- 1Department of Medicine and Surgery, University of Parma, Parma, Italy
- 2Department of Pediatrics №2, Ivan Horbachevsky Ternopil National Medical University, Ternopil, Ukraine
- 3Unit of Paediatrics, University Hospital of Parma, P. Barilla Children’s Hospital, Parma, Italy
The increase in female reproductive disorders, such as polycystic ovary syndrome, endometriosis, and diminished ovarian reserve that lead to subfertility and infertility, has encouraged researchers to search and discover their underlying causes and risk factors. One of the crucial factors that may influence the increasing number of reproductive issues is environmental pollution, particularly exposure to man-made endocrine-disrupting chemicals (EDCs). EDCs can interfere with the ovarian microenvironment, impacting not only granulosa cell function but also other surrounding ovarian cells and follicular fluid (FF), which all play essential roles for oocyte development, maturation, and overall reproductive function. FF surrounds developing oocytes within an ovarian follicle and represents a dynamic milieu. EDCs are usually found in biological fluids, and FF is therefore of interest in this respect. This narrative review examines the current knowledge on specific classes of EDCs, including industrial chemicals, pesticides, and plasticizers, and their known effects on hormonal signaling pathways, gene expression, mitochondrial function, oxidative stress induction, and inflammation in FF. We describe the impact of EDCs on the development of reproductive disorders, oocyte quality, menstrual cycle regulation, and their effect on assisted reproductive technique outcomes. The potential transgenerational effects of EDCs on offspring through animal and first-human studies has been considered also. While significant progress has been made, the current understanding of EDCs’ effects on ovarian function, particularly in humans, remains limited, underscoring the need for further research to clarify actions and effects of EDCs in the ovary.
1 Introduction
A decrease in fertility rates and an increasing number of reproductive disorders are reported worldwide (1). According to the World Health Organization, infertility affects almost one out of every six people (2). Studies have shown that disorders in women such as endometriosis and polycystic ovary syndrome (PCOS) have a crucial impact on ovarian function and fertility; both have presented an alarming increase in the last decade (3). Furthermore, factors such as advanced maternal age, high body mass index, bad habits, and environmental exposure to pollutants, like endocrine-disrupting chemicals (EDCs), contribute to the modification of oocyte quality, changes in follicular fluid, and as a result, ovarian disorders, subfertility, and infertility (4).
One of the environmental factors that has been increasing with globalization in the last 50 years is exposure to EDCs (5, 6). EDCs are synthetic and/or natural chemicals that interfere with the body’s endocrine system, including ovaries. These chemicals can mimic hormones, disrupt hormonal signaling, block hormone receptors, or interfere with hormone synthesis, leading to a variety of adverse effects on reproductive health. They can be found in everyday products, such as personal care items, cosmetics, plastics, detergents and pesticides, electronics, building materials, etc. Exposure to EDCs can occur by ingestion, inhalation, and skin contact spreading to many organs in the human body through fluids, including ovaries, and placenta (5–7). Some studies have suggested that exposure to EDCs during critical periods of ovarian development, such as fetal development or puberty, can have long-lasting effects on ovarian function and reproductive health (8). There is scientific evidence that exposure to EDCs, such as bisphenol A (BPA), phthalates, and certain pesticides, can disrupt hormonal signaling, leading to irregularities in the menstrual cycle, fertility issues, and even to an increased risk of conditions such as polycystic ovary syndrome or ovarian cancer (9, 10). EDCs are usually found and easily assayed in human fluids such as urine, serum, and breast milk and in some tissues (mainly lysates), and cells (5–7). Some studies describe the impact of bisphenols, phthalates, and pesticides on oocyte and granulosa cells (11–14). However, there are only a few studies that have addressed the presence and composition of EDCs in follicular fluid.
Follicular fluid (FF) is a complex biological fluid surrounding the oocyte within the ovarian follicle and plays a supportive role in creating its microenvironment, supporting normal folliculogenesis and germ cell-somatic cell communication. It is secreted by granulosa cells in antral follicles and serves as a reservoir containing a diverse array of proteins, amino acids, lipids, hormones, growth factors, antioxidants, and metabolites essential for several cellular processes as protein synthesis, DNA replication, and mitochondrial function which are key for good fertilization and embryo development. Among the hormones contained in FF are estradiol, progesterone, human chorionic gonadotropin (hCG), follicle-stimulating hormone (FSH), luteinizing hormone (LH), growth hormone (GH), and insulin-like growth factors (IGF) system peptides. In addition, FF contains antioxidants such as superoxide dismutase and glutathione that help neutralize reactive oxygen species (ROS) generated during oocyte maturation. Maintaining optimal antioxidant levels in the follicular microenvironment is crucial for protecting oocytes from oxidative stress-induced damage and preserving their developmental competence. Growth factors such as epidermal growth factor (EGF), and vascular endothelial growth factor (VEGF) are also found in FF, and are important for cell proliferation, differentiation, angiogenesis, and tissue remodeling within the ovarian follicle (15, 16). Finally, FF contains high mobility group box 1 (HMGB1), which is a protein with cytokine activity associated with PCOS in women (17). Moreover, it contains extracellular vesicles containing cell-free RNA, particularly miRNAs (16, 17). The composition of FF thus mirrors the metabolic and hormonal state of the developing follicle and serves as a valuable substrate to assess oocyte quality. Consequently, studying FF provides insights that can optimize assisted reproductive technologies outcomes and enhance understanding of reproductive disorders (15, 18, 19). Therefore, EDCs exposure may contribute to the development of reproductive diseases.
The aim of this review is to explore current knowledge and the potential impact of EDCs in ovaries with a specific focus on FF. We discuss their mechanisms of action within the ovarian microenvironment, and the possible effects on oocyte quality, hormonal regulation, and reproductive outcomes.
2 Methods
The literature was researched using specific research strings in Pubmed, Scopus, and Mendeley via MeSH using specific keywords. The WHO and the European international official sites were also considered. The identified records were imported into Mendeley. After removing duplicates, reviewers separately screened the included papers found through the literature search by title and abstract, using the eligibility criteria, published from 1994 to March 2024. The following keywords were used for the search: endocrine-disrupting chemicals, endocrine disruptors, EDCs, bisphenol, phthalates, pesticides, dioxin, follicular fluid, ovaries, and reproductive health, which were differently matched for all required strings. A narrative synthesis was conducted to organize the search findings and summarize current knowledge to disclose knowledge gaps.
3 Mechanism of action of EDCs in follicular fluid and ovaries
Despite the limited number of studies on FF, some studies identified pesticides, industrial lubricants and solvents, phthalates, and flame retardants as the main EDCs present in ovaries and, particulary, follicular fluid (Table 1) (19–22). Most of these EDCs are able to bind to serum proteins such as albumin for efficient transport in the circulation (23, 24). Since albumin can cross the blood follicle barrier, it can be hypothesized that EDCs are readily transferred from the bloodstream into the developing follicle, where they exert their negative effects. As EDCs can affect initial and late phases of folliculogenesis, deplete the primordial follicle reserve through atresia or premature activation, reducing the number of follicles available for ovulation, inhibit FSH-dependent maturation, production of estrogen and LH (7, 25). However, most research describes the molecular effect of EDCs in ovaries, without considering their specific impact in FF. Therefore, in the following sections, we highlight relevant literature describing the numerous interconnected molecular mechanism that EDCs trigger mainly in the ovaries and partly in FF (Figure 1).
