrichment P value of 10-16 (Figure 6).
Figure 4. Bioinformatics analysis of proteomic data (n=24). (A) Distribution of proteins detected in patients with and without cachexia. (B) List of up- and down-regulated proteins among cachectic patients.
Figure 5. PANTHER classification of differentially expressed proteins detected by proteomic analysis.
Figure 6. STRING protein-protein interaction (PPI) network for 5 differentially expressed blood myokines (rectangles) and proteins identified by proteomic analysis. Disconnected nodes are hidden. Line thickness indicates the strength of data support.
This study carried out a comprehensive profiling of circulating myokines in a population of Western patients with gastric cancer. Five myokines were found to be potentially associated with cancer cachexia, but none of them were released by the primary tumor. However, we demonstrated that all these myokines were well integrated into the interaction network of circulating proteins identified among cachectic patients by proteomic profiling.
Data from animal and in silico models sufficiently demonstrated a complex network of interactions between several active mediators potentially associated with cachexia, including cytokines, myokines, adipokines, and tumor factors (20–24). However, there is still a translational gap between human and animal studies, which prevents a simple extrapolation of experimental results. This is particularly relevant for myokines, where most clinical studies measured only a limited number of mediators in relatively small populations recruiting patients with diverse cancers (25, 26).
Myokines are a heterogeneous group that includes more than 600 different signaling molecules produced and released by skeletal muscles (7). Their biological function is to control myocyte activity through an autocrine loop, as well as to provide communication with other tissues and organs (paracrine and endocrine effects). Interleukin 6 (IL-6) derived from skeletal muscles has been identified as the first myokine secreted into the systemic circulation (27) Subsequent clinical and experimental studies have demonstrated its pleiotropic activity and its multifaceted role in cancer cachexia (7, 28). The Janus-faced behavior of IL-6 is related to the fact that circulating cytokine promotes chronic inflammation leading to catabolic changes and skeletal muscle atrophy, while released from myocytes improves muscle metabolism by anti-inflammatory effects. Interleukin-8 (IL-8), a member of the chemokines family, is an inflammatory mediator that exerts chemoattractant activity for lymphocytes and neutrophils, as well as promotes angiogenesis (29). IL-8 was also found in skeletal muscles after exercise, but its physiological function as a myokine remains largely unknown. Fatty acid binding protein 3 (FABP3) is mainly responsible for intracellular transport of lipids, and high expression levels are found in cardiac and skeletal muscles (30). Various types of muscle injury are associated with an increase in circulating FABP3, and some studies suggested that this myokine may have a protective effect against oxidative stress (31). Follistatin-like protein 1 (FSTL-1) is found in most tissues, including cardiomyocytes and skeletal muscles (32). FSTL1 is related to the activation of angiogenic factors, possibly related to vascularization necessary for muscle fiber regeneration (33). Leptin, initially treated as a classic adipokine, was subsequently identified also in skeletal muscles along with abundant expression of its receptors (34). In addition to its metabolic effects, which involve anti-lipogenic activity and improved glucose disposal in skeletal muscles, myokine increases proliferation playing the role of a muscle growth factor (35).
The current study enrolled a homogeneous population of patients with gastric cancer characterized by a high prevalence of cachexia to evaluate the role of myokines in the pathogenesis of CAC. Based on data from previous observations, mostly conducted in experimental settings, 19 myokines possibly associated with cachexia were selected (1, 6–9, 36). We found that peripheral serum levels of FABP3, FSTL−1, interleukin 6, and interleukin 8 were significantly higher in cachectic patients, while leptin levels were decreased. Several previous studies reported similar observations for IL 6, IL 8, and leptin (25, 26). However, very little human data is available for the other two myokines identified in this study, i.e., FABP3 and FSTL−1. Recently, de Castro et al. evaluated plasma and tumor levels of selected myokines (IL 6, IL 8, IL 15, FABP3, FSTL−1, BDNF, irisin, and myostatin) in 94 patients with gastric or colorectal cancers (37). Of the eight candidate biomarkers, higher blood levels were found among cachectic subjects only for IL 6, IL 8, and FABP3. No further clinical data could be identified linking FABP3 or follistatin-like protein 1 (FSTL−1) with cachexia (38–40).