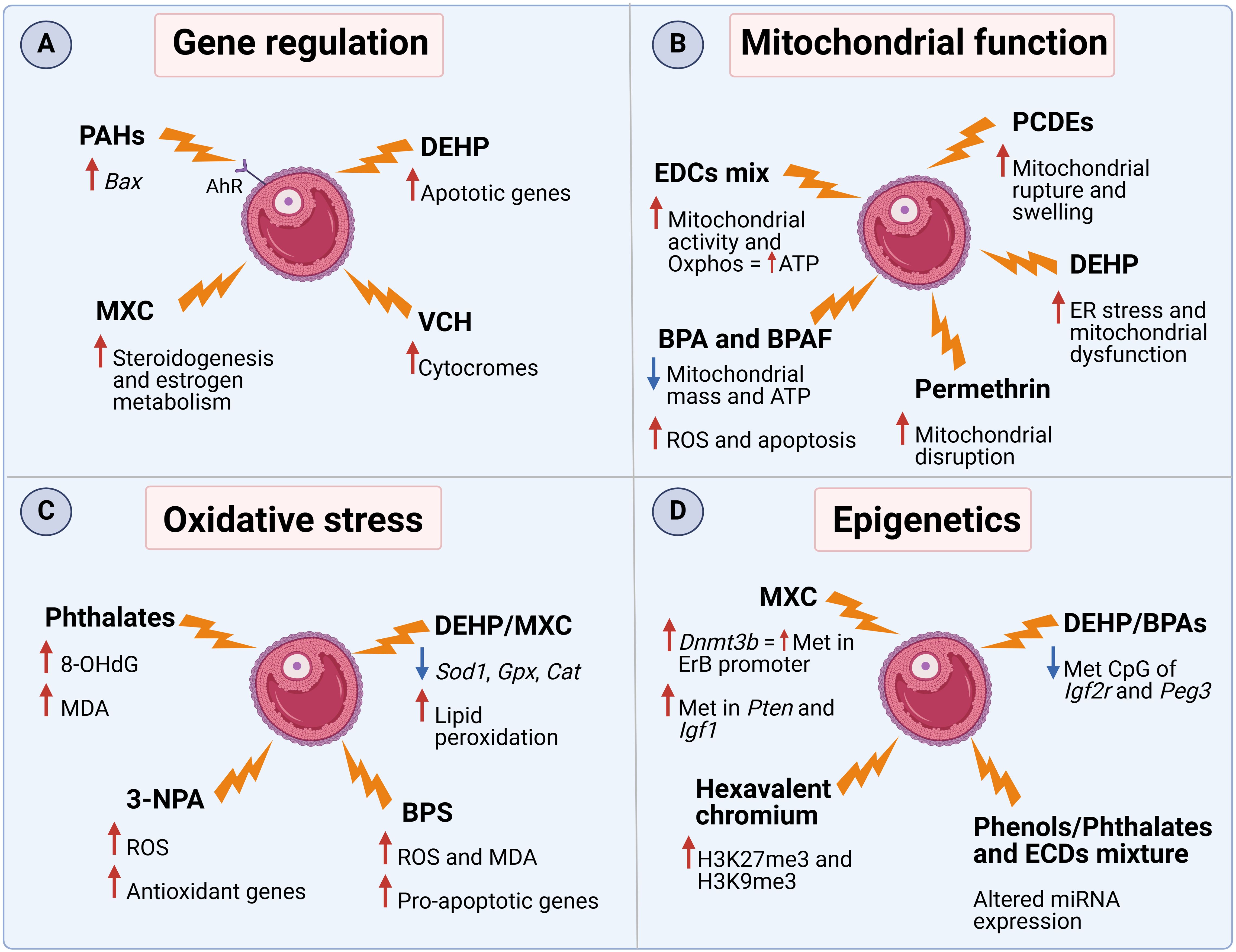
Figure 1. The mechanism of action of several EDCs in FF and ovaries. Molecular effects related to (A) gene expression, (B) mitochondrial function, (C) oxidative stress, and (D) epigenetics. PAHs (polycyclic aromatic hydrocarbons), DEHP (diethylhexyl phthalate), MXC (methoxychlor), VCH (4-vinylcyclohexene), PCDEs (polychlorinated diphenyl ethers), BPA (bisphenol A), 3-NPA (3-nitropropionic acid), BPS (bisphenol S). This Figure was created with BioRender.com.
3.1 Disruption of hormonal signaling pathways and changes in gene expression
As the structure of some EDCs’ is similar to that of endogenous steroid hormones, they are able to bind to aryl hydrocarbon receptors (AhR) or estrogen receptors (ERs), stimulating the secretion of 17β-estradiol (E2) (26). For example, it was demonstrated that a mix of EDCs, consisting of PFOA, PFOS, HCB, p,p’-DDE, and PCB153, was able to increase E2 secretion via both classical and non-classical ER pathways in a human granulosa tumor cell line (27). Furthermore, treatment of murine antral follicles with the pesticide methoxychlor (MXC) resulted in decreased expression of several steroidogenic enzymes as well as the estrogen metabolic enzyme Cyp1b1, resulting in diminished sex steroid hormone levels (28). Moreover, long-term exposure of granulosa cells to an EDCs mix (BPA [2 ng/mL], PCB 153 [70 pg/mL], B[a]P [1 ng/mL], and PFOS [100 pg/mL]) led to a reduction in the protein level of the key enzyme for estradiol synthesis, aromatase CYP19A1, with a subsequent decrease in estradiol production after 2 weeks of exposure (13). In addition to steroid synthesis, EDCs can influence other aspects of cell homeostasis. Activation of AhR by polycyclic aromatic hydrocarbons (PAHs), contaminants released via incomplete organic fuel combustion, drive the over-expression of Bax, a pro-apoptotic gene, resulting in accelerated oocyte depletion in female mice (29, 30). Similarly, exposure to diethylhexyl phthalate (DEHP), a plastic additive, increased the expression of several apoptotic genes in pre-pubertal murine ovaries (31). Another major contributor to ovotoxicity is 4-Vinylcyclohexene (VCH), a compound found in cigarette smoke. It was demonstrated that VCH administration in mice increases the expression of several ovarian cytochrome isoforms, which are responsible for the conversion of VCH into its more toxic metabolite, responsible for destruction of immature, small preantral follicles (32).
3.2 Interference with mitochondrial function
Cholesterol shuttling to mitochondria is essential for steroid hormone synthesis (33, 34). Polychlorinated diphenyl ethers (PCDEs), found mainly in aquatic samples, have been reported to interfere with many cellular organelles in Zebrafish ovarian cells, such as the endoplasmic reticulum (ER) and mitochondria, causing ruptures and swelling (35). Likewise, in rats treated with the pesticide permethrin, a negative effect of EDCs was observed on ovarian mitochondria morphology, resulting in organelle disruption (36). Another study involving the treatment of human granulosa cells with a mixture of several EDCs (PFOA, PFOS, HCB, p,p’-DDE, and PCB153) showed changes in mitochondrial shape associated with increased activity with a shift toward oxidative phosphorylation, that translated into increased ATP content (37). In addition, the co-exposure to BPA and bisphenol AF (BPAF) in human granulosa cells revealed reduced mitochondrial mass and ATP production, damage to the mitochondrial respiratory chain, and increased ROS production with consequent induction of apoptosis mechanisms (38). Finally, female mice exposed to DEHP showed ER stress and mitochondrial dysfunction with reduction of membrane potential and induction of apoptosis (39). It was then demonstrated that these changes were due to modifications in the structure of the ER membranes and the suppression of oxidative phosphorylation pathways (39). These data suggest that the altered mitochondrial morphology and function might be the cause of impaired steroid production and increased follicular atresia after EDC exposure.
3.3 Induction of oxidative stress and inflammation
An additional mechanism that EDCs exploit to interfere with ovarian functions is activating ROS production. For example, exposure to DEHP or MXC was able to decrease the expression of Sod1, Gpx and Cat, key antioxidant enzymes, in murine ovarian somatic cells and antral follicles respectively (31, 40). The production of ROS after exposure to MXC was associated with a higher rate of lipid peroxidation, resulting in increased ovarian toxicity in rats (41). In addition, the presence of several phthalate metabolites in the FF of women undergoing in vitro fertilization was positively associated with 8-hydroxy-2′-deoxyguanosine (8-OHdG), an oxidized derivative of deoxyguanosine, and malondialdehyde (MDA), both used as oxidative stress biomarkers (42). Accumulation of MDA and ROS were also found in the offspring of BPS-treated ICR female mice, accompanied by increased expression levels of pro-apoptotic markers (Bax, Caspase 3, and Caspase 9) and decreased expression levels of anti-apoptotic marker (Bcl2) (43). In addition, BPS also activated the mTOR pathway, subsequently down-regulating autophagy (43). Similarly, also rats injected with atrazine, a common herbicide, showed increased ovarian levels of MDA, reduction in the activity of ovarian GST and SOD enzymes, higher ovarian expression of inflammatory cytokines and pro-apoptoric markers (44). Furthermore, exposure to 3-nitropropionic acid (3-NPA), a mycotoxin, increased ROS concentration in granulosa cells, despite the increase in the aforementioned antioxidant genes (45).
3.4 Epigenetic regulation
A third way that EDCs exploit to disrupt ovarian homeostasis is the modulation through epigenetic modifications. It was shown that DEHP or BPA exposure was able to reduce the number of methylated CpG sites of Igf2r and Peg3 in murine germ cells (46, 47). These genes are representatives of imprinted genes during gametogenesis and the modification was also maintained in the offspring. In addition, temporary exposure to MXC during the development of the rat fetus and newborn led to higher expression of Dnmt3b, a DNA methyltransferase, that causes increased methylation of the ERβ promoter regions (48). Furthermore, exposure to hexavalent chromium in rats increased H3K27me3 and H3K9me3, heterochromatin-associated repressive histone markers, whereas decreased H3K9ac and H3K27ac, acetylation marks in histones H3 (49). A more recent study suggested that other genes are affected by MXC-induced methylation. Key pathways such as PTEN signaling, IGF-1 signaling, or rapid estrogen signaling were hypermethylated and, therefore, suppressed, resulting in dysregulated early folliculogenesis as well as follicular maturation and ovulation in rats (50). Furthermore, a recent publication associated urine metabolites of several EDCs with changes in the expression of miRNAs related to follicular development and oocyte competence (51). Finally, a follow up study assessed the correlation between EDC concentrations in FF and miRNA changes. It was observed that the expression of 39 miRNAs correlated with MEP, MCOMOP, MCOMHP, MBzP and/or with mECPP content (52). Interestingly, these miRNAs were associated with pathways involved in oocyte development, oocyte maturation and fertilization, suggesting that miRNAs play an important role in regulating female fertility (52).
In summary, the above mentioned studies provide valuable insights into the mechanisms underlying EDC-induced ovarian dysfunction, there remains a notable gap in our understanding of how concurrent exposure to multiple EDCs may exacerbate adverse outcomes. Future investigations incorporating the complexities of EDC mixtures are essential for a comprehensive assessment of their impact on ovarian health.
4 Effects of EDCs on oocyte quality and development
4.1 Implications for ovulation, PCOS, fertility and endometriosis
Exposure to EDCs can cause changes in the female reproductive system. Higher and persistent exposure to EDCs can modify the follicular micro-environment, affect oocyte quality, and may trigger disease development, such as PCOS, endometriosis, fertility and ovulation disorders (7, 20, 53).
PCOS is clinically characterized by hormonal changes, as hyperandrogenism, chronic oligo/anovulation, characteristic ovarian morphology on gynecological ultrasound, increased anti‐Müllerian hormone (AMH) levels, and metabolic disorders such as obesity, insulin resistance, and atherogenic dyslipidemia. The etiology of PCOS is not fully understood, but potential contributing factors may include exposure to high levels of AMH, androgens and EDCs during the prenatal period (54–56). For example, a significant correlation was described between BPA and PCOS (56, 57). BPA is a xenoestrogen that mimics the activity of 17-β-estradiol and can block its feedback pathway at the hypothalamic-pituitary and ovarian levels, causing inadequate hormone production, menstrual cycle abnormalities, infertility and impaired development and functioning of the reproductive system (55, 58). Dysregulation of gene expression in the hypothalamic-pituitary region caused by BPA exposure can lead to an excessive release of GnRH (59). This, in turn, induces a continuous increase in LH and a reduction in FSH secretion. The disrupted FSH/LH balance impairs ovarian follicle development, while the elevated LH levels contribute to increased ovarian androgen production (60, 61). Additionally, BPA may alter the gene expression of ovarian steroidogenesis genes, such as 17α-hydroxylase, downregulating estradiol synthesis and contributing to hyperandrogenism and ovulatory dysfunction (62). In addition, another recent study observed an increased concentration of BPA in FF in women with PCOS (63). This dose-dependent increase in the EDC was associated with a downregulation of aromatase, a key enzyme in the aromatization of androgens into estrogens, in granulosa cells, leading to reduced E2 synthesis and hyperandrogenism (63).
Similarly, other studies have shown that exposure to PFAS and DEHP is associated with clinical features of PCOS, such as irregular menstrual cycles or polycystic ovaries (64–66). A study comparing women with PCOS undergoing in-vitro fertilization (IVF) to infertile women without PCOS found that the former had significantly higher concentrations of DEPH in FF, leading to lower clinical pregnancy rate after IVF. Cell culture studies revealed that in vitro DEHP treatment on primary-cultured human granulosa cells and a steroidogenic human granulosa-like tumor cell line resulted in altered steroid production, decreased cell viability and proliferation, and increased apoptosis (57). This association is likely due to the action of the DEHP metabolite, mono(2-ethylhexyl) phthalate (MEHP), which acts on granulosa cells suppressing estradiol production, leading to anovulation (64, 65).
Furthermore, several studies have investigated the potential link between exposure to environmental pollutants and the development of endometriosis, a gynecological condition characterized by the presence of endometrial-like tissue outside the uterus. Studies have shown a positive correlation between endometriosis and exposure to organochlorine pesticides such as DDT and chlordane (67, 68), lead (69), and specific polychlorinated biphenyls (PCBs) (68, 70).
EDCs, as bisphenols and pesticides (DDE, DDT), may promote the crosstalk between cancer cells and surrounding stromal cells, increasing tumor development and invasion by influencing tumor microenvironment via mechanisms such as enhanced aromatase expression and growth factor release that activate estrogen-related pathways (71). Moreover, they can affect tumor growth by targeting protein Receptor for Activated C Kinase 1 (RACK1), which is the bridge between cancer progression and the immune system. EDCs modulate RACK1 expression thus promoting tumor growth by enhancing cell cycle progression and apoptosis regulation BPA has been shown to activate signaling pathways that facilitate cancer cell migration by increasing RACK1’s interaction with focal adhesion kinase, a key protein involved in cell adhesion and migration. Conversely, DDT may downregulate RACK1 expression resulting in decreased immunological responses and reduced production of pro-inflammatory cytokines (71).
4.2 EDCs and assisted reproductive technologies (ART)
The prevalence of reproductive health problems is increasing worldwide, together with assisted reproductive techniques (ARTs) (72). Despite the increased use of ART, the Assisted Reproductive Technology Surveillance report carried out in the United States in 2016 showed that success rates of live births remained similar (73). Several studies have investigated the link between EDCs and quality of embryos (20, 21, 74–76) and pregnancy rates (77–80). However, these studies were usually monocentric and failed to distinguish between factors affecting the mother’s ability to conceive and the impact on the father’s sperm health. Furthermore, these studies often examined the effects of individual EDCs, whereas real-world exposure typically involves a complex mixture of these chemicals. To address these limitations, a recent multicenter epidemiological study (81) investigated the link between EDCs and female fertility in medically assisted conception. The authors observed an inverse correlation of phthalates (82), DEHP metabolites and methylparaben, or a mixture of the two, with ovarian reserve (83, 84).
Supporting the notion that EDC mixtures might have a stronger impact on female fertility than single EDCs, a case-control study by Tian et al. investigated the effects of a mixture of 21 environmental contaminants (parabens, phenol, phthalates, and PFAS) found in follicular fluid of women who underwent IVF or intracytoplasmic sperm injection therapy. The study found that mixed and single EDCs were associated with an increased risk of diminished ovarian reserve. Moreover, EDC mixtures had a potentiating effect, meaning that the combined effect on reducing ovarian reserve was greater than the effect of each individual EDC alone. This reduction in ovarian reserve was particularly evident in women under 30 years of age (84).