One of the unresolved issues related to the mechanisms of CAC is the source of circulating mediators. For gastrointestinal malignancies, any tumor-derived signalling molecule released into the portal blood must pass through the liver before reaching the systemic circulation. Studies evaluating hepatic clearance of IL 6 and IL 8 among subjects with normal liver function suggested either decreased (41, 42) or unchanged (43–45) concentrations in the peripheral blood compared to the portal compartment. Therefore, cytokines measured in routine blood samples do not necessarily represent the cytokine profile released by gastric cancers and no appropriate studies were carried out for most myokines. To verify the hypothesis that the primary tumor was responsible for releasing factors inducing cachexia, we compared myokine concentrations in paired samples of portal and peripheral blood, as well as cancer tissue and surrounding healthy mucosa. Relative tissue myokine expression evaluated by the LC-MS/MS method showed increased tumor levels only for IL-15 and myostatin, arguing against the possibility of increased production of cachexia related mediators within the tumor. Moreover, the analysis of paired samples from tumor-draining veins and the peripheral circulation failed to demonstrate a decreasing concentration gradient between the portal and peripheral blood for any of the evaluated myokines, including those associated with cachexia (FABP3, FSTL−1, IL 6, IL 8, leptin). In fact, leptin levels were significantly higher in the peripheral circulation. Altogether, these observations clearly demonstrated that the origin of circulating myokines associated with CAC was different from the primary tumor.
Given the paucity of human data, mechanisms involving circulating myokines in CAC are poorly understood. Therefore, our objective was to examine the network of cachexia-related mediators using a label-free quantitative proteomic approach. Analysis of serum samples from patients with and without CAC applying the proteomic workflow based on a nano LC-MS/MS system identified 52 differentially expressed proteins. We interrogated molecular pathways of these proteins and five differentially expressed myokines by the protein–protein interaction (PPI) network analysis using the STRING database. The P value of the PPI enrichment model shoved a highly significant network of interactions. There were 60 gene ontology biological processes related to the identified myokines, including 23 for leptin and FABP3, 21 for IL 6, and 8 for IL 8.
Very few studies have used complex proteomic profiling of patients with cachexia, and most of them were focused on muscle samples (46–50). To the best of our knowledge, only one previous attempt has been made to characterize circulating blood proteins in this group of patients. Narasimhan et al. used an aptamer-based platform to screen 1294 plasma proteins from 30 patients with pancreatic ductal adenocarcinoma using weight loss of at least 5% during the prior 6 months as the definition of cachexia (51). They found 67 differentially expressed proteins, including 10 up-regulated and 57 down-regulated. Although no protein overlapped with the current study, leptin correlated with weight loss grade, skeletal muscle index, and total adipose index, while FABP3 correlated with skeletal muscle density. Moreover, IL 6 was suggested as one of the possible upstream regulators for molecules involved in the pathogenesis of cachexia.
The results of this study provide important clinical evidence for further research focused on circulating myokines in the pathogenesis of cancer-associated cachexia. However, certain important limitations should be considered. First, we were unable to provide some functional parameters associated with cachexia, including quality of life and muscle strength. Although such information was not necessary to diagnose cachectic patients, it could provide some additional insights. Second, we did not validate the results of proteomic analyses using other methods, such as ELISA. However, the main aim of proteomic profiling in this study was to dissect protein-protein interaction networks of myokines potentially involved in CAC instead of validation of new cachexia-associated biomarkers. Third, we used ‘myokines’ to designate the molecules evaluated in our study. All were selected based on previous reports suggesting their pivotal role in muscle–organ crosstalk potentially related to cachexia. However, by definition, myokines refer to a group of mediators that are produced and released by skeletal muscle cells. All myokines identified in our study as potentially associated with cancer cachexia are normally released by various cells, predominantly within the immune system (IL-6, IL−8, FABP3) and adipose tissue (leptin, FSTL-1). Since there is no analytical method currently available to determine the actual source of circulating mediators, there is still a gap in mechanistic understanding of the members of the myokine family found in blood.
Overall, our study provides clinical evidence that some myokines are involved in the pathogenesis of cachexia and are well integrated into the regulatory network of circulating blood proteins identified among cachectic patients. As none of these myokines was released by the primary tumor, this has important implications for further studies on the pathogenesis of cancer-associated cachexia.
The datasets presented in this study can be found in online repositories. The names of the repository/repositories and accession number(s) can be found below: https://www.ebi.ac.uk/pride/archive/, PXD049334.