Exposure to EDCs, which has also been linked to an increased risk of miscarriage. Some studies have demonstrated that individuals exposed to pesticides and PCBs may have a thinner endometrial lining (85, 86), making implantation more difficult (20, 79, 86, 87). Also, BPA has been associated with an increase in aneuploid oocytes, which can lead to miscarriage (86, 88). A link with miscarriage has also been observed for phthalates, bisphenols, benzophenones, and PCBs (85–89).
4.3 Potential transgenerational effects on offspring health and fertility
A large body of research has demonstrated that six major classes of EDCs – phthalates, phenols, perfluorinated compounds, flame retardants, PCBs, and organochlorine pesticides – can cross the placental barrier (5, 6, 90). Despite this, few researchers have investigated the link between prenatal exposure to EDCs and potential adverse effects on fetal development, immediate postnatal health, and fertility. Several studies in mice have observed a correlation between prenatal exposure to DEHP and BPA and the effects on future generations (91, 92). Data suggest that prenatal and ancestral exposure to DEHP negatively impacts reproductive outcomes in F1-F3 generations of mice, by accelerating puberty, disrupting estrous cycle, and decreasing pregnancy rate and fertility index (91). Likewise, studies of in utero bisphenol treatment in mice investigated the negative outcomes of EDC exposure such as in three subsequent generations. Their findings evidence that this EDC can influence pubertal onset and reproductive capacity throughout several generations (93, 94).
Fewer publications are available on transgenerational effects in humans. Urinary BPA and BPS concentrations in mothers prior to conception have been shown to be associated to an increased risk of premature birth (95). Few, if any, studies have examined the effects of prenatal EDCs exposure in human offspring. The limited research available focuses on the effects of prenatal exposure on neonatal health. Other studies have shown a negative correlation between prenatal BPA exposure and gestational age (95), while others have investigated a correlation with an increased incidence of systemic anomalies. In particular, prenatal exposure to phthalates appears to be associated with a reduced ano-genital distance in males, an association that does not seem to be confirmed in females (96). No clear correlations have been found between phthalate exposure and cryptorchidism (97), and controversial results have also been found for hypospadias (98, 99).
Further research is needed to determine whether there is a threshold of prenatal exposure above which the harmful effects of EDCs on offspring manifest. More studies are also required to understand the mechanisms by which EDCs influence offspring reproductive health and whether epigenetic mechanisms may be involved in the transmission.
A summary of the effects and outcomes of endocrine-disrupting chemicals in the follicular fluid of women undergoing ART is presented in Table 2.
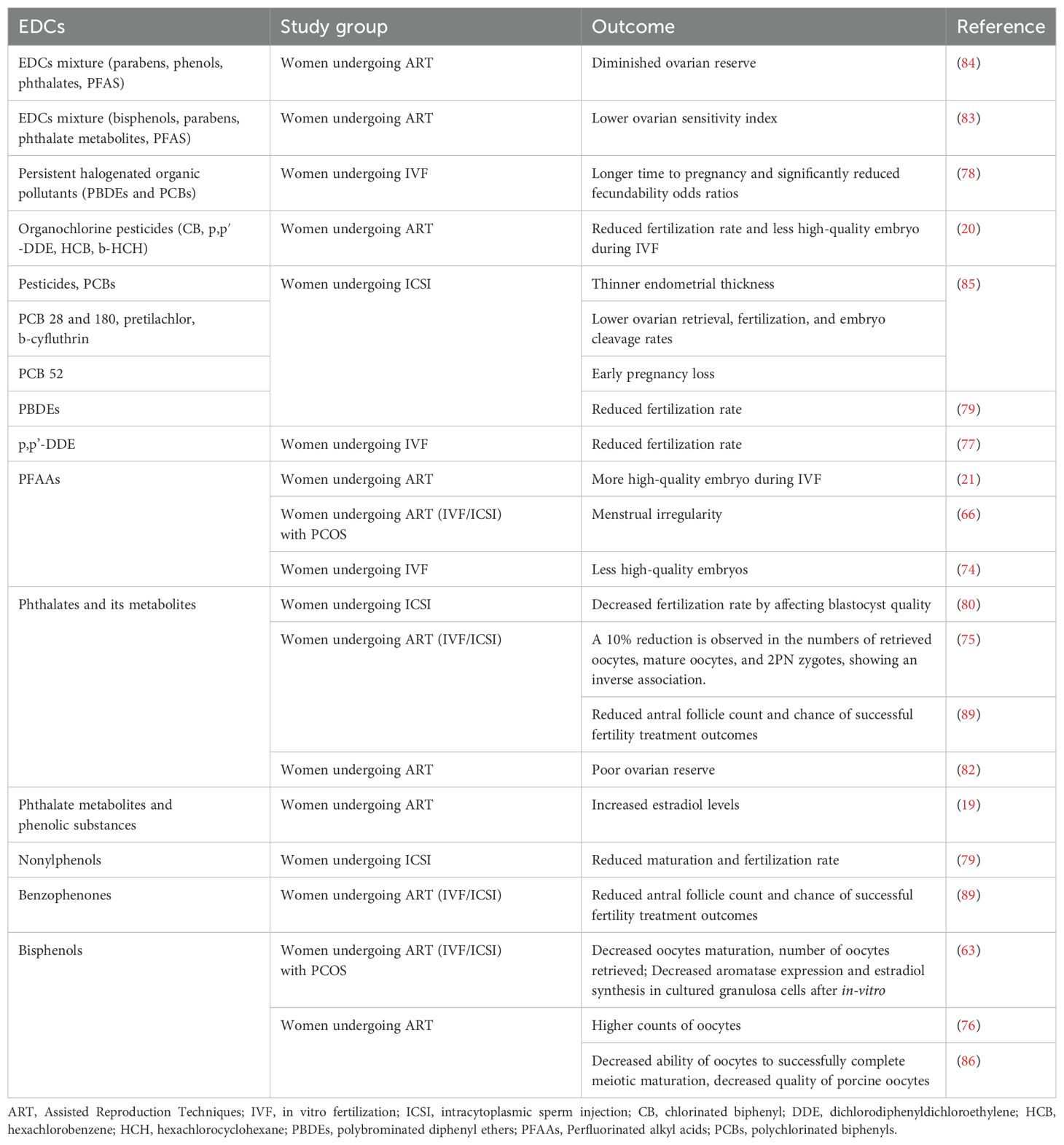
Table 2. Human studies on the effects/associations of endocrine-disrupting chemicals in follicular fluid.
5 Conclusions and further perspectives
A good number of studies have suggested that EDCs are found in the ovary and FF. Mainly, studies in animals have proven that exposure is harmful and is affecting negatively human fertility, ovarian reserve, and function, and exposure to mixtures seems to worsen these effects. EDCs may alter mitochondrial morphology and function, epigenetics, activate oxidative stress, apoptosis and disrupt hormonal signaling in ovaries and FF. However, current knowledge on this topic is yet scarce, particularly in humans. Further studies are urgently required to investigate the causes of diseases and the mechanisms implicatedUnderstanding how EDCs alter FF composition and function could offer crucial insights into the mechanisms driving reproductive disorders, like PCOS, endometriosis, and infertility. Developing this knowledge is crucial not only for mitigating these adverse effects but also for exploring ways to reverse EDC-induced epigenetic changes, potentially leading to improved reproductive outcomes. In vitro mechanistic and in vivo studies with the aid of omics are required. Addressing the gaps is essential for developing more effective regulatory strategies and interventions to safeguard female reproductive function against the deleterious effects of EDC exposure.
Author contributions
A-MS: Writing – review & editing, Writing – original draft, Formal analysis, Conceptualization. VB: Writing – review & editing, Writing – original draft, Visualization, Formal analysis. VD: Writing – review & editing, Writing – original draft, Formal analysis. SE: Writing – review & editing. MS: Writing – review & editing, Supervision, Conceptualization.
Funding
The author(s) declare that no financial support was received for the research, authorship, and/or publication of this article.
Conflict of interest
The authors declare that the research was conducted in the absence of any commercial or financial relationships that could be construed as a potential conflict of interest.