The studies involving humans were approved by Bioethics Committee of the Jagiellonian University. The studies were conducted in accordance with the local legislation and institutional requirements. The participants provided their written informed consent to participate in this study.
MSi: Conceptualization, Data curation, Formal analysis, Funding acquisition, Investigation, Methodology, Project administration, Supervision, Visualization, Writing – original draft, Writing – review & editing. AD: Data curation, Investigation, Methodology, Visualization, Writing – original draft. MSa: Data curation, Investigation, Methodology, Visualization, Writing – review & editing. RC: Data curation, Investigation, Methodology, Visualization, Writing – review & editing. PR: Conceptualization, Project administration, Supervision, Writing – review & editing.
The author(s) declare financial support was received for the research, authorship, and/or publication of this article. This study was supported by the Polish National Science Centre, grant No. UMO-2013/09/B/NZ4/02531 and UMO-2017/27/B/NZ4/02143 The funding source had no involvement in study design, in the collection, analysis and interpretation of data; in the writing of the report; and in the decision to submit the article for publication.
The authors declare that the research was conducted in the absence of any commercial or financial relationships that could be construed as a potential conflict of interest.
All claims expressed in this article are solely those of the authors and do not necessarily represent those of their affiliated organizations, or those of the publisher, the editors and the reviewers. Any product that may be evaluated in this article, or claim that may be made by its manufacturer, is not guaranteed or endorsed by the publisher.
The Supplementary Material for this article can be found online at: https://www.frontiersin.org/articles/10.3389/fendo.2024.1437197/full#supplementary-material
Supplementary Methods | Detailed description of label-free quantitative proteomics with a nano LC-MS/MS system.
AJCC, American Joint Committee on Cancer; ASA, American Society of Anesthesiologists; AUC, area under the curve; BDNF, brain-derived neurotrophic factor; BMI, body-mass index; CAC, cancer-associated cachexia; CRP, C-reactive protein; CT, computed tomography; FABP3, fatty acid-binding protein 3; FGF2, fibroblast growth factor 2; FGF21, fibroblast growth factor 21; FSTL-1, follistatin-like 1 protein; HU, Hounsfield units; IL, interleukin; LC-MS/MS, liquid chromatography-tandem mass spectrometry; LIF, leukemia inhibitory factor; MARS, multiple affinity removal system; NRS-2002, Nutrition Risk Screening-2002; PCA, principal component analysis; PNI, prognostic nutrition index; PTHrP, parathyroid hormone-related protein; ROC, receiver operating characteristic curve; SMI, skeletal muscle index.
1. Baracos VE, Martin L, Korc M, Guttridge DC, Fearon KCH. Cancer-associated cachexia. Nat Rev Dis Primers. (2018) 4:17105. doi: 10.1038/nrdp.2017.105
2. Ryan AM, Sullivan ES. Impact of musculoskeletal degradation on cancer outcomes and strategies for management in clinical practice. Proc Nutr Soc. (2021) 80:73–91. doi: 10.1017/S0029665120007855
3. Mochamat M, Cuhls H, Marinova M, Kaasa S, Stieber C, Conrad R, et al. A systematic review on the role of vitamins, minerals, proteins, and other supplements for the treatment of cachexia in cancer: a European Palliative Care Research Centre cachexia project. J Cachexia Sarcopenia Muscle. (2017) 8:25–39. doi: 10.1002/jcsm.12127
4. Saeteaw M, Sanguanboonyaphong P, Yoodee J, Craft K, Sawangjit R, Ngamphaiboon N, et al. Efficacy and safety of pharmacological cachexia interventions: systematic review and network meta-analysis. BMJ Support Palliat Care. (2021) 11:75–85. doi: 10.1136/bmjspcare-2020-002601