Publisher’s note
All claims expressed in this article are solely those of the authors and do not necessarily represent those of their affiliated organizations, or those of the publisher, the editors and the reviewers. Any product that may be evaluated in this article, or claim that may be made by its manufacturer, is not guaranteed or endorsed by the publisher.
Abbreviations
EDCs, endocrine-disrupting chemicals; FF, follicular fluid; PCOS, polycystic ovary syndrome; FSH, follicle-stimulating hormone; LH, luteinizing hormone; GH, growth hormone; GnRH, Gonadotropin-releasing hormone; IGF, insulin-like growth factors; BPA, bisphenol A; AhR, aryl hydrocarbon receptors; ER, endoplasmic reticulum; ERs, estrogen receptors; E2, 17β-estradiol; ARTs, assisted reproductive techniques; PAHs, polycyclic aromatic hydrocarbons; DEHP, diethylhexyl phthalate; MEP, monoethyl phthalate; MEHP, mono(2-ethylhexyl) phthalate; MBzP, monobenzyl phthalate; MCOMOP, mono (7-COOH-2-methyloctyl) phthalate; MCOMHP, mono (6-COOH-2-methylheptyl) phthalate; mECPP, mono-2-ethyl-5-carboxypentyl phthalate; VCH, 4-Vinylcyclohexene; MXC, methoxychlor; PCDEs, polychlorinated diphenyl ethers; 3-NPA, 3-nitropropionic acid; B[a]P, Benzo(a)pyrene; PCBs, polychlorinated biphenyls; PFAS, per- and polyfluoroalkyl substances; PFAAs, perfluorinated alkyl acids; PFOS, perfluorooctane sulfonic acid; PCDEs, Polychlorinated diphenyl ethers; PFOA, perfluorooctanoic acid; CB, chlorinated biphenyl; DDE, p,p’-DDE, dichlorodiphenyldichloroethylene; DDT, dichlorodiphenyltrichloroethane; HCB, hexachlorobenzene; HCH, hexachlorocyclohexane; PBDEs, polybrominated diphenyl ethers; AMH, anti‐müllerian hormone; IVF, in vitro fertilization; ICSI, intracytoplasmic sperm injection; 8-OHdG, 8-hydroxy-2′-deoxyguanosine; MDA, malondialdehyde.
References
1. GBD 2021 Fertility and Forecasting Collaborators. Global fertility in 204 countries and territories 1950-2021, with forecasts to 2100: a comprehensive demographic analysis for the Global Burden of Disease Study 2021. Lancet Published Online March. (2024) 19:2024. doi: 10.1016/S0140-6736(24)00550-6
2. The World Health Organization. Infertility prevalence estimates 1990–2021. Geneva: World Health Organization (2023). Licence: CC BY-NC-SA 3.0 IGO.
3. Mercuri ND, Cox BJ. The need for more research into reproductive health and disease. Elife. (2022) 11:e75061. doi: 10.7554/eLife.75061
4. Bala R, Singh V, Rajender S, Singh K. Environment, lifestyle, and female infertility. Reprod Sci. (2021) 28:617–38. doi: 10.1007/s43032-020-00279-3
5. Bergman A. State of the science of endocrine disrupting chemicals - 2012 an assessment of the state of the science of endocrine disruptors. Geneva: UNEP/WHO (2013). United Nations Environment Programme & World Health Organization.
6. ECHA/EFSA. Guidance for the identification of endocrine disruptors in the context of Regulations (EU) No 528/2012 and (EC) No 1107/2009. EFSA J. (2018) 16:5311.
7. Land KL, Miller FG, Fugate AC, Hannon PR. The effects of endocrine-disrupting chemicals on ovarian- and ovulation-related fertility outcomes. Mol Reprod Dev. (2022) 89:608–31. doi: 10.1002/mrd.23652
8. Zama AM, Uzumcu M. Effects of endocrine-disrupting chemicals on female reproductive health. Open Biotechnol J. (2016) 10:54–75. doi: 10.2174/1874070701610010054
9. Panagopoulos P, Mavrogianni D, Christodoulaki C, Drakaki E, Chrelias G, Panagiotopoulos D, et al. Effects of endocrine disrupting compounds on female fertility. Best Pract Res Clin Obstet Gynaecol. (2023) 88:102347. doi: 10.1016/j.bpobgyn.2023.102347
10. Exposure to hazardous chemicals at work and resulting health impacts: A global review International Labour Office. Geneva: ILO (2021).
11. Cabry R, Merviel P, Madkour A, Lefranc E, Scheffler F, Desailloud R, et al. The impact of endocrine disruptor chemicals on oocyte/embryo and clinical outcomes in IVF. Endocr Connect. (2020) 9:R134–42. doi: 10.1530/EC-20-0135
12. Palmerini MG, Zhurabekova G, Balmagambetova A, Nottola SA, Miglietta S, Belli M, et al. The pesticide Lindane induces dose-dependent damage to granulosa cells in an in vitro culture. Reprod Biol. (2017) 17:349–56. doi: 10.1016/j.repbio.2017.09.008
13. Samardzija Nenadov D, Tesic B, Fa S, Pogrmic-Majkic K, Kokai D, Stanic B, et al. Long-term in vitro exposure of human granulosa cells to the mixture of endocrine disrupting chemicals found in human follicular fluid disrupts steroidogenesis. Toxicol In Vitro. (2022) 79:105302. doi: 10.1016/j.tiv.2021.105302
14. Nenadov DS, Pogrmic-Majkic K, Tesic B, Kokai D, Fa Nedeljkovic S, Stanic B, et al. Impact of in vitro long-term low-level DEHP exposure on gene expression profile in human granulosa cells. Cells. (2022) 11:2304. doi: 10.3390/cells11152304
15. Basuino L, Silveira CF Jr. Human follicular fluid and effects on reproduction. JBRA Assist Reprod. (2016) 20:38–40. doi: 10.5935/1518-0557.20160009
16. Del Collado M, Andrade GM, Meirelles FV, da Silveira JC, Perecin F. Contributions from the ovarian follicular environment to oocyte function. Anim Reprod. (2018) 15:261–70. doi: 10.21451/1984-3143-AR2018-0082
17. Cirillo F, Catellani C, Lazzeroni P, Sartori C, Nicoli A, Amarri S, et al. MiRNAs regulating insulin sensitivity are dysregulated in polycystic ovary syndrome (PCOS) ovaries and are associated with markers of inflammation and insulin sensitivity. Front Endocrinol (Lausanne). (2019) 10:879. doi: 10.3389/fendo.2019.00879
18. Brinca AT, Ramalhinho AC, Sousa Â, Oliani AH, Breitenfeld L, Passarinha LA, et al. Follicular fluid: A powerful tool for the understanding and diagnosis of polycystic ovary syndrome. Biomedicines. (2022) 10:1254. doi: 10.3390/biomedicines10061254
19. Hoffmann-Dishon N, Barnett-Itzhaki Z, Zalko D, Hemi R, Farzam N, Hauser R, et al. Endocrine-disrupting chemical concentrations in follicular fluid and follicular reproductive hormone levels. J Assist Reprod Genet. (2024) 41:1637–42. doi: 10.1007/s10815-024-03101-0
20. Petro EML, Leroy JLMR, Covaci A, Fransen E, De Neubourg D, Dirtu AC, et al. Endocrine-disrupting chemicals in human follicular fluid impair in vitro oocyte developmental competence. Hum Reprod. (2012) 27:1025–33. doi: 10.1093/humrep/der448
21. Petro EML, D’Hollander W, Covaci A, Bervoets L, Fransen E, De Neubourg D, et al. Perfluoroalkyl acid contamination of follicular fluid and its consequence for in vitro oocyte developmental competence. Sci Total Environ. (2014) 496:282–8. doi: 10.1016/j.scitotenv.2014.07.028
22. Du Y, Guo N, Wang Y, Teng X, Hua X, Deng T, et al. Follicular fluid concentrations of phthalate metabolites are associated with altered intrafollicular reproductive hormones in women undergoing in vitro fertilization. Fertility and Sterility. (2019) 111(5):953–61. doi: 10.1016/j.fertnstert.2019.01.021