5. McGovern J, Dolan RD, Skipworth RJ, Laird BJ, McMillan DC. Cancer cachexia: a nutritional or a systemic inflammatory syndrome? Br J Cancer. (2022) 127:379–82. doi: 10.1038/s41416-022-01826-2
6. Argiles JM, Stemmler B, Lopez-Soriano FJ, Busquets S. Inter-tissue communication in cancer cachexia. Nat Rev Endocrinol. (2018) 15:9–20. doi: 10.1038/s41574-018-0123-0
7. Severinsen MCK, Pedersen BK. Muscle-organ crosstalk: the emerging roles of myokines. Endocr Rev. (2020) 41:594–609. doi: 10.1210/endrev/bnaa016
8. Huang Q, Wu M, Wu X, Zhang Y, Xia Y. Muscle-to-tumor crosstalk: The effect of exercise-induced myokine on cancer progression. Biochim Biophys Acta Rev Cancer. (2022) 1877:188761. doi: 10.1016/j.bbcan.2022.188761
9. Pin F, Bonewald LF, Bonetto A. Role of myokines and osteokines in cancer cachexia. Exp Biol Med (Maywood). (2021) 246:2118–27. doi: 10.1177/15353702211009213
10. Freire PP, Fernandez GJ, de Moraes D, Cury SS, Dal Pai-Silva M, Dos Reis PP, et al. The expression landscape of cachexia-inducing factors in human cancers. J Cachexia Sarcopenia Muscle. (2020) 11:947–61. doi: 10.1002/jcsm.12565
11. Nogueira-Ferreira R, Sousa-Nunes F, Leite-Moreira A, Moreira-Costa L, Vitorino R, Lara Santos L, et al. Cancer- and cardiac-induced cachexia: same fate through different inflammatory mediators? Inflammation Res. (2022) 71:771–83. doi: 10.1007/s00011-022-01586-y
12. Edge SB, Byrd DR, Compton CC, Fritz AG, Greene FL, Trotti A. AJCC Cancer Staging Manual. 7th ed. New York: Springer (2010).
13. Japanese Gastric Cancer A. Japanese gastric cancer treatment guidelines 2010 (ver. 3). Gastric Cancer. (2011) 14:113–23. doi: 10.1007/s10120-011-0042-4
14. Fearon K, Strasser F, Anker SD, Bosaeus I, Bruera E, Fainsinger RL, et al. Definition and classification of cancer cachexia: an international consensus. Lancet Oncol. (2011) 12:489–95. doi: 10.1016/S1470-2045(10)70218-7
15. Sierzega M, Chrzan R, Wiktorowicz M, Kolodziejczyk P, Richter P. Prognostic and predictive implications of sarcopenia in Western patients undergoing gastric resections for carcinoma of the stomach. J Surg Oncol. (2019) 120:473–82. doi: 10.1002/jso.v120.3
16. Manole E, Ceafalan LC, Popescu BO, Dumitru C, Bastian AE. Myokines as possible therapeutic targets in cancer cachexia. J Immunol Res. (2018) 2018:8260742. doi: 10.1155/2018/8260742
17. Perez-Riverol Y, Bai J, Bandla C, Garcia-Seisdedos D, Hewapathirana S, Kamatchinathan S, et al. The PRIDE database resources in 2022: a hub for mass spectrometry-based proteomics evidences. Nucleic Acids Res. (2022) 50:D543–52. doi: 10.1093/nar/gkab1038
18. Mi H, Ebert D, Muruganujan A, Mills C, Albou LP, Mushayamaha T, et al. PANTHER version 16: a revised family classification, tree-based classification tool, enhancer regions and extensive API. Nucleic Acids Res. (2021) 49:D394–403. doi: 10.1093/nar/gkaa1106
19. Szklarczyk D, Gable AL, Nastou KC, Lyon D, Kirsch R, Pyysalo S, et al. The STRING database in 2021: customizable protein-protein networks, and functional characterization of user-uploaded gene/measurement sets. Nucleic Acids Res. (2021) 49:D605–12. doi: 10.1093/nar/gkaa1074
20. Baazim H, Antonio-Herrera L, Bergthaler A. The interplay of immunology and cachexia in infection and cancer. Nat Rev Immunol. (2022) 22:309–21. doi: 10.1038/s41577-021-00624-w
21. Talbert EE, Guttridge DC. Emerging signaling mediators in the anorexia-cachexia syndrome of cancer. Trends Cancer. (2022) 8:397–403. doi: 10.1016/j.trecan.2022.01.004
22. Argiles JM, Busquets S, Stemmler B, Lopez-Soriano FJ. Cancer cachexia: understanding the molecular basis. Nat Rev Cancer. (2014) 14:754–62. doi: 10.1038/nrc3829
23. Yeom E, Yu K. Understanding the molecular basis of anorexia and tissue wasting in cancer cachexia. Exp Mol Med. (2022) 54:426–32. doi: 10.1038/s12276-022-00752-w
24. Webster JM, Kempen L, Hardy RS, Langen RCJ. Inflammation and skeletal muscle wasting during cachexia. Front Physiol. (2020) 11:597675. doi: 10.3389/fphys.2020.597675