23. Równicka-Zubik J, Sułkowski L, Toborek M. Interactions of PCBs with human serum albumin: in vitro spectroscopic study. Spectrochim Acta A Mol Biomol Spectrosc. (2014) 124:632–7. doi: 10.1016/j.saa.2014.01.069
24. Jones PD, Hu W, De Coen W, Newsted JL, Giesy JP. Binding of perfluorinated fatty acids to serum proteins. Environ Toxicol Chem. (2003) 22:2639–49. doi: 10.1897/02-553
25. Hannon PR, Brannick KE, Wang W, Flaws JA. Mono(2-ethylhexyl) phthalate accelerates early folliculogenesis and inhibits steroidogenesis in cultured mouse whole ovaries and antral follicles1. Biol Reprod. (2015) 92:120. doi: 10.1095/biolreprod.115.129148
26. La Merrill MA, Vandenberg LN, Smith MT, Goodson W, Browne P, Patisaul HB, et al. Consensus on the key characteristics of endocrine-disrupting chemicals as a basis for hazard identification. Nat Rev Endocrinol. (2020) 16:45–57. doi: 10.1038/s41574-019-0273-8
27. Gogola J, Hoffmann M, Nimpsz S, Ptak A. Disruption of 17β-estradiol secretion by persistent organic pollutants present in human follicular fluid is dependent on the potential of ovarian granulosa tumor cell lines to metabolize estrogen. Mol Cell Endocrinol. (2020) 503:110698. doi: 10.1016/j.mce.2019.110698
28. Basavarajappa MS, Craig ZR, Hernández-Ochoa I, Paulose T, Leslie TC, Flaws JA. Methoxychlor reduces estradiol levels by altering steroidogenesis and metabolism in mouse antral follicles in vitro. Toxicol Appl Pharmacol. (2011) 253:161–9. doi: 10.1016/j.taap.2011.04.007
29. Pru JK, Kaneko-Tarui T, Jurisicova A, Kashiwagi A, Selesniemi K, Tilly JL. Induction of proapoptotic gene expression and recruitment of p53 herald ovarian follicle loss caused by polycyclic aromatic hydrocarbons. Reprod Sci. (2009) 16:347–56. doi: 10.1177/1933719108327596
30. Matikainen T, Perez GI, Jurisicova A, Pru JK, Schlezinger JJ, Ryu HY, et al. Aromatic hydrocarbon receptor-driven Bax gene expression is required for premature ovarian failure caused by biohazardous environmental chemicals. Nat Genet. (2001) 28:355–60. doi: 10.1038/ng575
31. Li L, Liu JC, Lai FN, Liu HQ, Zhang XF, Dyce PW, et al. Di (2-ethylhexyl) phthalate exposure impairs growth of antral follicle in mice. PLoS One. (2016) 11:e0148350. doi: 10.1371/journal.pone.0148350
32. Cannady EA. Expression and activity of cytochromes P450 2E1, 2A, and 2B in the mouse ovary: the effect of 4-vinylcyclohexene and its diepoxide metabolite. Toxicol Sci. (2003) 73:423–30. doi: 10.1093/toxsci/kfg077
33. Miller WL. Steroid hormone synthesis in mitochondria. Mol Cell Endocrinol. (2013) 379:62–73. doi: 10.1016/j.mce.2013.04.014
34. Miller WL. Disorders in the initial steps of steroid hormone synthesis. J Steroid Biochem Mol Biol. (2017) 165:18–37. doi: 10.1016/j.jsbmb.2016.03.009
35. Ye C, Xiong W, Shi S, Shi J, Yang W, Zhang X. Biomarker responses, gene expression alterations, and histological changes in zebrafish (Danio rerio) after in vivo exposure to polychlorinated diphenyl ethers. Front Physiol. (2022) 13:907906. doi: 10.3389/fphys.2022.907906
36. Kotil T, Yön ND. The effects of permethrin on rat ovarian tissue morphology. Exp Toxicol Pathol. (2015) 67:279–85. doi: 10.1016/j.etp.2015.01.005
37. Krawczyk K, Marynowicz W, Gogola-Mruk J, Jakubowska K, Tworzydło W, Opydo-Chanek M, et al. A mixture of persistent organic pollutants detected in human follicular fluid increases progesterone secretion and mitochondrial activity in human granulosa HGrC1 cells. Reprod Toxicol Elmsford N. (2021) 104:114–24. doi: 10.1016/j.reprotox.2021.07.009
38. Huang M, Xu G, Li M, Yang M. Bisphenol A and bisphenol AF co-exposure induced apoptosis of human ovarian granulosa cells via mitochondrial dysfunction. Food Chem Toxicol. (2024) 191:114894. doi: 10.1016/j.fct.2024.114894
39. Guo Q, Deng T, Du Y, Yao W, Tian W, Liao H, et al. Impact of DEHP on mitochondria-associated endoplasmic reticulum membranes and reproductive toxicity in ovary. Ecotoxicol Environ Saf. (2024) 282:116679. doi: 10.1016/j.ecoenv.2024.116679
40. Gupta RK, Miller KP, Babus JK, Flaws JA. Methoxychlor inhibits growth and induces atresia of antral follicles through an oxidative stress pathway. Toxicol Sci. (2006) 93:382–9. doi: 10.1093/toxsci/kfl052
41. El-Sharkawy EE, Kames AOG, Sayed SM, Nisr NAEL, Wahba NM, Elsherif WM, et al. The ameliorative effect of propolis against methoxychlor induced ovarian toxicity in rat. Exp Toxicol Pathol. (2014) 66:415–21. doi: 10.1016/j.etp.2014.06.003
42. Yuan XQ, Du YY, Liu C, Guo N, Teng XM, Hua X, et al. Phthalate metabolites and biomarkers of oxidative stress in the follicular fluid of women undergoing in vitro fertilization. Sci Total Environ. (2020) 738:139834. doi: 10.1016/j.scitotenv.2020.139834
43. Gao Z, He W, Liu Y, Gao Y, Fan W, Luo Y, et al. Perinatal bisphenol S exposure exacerbates the oxidative burden and apoptosis in neonatal ovaries by suppressing the mTOR/autophagy axis. Environ Pollut. (2024) 349:123939. doi: 10.1016/j.envpol.2024.123939
44. Zhao J, Huang G, Fu Y, Lou Z, Yu H, Wang W, et al. Exposure to atrazine stimulates progesterone secretion and induces oxidative stress, inflammation, and apoptosis in the ovary of pseudopregnant rats. Chemosphere. (2024) 356:141906. doi: 10.1016/j.chemosphere.2024.141906
45. Zhang JQ, Shen M, Zhu CC, Yu FX, Liu ZQ, Ally N, et al. 3-nitropropionic acid induces ovarian oxidative stress and impairs follicle in mouse. PLoS One. (2014) 9:e86589. doi: 10.1371/journal.pone.0086589
46. Li L, Zhang T, Qin XS, Ge W, Ma HG, Sun LL, et al. Exposure to diethylhexyl phthalate (DEHP) results in a heritable modification of imprint genes DNA methylation in mouse oocytes. Mol Biol Rep. (2014) 41:1227–35. doi: 10.1007/s11033-013-2967-7
47. Chao HH, Zhang XF, Chen B, Pan B, Zhang LJ, Li L, et al. Bisphenol A exposure modifies methylation of imprinted genes in mouse oocytes via the estrogen receptor signaling pathway. Histochem Cell Biol. (2012) 137:249–59. doi: 10.1007/s00418-011-0894-z
48. Zama AM, Uzumcu M. Fetal and neonatal exposure to the endocrine disruptor methoxychlor causes epigenetic alterations in adult ovarian genes. Endocrinology. (2009) 150:4681–91. doi: 10.1210/en.2009-0499
49. Dutta S, Sivakumar KK, Erwin JW, Stanley JA, Arosh JA, Taylor RJ, et al. Alteration of epigenetic methyl and acetyl marks by postnatal chromium(VI) exposure causes apoptotic changes in the ovary of the F1 offspring. Reprod Toxicol. (2024) 123:108492. doi: 10.1016/j.reprotox.2023.108492
50. Zama AM, Uzumcu M. Targeted genome-wide methylation and gene expression analyses reveal signaling pathways involved in ovarian dysfunction after developmental EDC exposure in rats1. Biol Reprod. (2013) 88:52. doi: 10.1095/biolreprod.112.104802
51. Martinez RM, Hauser R, Liang L, Mansur A, Adir M, Dioni L, et al. Urinary concentrations of phenols and phthalate metabolites reflect extracellular vesicle microRNA expression in follicular fluid. Environ Int. (2019) 123:20–8. doi: 10.1016/j.envint.2018.11.043
52. Barnett-Itzhaki Z, Knapp S, Avraham C, Racowsky C, Hauser R, Bollati V, et al. Association between follicular fluid phthalate concentrations and extracellular vesicle microRNAs expression. Hum Reprod. (2021) 36:1590–9. doi: 10.1093/humrep/deab063
53. Björvang RD, Damdimopoulou P. Persistent environmental endocrine-disrupting chemicals in ovarian follicular fluid and in vitro fertilization treatment outcome in women. Ups J Med Sci. (2020) 125:85–94. doi: 10.1080/03009734.2020.1727073
54. Siddiqui S, Mateen S, Ahmad R, Moin S. A brief insight into the etiology, genetics, and immunology of polycystic ovarian syndrome (PCOS). J Assist Reprod Genet. (2022) 39:2439–73. doi: 10.1007/s10815-022-02625-7
55. Urbanetz LAML, Junior JMS, Maciel GAR, Simões RDS, Baracat MCP, Baracat EC. Does bisphenol A (BPA) participates in the pathogenesis of Polycystic Ovary Syndrome (PCOS)? Clinics. (2023) 78:100310. doi: 10.1016/j.clinsp.2023.100310
56. Zhan W, Tang W, Shen X, Xu H, Zhang J. Exposure to bisphenol A and its analogs and polycystic ovarian syndrome in women of childbearing age: A multicenter case-control study. Chemosphere. (2023) 313:137463. doi: 10.1016/j.chemosphere.2022.137463
57. Jin Y, Zhang Q, Pan J-X, Wang F-F, Qu F. The effects of di(2-ethylhexyl) phthalate exposure in women with polycystic ovary syndrome undergoing in vitro fertilization. J Int Med Res. (2019) 47:6278–93. doi: 10.1177/0300060519876467
58. Alonso-Magdalena P, Ropero AB, Soriano S, García-Arévalo M, Ripoll C, Fuentes E, et al. Bisphenol-A acts as a potent estrogen via non-classical estrogen triggered pathways. Mol Cell Endocrinol. (2012) 355:201–7. doi: 10.1016/j.mce.2011.12.012
59. Mahoney MM, Padmanabhan V. Developmental programming: impact of fetal exposure to endocrine-disrupting chemicals on gonadotropin-releasing hormone and estrogen receptor mRNA in sheep hypothalamus. Toxicol Appl Pharmacol. (2010) 247:98–104. doi: 10.1016/j.taap.2010.05.017
60. Gámez JM, Penalba R, Cardoso N, Bernasconi PS, Carbone S, Ponzo O, et al. Exposure to a low dose of bisphenol A impairs pituitary-ovarian axis in prepubertal rats: effects on early folliculogenesis. Environ Toxicol Pharmacol. (2015) 39:9–15. doi: 10.1016/j.etap.2014.10.015
61. Ma S, Shi W, Wang X, Song P, Zhong X. Bisphenol A exposure during pregnancy alters the mortality and levels of reproductive hormones and genes in offspring mice. BioMed Res Int. (2017) 2017:3585809. doi: 10.1155/2017/3585809
62. Zhou W, Liu J, Liao L, Han S, Liu J. Effect of bisphenol A on steroid hormone production in rat ovarian theca-interstitial and granulosa cells. Mol Cell Endocrinol. (2008) 283:12–8. doi: 10.1016/j.mce.2007.10.010
63. Wang Y, Zhu Q, Dang X, He Y, Li X, Sun Y. Local effect of bisphenol A on the estradiol synthesis of ovarian granulosa cells from PCOS. Gynecol Endocrinol. (2017) 33:21–5. doi: 10.1080/09513590.2016.1184641
64. Davis BJ, Maronpot RR, Heindel JJ. Di-(2-ethylhexyl) phthalate suppresses estradiol and ovulation in cycling rats. Toxicol Appl Pharmacol. (1994) 128:216–23. doi: 10.1006/taap.1994.1200
65. Lovekamp-Swan T, Davis BJ. Mechanisms of phthalate ester toxicity in the female reproductive system. Environ Health Perspect. (2003) 111:139–45. doi: 10.1289/ehp.5658
66. Heffernan AL, Cunningham TK, Drage DS, Aylward LL, Thompson K, Vijayasarathy S, et al. Perfluorinated alkyl acids in the serum and follicular fluid of UK women with and without polycystic ovarian syndrome undergoing fertility treatment and associations with hormonal and metabolic parameters. Int J Hyg Environ Health. (2018) 221:1068–75. doi: 10.1016/j.ijheh.2018.07.009
67. Pollack AZ, Krall JR, Kannan K, Buck Louis GM. Adipose to serum ratio and mixtures of persistent organic pollutants in relation to endometriosis: findings from the ENDO Study. Environ Res. (2021) 195:110732. doi: 10.1016/j.envres.2021.110732
68. Matta K, Lefebvre T, Vigneau E, Cariou V, Marchand P, Guitton Y, et al. Associations between persistent organic pollutants and endometriosis: a multiblock approach integrating metabolic and cytokine profiling. Environ Int. (2022) 158:106926. doi: 10.1016/j.envint.2021.106926
69. Kim MG, Min YS, Ahn YS. Does exposure of lead and cadmium affect the endometriosis? Int J Environ Res Public Health. (2021) 18:9077. doi: 10.3390/ijerph18179077
70. Hammarstrand S, Jakobsson K, Andersson E, Xu Y, Li Y, Olovsson M, et al. Perfluoroalkyl substances (PFAS) in drinking water and risk for polycystic ovarian syndrome, uterine leiomyoma, and endometriosis: a Swedish cohort study. Environ Int. (2021) 157:106819. doi: 10.1016/j.envint.2021.106819
71. Buoso E, Masi M, Racchi M, Corsini E. Endocrine-disrupting chemicals' (EDCs) effects on tumour microenvironment and cancer progression: emerging contribution of RACK1. Int J Mol Sci. (2020) 21:9229. doi: 10.3390/ijms21239229
72. Boivin J, Bunting L, Collins JA, Nygren KG. International estimates of infertility prevalence and treatment-seeking: potential need and demand for infertility medical care. Hum Reprod. (2007) 22:1506–12. doi: 10.1093/humrep/dem046
73. Sunderam S, Kissin DM, Zhang Y, Folger SG, Boulet SL, Warner L, et al. Assisted reproductive technology surveillance — United state. MMWR Surveill Summ. (2019) 68:1–23. doi: 10.15585/mmwr.ss6804a1
74. Zeng XW, Bloom MS, Wei F, Liu L, Qin J, Xue L, et al. Perfluoroalkyl acids in follicular fluid and embryo quality during IVF: A prospective IVF cohort in China. Environ Health Perspect. (2023) 131:27002. doi: 10.1289/EHP10857
75. Yao W, Liu C, Qin DY, Yuan XQ, Yao QY, Li NJ, et al. Associations between phthalate metabolite concentrations in follicular fluid and reproductive outcomes among women undergoing in vitro fertilization/intracytoplasmic sperm injection treatment. Environ Health Perspect. (2023) 131:127019. doi: 10.1289/EHP11998
76. Poormoosavi SM, Behmanesh MA, Janati S, Najafzadehvarzi H. Level of bisphenol A in follicular fluid and serum and oocyte morphology in patients undergoing IVF treatment. J Family Reprod Health. (2019) 13:154–9. doi: 10.18502/jfrh.v13i3.2129
77. Younglai E, Foster W, Hughes E, Hughes EG, Trim K, Jarrell JF. Levels of environmental contaminants in human follicular fluid, serum, and seminal plasma of couples undergoing in vitro fertilization. Arch Environ Contam. Toxicol. (2002) 43:121–6. doi: 10.1007/s00244-001-0048-8