25. Dolly A, Dumas JF, Servais S. Cancer cachexia and skeletal muscle atrophy in clinical studies: what do we really know? J Cachexia Sarcopenia Muscle. (2020) 11:1413–28. doi: 10.1002/jcsm.12633
26. Paval DR, Patton R, McDonald J, Skipworth RJE, Gallagher IJ, Laird BJ, et al. A systematic review examining the relationship between cytokines and cachexia in incurable cancer. J Cachexia Sarcopenia Muscle. (2022) 13:824–38. doi: 10.1002/jcsm.12912
27. Pedersen BK, Febbraio MA. Muscles, exercise and obesity: skeletal muscle as a secretory organ. Nat Rev Endocrinol. (2012) 8:457–65. doi: 10.1038/nrendo.2012.49
28. Daou HN. Exercise as an anti-inflammatory therapy for cancer cachexia: a focus on interleukin-6 regulation. Am J Physiol Regul Integr Comp Physiol. (2020) 318:R296–310. doi: 10.1152/ajpregu.00147.2019
29. Nielsen AR, Pedersen BK. The biological roles of exercise-induced cytokines: IL-6, IL-8, and IL-15. Appl Physiol Nutr Metab. (2007) 32:833–9. doi: 10.1139/H07-054
30. Thumser AE, Moore JB, Plant NJ. Fatty acid binding proteins: tissue-specific functions in health and disease. Curr Opin Clin Nutr Metab Care. (2014) 17:124–9. doi: 10.1097/MCO.0000000000000031
31. Pelsers MM, Hermens WT, Glatz JF. Fatty acid-binding proteins as plasma markers of tissue injury. Clin Chim Acta. (2005) 352:15–35. doi: 10.1016/j.cccn.2004.09.001
32. Du R, Li K, Guo K, Chen Z, Han L, Bian H. FSTL1: A double-edged sword in cancer development. Gene. (2024) 906:148263. doi: 10.1016/j.gene.2024.148263
33. Parfenova OK, Kukes VG, Grishin DV. Follistatin-like proteins: structure, functions and biomedical importance. Biomedicines. (2021) 9:999. doi: 10.3390/biomedicines9080999
34. Hamrick MW. Role of the cytokine-like hormone leptin in muscle-bone crosstalk with aging. J Bone Metab. (2017) 24:1–8. doi: 10.11005/jbm.2017.24.1.1
35. Pereira S, Cline DL, Glavas MM, Covey SD, Kieffer TJ. Tissue-specific effects of leptin on glucose and lipid metabolism. Endocr Rev. (2021) 42:1–28. doi: 10.1210/endrev/bnaa027
36. Argiles JM, Lopez-Soriano FJ, Busquets S. Mediators of cachexia in cancer patients. Nutrition. (2019) 66:11–5. doi: 10.1016/j.nut.2019.03.012
37. de Castro GS, Correia-Lima J, Simoes E, Orsso CE, Xiao J, Gama LR, et al. Myokines in treatment-naive patients with cancer-associated cachexia. Clin Nutr. (2021) 40:2443–55. doi: 10.1016/j.clnu.2020.10.050
38. Qi C, Song X, Wang H, Yan Y, Liu B. The role of exercise-induced myokines in promoting angiogenesis. Front Physiol. (2022) 13:981577. doi: 10.3389/fphys.2022.981577
39. McKillop IH, Girardi CA, Thompson KJ. Role of fatty acid binding proteins (FABPs) in cancer development and progression. Cell Signal. (2019) 62:109336. doi: 10.1016/j.cellsig.2019.06.001
40. Xu H, Diolintzi A, Storch J. Fatty acid-binding proteins: functional understanding and diagnostic implications. Curr Opin Clin Nutr Metab Care. (2019) 22:407–12. doi: 10.1097/MCO.0000000000000600
41. Porowski D, Wirkowska A, Hryniewiecka E, Wyzgal J, Pacholczyk M, Paczek L. Liver failure impairs the intrahepatic elimination of interleukin-6, tumor necrosis factor-alpha, hepatocyte growth factor, and transforming growth factor-beta. BioMed Res Int. (2015) 2015:934065. doi: 10.1155/2015/934065
42. Wiest R, Weigert J, Wanninger J, Neumeier M, Bauer S, Schmidhofer S, et al. Impaired hepatic removal of interleukin-6 in patients with liver cirrhosis. Cytokine. (2011) 53:178–83. doi: 10.1016/j.cyto.2010.06.013
43. Maeba T, Maeta H, Usuki H, Wakabayashi H, Tsubouchi T, Okada S, et al. Increase in portal blood interleukin-6 soon after the commencement of digestive surgery. Surg Today. (1996) 26:890–4. doi: 10.1007/BF00311790
44. Riche F, Dosquet C, Panis Y, Valleur P, Laisne MJ, Briard C, et al. Levels of portal and systemic blood cytokines after colectomy in patients with carcinoma or Crohn's disease. J Am Coll Surg. (1995) 180:718–24.