78. Huang Y, Yan M, Nie H, Wang W, Wang J. Persistent halogenated organic pollutants in follicular fluid of women undergoing in vitro fertilization from China: Occurrence, congener profiles, and possible sources. Environ pollut. (2019) 244:1–8. doi: 10.1016/j.envpol.2018.09.134
79. Bullach A, Trapphoff T, Zühlke S, Spiteller M, Dieterle S. Impact of nonylphenols and polyhalogenated compounds in follicular fluid on the outcome of intracytoplasmic sperm injection. Reprod Sci. (2021) 28:2118–28. doi: 10.1007/s43032-021-00472-y
80. Deng T, Du Y, Wang Y, Teng X, Hua X, Yuan X, et al. The associations of urinary phthalate metabolites with the intermediate and pregnancy outcomes of women receiving IVF/ICSI treatments: A prospective single-center study. Ecotoxicol Environ Saf. (2020) 188:109884. doi: 10.1016/j.ecoenv.2019.109884
81. Van Duursen MBM, Boberg J, Christiansen S, Connolly L, Damdimopoulou P, Filis P, et al. Safeguarding female reproductive health against endocrine disrupting chemicals—The FREIA project. IJMS. (2020) 21:3215. doi: 10.3390/ijms21093215
82. Parikh FR, Uttamchandani S, Sawkar S, Panpalia M, Naik N, Sinkar P, et al. The impact of follicular fluid phthalate metabolites on the ovarian reserve and ovarian function in Indian women undergoing intracytoplasmic sperm injection. F S Sci. (2024) 5:107–20. doi: 10.1016/j.xfss.2023.11.001
83. Bellavia A, Zou R, Björvang RD, Roos K, Sjunnesson Y, Hallberg I, et al. Association between chemical mixtures and female fertility in women undergoing assisted reproduction in Sweden and Estonia. Environ Res. (2023) 216:114447. doi: 10.1016/j.envres.2022.114447
84. Tian T, Hao Y, Wang Y, Xu X, Long X, Yan L, et al. Mixed and single effects of endocrine disrupting chemicals in follicular fluid on likelihood of diminished ovarian reserve: A case-control study. Chemosphere. (2023) 330:138727. doi: 10.1016/j.chemosphere.2023.138727
85. Al-Hussaini TK, Abdelaleem AA, Elnashar I, Shabaan OM, Mostafa R, El-Baz MAH, et al. The effect of follicullar fluid pesticides and polychlorinated biphenyls concentrations on intracytoplasmic sperm injection (ICSI) embryological and clinical outcome. Eur J Obstet Gynecol Reprod Biol. (2018) 220:39–43. doi: 10.1016/j.ejogrb.2017.11.003
86. Žalmanová T, Hošková K, Prokešová Š, Nevoral J, Ješeta M, Benc M, et al. The bisphenol S contamination level observed in human follicular fluid affects the development of porcine oocytes. Front Cell Dev Biol. (2023) 11:1145182. doi: 10.3389/fcell.2023.1145182
87. Mínguez-Alarcón L, Gaskins AJ, Chiu YH, Williams PL, Ehrlich S, Chavarro JE, et al. Urinary bisphenol A concentrations and association with in vitro fertilization outcomes among women from a fertility clinic. Hum Reprod (Oxford England). (2015) 30:2120–8. doi: 10.1093/humrep/dev183
88. Hunt PA, Koehler KE, Susiarjo M, Hodges CA, Ilagan A, Voigt RC, et al. Bisphenol a exposure causes meiotic aneuploidy in the female mouse. Curr biology: CB. (2003) 13:546–53. doi: 10.1016/S0960-9822(03)00189-1
89. Beck AL, Rehfeld A, Mortensen LJ, Lorenzen M, Andersson AM, Juul A, et al. Ovarian follicular fluid levels of phthalates and benzophenones in relation to fertility outcomes. Environ Int. (2024) 183:108383. doi: 10.1016/j.envint.2023.108383
90. Aylward LL, Hays SM, Kirman CR, Marchitti SA, Kenneke JF, English C, et al. Relationships of chemical concentrations in maternal and cord blood: a review of available data. J Toxicol Environ Health Part B Crit Rev. (2014) 17:175–203. doi: 10.1080/10937404.2014.884956
91. Rattan S, Brehm E, Gao L, Flaws JA. Di(2-ethylhexyl) phthalate exposure during prenatal development causes adverse transgenerational effects on female fertility in mice. Toxicological Sci. (2018) 163:420–9. doi: 10.1093/toxsci/kfy042
92. Kaimal A, Hooversmith JM, Cherry AD, Garrity JT, Al Mansi MH, Martin NM, et al. Prenatal exposure to bisphenol A and/or diethylhexyl phthalate alters stress responses in rat offspring in a sex- and dose-dependent manner. Front Toxicol. (2023) 5:1264238. doi: 10.3389/ftox.2023.1264238
93. Ziv-Gal A, Wang W, Zhou C, Flaws JA. The effects of in utero bisphenol A exposure on reproductive capacity in several generations of mice. Toxicol Appl Pharmacol. (2015) 284:354–62. doi: 10.1016/j.taap.2015.03.003
94. Shi M, Whorton AE, Sekulovski N, MacLean JA, Hayashi K. Prenatal exposure to bisphenol A, E, and S induces transgenerational effects on female reproductive functions in mice. Toxicological Sci. (2019) 170:320–9. doi: 10.1093/toxsci/kfz124
95. Mustieles V, Zhang Y, Yland J, Braun JM, Williams PL, Wylie BJ, et al. Maternal and paternal preconception exposure to phenols and preterm birth. Environ Int. (2020) 137:105523. doi: 10.1016/j.envint.2020.105523
96. Swan SH, Sathyanarayana S, Barrett ES, Janssen S, Liu F, Nguyen RH, et al. First trimester phthalate exposure and anogenital distance in newborns. Hum Reprod (Oxford England). (2015) 30:963–72. doi: 10.1093/humrep/deu363
97. Hart RJ, Frederiksen H, Doherty DA, Keelan JA, Skakkebaek NE, Minaee NS, et al. The possible impact of antenatal exposure to ubiquitous phthalates upon male reproductive function at 20 Years of age. Front Endocrinol. (2018) 9:288. doi: 10.3389/fendo.2018.00288
98. Vrijheid M, Armstrong B, Dolk H, van Tongeren M, Botting B. Risk of hypospadias in relation to maternal occupational exposure to potential endocrine disrupting chemicals. Occup Environ Med. (2003) 60:543–50. doi: 10.1136/oem.60.8.543
Keywords: endocrine-disrupting chemicals, EDCs, follicular fluid, female fertility, ovary, oocytes
Citation: Shulhai A-M, Bianco V, Donini V, Esposito S and Street ME (2024) Which is the current knowledge on man-made endocrine- disrupting chemicals in follicular fluid? An overview of effects on ovarian function and reproductive health. Front. Endocrinol. 15:1435121. doi: 10.3389/fendo.2024.1435121
Received: 19 May 2024; Accepted: 11 September 2024;
Published: 02 October 2024.
Edited by:
Signe Altmäe, University of Granada, SpainReviewed by:
Nisha Thomas, University of Oklahoma, United StatesAlka Gupta, University of California, Santa Cruz, United States
Copyright © 2024 Shulhai, Bianco, Donini, Esposito and Street. This is an open-access article distributed under the terms of the Creative Commons Attribution License (CC BY). The use, distribution or reproduction in other forums is permitted, provided the original author(s) and the copyright owner(s) are credited and that the original publication in this journal is cited, in accordance with accepted academic practice. No use, distribution or reproduction is permitted which does not comply with these terms.
*Correspondence: Maria Elisabeth Street, bWFyaWFlbGlzYWJldGguc3RyZWV0QHVuaXByLml0