45. Palombo JD, Burke PA, Moldawer LL, Forse RA, Lewis WD, Jenkins RL, et al. Assessment of the cytokine response in liver donors at the time of organ procurement and association with allograft function after orthotopic transplantation. J Am Coll Surg. (1994) 179:209–19.
46. Dillon EL WT, Filley AR, Pulliam AB, Randolph KM, Danesi CP, Gilkison CR, et al. Cancer and associated therapies impact the skeletal muscle proteome. Front Physiol. (2022) 13:879263. doi: 10.3389/fphys.2022.879263
47. Zhou CJ, Zhang FM, Zhang FY, Yu Z, Chen XL, Shen X, et al. Sarcopenia: a new predictor of postoperative complications for elderly gastric cancer patients who underwent radical gastrectomy. J Surg Res. (2017) 211:137–46. doi: 10.1016/j.jss.2016.12.014
48. Ebhardt HA, Degen S, Tadini V, Schilb A, Johns N, Greig CA, et al. Comprehensive proteome analysis of human skeletal muscle in cachexia and sarcopenia: a pilot study. J Cachexia Sarcopenia Muscle. (2017) 8:567–82. doi: 10.1002/jcsm.12188
49. Gallagher IJ, Jacobi C, Tardif N, Rooyackers O, Fearon K. Omics/systems biology and cancer cachexia. Semin Cell Dev Biol. (2016) 54:92–103. doi: 10.1016/j.semcdb.2015.12.022
50. Twelkmeyer B, Tardif N, Rooyackers O. Omics and cachexia. Curr Opin Clin Nutr Metab Care. (2017) 20:181–5. doi: 10.1097/MCO.0000000000000363
Keywords: gastric cancer, cachexia, myokines, interleukins, fatty acid-binding protein 3, follistatin-like 1 protein
Citation: Sierzega M, Drabik A, Sanak M, Chrzan R and Richter P (2024) Dissecting the importance and origin of circulating myokines in gastric cancer cachexia. Front. Endocrinol. 15:1437197. doi: 10.3389/fendo.2024.1437197
Received: 23 May 2024; Accepted: 11 September 2024;
Published: 01 October 2024.
Edited by:
Eva Surmacz, Senior Advisor, Allysta Pharmaceuticals, Inc., United StatesReviewed by:
Brittany Counts, Oregon Health and Science University, United StatesCopyright © 2024 Sierzega, Drabik, Sanak, Chrzan and Richter. This is an open-access article distributed under the terms of the Creative Commons Attribution License (CC BY). The use, distribution or reproduction in other forums is permitted, provided the original author(s) and the copyright owner(s) are credited and that the original publication in this journal is cited, in accordance with accepted academic practice. No use, distribution or reproduction is permitted which does not comply with these terms.
*Correspondence: Marek Sierzega, bWFyZWsuc2llcnplZ2FAdWouZWR1LnBs
†These authors have contributed equally to this work and share first authorship
Disclaimer: All claims expressed in this article are solely those of the authors and do not necessarily represent those of their affiliated organizations, or those of the publisher, the editors and the reviewers. Any product that may be evaluated in this article or claim that may be made by its manufacturer is not guaranteed or endorsed by the publisher.
Research integrity at Frontiers
Learn more about the work of our research integrity team to safeguard the quality of each article we publish.